- Instituto de Investigação e Tecnologias Agrárias e do Ambiente, Universidade dos Açores, Angra do Heroísmo, Portugal
In the last years, consumers are becoming increasingly aware of the human health risk posed by the use of chemical preservatives in foods. In contrast, the increasing demand by the dairy industry to extend shelf-life and prevent spoilage of dairy products has appeal for new preservatives and new methods of conservation. Bacteriocins are antimicrobial peptides, which can be considered as safe since they can be easily degraded by proteolytic enzymes of the mammalian gastrointestinal tract. Also, most bacteriocin producers belong to lactic acid bacteria (LAB), a group that occurs naturally in foods and have a long history of safe use in dairy industry. Since they pose no health risk concerns, bacteriocins, either purified or excreted by bacteriocin producing strains, are a great alternative to the use of chemical preservatives in dairy products. Bacteriocins can be applied to dairy foods on a purified/crude form or as a bacteriocin-producing LAB as a part of fermentation process or as adjuvant culture. A number of applications of bacteriocins and bacteriocin-producing LAB have been reported to successful control pathogens in milk, yogurt, and cheeses. One of the more recent trends consists in the incorporation of bacteriocins, directly as purified or semi-purified form or in incorporation of bacteriocin-producing LAB into bioactive films and coatings, applied directly onto the food surfaces and packaging. This review is focused on recent developments and applications of bacteriocins and bacteriocin-producing LAB for reducing the microbiological spoilage and improve safety of dairy products.
Introduction
Bacteriocins are generally defined as peptides or proteins ribosomal synthesized by bacteria that inhibit or kill other related or unrelated microorganisms (Leroy and De Vuyst, 2004; Cotter et al., 2005). Bacteriocins may have a narrow spectrum, by inhibiting bacteria taxonomically close, or a broad spectrum, by inhibiting a wide variety of bacteria (Cotter et al., 2005; Mills et al., 2011).
For the past years, bacteriocins have attracted considerable interest for their use as safe food preservatives, as they are easily digested by the human gastrointestinal tract (Mills et al., 2011). The use of bacteriocins as natural food preservatives fulfills consumer demands for high quality and safe foods without the use of chemical preservatives. However, the application of bacteriocins as food additives can be limited for various reasons, such as effectiveness of pathogen elimination or its high price (Chen and Hoover, 2003). Nevertheless, research interest in bacteriocins has continued over the past years, as investigators continuing to search for new and more effective bacteriocins to address both biologic and economic concerns.
The application of bacteriocins for biopreservation of foods usually includes the following approaches: inoculation of food with the bacteriocin-producer strain; addition of purified or semi-purified bacteriocin as food additive; and use of a product previously fermented with a bacteriocin-producing strain as an ingredient in food processing (Chen and Hoover, 2003).
An increasingly number of bacteriocins have been isolated and identified from Gram-positive and Gram-negative microorganisms. As a result, databases have been created to compile the information that can be used for the automated screening of bacteriocin gene clusters (Blin et al., 2013; van Heel et al., 2013).
Classification of Bacteriocins
For the past years, several classifications of bacteriocins have been proposed taking into consideration the first classification proposed by Klaenhammer (1993). Recently, in order to classify novel bacteriocins, Alvarez-Sieiro et al. (2016) proposed an adjusted classification scheme based on the biosynthesis mechanism and biological activity in accordance with other proposals (Arnison et al., 2013). They propose three major classes: Class I – small post-translationally modified peptides; Class II – unmodified bacteriocins; and Class III – larger peptides (>10 kDa, thermo-labile), being each one subdivided into subclasses.
