- Department of Biomedical Sciences and Pathobiology, Virginia-Maryland College of Veterinary Medicine, Virginia Tech, Blacksburg, VA, United States
Lactobacillus reuteri (L. reuteri) is a well-studied probiotic bacterium that can colonize a large number of mammals. In humans, L. reuteri is found in different body sites, including the gastrointestinal tract, urinary tract, skin, and breast milk. The abundance of L. reuteri varies among different individuals. Several beneficial effects of L. reuteri have been noted. First, L. reuteri can produce antimicrobial molecules, such as organic acids, ethanol, and reuterin. Due to its antimicrobial activity, L. reuteri is able to inhibit the colonization of pathogenic microbes and remodel the commensal microbiota composition in the host. Second, L. reuteri can benefit the host immune system. For instance, some L. reuteri strains can reduce the production of pro-inflammatory cytokines while promoting regulatory T cell development and function. Third, bearing the ability to strengthen the intestinal barrier, the colonization of L. reuteri may decrease the microbial translocation from the gut lumen to the tissues. Microbial translocation across the intestinal epithelium has been hypothesized as an initiator of inflammation. Therefore, inflammatory diseases, including those located in the gut as well as in remote tissues, may be ameliorated by increasing the colonization of L. reuteri. Notably, the decrease in the abundance of L. reuteri in humans in the past decades is correlated with an increase in the incidences of inflammatory diseases over the same period of time. Direct supplementation or prebiotic modulation of L. reuteri may be an attractive preventive and/or therapeutic avenue against inflammatory diseases.
Introduction
Probiotics are defined as “live microorganisms which, when administered in adequate amounts, confer a health benefit on the host” by the World Health Organization. While the idea to use probiotics for health benefits is not new, the interest has significantly increased in recent years (Islam, 2016). This may be due, in part, to the increase in antibiotic resistance particularly in the treatment of diseases in the gastrointestinal (GI) system, as well as an increasing desire by the public for natural health promotants. Those probiotic microorganisms that have been shown to have beneficial properties include Lactobacillus spp., Bifidobacterium spp., Saccharomyces boulardii, Propionibacterium spp., Streptococcus spp., Bacillus spp., Enterococcus spp., and some specific strains of Escherichia coli (Kechagia et al., 2013).
There are certain criteria that a probiotic must have to be considered efficacious. These include the capacity to survive in the GI tract, a high resistance to gastric acids, the lack of any transferable antibiotic resistance genes, and the capacity to exert clear benefits in the host (Montalban-Arques et al., 2015). Probiotics promote a healthy body through diverse mechanisms. A widespread generalization describing common mechanisms among studied probiotic genera includes colonizing resistance, producing acid, and short chain fatty acid (SCFA), regulating intestinal transit, normalizing perturbed microbiota, increasing enterocyte turnover, and competitive exclusion of pathogens (Hill et al., 2014). Though not widely observed, there are a lot of effects among specific probiotic species, some being strain specific. For instance, some probiotic strains can improve host food digestion by metabolizing bile salt or complementing the functions of missing digestive enzymes (Amara and Shibl, 2015; Shi et al., 2016).
Lactobacillus spp. are one of the most widely used probiotics and can be found in a large variety of food products throughout the world (Giraffa et al., 2010). The genus Lactobacillus comprises a large heterogeneous group of Gram-positive, nonsporulating, facultative anaerobic bacteria which include L. acidophilus, L. rhamnosus, L. bulgaricus, L. casei, and L. reuteri. This genus plays a very important role in food fermentation and can also be found in the GI system of humans and animals in variable amounts depending on the species, age of the host, or location within the gut (Duar et al., 2017).
Animal studies and preclinical results have shown that Lactobacilli may help in the prevention and treatment of numerous GI tract disorders. Among these disorders are enteric infections, antibiotic-associated diarrhea, necrotizing enterocolitis in preterm neonates, inflammatory bowel disease, colorectal cancer, and irritable bowel syndrome (Lebeer et al., 2008). Although the GI tract is the site where Lactobacilli are believed to show the most benefits, probiotic applications of some Lactobacillus strains at other sites of the body have been reported. These include the prevention and treatment of urogenital diseases and bacterial vaginosis in women, atopic disease, food hypersensitivity, and the prevention of dental caries (Lebeer et al., 2008).
One species of Lactobacillus, L. reuteri has multiple beneficial effects on host health such as prevention and/or amelioration of diverse disorders. L. reuteri was first isolated in 1962. It has been characterized as heterofermentative species that grows in oxygen-limited atmospheres and colonizes the GI tract of humans and animals (Kandler et al., 1980). The fact that it normally colonizes the GI tract may be the reason it confers great probiotic properties. This organism can withstand a wide variety of pH environments, employs multiple mechanisms that allow it to successfully inhibit pathogenic microorganisms, and has been shown to secrete antimicrobial intermediaries (Jacobsen et al., 1999; Valeur et al., 2004).
L. reuteri has been shown to be one of the truly indigenous bacteria of the human GI tract (Sinkiewicz, 2010). It naturally colonizes a wide range of vertebrates, including pigs, rodents, and chickens. In fact, it has gone through long-term evolution to diversify into host-adapted lineages (Oh et al., 2010; Walter et al., 2011). This organism is most typically found in the proximal digestive tract of the host (Frese et al., 2013). Several studies have assessed the safety of this organism in adults, children, infants, and even in an HIV-infected population (Wolf et al., 1998; Valeur et al., 2004; Weizman and Alsheikh, 2006; Mangalat et al., 2012; Jones et al., 2012a,c; Hoy-Schulz et al., 2016). The results showed that a dose as high as 2.9 × 109 colony-forming units (cfu)/day was still well tolerated, safe, and efficacious in humans. There have also been numerous articles enumerating the benefits of L. reuteri as a probiotic. These benefits include promoting health, reducing infections, improving feed tolerance, enhancing the absorption of nutrients, minerals, and vitamins, modulating host immune responses, promoting gut mucosal integrity, and reducing bacterial translocation (Tubelius et al., 2005; McFall-Ngai, 2007; Indrio et al., 2008; Spinler et al., 2008; Hou et al., 2015). In the current review, we will focus on the particular probiotic, L. reuteri, and discuss its beneficial functions in promoting health and preventing infections and diverse diseases.
Probiotic Properties of L. reuteri
There are some prerequisites for becoming potential probiotics: to survive in low pH and enzyme-enriched environments, to adhere to epithelium for host-probiotic interaction, competition with pathogenic microorganisms, and most importantly, safety. L. reuteri meets all of these requirements. Here, additional probiotic properties of L. reuteri are discussed that contribute to its diverse beneficial effects on host health and disease prevention and/or amelioration (Figure 1).
Gut Colonization of L. reuteri
Built for digestion and absorption, some sites of the GI system have developed to be harsh for microorganism colonization. Examples of this can be seen in the low pH conditions caused by gastric acids and bile salts in upper small intestine. Thus, the very first step of colonizing the GI tract is to survive in such environments. Multiple L. reuteri stains are resistant to low pH and bile salts (Seo et al., 2010; Krumbeck et al., 2016). This resistance is believed to be at least partially dependent on its ability to form biofilms (Salas-Jara et al., 2016).
L. reuteri is capable of attaching to mucin and intestinal epithelia, and some strains can adhere to gut epithelial cells in a range of vertebrate hosts (Li et al., 2008; Hou et al., 2014, 2015). A possible mechanism for adherence is the binding of bacterial surface molecules to the mucus layer. Mucus-binding proteins (MUBs) and MUB-like proteins, encoded by Lactobacillales-specific clusters of orthologous protein coding genes, serve as adherence mediators, or so-called adhesins (Roos and Jonsson, 2002; Kleerebezem et al., 2010; Gunning et al., 2016). The considerable diversity of MUBs among L. reuteri strains and the variation in the abundance of cell-surface MUBs significantly correlates with their mucus binding ability (Mackenzie et al., 2010). The strain-specific role of MUBs in recognizing mucus elements and/or their capability of promoting aggregation can explain the contribution of MUBs on the adherence of L. reuteri. Factors that mediate the attachment to the surfaces include multiple large surface proteins (Walter et al., 2005; Wang et al., 2008; Frese et al., 2011), MUB A (Jensen et al., 2014), glucosyltransferase A (GtfA) and inulosucrase (Inu) (Walter et al., 2008), and D-alanyl ester (Walter et al., 2007).
As L. reuteri that has colonized to the host GI tract can form biofilms, efforts have been made to study the regulation of L. reuteri biofilm secretion and its association with the adherence of bacteria to host GI epithelium. By doing in vitro biofilm assay, Water, J. et al. uncovered the contribution of GtfA and Inu in the biofilm formation of L. reuteri TMW1.106 (Walter et al., 2008). The in vivo biofilm formation of L. reuteri strains seems to be dependent on the host origin of the strains. In one study, nine L. reuteri strains isolated from different hosts (human, mouse, rat, chicken, and pig) were given to germ-free mice and the biofilms were evaluated after 2 days. Interestingly, only rodent strains were able to form biofilms and adhere to the forestomach epithelium, although the luminal populations were comparable among strains of different origins (Frese et al., 2013). Another study by the same authors showed that a specialized transport pathway (the SecA2-SecY2 system) was unique in the rodent and porcine strains (Frese et al., 2011). By using a rodent strain L. reuteri 100-23, they compared extracellular and cell wall-associated proteins between the wild-type strain and the secA2 mutant. Only one surface protein, L. reuteri 70902, was absent in the secA2 mutant. In vivo colonization studies showed that the absence of L. reuteri 70902 leads to almost completely eliminated biofilm formation. This strongly suggests that L. reuteri 70902 and the SecA2-SecY2 system are key factors regulating biofilm production from L. reuteri 100-23 in germ-free mice (Frese et al., 2013). Another group investigated the role of two-component systems bfrKRT and cemAKR in in vitro biofilm formation of L. reuteri 100-23 (Su and Ganzle, 2014). They found the deletion of certain genes in the operons enhanced the adherence and biofilm formation. However, the contribution of the bfrKRT and cemAKR to in vivo biofilm formation remains to be elucidated. The role of exopolysaccharide (EPS) in assisting colonization was also examined with L. reuteri 100-23. The production of EPS was eliminated due to a mutation of the fructosyl transferase (ftf) gene (Sims et al., 2011). After administration to Lactobacillus-free mice, compared to the wild-type strain, the colonization of the ftf mutant in the forestomach and cecum was largely impaired. This indicates EPS production can enhance the colonizing ability of strain 100-23 in the gut. Interestingly, L. reuteri RC-14 has been demonstrated to be able to penetrate mature E. coli biofilm and become part of it (McMillan et al., 2011). Recently, L. reuteri was delivered as a biofilm on microsphere and such delivery was found to promote the adherence of L. reuteri to intestinal epithelium and enhance its probiotic property (Olson et al., 2016; Navarro et al., 2017).
Production of Metabolites With Health-Promoting Effect
The antimicrobial and immunomodulatory effects of L. reuteri strains are linked to their metabolite production profile. Here, we discuss a few well-studied metabolites with regard to the probiotic potential of L. reuteri.
Reuterin
Most L. reuteri strains of human and poultry lineage are able to produce and excrete reuterin, a well-known antimicrobial compound (Talarico et al., 1988; Talarico and Dobrogosz, 1989; Cadieux et al., 2008; Jones and Versalovic, 2009; Mishra et al., 2012; Greifova et al., 2017). Reuterin is a mixture of different forms of 3-hydroxypropionaldehyde (3-HPA) (Talarico and Dobrogosz, 1989). It is known that L. reuteri can metabolize glycerol to generate 3-HPA in a coenzyme B12-dependent, glycerol dehydratase-mediated reaction (Talarico and Dobrogosz, 1990; Chen and Chen, 2013). The production of 3-HPA has also been demonstrated in a few other bacterial species (Zhu et al., 2002; Raynaud et al., 2003; Yang et al., 2007). However, L. reuteri is unique in its ability to produce and secrete 3-HPA in a manner more than its bioenergetics requirement (Stevens et al., 2011). Moreover, the antimicrobial activity of reuterin seems to rely on the spontaneous conversion of 3-HPA to acrolein, a cytotoxic electrophile (Stevens and Maier, 2008; Engels et al., 2016). Reuterin can inhibit a wide range of microorganisms, mainly Gram-negative bacteria (Cleusix et al., 2007). Not surprisingly, most Lactobacillus species are resistant to reuterin, among which L. reuteri strains exert the most resistance (Jones and Versalovic, 2009; Mishra et al., 2012). In addition to its antimicrobial property, reuterin is able to conjugate heterocyclic amines, which also seems to be dependent on the formation of acrolein (Engels et al., 2016). This suggests acrolein is an essential compound in the activity of reuterin.
Apart from reuterin, several other antimicrobial substances, including lactic acid, acetic acid, ethanol, and reutericyclin, have been determined as products of some L. reuteri strains (Ganzle and Vogel, 2003; Burge et al., 2015; Gopi et al., 2015; Yang Y. et al., 2015; Greifova et al., 2017). With the synthesis of these substances, L. reuteri has been shown to be effective against a variety of GI bacterial infections. These infections include Helicobacter pylori, E. coli, Clostridium difficile, and Salmonella (Reid and Burton, 2002; Cherian et al., 2015; Abhisingha et al., 2017; Genis et al., 2017). One of the more notable illustrations of the efficacy of L. reuteri as a probiotic against infections is the use of L. reuteri to treat H. pylori. H. pylori infection is a major cause of chronic gastritis and peptic ulcers, as well as a risk factor for gastric malignancies (Franceschi et al., 2007; Lesbros-Pantoflickova et al., 2007; Park et al., 2007). The use of L. reuteri against H. pylori has been explored in many studies (Table 1). It has been suggested that L. reuteri works by competing with H. pylori and inhibiting its binding to glycolipid receptors (Mukai et al., 2002). The competition reduces the bacterial load of H. pylori and decreases the related symptoms (Lionetti et al., 2006; Francavilla et al., 2008). Some studies have shown that L. reuteri has the potential to completely eradicate H. pylori from the intestine (Ojetti et al., 2012). Importantly, L. reuteri is advantageous in the treatment of H. pylori as the supplementation eradicates the pathogen without causing the common side effects associated with antibiotic therapies (Francavilla et al., 2014).
A considerable amount of research has been done to determine the beneficial effects of L. reuteri against viruses and/or fungi. There is evidence showing the benefit of L. reuteri against pneumoviruses, circoviruses, rotaviruses, coxsackieviruses, and papillomaviruses (Shornikova et al., 1997a,b; Preidis et al., 2012; Ang et al., 2016; Brenner et al., 2016; Piyathilake et al., 2016; Karaffova et al., 2017). It has been suggested that L. reuteri ameliorates viral infection by regulating the microbiota and secreting metabolites that have antiviral components (Ang et al., 2016). Furthermore, some studies suggest that L. reuteri may have antifungal properties as well, where L. reuteri antagonizes, stops the growth of, and eventually kills various species of Candida (Jorgensen et al., 2017).