Mode of Action
Bacteriocins have distinct mechanisms of action and can be divided into those that promote a bactericidal effect, with or without cell lysis, or bacteriostatic, inhibiting cell growth (da Silva Sabo et al., 2014). Most of the bacteriocins produced from LAB, in particular those inhibiting Gram-positive bacteria, exert their antibacterial effect by targeting the cell envelope-associated mechanisms (Cotter et al., 2013). Several lantibiotics and some class II bacteriocins target Lipid II, an intermediate in the peptidoglycan biosynthesis machinery within the bacterial cell envelope and, by this way they inhibit peptidoglycan synthesis (Breukink and de Kruijff, 2006). Other bacteriocins use Lipid II as a docking molecule to facilitate pore formation resulting in variation of the cytoplasm membrane potential and ultimately, cell death (Machaidze and Seelig, 2003). Nisin, the most studied lantibiotic, is capable of both mechanisms (Cotter et al., 2005). Some bacteriocins damage or kill target cells by binding to the cell envelope-associated mannose phosphotransferase system (Man-PTS) and subsequent formation of pores in the cell membrane (Cotter et al., 2013). Other bacteriocins can kill their target cells by inhibition of gene expression (Parks et al., 2007; Vincent and Morero, 2009) and protein production (Metlitskaya et al., 2006).
Bacteriocins Produced by Lab
Although there are several microorganisms that produce bacteriocins, those produced by the lactic acid bacteria (LAB) are of particular interest to the dairy industry (Egan et al., 2016). LAB have long been used in a variety of food fermentations by converting lactose to lactic acid, as well as producing additional antimicrobial molecules such as other organic acids, diacetyl, acetoin, hydrogen peroxide, antifungal peptides, and bacteriocins (Egan et al., 2016). As a result of their extensive use in traditional fermented products, most of the LAB are Generally Regarded as Safe (GRAS), granted by the American Food and Drug Agency (FDA). The European Food Safety Authority (EFSA) also granted the Qualified Presumption of Safety (QPS) status to most of the LAB genera, such as Lactococcus, Lactobacillus, Leuconostoc, Pediococcus, and some Streptococcus (EFSA, 2007). Nevertheless, species of the genus Enterococcus and some Streptococcus are pathogenic, thus, they do not have GRAS status and were not proposed for QPS status (EFSA, 2007).
Lactic acid bacteria bacteriocins are often active across a range of pH values, resistant to high temperatures and active against a range of food pathogenic and spoilage bacteria (Ahmad et al., 2017). In addition, LAB bacteriocins are sensitive to digestive proteases such as pancreatin complex, trypsin and chymotrypsin, and thus do not impact negatively on the gut microbiota (Egan et al., 2016).
Application of Purified/Semi-Purified Bacteriocins to Dairy Products
Bacteriocins have been used in the biopreservation of various foods, either alone or in combination with other methods of preservation, known as hurdle technology (De Vuyst and Leroy, 2007; Perez et al., 2014). Although results obtained from culture media may show that bacteriocins inhibit target organisms, the application of bacteriocins into foods must be tested to confirm their effectiveness. Many studies showed the potential of applying bacteriocins or bacteriocin-producing strains into foods, such as meat, dairy products, fish, alcoholic beverages, salads, and fermented vegetables (O’Sullivan et al., 2003; Ramu et al., 2015). To date, only nisin (Nisaplin, Danisco) and pediocin PA1 (MicrogardTM, ALTA 2431, Quest) have been commercialized as food preservatives (Simha et al., 2012). Although other LAB bacteriocins offered promising perspectives to be used as biopreservatives, as for instance the enterocin AS-48 (Sánchez-Hidalgo et al., 2011) or lacticin 3147 (Suda et al., 2012), no other bacteriocin has been proposed for industrial application. The screening of bacteriocins to be applied to foods requires the fulfillment of some important criteria. Producing strains should be food grade (GRAS or QPS), exhibit a broad spectrum of inhibition, present high specific activity, have no associated health risks, present beneficial effects (e.g., improve safety, quality, and flavor of foods), display heat and pH stability, and optimal solubility and stability for a particular food (Cotter et al., 2005; Leroy and De Vuyst, 2010). Various authors have reported that inactivation of several foodborne pathogens by bacteriocins may differ greatly depending on the food matrix used (Muñoz et al., 2007). Therefore, the effectiveness of different bacteriocins to foodborne pathogens must be tested in all food systems.
Recent applications of bacteriocins into dairy foods to control food-borne pathogens included the inoculation of food with LAB that produce bacteriocins (Table 1) or the addition of purified or semi-purified bacteriocins directly to food (Table 2). Applying bacteriocin-producing LAB strains as antibacterial starter cultures and protective cultures may confer an advantage over the use of semi-purified/purified bacteriocins. In most cases, bacteriocins are adsorbed into food matrices and are easily degraded, which results in a loss of antibacterial activity. Therefore, an alternative method is the incorporation of bacteriocins into food packaging films/coatings, which improve their activity and stability in complex food systems (Salgado et al., 2015). Many researchers reported the potential of using immobilized bacteriocins in the development of antimicrobial packaging films to control food-borne pathogenic bacteria, such as L. monocytogenes (Sánchez-González et al., 2013; Ibarguren et al., 2015; Narsaiah et al., 2015). In fermented foods, the contamination with L. monocytogenes is a major concern. Several listeriosis outbreaks have been linked with the consumption of dairy products, in particular soft cheeses, causing a problem to dairy industry and public health authorities (Melo et al., 2015). Despite most dairy products, in particular cheeses, being made from pasteurized milk, contamination with Listeria still occurs. Cheeses are ready to use products and are usually conserved at refrigeration temperatures that allow the survival and growth of psychrotrophic bacteria, such as L. monocytogenes. Therefore, contamination can occur in later stages of dairy product processing (Carpentier and Cerf, 2011; Melo et al., 2015). Consequently, Listeria active bacteriocins emerge as an ideal solution for preventing the growth of this pathogen after cooking or packaging (Cotter et al., 2005). Bacteriocins can also be used to control adventitious non-starter flora, such as non-starter lactic acid bacteria (NSLAB) in cheese and wine, and in this way, contribute to the quality of the final product (Oumer et al., 2001; O’Sullivan et al., 2003). In addition, bacteriocins can also be used to enhance food fermentation, accelerating cheese ripening, and even improve its flavor (Oumer et al., 2001).
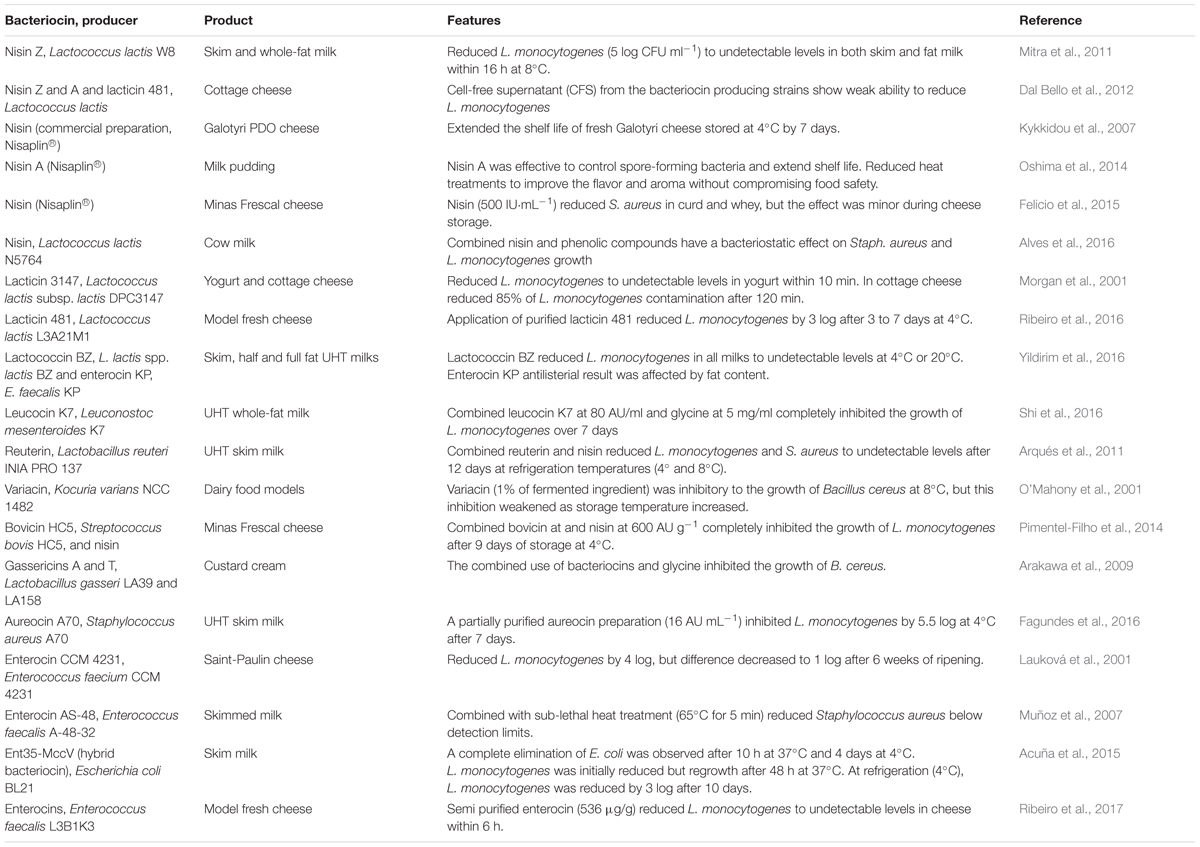
TABLE 2. Applications of purified/semi-purified bacteriocins to control food-borne pathogens in dairy products (2000–present).