Histamine
A few strains of L. reuteri are able to convert the amino acid L-histidine, a dietary component, to the biogenic amine histamine (Diaz et al., 2016; Greifova et al., 2017). A human commensal bacterium, L. reuteri 6475 was used as the model strain for studying histamine in L. reuteri. J. Versalovic’s group reported that L. reuteri 6475-derived histamine suppressed tumor necrosis factor (TNF) production from stimulated human monocytes (Thomas et al., 2012). This suppression was dependent on the activation of histamine H2 receptor, increased intracellular cAMP and protein kinase A, and the inhibition of MEK/ERK signaling. The production of histamine and subsequent in vitro TNF-suppressing function are regulated by a complete chromosomal histidine decarboxylase (hdc) gene cluster, which contains hdcA, hdcB, and hdcP (Rossi et al., 2011; Thomas et al., 2012). The same group of researchers also found that oral administration of hdc+ L. reuteri could effectively suppress intestinal inflammation in a trinitrobenzene sulfonic acid (TNBS)-induced mouse colitis model (Gao et al., 2015). Moreover, intraperitoneal injection of L. reuteri 6475 culture supernatant to TNBS-treated mice resulted in similar colitis attenuation. These results strongly indicate the involvement of L. reuteri metabolites, including histamine, in intestinal immunomodulation (Thomas et al., 2016). Further investigations showed that a gene called rsiR was necessary for the expression of hdc gene cluster in L. reuteri 6475 (Hemarajata et al., 2013). Inactivation of rsiR gene led to reduced TNF inhibition in vitro and diminished anti-inflammatory function in vivo. Additionally, both the in vitro TNF suppression and the in vivo anti-colitis effects appear to be regulated by a gene named folC2 (Thomas et al., 2016). Inactivation of folC2 gene resulted in suppression of the hdc gene cluster and diminished histamine production. Notably, histamine production by L. reuteri is highly strain-dependent, and most studies have been focused on strains of human origin (Mishra et al., 2012).
Vitamins
There are 13 essential vitamins for humans due to the inability of the human body to synthesize them (Linares et al., 2017). Like many other Lactobacillus spp., several L. reuteri strains are able to produce different types of vitamins, including vitamin B12 (cobalamin) and B9 (folate). As mentioned earlier, B12 is vital in reuterin production because the reduction of glycerol to 3-HPA requires a B12-dependent coenzyme. Up to now, at least 4 L. reuteri strains with various origins have been found to produce B12 (Taranto et al., 2003; Santos et al., 2008b; Sriramulu et al., 2008; Gu et al., 2015). Among these strains, L. reuteri CRL1098 and L. reuteri JCM1112 are the most studied (Morita et al., 2008; Santos et al., 2008a, 2011). In one study, the administration of L. reuteri CRL1098 together with a diet lacking vitamin B12 was shown to ameliorate pathologies in B12-deficient pregnant female mice and their offspring (Molina et al., 2009). This clearly points to the potential application of L. reuteri in treating B12 deficiency. In addition to B12, folate can also be synthesized by some specific L. reuteri strains, including L. reuteri 6475 and L. reuteri JCM1112 (Santos et al., 2008b; Thomas et al., 2016).
Exopolysaccharide (EPS)
The EPS produced by L. reuteri is important for biofilm formation and adherence of L. reuteri to epithelial surfaces (Salas-Jara et al., 2016). In addition, EPS synthesized by L. reuteri is able to inhibit E. coli adhesion to porcine epithelial cells in vitro (Ksonzekova et al., 2016). More importantly, EPS-mediated blocking of adhesion also suppresses gene expression of pro-inflammatory cytokines that are induced by E. coli infection, including IL-1β and IL-6. Further in vivo experiments in piglets showed similar results in that EPS originated from L. reuteri prevented piglet diarrhea in bacterial infection by reducing the adhesion of E. coli (Chen et al., 2014). In addition, EPS of L. reuteri origin has been reported to suppress the binding of enterotoxigenic E. coli to porcine erythrocytes (Wang et al., 2010). EPS produced by rodent L. reuteri 100-23 was also demonstrated to induce Foxp3+ regulatory T (Treg) cells in the spleen (Sims et al., 2011). In contrast, an L. reuteri 100-23 strain with the ftf mutation that eliminates EPS production from L. reuteri did not induce splenic Treg cells. This suggests that EPS is required for the L. reuteri-mediated induction of Treg cells and indicates the potential of using wild-type L. reuteri 100-23 to treat Treg deficiency.
L. reuteri-Mediated Modulation of Host Microbiota
Emerging evidence suggests that the host microbiota and immune system interact to maintain tissue homeostasis in healthy individuals (Kamada et al., 2013; Bene et al., 2017). Many diseases have been associated with perturbation of the microbiota (Mu et al., 2015), whereas restoration of the microbiota has been demonstrated to prevent or ameliorate several diseases (Scott et al., 2015). L. reuteri is able to influence the diversity, composition and metabolic function of the gut, oral, and vaginal microbiotas. These effects are largely strain-specific (Yang Y. et al., 2015; Garcia Rodenas et al., 2016; Galley et al., 2017; Su et al., 2017).
Gut Microbiota
Studies have shown the modulatory effects of L. reuteri on the microbiotas of rodents, piglets, and humans. One study assessed oral administration of a human-origin L. reuteri strain (DSM17938) to scurfy mice, which have gut microbial dysbiosis due to the foxp3 gene mutation. The results indicated that this strain of L. reuteri was able to prolong the lifespan of the mice and reduce multi-organ inflammation while remodeling the gut microbiota (He et al., 2017). Changes of gut microbiota included increases in the phylum Firmicutes and the genera Lactobacillus and Oscilospira. Notably, the disease-ameliorating effect of L. reuteri was attributed to the remodeled gut microbiota, though the community composition was still distinct from wild-type littermates. Further investigation showed that inosine production was enhanced by the gut microbiota upon L. reuteri administration. Through adenosine A2A receptor engagement, inosine can reduce Th1/Th2 cells and their associated cytokines. These results suggested that the L. reuteri – gut microbiota – inosine – adenosine A2A receptor axis serves as a potential therapeutic method for Treg-deficient disorders. Moreover, oral L. reuteri 6475 treatment led to a higher diversity of microbiota in both jejunum and ileum in an ovariectomy-induced bone loss mouse model (Britton et al., 2014). Specifically, there were more abundant Clostridiales but less Bacteriodales. However, whether or not the changed gut microbiota was directly associated with the prevention of bone loss requires further investigation. Furthermore, L. reuteri C10-2-1 has been shown to modulate the diversity of gut microbiota in the ileum of rats (Wang P. et al., 2016).
Compared to vaginally delivered infants, Cesarean (C)-section delivered infants display a higher abundance of Enterobacter but less Bifidobacterium in their gut microbiota (Garcia Rodenas et al., 2016; Nagpal et al., 2016). In one study, treating C-section babies with L. reuteri DSM 17938 from 2 weeks to 4 months of age modulated the development of gut microbiota toward the community pattern found in vaginally delivered infants (Garcia Rodenas et al., 2016). The gut microbiota structure of vaginally born infants remained unaltered upon L. reuteri supplementation. In another study, treating infants with the same L. reuteri strain resulted in decreased anaerobic Gram-negative and increased Gram-positive bacterial counts in gut microbiota, whereas the abundances of Enterobacteriaceae and Enterococci were largely lowered by L. reuteri treatment (Savino et al., 2015b). The differences in infant age, duration of treatment, route of administration, and dosage may explain the differences in results from the two studies.
For human adults, L. reuteri NCIMB 30242 administered as delayed release capsules for 4 weeks was able to increase the ratio of Firmicutes to Bacteroidetes in healthy individuals (Martoni et al., 2015). This strain of L. reuteri is known to be able to activate bile salt hydrolase and its effect in increasing circulating bile acid has been reported (Jones et al., 2012b). The upregulation of circulating bile acid has been proposed as a reason for the modulated gut microbiota (Jones et al., 2012b). In type 2 diabetes patients, although 3 months of L. reuteri DSM 17938 supplementation did not significantly change the gut microbial structure, the disease outcome of L. reuteri treatment was highly correlated with the baseline gut microbiota structure of individuals (Mobini et al., 2017). Furthermore, the administration of L. reuteri DSM 17938 in cystic fibrosis (CF) patients rescued gut microbiota dysbiosis by reducing Proteobacteria while also enhancing the relative abundance of Firmicutes (del Campo et al., 2014). However, whether or not the modulated gut microbiota contributed to improved GI health in probiotic-treated CF patients needs to be explored further.
L. reuteri influences the gut microbial community in piglets in a strain-specific manner. For instance, oral L. reuteri ZLR003 administration was able to change both the diversity and the composition of the gut microbiota (Zhang et al., 2016). However, treatment with the I5007 strain did not affect colonic microbial structure in piglets (Liu H. et al., 2017). In another study, fodder fermented with L. reuteri changed the abundances of 6 different bacterial taxa, particularly the family Enterobacteriacae, in weanling pigs (Yang Y. et al., 2015). However, the major alterations including increased Mitsuokella and decreased a family under phylum Bacteroidetes could only be seen with L. reuteri TMW1.656 rather than L. reuteri LTH5794. TMW1.656 is a reutericyclin-producing strain while LTH5794 is not, suggesting the possible contribution of reutericyclin in modulating gut microbiota in piglets (Yang Y. et al., 2015).
Oral Microbiota
The phyla Firmicutes, Bacteroidetes, Fusobacteria, Proteobacteria, and Actinobacteria are most abundant in the human oral microbiome (Romani Vestman et al., 2015). In a randomized controlled trial, 12 weeks of daily consumption of two L. reuteri strains – DSM 17938 and PTA 5289 led to a shift in oral microbiota composition, though the bacterial species richness was not altered (Romani Vestman et al., 2015). The alterations disappeared 4 weeks after the treatments were terminated, suggesting the fast turnover of the oral microbiome. In another human study, oral L. reuteri treatment reduced the amount of periodontal pathogens in the subgingival microbiota, though no clinical impact was seen (Iniesta et al., 2012).
Vaginal Microbiota
Lactobacilli dominate the vaginal bacterial community in healthy women (Macklaim et al., 2015). One study showed that only 14 days of oral L. reuteri RC-14 administration could restore the normal vaginal flora in postmenopausal women (Petricevic et al., 2008). Interestingly, the relative abundance of Lactobacilli is largely decreased in bacterial vaginosis patients (Macklaim et al., 2015). A total of 4 weeks of oral capsule consumption of two Lactobacilli strains including L. reuteri RC-14 increased the relative abundance of Lactobacilli. A similar increase of Lactobacilli was seen when L. reuteri RC-14 was administered vaginally together with a L. rhamnosus strain (Bisanz et al., 2014). However, in pregnant women, 8 weeks of oral L. reuteri RC-14 treatment did not efficiently restore the normal vaginal microbiota (Gille et al., 2016). This suggests that L. reuteri RC-14 may not be able to act alone.
Role of L. reuteri in Immunomodulation
Lactobacillus reuteri is able to increase free secretory IgA (sIgA) levels in rats (Wang P. et al., 2016). However, the upregulation of sIgA was eliminated in vitamin A-deficient rats, suggesting that L. reuteri functions in a vitamin A-dependent manner. In pregnant women, the intake of L. reuteri did not alter the levels of total IgA or sIgA in breast milk (Bottcher et al., 2008). When it comes to the effect of L. reuteri in inducing salivary IgA, the results are controversial. Increased salivary IgA levels were reported in humans’ chewing gum containing L. reuteri (Ericson et al., 2013). However, other studies showed that L. reuteri did not affect IgA concentration in saliva (Garofoli et al., 2014; Jorgensen et al., 2016; Braathen et al., 2017). The difference in the strains of L. reuteri used in the studies may explain the difference in results. Notably, an important commonality is that salivary L. reuteri-positive individuals have higher salivary IgA levels. Whether L. reuteri affects IgA levels by directly regulating B cells requires further investigations.
Many studies have shown that L. reuteri can induce anti-inflammatory Treg cells, which likely contributes to the beneficial effects of L. reuteri in a wide range of diseased and non-diseased conditions (Table 2). The Treg-inducing property of L. reuteri is largely strain-dependent. However, the anti-inflammatory effect of L. reuteri does not always rely on the induction of Treg cells. A good example is L. reuteri-mediated suppression of Th1/Th2 responses in Treg-deficient mice (He et al., 2017). Certain L. reuteri strains are able to reduce the production of many pro-inflammatory cytokines. For example, L. reuteri GMNL-263 can reduce serum MCP-1, TNF, and IL-6 levels in mice fed with high fat diet (Hsieh et al., 2016). Similar effects were observed in mice treated with heat-killed GMNL-263. However, in some cases, the immunomodulatory effects of L. reuteri appear to rely on its metabolites, as the culture supernatant of L. reuteri BM36301 could reduce TNF production from human myeloid THP-1 cells (Lee et al., 2016). Interestingly, tryptophan catabolites of L. reuteri have been recognized as ligands for aryl hydrocarbon receptor (AhR). Through activating AhR, L. reuteri can promote local IL-22 production from innate lymphoid cells (ILCs) (Zelante et al., 2013). In addition, the derivatives of tryptophan generated by L. reuteri can induce the development of regulatory CD4+CD8αα+ double-positive intraepithelial lymphocytes in an AhR-dependent manner (Cervantes-Barragan et al., 2017). Considering that AhR is ubiquitously expressed, L. reuteri and its metabolites may be able to influence many more types of immune cells beyond ILCs and T cells (Nguyen et al., 2013).
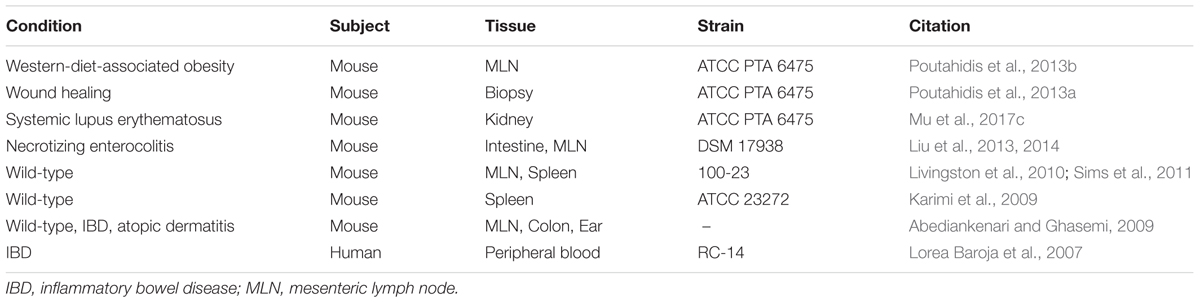
TABLE 2. L. reuteri-mediated induction of Treg cells under various diseased and non-diseased conditions.