Over 230 bacteriocins produced by LAB have been isolated and reported, but only half of them were identified at the protein or DNA levels (Alvarez-Sieiro et al., 2016). Moreover, a limited number of purified or semi-purified bacteriocins have been tested in food systems, especially in dairy foods.
Nisin
The bacteriocin nisin is classified as a class-Ia bacteriocin or lantibiotic and is the most characterized and commercially important bacteriocin (Ross et al., 2002). Nisin is licensed as a food preservative (E234) and is recognized to be safe by the Joint Food and Agriculture Organization/World Health Organization (FAO/WHO) Expert Committee on Food Additives (FAO and WHO, 2006; Favaro et al., 2015). To date, eight types of nisin variants were discovered and characterized: nisins A, Z, F, and Q produced by Lactococcus lactis and nisins U, U2, P, and H produced by some Streptococcus strains (O’Connor et al., 2015). The commercially available form of nisin for use as a food preservative is NisaplinTM, with the active ingredient nisin A (2.5%) and other ingredients such as NaCl and non-fat dry milk (Chen and Hoover, 2003).
Nisin has antimicrobial activity against numerous Gram-positive bacteria, including LAB, pathogens such as Listeria and Staphylococcus, and the spore forming bacteria, Bacillus and Clostridium (Chen and Hoover, 2003). One of the earliest applications of nisin was to prevent late blowing in cheese caused by gas-producing Clostridium spp. (Galvez et al., 2008).
Nisin has been widely applied in cheese and pasteurized cheese spreads to replace nitrate for preventing the outgrowth of clostridia spores (Abee et al., 1995; Chen and Hoover, 2003). Nisin has also been shown to be effective in the control of different pathogens such as L. monocytogenes and Staphylococcus aureus in dairy products (Sobrino-López and Martín-Belloso, 2008). In a study of Arqués et al. (2011), nisin was shown to reduce L. monocytogenes and S. aureus in milk stored at refrigeration temperatures. Several studies also tested the addition of nisin to diverse cheese types (cottage cheese, cheddar, and ricotta-type cheeses) and show an effective reduction of L. monocytogenes growth, although limited to 1–3 log cycles (Chen and Hoover, 2003). Several reports indicate that nisin A is not very active against L. monocytogenes, but its anti-listerial effect is enhanced by the reduction of pH and addition of NaCl (Chen and Hoover, 2003; Khan and Oh, 2016).
In Minas Frescal cheese, S. aureus counts were reduced by approximately 1.5 log cycles after addition of nisin (Felicio et al., 2015). In processed cheese, Bacillus cereus and Bacillus subtilis were also inhibited by nisin (Sobrino-López and Martín-Belloso, 2008). The use of nisin as an antimicrobial treatment extended the shelf-life of a Greek soft acid–curd cheese (Galotyri) by >21 days (Kykkidou et al., 2007). Incorporation of nisin (at a level of 2.5 mg l-1) has also shown to increase shelf-life of Ricotta-type cheese by inhibiting the growth of L. monocytogenes for >8 weeks (Davies et al., 1997). Moreover, a high level of retention of nisin was observed over the 10-week storage period, with only 10–32% of nisin loss. Ferreira and Lund (1996) also studied the effect of nisin on survival of the most resistant strains of L. monocytogenes in cottage cheese and showed a reduction to approximately 3 log cycles in 3 days. This bacteriocin was also tested in other pasteurized dairy products, such as chilled desserts, flavored milk, clotted cream, and canned evaporated milks, and was shown to reduce post process contaminating bacteria such as L. monocytogenes (Galvez et al., 2008). The efficacy of a combination of nisin and bovicin HC5 against L. monocytogenes and S. aureus in fresh cheese was studied by Pimentel-Filho et al. (2014). They observed a reduction of L. monocytogenes to undetected levels after 9 days of storage at 4°C, although the combination of bacteriocins did not prevent the growth of S. aureus.