Neuromodulatory Capability of L. reuteri
The intestinal microbiota plays a role in the functions of the enteric nervous system (ENS) (Yoo and Mazmanian, 2017). Subjects with microbiota depletion exhibit an abnormal ENS state (Anitha et al., 2012; Brun et al., 2013, 2015; Yoo and Mazmanian, 2017). Antibiotic treatment reduces the number of neurons in the ENS. This may be related to the decrease in Glial cell line-derived neurotrophic factor (GDNF), which can be restored by TLR2 stimulation (Brun et al., 2013). Moreover, germ-free animals show defective ENS morphology and excitability, which can be reversed by microbiota colonization (McVey Neufeld et al., 2013; Collins et al., 2014). L. reuteri, specifically, can prevent visceral pain response mainly by reducing the enteric nerve activity during the colorectal distension pressure in mice (Kamiya et al., 2006; Ma et al., 2009). Interestingly, live, heat-killed, gamma-irradiated L. reuteri, or even the conditioned media all had a similar effect (Kamiya et al., 2006). L. reuteri can also produce gamma-aminobutyric acid (GABA), the major inhibitory neurotransmitter in the central nervous system (Su et al., 2011; Barrett et al., 2012; Pallin et al., 2016). However, the in vivo bioactivity of the produced GABA has not been addressed (Yoo and Mazmanian, 2017). Furthermore, L. reuteri can increase the excitability and the number of action potentials in rat colonic sensory neurons (Kunze et al., 2009). These distinct effects of L. reuteri may be due to the difference in target neurons (Lai et al., 2017).
Role of L. reuteri in Reversing the Leaky Gut
Physical, biochemical, and immunological barriers comprise the gut barrier function, which is required to block the entry of exterior antigens and toxins (Mu et al., 2017a). If any abnormalities occur in the intestinal barrier, the permeability may increase resulting in a leaky gut. Various probiotics are known for their abilities to enhance mucosal barrier function, of which L. reuteri is a well-known example (Mu et al., 2017a). In DSS-induced colitis, L. reuteri administration could reduce bacterial translocation from the GI tract to the mesenteric lymph nodes (MLN) (Dicksved et al., 2012). In addition, treatment of lupus-prone mice with a mixture of Lactobacillus species including L. reuteri led to a higher expression of tight junction (TJ) proteins in intestinal epithelial cells (Mu et al., 2017c). Subsequently, the translocation of pro-inflammatory molecules such as LPS was significantly suppressed, which correlated with attenuated disease. In addition to mouse studies, several strains of L. reuteri have been shown to possess the ability to modulate TJ protein expression and maintain intestinal barrier integrity in pigs (Yang F. et al., 2015; Wang Z. et al., 2016). Moreover, the ability of L. reuteri to decrease intestinal permeability has been seen in humans. In children with atopic dermatitis, where the impairment of intestinal barrier function has been positively correlated with disease pathogenesis (De Benedetto et al., 2011), treatment with L. reuteri DSM 12246 (and L. rhamnosus 19070-2) significantly reduced the frequency of GI symptoms while decreasing the lactulose to mannitol ratio (Rosenfeldt et al., 2004), which reflects the reversal of a leaky gut (Camilleri et al., 2010).
L. reuteri Attenuate Human Diseases
A growing body of evidences links microbiota and bacterial translocation with multiple diseases, including several autoimmune disorders (Mu et al., 2015, 2017a). Due to its strong modulatory effects on host microbiota and immune responses with almost no safety concerns, L. reuteri is a good candidate for disease prevention and/or treatment. Indeed, the therapeutic potential of various L. reuteri strains has been studied in diverse diseases and the results are promising in many cases.
Early-Life Disorders
Taking advantage of the safety and tolerance of L. reuteri in infants and young children, a lot of efforts have been made to test the potential application of L. reuteri against disorders early in life (Table 3). In general, the results are promising. L. reuteri has been demonstrated beneficial in the prevention and/or treatment of many conditions including diarrhea, functional abdominal pain, caries, atopic dermatitis, allergy, feeding intolerance, and regurgitation. Infant colic, for example, has been the major therapeutic target of L. reuteri (Table 3). Infant colic is characterized by immoderate crying and affects 10–30% infants (Mi et al., 2015). The exact cause and efficient treatment of this condition have remained elusive. The clinical efficacy of L. reuteri DSM 17938 has been demonstrated as most of the clinical trials were successful (Table 3). The failure of some studies may be explained by the differences in the dosage of L. reuteri, the infant age when the studies initiated, or the baseline microbiota structure. It is worth mentioning that L. reuteri is naturally contained in human breast milk (Soto et al., 2014), though inconsistencies exist among individuals. The presence of L. reuteri in milk may complicate the results of studies that involved breastfeeding. When given during pregnancy, L. reuteri did not show a significant effect on allergy and eczema in infants after they were born (Table 3).
Systemic Lupus Erythematosus
The SLE is a multi-system autoimmune disease that involves both genetics and environment as the major disease causative factors (Tsokos, 2011; Edwards et al., 2017). The role of gut microbiota in SLE development was suggested by recent studies, and probiotics have been proposed as potential immunoregulators in SLE (Mu et al., 2015, 2017b; de Oliveira et al., 2017; Edwards et al., 2017; Esmaeili et al., 2017). We reported a significantly decreased level of Lactobacillaceae in lupus-prone MRL/lpr female mice compared to healthy control mice both before and after the disease initiated in MRL/lpr mice (Zhang et al., 2014). Moreover, we found that treatment with retinoic acid improved kidney disease in MRL/lpr mice, and that the improvement of lupus symptoms was associated with restoration of Lactobacilli. This suggests a possible beneficial effect of Lactobacilli in lupus. Therefore, we treated MRL/lpr mice with a mixture of five strains of Lactobacilli to determine their therapeutic benefit. As anticipated, increasing Lactobacilli in the gut improved renal function, reduced serum autoantibodies, and prolonged the survival of MRL/lpr mice (Mu et al., 2017c). Interestingly, L. reuteri and an uncultured Lactobacillus sp. accounted for > 99% of the observed effects. It suggests a central role of L. reuteri in attenuating lupus nephritis. Furthermore, we found that MRL/lpr mice had a “leaky” gut during disease progression, whereas Lactobacillus treatment enhanced the intestinal barrier function in these mice and subsequently decreased metabolic endotoxemia (Mu et al., 2017c). At the same time, the local and systemic pro- and anti-inflammatory network was rebalanced by Lactobacillus treatment. Specifically, IL-10 production was enhanced while the level of IL-6 was decreased systemically. Strikingly, the benefits of Lactobacilli were only observed in females and castrated males but not in intact males. Coincidently, the relative abundance of Lactobacilli in gut microbiota did not decrease as disease progressed in male MRL/lpr mice (Zhang et al., 2014). Consistent with our observations, daily consumption of L. reuteri BM36301 significantly lowered serum TNF level in females but not in males (Lee et al., 2016). The high serum level of testosterone in males may have led to the difference in the response to L. reuteri. Together, these results suggest possible interaction between sex hormones and gut microbiota in autoimmune disease development (Markle et al., 2013; Yurkovetskiy et al., 2013). Further investigation of this link is required. In another lupus mouse model, NZB/W F1, the administration of two L. reuteri strains, together with one L. paracasei strain, was shown to be effective in ameliorating lupus hepatitis (Hsu et al., 2017). Liver abnormalities, manifested as increased liver enzymes, portal inflammation and histopathological changes, have been observed in both lupus mouse models and SLE patients (Hsu et al., 2008; Grover et al., 2014). In this study, the oral L. reuteri treatment largely mitigated hepatic apoptosis and inflammation, suggesting a protective function of L. reuteri against lupus-associated liver disease (Hsu et al., 2017). The protection seems to rely on the capability of L. reuteri to increase antioxidant activity and reduce cytokines associated with more severe lupus, such as IL-6 and TNF (Tzang et al., 2017). Interestingly, within these two L. reuteri strains, only GMNL-263 can significantly promote the differentiation of Treg cells, again emphasizing the uneven immunoregulatory abilities of different L. reuteri strains.
Obesity
The correlation between gut microbiota and obesity is well documented (Okeke et al., 2014; Harakeh et al., 2016). The microbiota composition varies between lean and obese individuals, and a surprisingly high level of Lactobacillus spp. has been found in the microbiota of both obese adults and obese children (Armougom et al., 2009; Bervoets et al., 2013). Among different Lactobacillus spp., L. reuteri was specifically described to be associated with obesity (Million et al., 2012, 2013a). The association was further established when vancomycin-resistant L. reuteri in gut microbiota was determined as a body weight gain predictor during vancomycin treatment (Million et al., 2013b). However, in a randomized, double-blind and placebo-controlled clinical trial, the administration of L. reuteri JBD301 for 12 weeks significantly reduced body weight in overweight adults (Chung et al., 2016). Moreover, supplementation of infant formula with L. reuteri did not increase weight gain in infants (Braegger et al., 2011; Koleva et al., 2015). These conflicting results indicate that L. reuteri may influence the development of obesity in a strain-dependent manner. This hypothesis is partially verified in an animal study. In that study, three different strains of L. reuteri were used to test their influence on diet-induced obesity (Fåk and Bäckhed, 2012). It was demonstrated that only L. reuteri PTA 4659 efficiently reduced the body weight of mice fed with high-fat diet (HFD), whereas L. reuteri L6798-treated mice even gained some weight. The changes of adipose and liver weights were consistent with the body weight change.
In animal studies, several strains of L. reuteri have been reported to negatively regulate the development of obesity (Dahiya et al., 2017). In addition to the beneficial effect of L. reuteri JBD301 to human obese patients mentioned earlier, the favorable role of this strain of L. reuteri against weight gain was confirmed in HFD-fed mice (Chung et al., 2016). In HFD-induced obese mouse models, the beneficial role of L. reuteri GMNL-263 was also noted (Hsieh et al., 2016). Treatment with L. reuteri GMNL-263 reduced the body weight as well as the percentages of adipose tissue and liver to body weight. Interestingly, heat-killed GMNL-263 appeared to have a very similar beneficial function (Hsieh et al., 2016; Liao et al., 2016). L. reuteri 6475 has also been shown to be beneficial against obesity in mice (Poutahidis et al., 2013b). The function of L. reuteri 6475 was suggested to be largely dependent on its capability to induce Treg cells without changing the gut microbial ecology. Furthermore, the weight loss properties of some reagents have been attributed to their abilities to increase L. reuteri in mice. Polymannuronic acid, for example, was able to increase the relative abundance of L. reuteri and significantly reduce HFD-induced body weight gain (Liu F. et al., 2017). Whether the increase of L. reuteri is the cause of weight loss requires further investigation.
Neurodevelopmental Disorder
Exposure to maternal obesity in utero increases the chance of neurodevelopmental disorders, such as autism spectrum disorder, in children (Connolly et al., 2016). In a recent mouse study, maternal HFD (MHFD) was shown to induce social deficits in the offspring (Buffington et al., 2016). The impaired social ability in GF mice was restored by fecal microbiota transplantation from offspring with maternal regular diet (MRD) but not MHFD, suggesting a potential role of microbiota in this process. Further analysis showed that the abundance of L. reuteri was reduced more than ninefold in the gut microbiome of MHFD vs. MRD offspring. The social defects in MHFD offspring were rescued by direct L. reuteri administration, suggesting an effect of L. reuteri in regulating neurodevelopment in MHFD mice. This regulatory function of L. reuteri was attributed to its capability to increase the level of oxytocin (Poutahidis et al., 2013a; Buffington et al., 2016). The results of these studies suggest a potential application of L. reuteri in the treatment of patients who suffer from neurodevelopmental disorders.
Stressor Exposure and Enteric Infection
The composition of gut microbiota shift when the host is exposed to stressors (Bailey et al., 2010; Galley et al., 2014). In C57BL/6 males, social stressors led to an altered intestinal microbiota composition, though there was no significant change in community diversity (Galley et al., 2014). Further analysis showed stressor-induced reductions in the families Porphyromonadaceae and Lactobacillaceae, especially in the genus Lactobacillus. Among Lactobacillus spp., L. reuteri was specifically measured and a lower abundance of L. reuteri was evident in stressor-exposed CD-1 mice but not C57BL/6 mice. In fact, the level of L. reuteri in C57BL/6 male mice was below the detection limit with or without stressor exposure (Galley et al., 2014). It is important to note that stressor exposure increased the severity of Citrobacter rodentium-induced inflammation in the gut (Bailey et al., 2010; Mackos et al., 2016). The colonization of C. rodentium was promoted by stressor exposure, which subsequently resulted in more severe colonic pathology and increased production of inflammatory cytokines and chemokines (Mackos et al., 2016). Further studies revealed that stressor-induced C. rodentium colitis was C-C motif chemokine ligand 2 (CCL2)-dependent. Interestingly, administration of L. reuteri ATCC 23272 was able to reverse stressor-induced C. rodentium infection, which also relied on CCL2 (Mackos et al., 2013, 2016). However, L. reuteri was not able to restore the gut microbiome altered by social stressors. This indicates that the beneficial effect of L. reuteri on stressor exposure and subsequent enteric infection is not microbiota-dependent (Galley et al., 2017).
Conclusion
There has been a decrease in the abundance of L. reuteri in humans in the past few decades likely caused by the modern lifestyle (Antibiotic use, western diet, improved hygiene). Such decrease coincides with higher incidences of inflammatory diseases over the same period of time. While evidence is lacking to establish the correlation, it may be helpful to increase L. reuteri colonization and/or facilitate its probiotic functions as a new and relatively safe strategy against inflammatory diseases. In addition, through direct regulation or indirect modulation via the host microbiota, L. reuteri plays an impressive role in eliminating infections and attenuating both GI diseases and diseases in remote tissues. The safety and tolerance of L. reuteri has been proven by the numerous clinical studies. There are multiple L. reuteri strains with different host origins, and many of the probiotic functions of L. reuteri are strain-dependent. Therefore, it may be advantageous to combine different strains of L. reuteri to maximize their beneficial effects.
Author Contributions
QM and VT wrote the first draft of the review. XL edited and finalized the manuscript.
Funding
This work was funded by NIH R15AR067418 and R01AR073240.
Conflict of Interest Statement
The authors declare that the research was conducted in the absence of any commercial or financial relationships that could be construed as a potential conflict of interest.
References
Abediankenari, S., and Ghasemi, M. (2009). Generation of immune inhibitory dendritic cells and CD4+T regulatory cells inducing by TGF-beta. Iran. J. Allergy Asthma Immunol. 8, 25–30.
Abhisingha, M., Dumnil, J., and Pitaksutheepong, C. (2017). Selection of potential probiotic Lactobacillus with inhibitory activity against Salmonella and fecal coliform bacteria. Probiotics Antimicrob. Proteins doi: 10.1007/s12602-017-9304-8 [Epub ahead of print].