Several studies showed that the antimicrobial activity of nisin is affected by several factors including pH, temperature, composition, structure, and natural microbiota of food (Zhou et al., 2014). Proteolysis in cheese-making process may also affect the activity of nisin and limit its antimicrobial efficacy. Nevertheless, some authors described a limited loss in nisin activity (10–32%) in Ricotta cheese after 10 weeks of storage (Cleveland et al., 2001). Moreover, nisin activity was not affected by proteases in a study on Emmental cheese (Favaro et al., 2015).
The application of nisin in dairy products is also limited to pH values lower than 7, as nisin greatly loses the activity at higher pH (de Arauz et al., 2009). Other studies also mention several limitations on the use of nisin in dairy products, due to its interaction with fat and other components in the food matrix (Favaro et al., 2015). However, the role of fat in the activity of nisin is not entirely clear, as studies on heat-treated cream show the inhibition of B. cereus growth by low concentrations of nisin (Nissen et al., 2001). Additionally, nisin was found to prevent spoilage bacteria and extend shelf-life in high-fat milk-based pudding (Oshima et al., 2014). In contrast, homogenization of milk was show to reduce the anti-listerial effects of nisin, demonstrating that the treatment of foods may play an important role in the efficacy of bacteriocins such as nisin (Bhatti et al., 2004).
The wide spectrum of inhibition associated with nisin includes LAB themselves (Abee et al., 1995). Therefore, in dairy foods which require LAB for fermentation processes, the application of nisin presents a great limitation. An alternative consists in employing other bacteriocins with a highly specific activity range (Abee et al., 1995). As a result, there has been a great interest in the search for new bacteriocins with a widespread range of antibacterial activity, stability in different food environments, tolerance to heat, and resistance to proteolytic enzymes.
Pediocins
Pediocins are a class IIa bacteriocins produced by Pediococcus spp. and are commercially available under the name Alta 2341TM or MicrogardTM (Garsa et al., 2014). This bacteriocin has been shown to be more effective than nisin against some food-borne pathogens such as L. monocytogenes and S. aureus (Cintas et al., 1998; Eijsink et al., 1998) and Gram-negative organisms such as Pseudomonas and Escherichia coli (Jamuna and Jeevaratnam, 2004). The potential application of pediocins to dairy products is further enhanced by its stability in aqueous solutions, its wide pH range, and high resistance to heating or freezing (Sobrino-López and Martín-Belloso, 2008). Despite this high potential, few studies have investigated the addition of pediocins to milk or dairy foods. Pediocin (PA-1) was found to reduce L. monocytogenes counts in cottage cheese, cream, and cheese sauce (Pucci et al., 1988). The anti-listerial effect was noticeable over a wide temperature and pH ranges and was particularly effective at low initial L. monocytogenes contamination (102 cfu ml-1). Recently, Verma et al. (2017) described the production of food-grade pediocin from supplemented cheese whey medium. This semi-purified pediocin containing fermented cheese whey was shown to be effective in reducing S. aureus counts and enhancing shelf-life of raw buffalo milk (Verma et al., 2017).
Lacticins
Lacticins are produced by certain strains of Lc. lactis and comprise lacticin 3147 and lacticin 481 (Piard et al., 1992; McAuliffe et al., 1998).
Lacticin 3147 was isolated from an Irish kefir grain used for making buttermilk and is a two-component lantibiotic, requiring both structural proteins to give full biological activity (McAuliffe et al., 1998). This bacteriocin exhibit antimicrobial activity against a wide range of food pathogenic and food spoilage bacteria in addition to other LAB (Sobrino-López and Martín-Belloso, 2008; Martínez-Cuesta et al., 2010). A lacticin 3147 powder preparation was shown to be effective for the control of Listeria and Bacillus in infant milk formulation, natural yogurt, and cottage cheese (Morgan et al., 2001). However, the high concentrations of lacticin powder used, which represent 10% of product weight, were considered impracticable and non-economic by the authors (Morgan et al., 2001).
Lacticin 481 is a single-peptide lantibiotic that exhibits a medium spectrum of inhibition, mainly active against other LAB, Clostridium tyrobutyricum (O’Sullivan et al., 2003) and L. monocytogenes (Ribeiro et al., 2016). The use of non-purified lacticin 481 was show to have a mild bacteriostatic activity in milk stored at refrigeration temperatures (Arqués et al., 2011). Yet, the application of semi-purified lacticin 481 to fresh cheeses stored at refrigeration temperatures reduced L. monocytogenes by 3 log cycles in 3–7 days (Ribeiro et al., 2016). Nevertheless, the application of lacticins in food systems is not likely to ensure complete elimination of pathogens such as L. monocytogenes.
Enterocins
Enterocins are produced by Enterococcus species and comprise a diverse group of bacteriocins, both in terms of their classification and inhibitory spectrum (Egan et al., 2016).
Although most LAB have the GRAS status and can be used safely in food applications, bacteriocinogenic enterococci raise some safety concerns (EFSA, 2007). Enterococci are among the pathogens that have been associated with a number of infections in humans (Moreno et al., 2006). In addition, some enterococci may harbor virulence determinants and antibiotic resistance genes (EFSA, 2007). While food enterococci have fewer virulence determinants than clinical strains, they are known for their capacity to exchange genetic information (Eaton and Gasson, 2001). Considering the safe concerns of using bacteriocinogenic enterococci, the use of purified enterocins may be considered more suitable for food consumption.
Enterocin AS-48 is produced by Enterococcus faecalis and is a class IIc cyclic bacteriocin that is active against a number of Bacillus and Clostridium sp. (Egan et al., 2016). This is one of most studied bacteriocins, showing high stability to pH and heat, which makes a great candidate for application in food. Muñoz et al. (2007) tested the efficacy of the enterocin AS-48 for controlling staphylococci in skimmed milk. They observed a bactericidal effect proportional to the bacteriocin concentration (10–50 μg ml-1), but complete elimination of staphylococci was not achieved for any of the concentrations tested. This apparently lower effectiveness of AS-48 in milk when compared to culture medium could be attributed to a higher retention of the bacteriocin molecules by milk components and slower diffusion (Muñoz et al., 2007).
Yildirim et al. (2016) investigated the antimicrobial effects of enterocin KP toward L. monocytogenes in skim, half fat, and full fat milks. Enterocin KP had a high anti-listerial effect, but this bactericidal effect decreased as both the fat content of milk and inoculation amount of L. monocytogenes increased. Also, Lauková and Czikková (1999) reported an inhibitory effect of purified enterocin CCM 4231 (3200 AU ml-1) on the growth of S. aureus and L. monocytogenes in skimmed milk and yogurt. Still, the antagonistic effect of enterocin on S. aureus in yogurt was less influential after 24 h.
Usually, enterocins are active against foodborne pathogens, such as Listeria spp. and Clostridium spp., but their application in food systems is not likely to prevent the re-growth of pathogens throughout the storage time (Zacharof and Lovitt, 2012; de Souza Barbosa et al., 2015). However, Arqués et al. (2011) observed the reduction of L. monocytogenes counts in milk below the detection limit after 4 and 24 h by the combined effect of two bacteriocins, reuterin and enterocin AS-48.
Recently, Ribeiro et al. (2017) reported the efficacy of a semi-purified enterocin produced by an E. faecalis strain, in reducing the contamination of L. monocytogenes in fresh cheese in a dose-dependent manner. Moreover, the highest dose applied to cheeses (approximately 2000 AU g-1 of cheese) resulted in reduction of this pathogen below detection levels and this effect remained for all the storage time (72 h).