Abrahamsson, T. R., Jakobsson, T., Bjorksten, B., Oldaeus, G., and Jenmalm, M. C. (2013). No effect of probiotics on respiratory allergies: a seven-year follow-up of a randomized controlled trial in infancy. Pediatr. Allergy Immunol. 24, 556–561. doi: 10.1111/pai.12104
Abrahamsson, T. R., Jakobsson, T., Bottcher, M. F., Fredrikson, M., Jenmalm, M. C., Bjorksten, B., et al. (2007). Probiotics in prevention of IgE-associated eczema: a double-blind, randomized, placebo-controlled trial. J. Allergy Clin. Immunol. 119, 1174–1180. doi: 10.1016/j.jaci.2007.01.007
Agustina, R., Kok, F. J., van de Rest, O., Fahmida, U., Firmansyah, A., Lukito, W., et al. (2012). Randomized trial of probiotics and calcium on diarrhea and respiratory tract infections in Indonesian children. Pediatrics 129, e1155–e1164. doi: 10.1542/peds.2011-1379
Amara, A. A., and Shibl, A. (2015). Role of probiotics in health improvement, infection control and disease treatment and management. Saudi Pharm. J. 23, 107–114. doi: 10.1016/j.jsps.2013.07.001
Ang, L. Y., Too, H. K., Tan, E. L., Chow, T. K., Shek, P. C., Tham, E., et al. (2016). Antiviral activity of Lactobacillus reuteri Protectis against Coxsackievirus A and Enterovirus 71 infection in human skeletal muscle and colon cell lines. Virol. J. 13:111. doi: 10.1186/s12985-016-0567-6
Anitha, M., Vijay-Kumar, M., Sitaraman, S. V., Gewirtz, A. T., and Srinivasan, S. (2012). Gut microbial products regulate murine gastrointestinal motility via Toll-like receptor 4 signaling. Gastroenterology 143, 1006.e4–1016.e4. doi: 10.1053/j.gastro.2012.06.034
Armougom, F., Henry, M., Vialettes, B., Raccah, D., and Raoult, D. (2009). Monitoring bacterial community of human gut microbiota reveals an increase in Lactobacillus in obese patients and Methanogens in anorexic patients. PLoS One 4:e7125. doi: 10.1371/journal.pone.0007125
Bailey, M. T., Dowd, S. E., Parry, N. M., Galley, J. D., Schauer, D. B., and Lyte, M. (2010). Stressor exposure disrupts commensal microbial populations in the intestines and leads to increased colonization by Citrobacter rodentium. Infect. Immun. 78, 1509–1519. doi: 10.1128/IAI.00862-09
Barrett, E., Ross, R. P., O’Toole, P. W., Fitzgerald, G. F., and Stanton, C. (2012). gamma-Aminobutyric acid production by culturable bacteria from the human intestine. J. Appl. Microbiol. 113, 411–417. doi: 10.1111/j.1365-2672.2012.05344.x
Bene, K., Varga, Z., Petrov, V. O., Boyko, N., and Rajnavolgyi, E. (2017). Gut microbiota species can provoke both inflammatory and tolerogenic immune responses in human dendritic cells mediated by retinoic acid receptor alpha ligation. Front. Immunol. 8:427. doi: 10.3389/fimmu.2017.00427
Bervoets, L., Van Hoorenbeeck, K., Kortleven, I., Van Noten, C., Hens, N., Vael, C., et al. (2013). Differences in gut microbiota composition between obese and lean children: a cross-sectional study. Gut Pathog. 5:10. doi: 10.1186/1757-4749-5-10
Bisanz, J. E., Seney, S., McMillan, A., Vongsa, R., Koenig, D., Wong, L., et al. (2014). A systems biology approach investigating the effect of probiotics on the vaginal microbiome and host responses in a double blind, placebo-controlled clinical trial of post-menopausal women. PLoS One 9:e104511. doi: 10.1371/journal.pone.0104511
Bottcher, M. F., Abrahamsson, T. R., Fredriksson, M., Jakobsson, T., and Bjorksten, B. (2008). Low breast milk TGF-beta2 is induced by Lactobacillus reuteri supplementation and associates with reduced risk of sensitization during infancy. Pediatr. Allergy Immunol. 19, 497–504. doi: 10.1111/j.1399-3038.2007.00687.x
Braathen, G., Ingildsen, V., Twetman, S., Ericson, D., and Jorgensen, M. R. (2017). Presence of Lactobacillus reuteri in saliva coincide with higher salivary IgA in young adults after intake of probiotic lozenges. Benef. Microbes 8, 17–22. doi: 10.3920/BM2016.0081
Braegger, C., Chmielewska, A., Decsi, T., Kolacek, S., Mihatsch, W., Moreno, L., et al. (2011). Supplementation of infant formula with probiotics and/or prebiotics: a systematic review and comment by the ESPGHAN committee on nutrition. J. Pediatr. Gastroenterol. Nutr. 52, 238–250. doi: 10.1097/MPG.0b013e3181fb9e80
Brenner, T. A., Rice, T. A., Anderson, E. D., Percopo, C. M., and Rosenberg, H. F. (2016). Immortalized MH-S cells lack defining features of primary alveolar macrophages and do not support mouse pneumovirus replication. Immunol. Lett. 172, 106–112. doi: 10.1016/j.imlet.2016.02.012
Britton, R. A., Irwin, R., Quach, D., Schaefer, L., Zhang, J., Lee, T., et al. (2014). Probiotic L. reuteri treatment prevents bone loss in a menopausal ovariectomized mouse model. J. Cell. Physiol. 229, 1822–1830. doi: 10.1002/jcp.24636
Brun, P., Giron, M. C., Qesari, M., Porzionato, A., Caputi, V., Zoppellaro, C., et al. (2013). Toll-like receptor 2 regulates intestinal inflammation by controlling integrity of the enteric nervous system. Gastroenterology 145, 1323–1333. doi: 10.1053/j.gastro.2013.08.047
Brun, P., Gobbo, S., Caputi, V., Spagnol, L., Schirato, G., Pasqualin, M., et al. (2015). Toll like receptor-2 regulates production of glial-derived neurotrophic factors in murine intestinal smooth muscle cells. Mol. Cell. Neurosci. 68, 24–35. doi: 10.1016/j.mcn.2015.03.018
Buffington, S. A., Di Prisco, G. V., Auchtung, T. A., Ajami, N. J., Petrosino, J. F., and Costa-Mattioli, M. (2016). Microbial reconstitution reverses maternal diet-induced social and synaptic deficits in offspring. Cell 165, 1762–1775. doi: 10.1016/j.cell.2016.06.001
Burge, G., Saulou-Berion, C., Moussa, M., Pollet, B., Flourat, A., Allais, F., et al. (2015). Diversity of Lactobacillus reuteri strains in converting glycerol into 3-hydroxypropionic acid. Appl. Biochem. Biotechnol. 177, 923–939. doi: 10.1007/s12010-015-1787-8
Cadieux, P., Wind, A., Sommer, P., Schaefer, L., Crowley, K., Britton, R. A., et al. (2008). Evaluation of reuterin production in urogenital probiotic Lactobacillus reuteri RC-14. Appl. Environ. Microbiol. 74, 4645–4649. doi: 10.1128/AEM.00139-08
Camilleri, M., Nadeau, A., Lamsam, J., Nord, S. L., Ryks, M., Burton, D., et al. (2010). Understanding measurements of intestinal permeability in healthy humans with urine lactulose and mannitol excretion. Neurogastroenterol. Motil. 22, e15–e26. doi: 10.1111/j.1365-2982.2009.01361.x
Cekola, P. L., Czerkies, L. A., Storm, H. M., Wang, M. H., Roberts, J., and Saavedra, J. M. (2015). Growth and tolerance of term infants fed formula with probiotic Lactobacillus reuteri. Clin. Pediatr. 54, 1175–1184. doi: 10.1177/0009922815574076
Cervantes-Barragan, L., Chai, J. N., Tianero, M. D., Di Luccia, B., Ahern, P. P., Merriman, J., et al. (2017). Lactobacillus reuteri induces gut intraepithelial CD4+CD8alphaalpha+ T cells. Science 357, 806–810. doi: 10.1126/science.aah5825
Chau, K., Lau, E., Greenberg, S., Jacobson, S., Yazdani-Brojeni, P., Verma, N., et al. (2015). Probiotics for infantile colic: a randomized, double-blind, placebo-controlled trial investigating Lactobacillus reuteri DSM 17938. J. Pediatr. 166, 74–78. doi: 10.1016/j.jpeds.2014.09.020
Chen, G., and Chen, J. (2013). A novel cell modification method used in biotransformation of glycerol to 3-HPA by Lactobacillus reuteri. Appl. Microbiol. Biotechnol. 97, 4325–4332. doi: 10.1007/s00253-013-4723-2
Chen, X. Y., Woodward, A., Zijlstra, R. T., and Ganzle, M. G. (2014). Exopolysaccharides synthesized by Lactobacillus reuteri protect against enterotoxigenic Escherichia coli in piglets. Appl. Environ. Microbiol. 80, 5752–5760. doi: 10.1128/AEM.01782-14
Cherian, P. T., Wu, X., Yang, L., Scarborough, J. S., Singh, A. P., Alam, Z. A., et al. (2015). Gastrointestinal localization of metronidazole by a lactobacilli-inspired tetramic acid motif improves treatment outcomes in the hamster model of Clostridium difficile infection. J. Antimicrob. Chemother. 70, 3061–3069. doi: 10.1093/jac/dkv231
Chung, H. J., Yu, J. G., Lee, I. A., Liu, M. J., Shen, Y. F., Sharma, S. P., et al. (2016). Intestinal removal of free fatty acids from hosts by Lactobacilli for the treatment of obesity. FEBS Open Bio 6, 64–76. doi: 10.1002/2211-5463.12024
Cleusix, V., Lacroix, C., Vollenweider, S., Duboux, M., and Le Blay, G. (2007). Inhibitory activity spectrum of reuterin produced by Lactobacillus reuteri against intestinal bacteria. BMC Microbiol. 7:101. doi: 10.1186/1471-2180-7-101
Collins, J., Borojevic, R., Verdu, E. F., Huizinga, J. D., and Ratcliffe, E. M. (2014). Intestinal microbiota influence the early postnatal development of the enteric nervous system. Neurogastroenterol. Motil. 26, 98–107. doi: 10.1111/nmo.12236
Connolly, N., Anixt, J., Manning, P., Ping-I Lin, D., Marsolo, K. A., and Bowers, K. (2016). Maternal metabolic risk factors for autism spectrum disorder-An analysis of electronic medical records and linked birth data. Autism Res. 9, 829–837. doi: 10.1002/aur.1586
Dahiya, D. K., Renuka, Puniya, M., Shandilya, U. K., Dhewa, T., Kumar, N., et al. (2017). Gut microbiota modulation and its relationship with obesity using prebiotic fibers and probiotics: a review. Front. Microbiol. 8:563. doi: 10.3389/fmicb.2017.00563
De Benedetto, A., Rafaels, N. M., McGirt, L. Y., Ivanov, A. I., Georas, S. N., Cheadle, C., et al. (2011). Tight junction defects in patients with atopic dermatitis. J. Allergy Clin. Immunol. 127, 771.e7–786.e7. doi: 10.1016/j.jaci.2010.10.018
de Oliveira, G. L. V., Leite, A. Z., Higuchi, B. S., Gonzaga, M. I., and Mariano, V. S. (2017). Intestinal dysbiosis and probiotic applications in autoimmune diseases. Immunology 152, 1–12. doi: 10.1111/imm.12765
del Campo, R., Garriga, M., Perez-Aragon, A., Guallarte, P., Lamas, A., Maiz, L., et al. (2014). Improvement of digestive health and reduction in proteobacterial populations in the gut microbiota of cystic fibrosis patients using a Lactobacillus reuteri probiotic preparation: a double blind prospective study. J. Cyst. Fibros. 13, 716–722. doi: 10.1016/j.jcf.2014.02.007
Diaz, M., Ladero, V., del Rio, B., Redruello, B., Fernandez, M., Martin, M. C., et al. (2016). Biofilm-forming capacity in biogenic amine-producing bacteria isolated from dairy products. Front. Microbiol. 7:591. doi: 10.3389/fmicb.2016.00591
Dicksved, J., Schreiber, O., Willing, B., Petersson, J., Rang, S., Phillipson, M., et al. (2012). Lactobacillus reuteri maintains a functional mucosal barrier during DSS treatment despite mucus layer dysfunction. PLoS One 7:e46399. doi: 10.1371/journal.pone.0046399
Dinleyici, E. C., Dalgic, N., Guven, S., Metin, O., Yasa, O., Kurugol, Z., et al. (2015). Lactobacillus reuteri DSM 17938 shortens acute infectious diarrhea in a pediatric outpatient setting. J. Pediatr. 91, 392–396. doi: 10.1016/j.jped.2014.10.009
Dinleyici, E. C., Probage Study Group, and Vandenplas, Y. (2014). Lactobacillus reuteri DSM 17938 effectively reduces the duration of acute diarrhoea in hospitalised children. Acta Paediatr. 103, e300–e305. doi: 10.1111/apa.12617
Dore, M. P., Cuccu, M., Pes, G. M., Manca, A., and Graham, D. Y. (2014). Lactobacillus reuteri in the treatment of Helicobacter pylori infection. Intern. Emerg. Med. 9, 649–654. doi: 10.1007/s11739-013-1013-z
Dore, M. P., Soro, S., Rocchi, C., Loria, M. F., Bibbo, S., and Pes, G. M. (2016). Inclusion of Lactobacillus reuteri in the treatment of Helicobacter pylori in Sardinian patients: a case report series. Medicine 95:e3411. doi: 10.1097/MD.0000000000003411
Duar, R. M., Lin, X. B., Zheng, J., Martino, M. E., Grenier, T., Perez-Munoz, M. E., et al. (2017). Lifestyles in transition: evolution and natural history of the genus Lactobacillus. FEMS Microbiol. Rev. 41(Suppl. 1), S27–S48. doi: 10.1093/femsre/fux030
Edwards, M. R., Dai, R., Heid, B., Cecere, T. E., Khan, D., Mu, Q., et al. (2017). Commercial rodent diets differentially regulate autoimmune glomerulonephritis, epigenetics and microbiota in MRL/lpr mice. Int. Immunol. 29, 263–276. doi: 10.1093/intimm/dxx033
Engels, C., Schwab, C., Zhang, J., Stevens, M. J., Bieri, C., Ebert, M. O., et al. (2016). Acrolein contributes strongly to antimicrobial and heterocyclic amine transformation activities of reuterin. Sci. Rep. 6:36246. doi: 10.1038/srep36246
Ericson, D., Hamberg, K., Bratthall, G., Sinkiewicz-Enggren, G., and Ljunggren, L. (2013). Salivary IgA response to probiotic bacteria and mutans streptococci after the use of chewing gum containing Lactobacillus reuteri. Pathog. Dis. 68, 82–87. doi: 10.1111/2049-632X.12048
Esmaeili, S. A., Mahmoudi, M., Momtazi, A. A., Sahebkar, A., Doulabi, H., and Rastin, M. (2017). Tolerogenic probiotics: potential immunoregulators in Systemic Lupus Erythematosus. J. Cell. Physiol. 232, 1994–2007. doi: 10.1002/jcp.25748
Fåk, F., and Bäckhed, F. (2012). Lactobacillus reuteri prevents diet-induced obesity, but not atherosclerosis, in a strain dependent fashion in Apoe-/- mice. PLoS One 7:e46837. doi: 10.1371/journal.pone.0046837
Francavilla, R., Lionetti, E., Castellaneta, S., Ciruzzi, F., Indrio, F., Masciale, A., et al. (2012). Randomised clinical trial: Lactobacillus reuteri DSM 17938 vs. placebo in children with acute diarrhoea–a double-blind study. Aliment. Pharmacol. Ther. 36, 363–369. doi: 10.1111/j.1365-2036.2012.05180.x
Francavilla, R., Lionetti, E., Castellaneta, S. P., Magista, A. M., Maurogiovanni, G., Bucci, N., et al. (2008). Inhibition of Helicobacter pylori infection in humans by Lactobacillus reuteri ATCC 55730 and effect on eradication therapy: a pilot study. Helicobacter 13, 127–134. doi: 10.1111/j.1523-5378.2008.00593.x
Francavilla, R., Polimeno, L., Demichina, A., Maurogiovanni, G., Principi, B., Scaccianoce, G., et al. (2014). Lactobacillus reuteri strain combination in Helicobacter pylori infection: a randomized, double-blind, placebo-controlled study. J. Clin. Gastroenterol. 48, 407–413. doi: 10.1097/MCG.0000000000000007
Franceschi, F., Cazzato, A., Nista, E. C., Scarpellini, E., Roccarina, D., Gigante, G., et al. (2007). Role of probiotics in patients with Helicobacter pylori infection. Helicobacter 12(Suppl. 2), 59–63. doi: 10.1111/j.1523-5378.2007.00565.x
Frese, S. A., Benson, A. K., Tannock, G. W., Loach, D. M., Kim, J., Zhang, M., et al. (2011). The evolution of host specialization in the vertebrate gut symbiont Lactobacillus reuteri. PLoS Genet. 7:e1001314. doi: 10.1371/journal.pgen.1001314
Frese, S. A., Mackenzie, D. A., Peterson, D. A., Schmaltz, R., Fangman, T., Zhou, Y., et al. (2013). Molecular characterization of host-specific biofilm formation in a vertebrate gut symbiont. PLoS Genet. 9:e1004057. doi: 10.1371/journal.pgen.1004057
Galley, J. D., Mackos, A. R., Varaljay, V. A., and Bailey, M. T. (2017). Stressor exposure has prolonged effects on colonic microbial community structure in Citrobacter rodentium-challenged mice. Sci. Rep. 7:45012. doi: 10.1038/srep45012
Galley, J. D., Nelson, M. C., Yu, Z., Dowd, S. E., Walter, J., Kumar, P. S., et al. (2014). Exposure to a social stressor disrupts the community structure of the colonic mucosa-associated microbiota. BMC Microbiol. 14:189. doi: 10.1186/1471-2180-14-189
Ganzle, M. G., and Vogel, R. F. (2003). Studies on the mode of action of reutericyclin. Appl. Environ. Microbiol. 69, 1305–1307. doi: 10.1128/AEM.69.2.1305-1307.2003
Gao, C., Major, A., Rendon, D., Lugo, M., Jackson, V., Shi, Z., et al. (2015). Histamine H2 Receptor-Mediated Suppression of Intestinal Inflammation by Probiotic Lactobacillus reuteri. mBio 6:e01358-15. doi: 10.1128/mBio.01358-15
Garcia Rodenas, C. L., Lepage, M., Ngom-Bru, C., Fotiou, A., Papagaroufalis, K., and Berger, B. (2016). Effect of formula containing Lactobacillus reuteri DSM 17938 on fecal microbiota of infants born by cesarean-section. J. Pediatr. Gastroenterol. Nutr. 63, 681–687. doi: 10.1097/MPG.0000000000001198
Garofoli, F., Civardi, E., Indrio, F., Mazzucchelli, I., Angelini, M., Tinelli, C., et al. (2014). The early administration of Lactobacillus reuteri DSM 17938 controls regurgitation episodes in full-term breastfed infants. Int. J. Food Sci. Nutr. 65, 646–648. doi: 10.3109/09637486.2014.898251
Genis, S., Sanchez-Chardi, A., Bach, A., Fabregas, F., and Aris, A. (2017). A combination of lactic acid bacteria regulates Escherichia coli infection and inflammation of the bovine endometrium. J. Dairy Sci. 100, 479–492. doi: 10.3168/jds.2016-11671
Gille, C., Boer, B., Marschal, M., Urschitz, M. S., Heinecke, V., Hund, V., et al. (2016). Effect of probiotics on vaginal health in pregnancy. EFFPRO, a randomized controlled trial. Am. J. Obstet. Gynecol. 215, 608.e1–608.e7. doi: 10.1016/j.ajog.2016.06.021
Giraffa, G., Chanishvili, N., and Widyastuti, Y. (2010). Importance of lactobacilli in food and feed biotechnology. Res. Microbiol. 161, 480–487. doi: 10.1016/j.resmic.2010.03.001
Gopi, G. R., Ganesh, N., Pandiaraj, S., Sowmiya, B., Brajesh, R. G., and Ramalingam, S. (2015). A study on enhanced expression of 3-hydroxypropionic acid pathway genes and impact on its production in Lactobacillus reuteri. Food Technol. Biotechnol. 53, 331–336. doi: 10.17113/ftb.53.03.15.3976
Greifova, G., Majekova, H., Greif, G., Body, P., Greifova, M., and Dubnickova, M. (2017). Analysis of antimicrobial and immunomodulatory substances produced by heterofermentative Lactobacillus reuteri. Folia Microbiol. 62, 515–524. doi: 10.1007/s12223-017-0524-9
Grover, S., Rastogi, A., Singh, J., Rajbongshi, A., and Bihari, C. (2014). Spectrum of histomorphologic findings in liver in patients with SLE: a review. Hepat. Res. Treat. 2014:562979. doi: 10.1155/2014/562979
Gu, Q., Zhang, C., Song, D., Li, P., and Zhu, X. (2015). Enhancing vitamin B12 content in soy-yogurt by Lactobacillus reuteri. Int. J. Food Microbiol. 206, 56–59. doi: 10.1016/j.ijfoodmicro.2015.04.033
Gunning, A. P., Kavanaugh, D., Thursby, E., Etzold, S., MacKenzie, D. A., and Juge, N. (2016). Use of atomic force microscopy to study the multi-modular interaction of bacterial adhesins to mucins. Int. J. Mol. Sci. 17:E1854. doi: 10.3390/ijms17111854
Gutierrez-Castrellon, P., Lopez-Velazquez, G., Diaz-Garcia, L., Jimenez-Gutierrez, C., Mancilla-Ramirez, J., Estevez-Jimenez, J., et al. (2014). Diarrhea in preschool children and Lactobacillus reuteri: a randomized controlled trial. Pediatrics 133, e904–e909. doi: 10.1542/peds.2013-0652
Harakeh, S. M., Khan, I., Kumosani, T., Barbour, E., Almasaudi, S. B., Bahijri, S. M., et al. (2016). Gut microbiota: a contributing factor to obesity. Front. Cell. Infect. Microbiol. 6:95. doi: 10.3389/fcimb.2016.00095
He, B., Hoang, T. K., Wang, T., Ferris, M., Taylor, C. M., Tian, X., et al. (2017). Resetting microbiota by Lactobacillus reuteri inhibits T reg deficiency-induced autoimmunity via adenosine A2A receptors. J. Exp. Med. 214, 107–123. doi: 10.1084/jem.20160961
Hemarajata, P., Gao, C., Pflughoeft, K. J., Thomas, C. M., Saulnier, D. M., Spinler, J. K., et al. (2013). Lactobacillus reuteri-specific immunoregulatory gene rsiR modulates histamine production and immunomodulation by Lactobacillus reuteri. J. Bacteriol. 195, 5567–5576. doi: 10.1128/JB.00261-13
Hill, C., Guarner, F., Reid, G., Gibson, G. R., Merenstein, D. J., Pot, B., et al. (2014). Expert consensus document. The International Scientific Association for Probiotics and Prebiotics consensus statement on the scope and appropriate use of the term probiotic. Nat. Rev. Gastroenterol. Hepatol. 11, 506–514. doi: 10.1038/nrgastro.2014.66
Holz, C., Busjahn, A., Mehling, H., Arya, S., Boettner, M., Habibi, H., et al. (2015). Significant reduction in Helicobacter pylori load in humans with non-viable Lactobacillus reuteri DSM17648: a pilot study. Probiotics Antimicrob. Proteins 7, 91–100. doi: 10.1007/s12602-014-9181-3
Hou, C., Wang, Q., Zeng, X., Yang, F., Zhang, J., Liu, H., et al. (2014). Complete genome sequence of Lactobacillus reuteri I5007, a probiotic strain isolated from healthy piglet. J. Biotechnol. 179, 63–64. doi: 10.1016/j.jbiotec.2014.03.019
Hou, C., Zeng, X., Yang, F., Liu, H., and Qiao, S. (2015). Study and use of the probiotic Lactobacillus reuteri in pigs: a review. J. Anim. Sci. Biotechnol. 6:14. doi: 10.1186/s40104-015-0014-3
Hoy-Schulz, Y. E., Jannat, K., Roberts, T., Zaidi, S. H., Unicomb, L., Luby, S., et al. (2016). Safety and acceptability of Lactobacillus reuteri DSM 17938 and Bifidobacterium longum subspecies infantis 35624 in Bangladeshi infants: a phase I randomized clinical trial. BMC Complement. Altern. Med. 16:44. doi: 10.1186/s12906-016-1016-1
Hsieh, F. C., Lan, C. C., Huang, T. Y., Chen, K. W., Chai, C. Y., Chen, W. T., et al. (2016). Heat-killed and live Lactobacillus reuteri GMNL-263 exhibit similar effects on improving metabolic functions in high-fat diet-induced obese rats. Food Funct. 7, 2374–2388. doi: 10.1039/c5fo01396h
Hsu, T. C., Huang, C. Y., Chiang, S. Y., Lai, W. X., Tsai, C. H., and Tzang, B. S. (2008). Transglutaminase inhibitor cystamine alleviates the abnormality in liver from NZB/W F1 mice. Eur. J. Pharmacol. 579, 382–389. doi: 10.1016/j.ejphar.2007.10.059
Hsu, T. C., Huang, C. Y., Liu, C. H., Hsu, K. C., Chen, Y. H., and Tzang, B. S. (2017). Lactobacillus paracasei GMNL-32, Lactobacillus reuteri GMNL-89 and L. reuteri GMNL-263 ameliorate hepatic injuries in lupus-prone mice. Br. J. Nutr. 117, 1066–1074. doi: 10.1017/S0007114517001039
Imase, K., Tanaka, A., Tokunaga, K., Sugano, H., Ishida, H., and Takahashi, S. (2007). Lactobacillus reuteri tablets suppress Helicobacter pylori infection–a double-blind randomised placebo-controlled cross-over clinical study. Kansenshogaku Zasshi 81, 387–393. doi: 10.11150/kansenshogakuzasshi1970.81.387
Indrio, F., Di Mauro, A., Riezzo, G., Civardi, E., Intini, C., Corvaglia, L., et al. (2014). Prophylactic use of a probiotic in the prevention of colic, regurgitation, and functional constipation: a randomized clinical trial. JAMA Pediatr. 168, 228–233. doi: 10.1001/jamapediatrics.2013.4367
Indrio, F., Riezzo, G., Raimondi, F., Bisceglia, M., Cavallo, L., and Francavilla, R. (2008). The effects of probiotics on feeding tolerance, bowel habits, and gastrointestinal motility in preterm newborns. J. Pediatr. 152, 801–806. doi: 10.1016/j.jpeds.2007.11.005
Indrio, F., Riezzo, G., Raimondi, F., Bisceglia, M., Cavallo, L., and Francavilla, R. (2009). Effects of probiotic and prebiotic on gastrointestinal motility in newborns. J. Physiol. Pharmacol. 60(Suppl. 6), 27–31.
Iniesta, M., Herrera, D., Montero, E., Zurbriggen, M., Matos, A. R., Marin, M. J., et al. (2012). Probiotic effects of orally administered Lactobacillus reuteri-containing tablets on the subgingival and salivary microbiota in patients with gingivitis. A randomized clinical trial. J. Clin. Periodontol. 39, 736–744. doi: 10.1111/j.1600-051X.2012.01914.x
Islam, S. U. (2016). Clinical uses of probiotics. Medicine 95:e2658. doi: 10.1097/MD.0000000000002658
Jacobsen, C. N., Rosenfeldt Nielsen, V., Hayford, A. E., Moller, P. L., Michaelsen, K. F., Paerregaard, A., et al. (1999). Screening of probiotic activities of forty-seven strains of Lactobacillus spp. by in vitro techniques and evaluation of the colonization ability of five selected strains in humans. Appl. Environ. Microbiol. 65, 4949–4956.