Other Bacteriocins
Lactococcin BZ is produced by some strains of Lc. lactis spp. (lactis BZ) and has a wide antibacterial activity against Gram-positive and Gram-negative bacteria (Şahingil et al., 2011). This bacteriocin also displayed strong anti-listerial activity in milk. The partially purified lactococcin BZ (400–2500 AU ml-1) reduced L. monocytogenes counts to an undetectable level in both skim and full-fat milk during storage at 4 and 20°C (Yildirim et al., 2016). In addition, this anti-listerial activity was stable until the end of the storage period (25 days) and was not adversely affected by milk fat content.
Aureocin A70 is a class II bacteriocin produced by a S. aureus strain isolated from pasteurized commercial milk (Fagundes et al., 2016). This bacteriocin exerted a bactericidal effect on a wide range of Gram-positive bacteria, including L. monocytogenes, and was tested in UHT-treated skimmed milk by Fagundes et al. (2016). Aureocin A70 caused a time-dependent reduction in L. monocytogenes counts (5.51 log units) up to 7 days of incubation, but was insufficient to achieve the total elimination of the pathogen, resulting in <0.001% survivors (Fagundes et al., 2016).
Genetically engineered bacteriocins have been proposed to overcome the narrow range of activity of most bacteriocins. Acuña et al. (2012) reported the construction of a chimerical bacteriocin named Ent35-MccV. This hybrid bacteriocin combines in a single molecule the anti-listerial activity of enterocin CRL35 and the anti-E. coli activity of microcin V. This hybrid wide-spectrum bacteriocin was active against pathogenic strains of L. monocytogenes and E. coli O157:H7 and was effective in controlling the growth of both pathogens in skim milk (Acuña et al., 2015).
Application of Bacteriocin-Producing Bacteria to Dairy Products
Despite the recent advances in bacteriocin research for food applications, the use of purified bacteriocins in the dairy industry remains limited. Frequently, the application of a bacteriocin alone does not provide sufficient protection against microbial contamination of dairy products. The high cost of bacteriocin isolation and purification also limits the commercial exploration of new bacteriocins. In addition, the restrictive food legislation of the health regulatory authorities (FDA and EFSA) limits the approval of new bacteriocins as food preservatives and, as a consequence, only two bacteriocins (nisin and pediocin) are currently commercially available.
The use of bacteriocin-producing bacteria to control contamination microorganisms is an alternative for the use of purified bacteriocins as food additives. Many LAB genera and species have a long history of apparent safe use and they have been granted the GRAS and QPS status. In this regard, incorporation of such bacteria into foods offers a viable solution for controlling contamination microorganisms (Table 2). In addition, LAB are commonly used as starter cultures in food fermentations. Thus, researchers have explored the in situ production of bacteriocins by adding protective cultures that may grow and produce bacteriocins during the manufacture and storage of dairy foods. Many studies have also focused on the selection and development of bacteriocinogenic cultures as cell lysis-inducing agents to improve cheese maturation and flavor (Beshkova and Frengova, 2012). In addition, the use of bacteriocin-producing LAB has been proposed to prevent late blowing occurring in cheeses. Late blowing defect is a major cause of spoilage in ripened cheeses, resulting in the appearance of texture and flavor defects, due to the ubiquitous presence of Clostridium spores (Gómez-Torres et al., 2015). The most common strategies to reduce Clostridium spores are often not sufficient to prevent late blowing in cheeses (Garde et al., 2011) and the use of bacteriocinogenic LAB emerges as an alternative strategy (Table 3).