Jensen, H., Roos, S., Jonsson, H., Rud, I., Grimmer, S., van Pijkeren, J. P., et al. (2014). Role of Lactobacillus reuteri cell and mucus-binding protein A (CmbA) in adhesion to intestinal epithelial cells and mucus in vitro. Microbiology 160(Pt 4), 671–681. doi: 10.1099/mic.0.073551-0
Jones, M. L., Martoni, C. J., Di Pietro, E., Simon, R. R., and Prakash, S. (2012a). Evaluation of clinical safety and tolerance of a Lactobacillus reuteri NCIMB 30242 supplement capsule: a randomized control trial. Regul. Toxicol. Pharmacol. 63, 313–320. doi: 10.1016/j.yrtph.2012.04.003
Jones, M. L., Martoni, C. J., and Prakash, S. (2012b). Cholesterol lowering and inhibition of sterol absorption by Lactobacillus reuteri NCIMB 30242: a randomized controlled trial. Eur. J. Clin. Nutr. 66, 1234–1241. doi: 10.1038/ejcn.2012.126
Jones, M. L., Martoni, C. J., Tamber, S., Parent, M., and Prakash, S. (2012c). Evaluation of safety and tolerance of microencapsulated Lactobacillus reuteri NCIMB 30242 in a yogurt formulation: a randomized, placebo-controlled, double-blind study. Food Chem. Toxicol. 50, 2216–2223. doi: 10.1016/j.fct.2012.03.010
Jones, S. E., and Versalovic, J. (2009). Probiotic Lactobacillus reuteri biofilms produce antimicrobial and anti-inflammatory factors. BMC Microbiol. 9:35. doi: 10.1186/1471-2180-9-35
Jorgensen, M. R., Keller, M. K., Kragelund, C., Hamberg, K., Ericson, D., Nielsen, C. H., et al. (2016). Lactobacillus reuteri supplements do not affect salivary IgA or cytokine levels in healthy subjects: a randomized, double-blind, placebo-controlled, cross-over trial. Acta Odontol. Scand. 74, 399–404. doi: 10.3109/00016357.2016.1169439
Jorgensen, M. R., Kragelund, C., Jensen, P. O., Keller, M. K., and Twetman, S. (2017). Probiotic Lactobacillus reuteri has antifungal effects on oral Candida species in vitro. J. Oral Microbiol. 9:1274582. doi: 10.1080/20002297.2016.1274582
Kamada, N., Seo, S. U., Chen, G. Y., and Nunez, G. (2013). Role of the gut microbiota in immunity and inflammatory disease. Nat. Rev. Immunol. 13, 321–335. doi: 10.1038/nri3430
Kamiya, T., Wang, L., Forsythe, P., Goettsche, G., Mao, Y., Wang, Y., et al. (2006). Inhibitory effects of Lactobacillus reuteri on visceral pain induced by colorectal distension in Sprague-Dawley rats. Gut 55, 191–196. doi: 10.1136/gut.2005.070987
Kandler, O., Stetter, K. O., and Kohl, R. (1980). Lactobacillus reuteri sp. nov., a new species of heterofermentative lactobacilli. Zentralbl. Bakteriol. Hyg. I Abt Orig. 1, 264–269. doi: 10.1016/S0172-5564(80)80007-8
Karaffova, V., Csank, T., Mudronova, D., Kiraly, J., Revajova, V., Gancarcikova, S., et al. (2017). Influence of Lactobacillus reuteri L26 Biocenol on immune response against porcine circovirus type 2 infection in germ-free mice. Benef. Microbes 8, 367–378. doi: 10.3920/BM2016.0114
Karimi, K., Inman, M. D., Bienenstock, J., and Forsythe, P. (2009). Lactobacillus reuteri-induced regulatory T cells protect against an allergic airway response in mice. Am. J. Respir. Crit. Care Med. 179, 186–193. doi: 10.1164/rccm.200806-951OC
Kechagia, M., Basoulis, D., Konstantopoulou, S., Dimitriadi, D., Gyftopoulou, K., Skarmoutsou, N., et al. (2013). Health benefits of probiotics: a review. ISRN Nutr. 2013:481651. doi: 10.5402/2013/481651
Keller, M. K., Nohr Larsen, I., Karlsson, I., and Twetman, S. (2014). Effect of tablets containing probiotic bacteria (Lactobacillus reuteri) on early caries lesions in adolescents: a pilot study. Benef. Microbes 5, 403–407. doi: 10.3920/BM2013.0089
Kleerebezem, M., Hols, P., Bernard, E., Rolain, T., Zhou, M., Siezen, R. J., et al. (2010). The extracellular biology of the lactobacilli. FEMS Microbiol. Rev. 34, 199–230. doi: 10.1111/j.1574-6976.2010.00208.x
Koleva, P. T., Bridgman, S. L., and Kozyrskyj, A. L. (2015). The infant gut microbiome: evidence for obesity risk and dietary intervention. Nutrients 7, 2237–2260. doi: 10.3390/nu7042237
Krumbeck, J. A., Marsteller, N. L., Frese, S. A., Peterson, D. A., Ramer-Tait, A. E., Hutkins, R. W., et al. (2016). Characterization of the ecological role of genes mediating acid resistance in Lactobacillus reuteri during colonization of the gastrointestinal tract. Environ. Microbiol. 18, 2172–2184. doi: 10.1111/1462-2920.13108
Ksonzekova, P., Bystricky, P., Vlckova, S., Patoprsty, V., Pulzova, L., Mudronova, D., et al. (2016). Exopolysaccharides of Lactobacillus reuteri: their influence on adherence of E. coli to epithelial cells and inflammatory response. Carbohydr. Polym. 141, 10–19. doi: 10.1016/j.carbpol.2015.12.037
Kunze, W. A., Mao, Y. K., Wang, B., Huizinga, J. D., Ma, X., Forsythe, P., et al. (2009). Lactobacillus reuteri enhances excitability of colonic AH neurons by inhibiting calcium-dependent potassium channel opening. J. Cell Mol. Med. 13, 2261–2270. doi: 10.1111/j.1582-4934.2009.00686.x
Lai, N. Y., Mills, K., and Chiu, I. M. (2017). Sensory neuron regulation of gastrointestinal inflammation and bacterial host defence. J. Intern. Med. 282, 5–23. doi: 10.1111/joim.12591
Lebeer, S., Vanderleyden, J., and De Keersmaecker, S. C. (2008). Genes and molecules of lactobacilli supporting probiotic action. Microbiol. Mol. Biol. Rev. 72, 728–764. doi: 10.1128/MMBR.00017-08
Lee, J., Yang, W., Hostetler, A., Schultz, N., Suckow, M. A., Stewart, K. L., et al. (2016). Characterization of the anti-inflammatory Lactobacillus reuteri BM36301 and its probiotic benefits on aged mice. BMC Microbiol. 16:69. doi: 10.1186/s12866-016-0686-7
Lesbros-Pantoflickova, D., Corthesy-Theulaz, I., and Blum, A. L. (2007). Helicobacter pylori and probiotics. J. Nutr. 137(3 Suppl. 2), 812S–818S. doi: 10.1093/jn/137.3.812S
Li, X. J., Yue, L. Y., Guan, X. F., and Qiao, S. Y. (2008). The adhesion of putative probiotic lactobacilli to cultured epithelial cells and porcine intestinal mucus. J. Appl. Microbiol. 104, 1082–1091. doi: 10.1111/j.1365-2672.2007.03636.x
Liao, P. H., Kuo, W. W., Hsieh, D. J., Yeh, Y. L., Day, C. H., Chen, Y. H., et al. (2016). Heat-killed Lactobacillus reuteri GMNL-263 prevents epididymal fat accumulation and cardiac injury in high-calorie diet-fed rats. Int. J. Med. Sci. 13, 569–577. doi: 10.7150/ijms.15597
Linares, D. M., Gomez, C., Renes, E., Fresno, J. M., Tornadijo, M. E., Ross, R. P., et al. (2017). Lactic acid bacteria and bifidobacteria with potential to design natural biofunctional health-promoting dairy foods. Front. Microbiol. 8:846. doi: 10.3389/fmicb.2017.00846
Lionetti, E., Miniello, V. L., Castellaneta, S. P., Magista, A. M., de Canio, A., Maurogiovanni, G., et al. (2006). Lactobacillus reuteri therapy to reduce side-effects during anti-Helicobacter pylori treatment in children: a randomized placebo controlled trial. Aliment. Pharmacol. Ther. 24, 1461–1468. doi: 10.1111/j.1365-2036.2006.03145.x
Liu, F., Wang, X., Shi, H., Wang, Y., Xue, C., and Tang, Q. J. (2017). Polymannuronic acid ameliorated obesity and inflammation associated with a high-fat and high-sucrose diet by modulating the gut microbiome in a murine model. Br. J. Nutr. 117, 1332–1342. doi: 10.1017/S0007114517000964
Liu, H., Hou, C., Wang, G., Jia, H., Yu, H., Zeng, X., et al. (2017). Lactobacillus reuteri I5007 modulates intestinal host defense peptide expression in the model of IPEC-J2 cells and neonatal piglets. Nutrients 9:E559. doi: 10.3390/nu9060559
Liu, Y., Fatheree, N. Y., Dingle, B. M., Tran, D. Q., and Rhoads, J. M. (2013). Lactobacillus reuteri DSM 17938 changes the frequency of Foxp3+ regulatory T cells in the intestine and mesenteric lymph node in experimental necrotizing enterocolitis. PLoS One 8:e56547. doi: 10.1371/journal.pone.0056547
Liu, Y., Tran, D. Q., Fatheree, N. Y., and Marc Rhoads, J. (2014). Lactobacillus reuteri DSM 17938 differentially modulates effector memory T cells and Foxp3+ regulatory T cells in a mouse model of necrotizing enterocolitis. Am. J. Physiol. Gastrointest. Liver Physiol. 307, G177–G186. doi: 10.1152/ajpgi.00038.2014
Livingston, M., Loach, D., Wilson, M., Tannock, G. W., and Baird, M. (2010). Gut commensal Lactobacillus reuteri 100-23 stimulates an immunoregulatory response. Immunol. Cell Biol. 88, 99–102. doi: 10.1038/icb.2009.71
Lorea Baroja, M., Kirjavainen, P. V., Hekmat, S., and Reid, G. (2007). Anti-inflammatory effects of probiotic yogurt in inflammatory bowel disease patients. Clin. Exp. Immunol. 149, 470–479. doi: 10.1111/j.1365-2249.2007.03434.x
Ma, X., Mao, Y. K., Wang, B., Huizinga, J. D., Bienenstock, J., and Kunze, W. (2009). Lactobacillus reuteri ingestion prevents hyperexcitability of colonic DRG neurons induced by noxious stimuli. Am. J. Physiol. Gastrointest. Liver Physiol. 296, G868–G875. doi: 10.1152/ajpgi.90511.2008
Mackenzie, D. A., Jeffers, F., Parker, M. L., Vibert-Vallet, A., Bongaerts, R. J., Roos, S., et al. (2010). Strain-specific diversity of mucus-binding proteins in the adhesion and aggregation properties of Lactobacillus reuteri. Microbiology 156(Pt 11), 3368–3378. doi: 10.1099/mic.0.043265-0
Macklaim, J. M., Clemente, J. C., Knight, R., Gloor, G. B., and Reid, G. (2015). Changes in vaginal microbiota following antimicrobial and probiotic therapy. Microb. Ecol. Health Dis. 26:27799. doi: 10.3402/mehd.v26.27799
Mackos, A. R., Eubank, T. D., Parry, N. M., and Bailey, M. T. (2013). Probiotic Lactobacillus reuteri attenuates the stressor-enhanced severity of Citrobacter rodentium infection. Infect. Immun. 81, 3253–3263. doi: 10.1128/IAI.00278-13
Mackos, A. R., Galley, J. D., Eubank, T. D., Easterling, R. S., Parry, N. M., Fox, J. G., et al. (2016). Social stress-enhanced severity of Citrobacter rodentium-induced colitis is CCL2-dependent and attenuated by probiotic Lactobacillus reuteri. Mucosal Immunol. 9, 515–526. doi: 10.1038/mi.2015.81
Mangalat, N., Liu, Y., Fatheree, N. Y., Ferris, M. J., Van Arsdall, M. R., Chen, Z., et al. (2012). Safety and tolerability of Lactobacillus reuteri DSM 17938 and effects on biomarkers in healthy adults: results from a randomized masked trial. PLoS One 7:e43910. doi: 10.1371/journal.pone.0043910
Markle, J. G., Frank, D. N., Mortin-Toth, S., Robertson, C. E., Feazel, L. M., Rolle-Kampczyk, U., et al. (2013). Sex differences in the gut microbiome drive hormone-dependent regulation of autoimmunity. Science 339, 1084–1088. doi: 10.1126/science.1233521
Martoni, C. J., Labbe, A., Ganopolsky, J. G., Prakash, S., and Jones, M. L. (2015). Changes in bile acids, FGF-19 and sterol absorption in response to bile salt hydrolase active L. reuteri NCIMB 30242. Gut Microbes 6, 57–65. doi: 10.1080/19490976.2015.1005474
McFall-Ngai, M. (2007). Adaptive immunity: care for the community. Nature 445:153. doi: 10.1038/445153a
McMillan, A., Dell, M., Zellar, M. P., Cribby, S., Martz, S., Hong, E., et al. (2011). Disruption of urogenital biofilms by lactobacilli. Colloids Surf. B Biointerfaces 86, 58–64. doi: 10.1016/j.colsurfb.2011.03.016
McVey Neufeld, K. A., Mao, Y. K., Bienenstock, J., Foster, J. A., and Kunze, W. A. (2013). The microbiome is essential for normal gut intrinsic primary afferent neuron excitability in the mouse. Neurogastroenterol. Motil. 25, e183–e188. doi: 10.1111/nmo.12049
Mehling, H., and Busjahn, A. (2013). Non-viable Lactobacillus reuteri DSMZ 17648 (Pylopass) as a new approach to Helicobacter pylori control in humans. Nutrients 5, 3062–3073. doi: 10.3390/nu5083062
Mi, G. L., Zhao, L., Qiao, D. D., Kang, W. Q., Tang, M. Q., and Xu, J. K. (2015). Effectiveness of Lactobacillus reuteri in infantile colic and colicky induced maternal depression: a prospective single blind randomized trial. Antonie Van Leeuwenhoek 107, 1547–1553. doi: 10.1007/s10482-015-0448-9
Million, M., Angelakis, E., Maraninchi, M., Henry, M., Giorgi, R., Valero, R., et al. (2013a). Correlation between body mass index and gut concentrations of Lactobacillus reuteri, Bifidobacterium animalis, Methanobrevibacter smithii and Escherichia coli. Int. J. Obes. 37, 1460–1466. doi: 10.1038/ijo.2013.20
Million, M., Maraninchi, M., Henry, M., Armougom, F., Richet, H., Carrieri, P., et al. (2012). Obesity-associated gut microbiota is enriched in Lactobacillus reuteri and depleted in Bifidobacterium animalis and Methanobrevibacter smithii. Int. J. Obes. 36, 817–825. doi: 10.1038/ijo.2011.153
Million, M., Thuny, F., Angelakis, E., Casalta, J. P., Giorgi, R., Habib, G., et al. (2013b). Lactobacillus reuteri and Escherichia coli in the human gut microbiota may predict weight gain associated with vancomycin treatment. Nutr. Diabetes 3:e87. doi: 10.1038/nutd.2013.28
Miniello, V. L., Brunetti, L., Tesse, R., Natile, M., Armenio, L., and Francavilla, R. (2010). Lactobacillus reuteri modulates cytokines production in exhaled breath condensate of children with atopic dermatitis. J. Pediatr. Gastroenterol. Nutr. 50, 573–576. doi: 10.1097/MPG.0b013e3181bb343f
Mishra, S. K., Malik, R. K., Manju, G., Pandey, N., Singroha, G., Behare, P., et al. (2012). Characterization of a reuterin-producing Lactobacillus reuteri BPL-36 strain isolated from human infant fecal sample. Probiotics Antimicrob. Proteins 4, 154–161. doi: 10.1007/s12602-012-9103-1
Mobini, R., Tremaroli, V., Stahlman, M., Karlsson, F., Levin, M., Ljungberg, M., et al. (2017). Metabolic effects of Lactobacillus reuteri DSM 17938 in people with type 2 diabetes: a randomized controlled trial. Diabetes Obes. Metab. 19, 579–589. doi: 10.1111/dom.12861
Molina, V. C., Medici, M., Taranto, M. P., and Font de Valdez, G. (2009). Lactobacillus reuteri CRL 1098 prevents side effects produced by a nutritional vitamin B deficiency. J. Appl. Microbiol. 106, 467–473. doi: 10.1111/j.1365-2672.2008.04014.x
Montalban-Arques, A., De Schryver, P., Bossier, P., Gorkiewicz, G., Mulero, V., Gatlin, D. M., et al. (2015). Selective manipulation of the gut microbiota improves immune status in vertebrates. Front. Immunol. 6:512. doi: 10.3389/fimmu.2015.00512
Morita, H., Toh, H., Fukuda, S., Horikawa, H., Oshima, K., Suzuki, T., et al. (2008). Comparative genome analysis of Lactobacillus reuteri and Lactobacillus fermentum reveal a genomic island for reuterin and cobalamin production. DNA Res. 15, 151–161. doi: 10.1093/dnares/dsn009
Mu, Q., Kirby, J., Reilly, C. M., and Luo, X. M. (2017a). Leaky gut as a danger signal for autoimmune diseases. Front. Immunol. 8:598. doi: 10.3389/fimmu.2017.00598
Mu, Q., Tavella, V. J., Kirby, J. L., Cecere, T. E., Chung, M., Lee, J., et al. (2017b). Antibiotics ameliorate lupus-like symptoms in mice. Sci. Rep. 7:13675. doi: 10.1038/s41598-017-14223-0
Mu, Q., Zhang, H., Liao, X., Lin, K., Liu, H., Edwards, M. R., et al. (2017c). Control of lupus nephritis by changes of gut microbiota. Microbiome 5:73. doi: 10.1186/s40168-017-0300-8
Mu, Q., Zhang, H., and Luo, X. M. (2015). SLE: another autoimmune disorder influenced by microbes and diet? Front. Immunol. 6:608. doi: 10.3389/fimmu.2015.00608
Mukai, T., Asasaka, T., Sato, E., Mori, K., Matsumoto, M., and Ohori, H. (2002). Inhibition of binding of Helicobacter pylori to the glycolipid receptors by probiotic Lactobacillus reuteri. FEMS Immunol. Med. Microbiol. 32, 105–110. doi: 10.1111/j.1574-695X.2002.tb00541.x
Nagpal, R., Tsuji, H., Takahashi, T., Kawashima, K., Nagata, S., Nomoto, K., et al. (2016). Sensitive quantitative analysis of the meconium bacterial microbiota in healthy term infants born vaginally or by cesarean section. Front. Microbiol. 7:1997. doi: 10.3389/fmicb.2016.01997
Navarro, J. B., Mashburn-Warren, L., Bakaletz, L. O., Bailey, M. T., and Goodman, S. D. (2017). Enhanced probiotic potential of Lactobacillus reuteri when delivered as a biofilm on dextranomer microspheres that contain beneficial cargo. Front. Microbiol. 8:489. doi: 10.3389/fmicb.2017.00489
Nguyen, N. T., Hanieh, H., Nakahama, T., and Kishimoto, T. (2013). The roles of aryl hydrocarbon receptor in immune responses. Int. Immunol. 25, 335–343. doi: 10.1093/intimm/dxt011
Oh, P. L., Benson, A. K., Peterson, D. A., Patil, P. B., Moriyama, E. N., Roos, S., et al. (2010). Diversification of the gut symbiont Lactobacillus reuteri as a result of host-driven evolution. ISME J. 4, 377–387. doi: 10.1038/ismej.2009.123
Ojetti, V., Bruno, G., Ainora, M. E., Gigante, G., Rizzo, G., Roccarina, D., et al. (2012). Impact of Lactobacillus reuteri supplementation on anti-Helicobacter pylori levofloxacin-based second-line therapy. Gastroenterol. Res. Pract. 2012:740381. doi: 10.1155/2012/740381
Okeke, F., Roland, B. C., and Mullin, G. E. (2014). The role of the gut microbiome in the pathogenesis and treatment of obesity. Glob. Adv. Health Med. 3, 44–57. doi: 10.7453/gahmj.2014.018
Olson, J. K., Rager, T. M., Navarro, J. B., Mashburn-Warren, L., Goodman, S. D., and Besner, G. E. (2016). Harvesting the benefits of biofilms: a novel probiotic delivery system for the prevention of necrotizing enterocolitis. J. Pediatr. Surg. 51, 936–941. doi: 10.1016/j.jpedsurg.2016.02.062
Oncel, M. Y., Sari, F. N., Arayici, S., Guzoglu, N., Erdeve, O., Uras, N., et al. (2014). Lactobacillus reuteri for the prevention of necrotising enterocolitis in very low birthweight infants: a randomised controlled trial. Arch. Dis. Child. Fetal Neonatal Ed. 99, F110–F115. doi: 10.1136/archdischild-2013-304745
Pallin, A., Agback, P., Jonsson, H., and Roos, S. (2016). Evaluation of growth, metabolism and production of potentially bioactive components during fermentation of barley with Lactobacillus reuteri. Food Microbiol. 57, 159–171. doi: 10.1016/j.fm.2016.02.011
Park, S. K., Park, D. I., Choi, J. S., Kang, M. S., Park, J. H., Kim, H. J., et al. (2007). The effect of probiotics on Helicobacter pylori eradication. Hepatogastroenterology 54, 2032–2036.
Petricevic, L., Unger, F. M., Viernstein, H., and Kiss, H. (2008). Randomized, double-blind, placebo-controlled study of oral lactobacilli to improve the vaginal flora of postmenopausal women. Eur. J. Obstet. Gynecol. Reprod. Biol. 141, 54–57. doi: 10.1016/j.ejogrb.2008.06.003
Piyathilake, C. J., Ollberding, N. J., Kumar, R., Macaluso, M., Alvarez, R. D., and Morrow, C. D. (2016). Cervical microbiota associated with higher grade cervical intraepithelial neoplasia in women infected with high-risk human papillomaviruses. Cancer Prev. Res. 9, 357–366. doi: 10.1158/1940-6207.CAPR-15-0350
Poutahidis, T., Kearney, S. M., Levkovich, T., Qi, P., Varian, B. J., Lakritz, J. R., et al. (2013a). Microbial symbionts accelerate wound healing via the neuropeptide hormone oxytocin. PLoS One 8:e78898. doi: 10.1371/journal.pone.0078898
Poutahidis, T., Kleinewietfeld, M., Smillie, C., Levkovich, T., Perrotta, A., Bhela, S., et al. (2013b). Microbial reprogramming inhibits Western diet-associated obesity. PLoS One 8:e68596. doi: 10.1371/journal.pone.0068596
Preidis, G. A., Saulnier, D. M., Blutt, S. E., Mistretta, T. A., Riehle, K. P., Major, A. M., et al. (2012). Host response to probiotics determined by nutritional status of rotavirus-infected neonatal mice. J. Pediatr. Gastroenterol. Nutr. 55, 299–307. doi: 10.1097/MPG.0b013e31824d2548
Raynaud, C., Sarcabal, P., Meynial-Salles, I., Croux, C., and Soucaille, P. (2003). Molecular characterization of the 1,3-propanediol (1,3-PD) operon of Clostridium butyricum. Proc. Natl. Acad. Sci. U.S.A. 100, 5010–5015. doi: 10.1073/pnas.0734105100
Reid, G., and Burton, J. (2002). Use of Lactobacillus to prevent infection by pathogenic bacteria. Microbes Infect. 4, 319–324. doi: 10.1016/S1286-4579(02)01544-7
Rojas, M. A., Lozano, J. M., Rojas, M. X., Rodriguez, V. A., Rondon, M. A., Bastidas, J. A., et al. (2012). Prophylactic probiotics to prevent death and nosocomial infection in preterm infants. Pediatrics 130, e1113–e1120. doi: 10.1542/peds.2011-3584
Romani Vestman, N., Chen, T., Lif Holgerson, P., Ohman, C., and Johansson, I. (2015). Oral microbiota shift after 12-week supplementation with Lactobacillus reuteri DSM 17938 and PTA 5289; a randomized control trial. PLoS One 10:e0125812. doi: 10.1371/journal.pone.0125812
Romano, C., Ferrau, V., Cavataio, F., Iacono, G., Spina, M., Lionetti, E., et al. (2014). Lactobacillus reuteri in children with functional abdominal pain (FAP). J. Paediatr. Child Health 50, E68–E71. doi: 10.1111/j.1440-1754.2010.01797.x
Roos, S., Dicksved, J., Tarasco, V., Locatelli, E., Ricceri, F., Grandin, U., et al. (2013). 454 pyrosequencing analysis on faecal samples from a randomized DBPC trial of colicky infants treated with Lactobacillus reuteri DSM 17938. PLoS One 8:e56710. doi: 10.1371/journal.pone.0056710
Roos, S., and Jonsson, H. (2002). A high-molecular-mass cell-surface protein from Lactobacillus reuteri 1063 adheres to mucus components. Microbiology 148(Pt 2), 433–442. doi: 10.1099/00221287-148-2-433
Rosenfeldt, V., Benfeldt, E., Nielsen, S. D., Michaelsen, K. F., Jeppesen, D. L., Valerius, N. H., et al. (2003). Effect of probiotic Lactobacillus strains in children with atopic dermatitis. J. Allergy Clin. Immunol. 111, 389–395. doi: 10.1067/mai.2003.389
Rosenfeldt, V., Benfeldt, E., Valerius, N. H., Paerregaard, A., and Michaelsen, K. F. (2004). Effect of probiotics on gastrointestinal symptoms and small intestinal permeability in children with atopic dermatitis. J. Pediatr. 145, 612–616. doi: 10.1016/j.jpeds.2004.06.068
Rosenfeldt, V., Michaelsen, K. F., Jakobsen, M., Larsen, C. N., Moller, P. L., Pedersen, P., et al. (2002a). Effect of probiotic Lactobacillus strains in young children hospitalized with acute diarrhea. Pediatr. Infect. Dis. J. 21, 411–416. doi: 10.1097/00006454-200205000-00012
Rosenfeldt, V., Michaelsen, K. F., Jakobsen, M., Larsen, C. N., Moller, P. L., Tvede, M., et al. (2002b). Effect of probiotic Lactobacillus strains on acute diarrhea in a cohort of nonhospitalized children attending day-care centers. Pediatr. Infect. Dis. J. 21, 417–419.
Rossi, F., Gardini, F., Rizzotti, L., La Gioia, F., Tabanelli, G., and Torriani, S. (2011). Quantitative analysis of histidine decarboxylase gene (hdcA) transcription and histamine production by Streptococcus thermophilus PRI60 under conditions relevant to cheese making. Appl. Environ. Microbiol. 77, 2817–2822. doi: 10.1128/AEM.02531-10
Salas-Jara, M. J., Ilabaca, A., Vega, M., and Garcia, A. (2016). Biofilm forming Lactobacillus: new challenges for the development of probiotics. Microorganisms 4:E35. doi: 10.3390/microorganisms4030035
Santos, F., Spinler, J. K., Saulnier, D. M., Molenaar, D., Teusink, B., de Vos, W. M., et al. (2011). Functional identification in Lactobacillus reuteri of a PocR-like transcription factor regulating glycerol utilization and vitamin B12 synthesis. Microb. Cell Fact. 10:55. doi: 10.1186/1475-2859-10-55
Santos, F., Vera, J. L., van der Heijden, R., Valdez, G., de Vos, W. M., Sesma, F., et al. (2008a). The complete coenzyme B12 biosynthesis gene cluster of Lactobacillus reuteri CRL1098. Microbiology 154(Pt 1), 81–93. doi: 10.1099/mic.0.2007/011569-0
Santos, F., Wegkamp, A., de Vos, W. M., Smid, E. J., and Hugenholtz, J. (2008b). High-Level folate production in fermented foods by the B12 producer Lactobacillus reuteri JCM1112. Appl. Environ. Microbiol. 74, 3291–3294. doi: 10.1128/AEM.02719-07
Savino, F., Ceratto, S., Poggi, E., Cartosio, M. E., Cordero di Montezemolo, L., and Giannattasio, A. (2015a). Preventive effects of oral probiotic on infantile colic: a prospective, randomised, blinded, controlled trial using Lactobacillus reuteri DSM 17938. Benef. Microbes 6, 245–251. doi: 10.3920/BM2014.0090
Savino, F., Cordisco, L., Tarasco, V., Palumeri, E., Calabrese, R., Oggero, R., et al. (2010). Lactobacillus reuteri DSM 17938 in infantile colic: a randomized, double-blind, placebo-controlled trial. Pediatrics 126, e526–e533. doi: 10.1542/peds.2010-0433
Savino, F., Fornasero, S., Ceratto, S., De Marco, A., Mandras, N., Roana, J., et al. (2015b). Probiotics and gut health in infants: a preliminary case-control observational study about early treatment with Lactobacillus reuteri DSM 17938. Clin. Chim. Acta 451(Pt A), 82–87. doi: 10.1016/j.cca.2015.02.027
Savino, F., Pelle, E., Palumeri, E., Oggero, R., and Miniero, R. (2007). Lactobacillus reuteri (American Type Culture Collection Strain 55730) versus simethicone in the treatment of infantile colic: a prospective randomized study. Pediatrics 119, e124–e130. doi: 10.1542/peds.2006-1222
Scaccianoce, G., Zullo, A., Hassan, C., Gentili, F., Cristofari, F., Cardinale, V., et al. (2008). Triple therapies plus different probiotics for Helicobacter pylori eradication. Eur. Rev. Med. Pharmacol. Sci. 12, 251–256.
Scott, K. P., Antoine, J. M., Midtvedt, T., and van Hemert, S. (2015). Manipulating the gut microbiota to maintain health and treat disease. Microb. Ecol. Health Dis. 26:25877. doi: 10.3402/mehd.v26.25877
Seo, B. J., Mun, M. R., J, R. K., Kim, C. J., Lee, I., Chang, Y. H., et al. (2010). Bile tolerant Lactobacillus reuteri isolated from pig feces inhibits enteric bacterial pathogens and porcine rotavirus. Vet. Res. Commun. 34, 323–333. doi: 10.1007/s11259-010-9357-6
Shi, L. H., Balakrishnan, K., Thiagarajah, K., Mohd Ismail, N. I., and Yin, O. S. (2016). Beneficial properties of probiotics. Trop. Life Sci. Res. 27, 73–90. doi: 10.21315/tlsr2016.27.2.6
Shornikova, A. V., Casas, I. A., Isolauri, E., Mykkanen, H., and Vesikari, T. (1997a). Lactobacillus reuteri as a therapeutic agent in acute diarrhea in young children. J. Pediatr. Gastroenterol. Nutr. 24, 399–404.
Shornikova, A. V., Casas, I. A., Mykkanen, H., Salo, E., and Vesikari, T. (1997b). Bacteriotherapy with Lactobacillus reuteri in rotavirus gastroenteritis. Pediatr. Infect. Dis. J. 16, 1103–1107.
Sims, I. M., Frese, S. A., Walter, J., Loach, D., Wilson, M., Appleyard, K., et al. (2011). Structure and functions of exopolysaccharide produced by gut commensal Lactobacillus reuteri 100-23. ISME J. 5, 1115–1124. doi: 10.1038/ismej.2010.201
Sinkiewicz, G. (2010). Lactobacillus reuteri in Health and Disease. Doctoral dissertation, Malmö University, Malmö.
Soto, A., Martin, V., Jimenez, E., Mader, I., Rodriguez, J. M., and Fernandez, L. (2014). Lactobacilli and bifidobacteria in human breast milk: influence of antibiotherapy and other host and clinical factors. J. Pediatr. Gastroenterol. Nutr. 59, 78–88. doi: 10.1097/MPG.0000000000000347
Spinler, J. K., Taweechotipatr, M., Rognerud, C. L., Ou, C. N., Tumwasorn, S., and Versalovic, J. (2008). Human-derived probiotic Lactobacillus reuteri demonstrate antimicrobial activities targeting diverse enteric bacterial pathogens. Anaerobe 14, 166–171. doi: 10.1016/j.anaerobe.2008.02.001
Sriramulu, D. D., Liang, M., Hernandez-Romero, D., Raux-Deery, E., Lunsdorf, H., Parsons, J. B., et al. (2008). Lactobacillus reuteri DSM 20016 produces cobalamin-dependent diol dehydratase in metabolosomes and metabolizes 1,2-propanediol by disproportionation. J. Bacteriol. 190, 4559–4567. doi: 10.1128/JB.01535-07
Stensson, M., Koch, G., Coric, S., Abrahamsson, T. R., Jenmalm, M. C., Birkhed, D., et al. (2014). Oral administration of Lactobacillus reuteri during the first year of life reduces caries prevalence in the primary dentition at 9 years of age. Caries Res. 48, 111–117. doi: 10.1159/000354412
Stevens, J. F., and Maier, C. S. (2008). Acrolein: sources, metabolism, and biomolecular interactions relevant to human health and disease. Mol. Nutr. Food Res. 52, 7–25. doi: 10.1002/mnfr.200700412
Stevens, M. J., Vollenweider, S., Meile, L., and Lacroix, C. (2011). 1,3-Propanediol dehydrogenases in Lactobacillus reuteri: impact on central metabolism and 3-hydroxypropionaldehyde production. Microb. Cell Fact. 10:61. doi: 10.1186/1475-2859-10-61
Su, M. S., and Ganzle, M. G. (2014). Novel two-component regulatory systems play a role in biofilm formation of Lactobacillus reuteri rodent isolate 100-23. Microbiology 160(Pt 4), 795–806. doi: 10.1099/mic.0.071399-0
Su, M. S., Schlicht, S., and Ganzle, M. G. (2011). Contribution of glutamate decarboxylase in Lactobacillus reuteri to acid resistance and persistence in sourdough fermentation. Microb. Cell Fact. 10(Suppl. 1):S8. doi: 10.1186/1475-2859-10-S1-S8
Su, Y., Chen, X., Liu, M., and Guo, X. (2017). Effect of three lactobacilli with strain-specific activities on the growth performance, faecal microbiota and ileum mucosa proteomics of piglets. J. Anim. Sci. Biotechnol. 8:52. doi: 10.1186/s40104-017-0183-3
Sung, V., Hiscock, H., Tang, M. L., Mensah, F. K., Nation, M. L., Satzke, C., et al. (2014). Treating infant colic with the probiotic Lactobacillus reuteri: double blind, placebo controlled randomised trial. BMJ 348:g2107. doi: 10.1136/bmj.g2107
Szajewska, H., Gyrczuk, E., and Horvath, A. (2013). Lactobacillus reuteri DSM 17938 for the management of infantile colic in breastfed infants: a randomized, double-blind, placebo-controlled trial. J. Pediatr. 162, 257–262. doi: 10.1016/j.jpeds.2012.08.004
Talarico, T. L., Casas, I. A., Chung, T. C., and Dobrogosz, W. J. (1988). Production and isolation of reuterin, a growth inhibitor produced by Lactobacillus reuteri. Antimicrob. Agents Chemother. 32, 1854–1858. doi: 10.1128/AAC.32.12.1854
Talarico, T. L., and Dobrogosz, W. J. (1989). Chemical characterization of an antimicrobial substance produced by Lactobacillus reuteri. Antimicrob. Agents Chemother. 33, 674–679. doi: 10.1128/AAC.33.5.674
Talarico, T. L., and Dobrogosz, W. J. (1990). Purification and characterization of glycerol dehydratase from Lactobacillus reuteri. Appl. Environ. Microbiol. 56, 1195–1197.
Taranto, M. P., Vera, J. L., Hugenholtz, J., De Valdez, G. F., and Sesma, F. (2003). Lactobacillus reuteri CRL1098 produces cobalamin. J. Bacteriol. 185, 5643–5647. doi: 10.1128/JB.185.18.5643-5647.2003
Thomas, C. M., Hong, T., van Pijkeren, J. P., Hemarajata, P., Trinh, D. V., Hu, W., et al. (2012). Histamine derived from probiotic Lactobacillus reuteri suppresses TNF via modulation of PKA and ERK signaling. PLoS One 7:e31951. doi: 10.1371/journal.pone.0031951
Thomas, C. M., Saulnier, D. M., Spinler, J. K., Hemarajata, P., Gao, C., Jones, S. E., et al. (2016). FolC2-mediated folate metabolism contributes to suppression of inflammation by probiotic Lactobacillus reuteri. Microbiologyopen 5, 802–818. doi: 10.1002/mbo3.371
Tsokos, G. C. (2011). Systemic lupus erythematosus. N. Engl. J. Med. 365, 2110–2121. doi: 10.1056/NEJMra1100359
Tubelius, P., Stan, V., and Zachrisson, A. (2005). Increasing work-place healthiness with the probiotic Lactobacillus reuteri: a randomised, double-blind placebo-controlled study. Environ. Health 4:25. doi: 10.1186/1476-069X-4-25
Tzang, B. S., Liu, C. H., Hsu, K. C., Chen, Y. H., Huang, C. Y., and Hsu, T. C. (2017). Effects of oral Lactobacillus administration on antioxidant activities and CD4+CD25+forkhead box P3 (FoxP3)+ T cells in NZB/W F1 mice. Br. J. Nutr. 118, 333–342. doi: 10.1017/S0007114517002112
Valeur, N., Engel, P., Carbajal, N., Connolly, E., and Ladefoged, K. (2004). Colonization and immunomodulation by Lactobacillus reuteri ATCC 55730 in the human gastrointestinal tract. Appl. Environ. Microbiol. 70, 1176–1181. doi: 10.1128/AEM.70.2.1176-1181.2004
Walter, J., Britton, R. A., and Roos, S. (2011). Host-microbial symbiosis in the vertebrate gastrointestinal tract and the Lactobacillus reuteri paradigm. Proc. Natl. Acad. Sci. U.S.A. 108(Suppl. 1), 4645–4652. doi: 10.1073/pnas.1000099107
Walter, J., Chagnaud, P., Tannock, G. W., Loach, D. M., Dal Bello, F., Jenkinson, H. F., et al. (2005). A high-molecular-mass surface protein (Lsp) and methionine sulfoxide reductase B (MsrB) contribute to the ecological performance of Lactobacillus reuteri in the murine gut. Appl. Environ. Microbiol. 71, 979–986. doi: 10.1128/AEM.71.2.979-986.2005
Walter, J., Loach, D. M., Alqumber, M., Rockel, C., Hermann, C., Pfitzenmaier, M., et al. (2007). D-alanyl ester depletion of teichoic acids in Lactobacillus reuteri 100-23 results in impaired colonization of the mouse gastrointestinal tract. Environ. Microbiol. 9, 1750–1760. doi: 10.1111/j.1462-2920.2007.01292.x
Walter, J., Schwab, C., Loach, D. M., Ganzle, M. G., and Tannock, G. W. (2008). Glucosyltransferase A (GtfA) and inulosucrase (Inu) of Lactobacillus reuteri TMW1.106 contribute to cell aggregation, in vitro biofilm formation, and colonization of the mouse gastrointestinal tract. Microbiology 154(Pt 1), 72–80. doi: 10.1099/mic.0.2007/010637-0
Wang, B., Wei, H., Yuan, J., Li, Q., Li, Y., Li, N., et al. (2008). Identification of a surface protein from Lactobacillus reuteri JCM1081 that adheres to porcine gastric mucin and human enterocyte-like HT-29 cells. Curr. Microbiol. 57, 33–38. doi: 10.1007/s00284-008-9148-2
Wang, P., Li, Y., Xiao, H., Shi, Y., Le, G. W., and Sun, J. (2016). Isolation of lactobacillus reuteri from Peyer’s patches and their effects on sIgA production and gut microbiota diversity. Mol. Nutr. Food Res. 60, 2020–2030. doi: 10.1002/mnfr.201501065
Wang, Y., Ganzle, M. G., and Schwab, C. (2010). Exopolysaccharide synthesized by Lactobacillus reuteri decreases the ability of enterotoxigenic Escherichia coli to bind to porcine erythrocytes. Appl. Environ. Microbiol. 76, 4863–4866. doi: 10.1128/AEM.03137-09
Wang, Z., Wang, L., Chen, Z., Ma, X., Yang, X., Zhang, J., et al. (2016). In vitro evaluation of swine-derived Lactobacillus reuteri: probiotic properties and effects on intestinal porcine epithelial cells challenged with enterotoxigenic Escherichia coli K88. J. Microbiol. Biotechnol. 26, 1018–1025. doi: 10.4014/jmb.1510.10089
Wanke, M., and Szajewska, H. (2012). Lack of an effect of Lactobacillus reuteri DSM 17938 in preventing nosocomial diarrhea in children: a randomized, double-blind, placebo-controlled trial. J. Pediatr. 161, 40.e1–43.e1. doi: 10.1016/j.jpeds.2011.12.049
Weizman, Z., Abu-Abed, J., and Binsztok, M. (2016). Lactobacillus reuteri DSM 17938 for the management of functional abdominal pain in childhood: a randomized, double-blind, placebo-controlled trial. J. Pediatr. 174, 160.e1–164.e1. doi: 10.1016/j.jpeds.2016.04.003
Weizman, Z., and Alsheikh, A. (2006). Safety and tolerance of a probiotic formula in early infancy comparing two probiotic agents: a pilot study. J. Am. Coll. Nutr. 25, 415–419. doi: 10.1080/07315724.2006.10719554
Weizman, Z., Asli, G., and Alsheikh, A. (2005). Effect of a probiotic infant formula on infections in child care centers: comparison of two probiotic agents. Pediatrics 115, 5–9. doi: 10.1542/peds.2004-1815
Wolf, B. W., Wheeler, K. B., Ataya, D. G., and Garleb, K. A. (1998). Safety and tolerance of Lactobacillus reuteri supplementation to a population infected with the human immunodeficiency virus. Food Chem. Toxicol. 36, 1085–1094. doi: 10.1016/S0278-6915(98)00090-8
Yang, F., Wang, A., Zeng, X., Hou, C., Liu, H., and Qiao, S. (2015). Lactobacillus reuteri I5007 modulates tight junction protein expression in IPEC-J2 cells with LPS stimulation and in newborn piglets under normal conditions. BMC Microbiol. 15:32. doi: 10.1186/s12866-015-0372-1
Yang, G., Tian, J., and Li, J. (2007). Fermentation of 1,3-propanediol by a lactate deficient mutant of Klebsiella oxytoca under microaerobic conditions. Appl. Microbiol. Biotechnol. 73, 1017–1024. doi: 10.1007/s00253-006-0563-7
Yang, Y., Zhao, X., Le, M. H., Zijlstra, R. T., and Ganzle, M. G. (2015). Reutericyclin producing Lactobacillus reuteri modulates development of fecal microbiota in weanling pigs. Front. Microbiol. 6:762. doi: 10.3389/fmicb.2015.00762
Yoo, B. B., and Mazmanian, S. K. (2017). The enteric network: interactions between the immune and nervous systems of the gut. Immunity 46, 910–926. doi: 10.1016/j.immuni.2017.05.011
Yurkovetskiy, L., Burrows, M., Khan, A. A., Graham, L., Volchkov, P., Becker, L., et al. (2013). Gender bias in autoimmunity is influenced by microbiota. Immunity 39, 400–412. doi: 10.1016/j.immuni.2013.08.013
Zelante, T., Iannitti, R. G., Cunha, C., De Luca, A., Giovannini, G., Pieraccini, G., et al. (2013). Tryptophan catabolites from microbiota engage aryl hydrocarbon receptor and balance mucosal reactivity via interleukin-22. Immunity 39, 372–385. doi: 10.1016/j.immuni.2013.08.003
Zhang, D., Ji, H., Liu, H., Wang, S., Wang, J., and Wang, Y. (2016). Changes in the diversity and composition of gut microbiota of weaned piglets after oral administration of Lactobacillus or an antibiotic. Appl. Microbiol. Biotechnol. 100, 10081–10093. doi: 10.1007/s00253-016-7845-5
Zhang, H., Liao, X., Sparks, J. B., and Luo, X. M. (2014). Dynamics of gut microbiota in autoimmune lupus. Appl. Environ. Microbiol. 80, 7551–7560. doi: 10.1128/AEM.02676-14
Keywords: Lactobacillus reuteri, probiotic, microbiota, immune system, inflammatory diseases
Citation: Mu Q, Tavella VJ and Luo XM (2018) Role of Lactobacillus reuteri in Human Health and Diseases. Front. Microbiol. 9:757. doi: 10.3389/fmicb.2018.00757
Received: 29 September 2017; Accepted: 04 April 2018;
Published: 19 April 2018.
Edited by:
Rustam Aminov, University of Aberdeen, United KingdomReviewed by:
Julio Galvez, Universidad de Granada, SpainMichael Gänzle, University of Alberta, Canada
Teresa Zotta, Consiglio Nazionale Delle Ricerche (CNR), Italy
Copyright © 2018 Mu, Tavella and Luo. This is an open-access article distributed under the terms of the Creative Commons Attribution License (CC BY). The use, distribution or reproduction in other forums is permitted, provided the original author(s) and the copyright owner are credited and that the original publication in this journal is cited, in accordance with accepted academic practice. No use, distribution or reproduction is permitted which does not comply with these terms.
*Correspondence: Xin M. Luo, eGlubHVvQHZ0LmVkdQ==