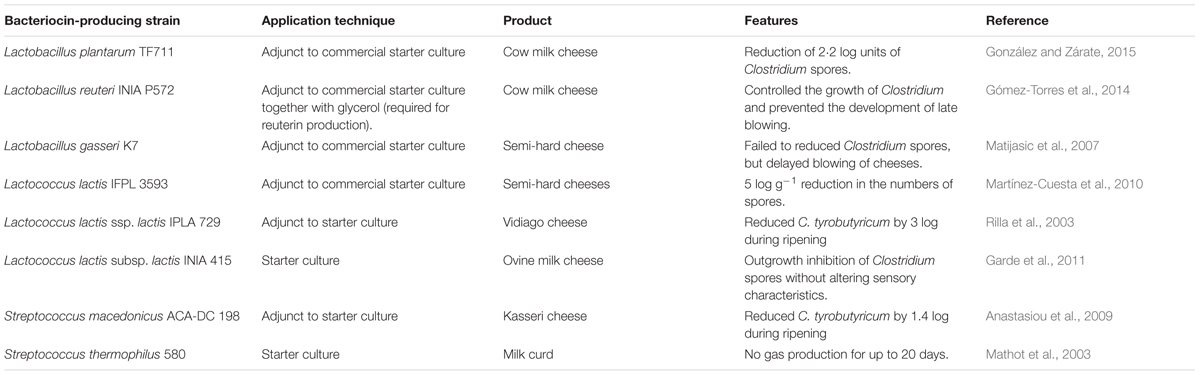
TABLE 3. Applications of bacteriocin-producing LAB to prevent gas blowing in cheese caused by Clostridium spp. (2000–present).
Among the various LAB species/strains producing bacteriocins, Lactococcus sp. has gained a particular interest in the biopreservation of dairy foods. Benkerroum et al. (2002) tested the effect of in situ bacteriocin production of L. lactis ssp. lactis against L. monocytogenes, in a traditional fermented milk (lben). They found that L. monocytogenes decreased to below the detectable level within 24 h of storage at 7°C in lben fermented with the bacteriogenic starter culture. Moreover, the pathogen was efficiently inactivated from contaminated samples despite the high level of contamination (107 cfu ml-1) for up to 6 days of storage at 7°C (Benkerroum et al., 2002).
The application of nisin-producing Lactococcus sp. in dairy foods which requires LAB starters presents a problem, because the wide spectrum of inhibition associated with nisin includes LAB themselves (Abee et al., 1995). Still, Yamauchi et al. (1996) produced a yogurt by incorporating a nisin-producing strain, L. lactis subsp. lactis, in raw milk. The bacteriocin-producing LAB were killed before the addition of the traditional yogurt cultures, and this resulted in increased storage life of the yogurts by preventing the growth of spoilage bacteria (Yamauchi et al., 1996).
Recently, Kondrotiene et al. (2018) reported a reduction in L. monocytogenes when three nisin A producing Lc. lactis strains were added to fresh cheese. However, the reduction on Listeria contamination was limited to 2 log units within 7 days of cheese storage. Likewise, the use of a nisin A-producing Lactococcus diacetylactis in a mixed starter culture was not effective in inhibiting L. innocua growth in Cheddar cheese (Benech et al., 2002). Maisnier-Patin et al. (1992) examined the inhibitory effect of another nisin-producing Lc. lactis on L. monocytogenes in Camembert cheese. In the presence of nisin-producing starter, the numbers of the pathogen decreased until the end of the second week, leading to a reduction of 3 log cfu g-1, but a regrowth of Listeria was observed in cheeses throughout ripening (6 weeks).
A reduction of L. monocytogenes counts was observed in raw milk cheese by the use of two nisin-producing Lactobacillus lactis subsp. lactis as starter cultures (Rodrígez et al., 2001). In contrast, the use of nisin-producing strains to control E. coli and L. monocytogenes in Feta and Camembert cheeses has shown limited results (Ramsaran et al., 1998). E. coli O157:H7 survived the manufacturing process of both cheeses and was present in cheese made with nisin-producing strains at the end of 75 days of storage in greater numbers than the initial inoculum. The Feta cheese that contained nisin was the only cheese in which L. monocytogenes remained at the level of the initial inoculum after 75 days of storage. The use of bacteriocinogenic pediococci in dairy products also exhibited limited results due to their inability to ferment lactose (Renye et al., 2011).
Mills et al. (2011) tested a plantaricin-producing Lactobacillus plantarum strain as an anti-listerial adjunct in the presence and absence of nisin-producing starters for the manufacture of cheeses. The combination of Lb. plantarum strain (at 108 cfu ml-1) with a nisin producer reduced Listeria to undetectable levels by day 28. Moreover, they found that Lb. plantarum was much more effective at inhibiting Listeria than the nisin producer alone.
Carnio et al. (2000) studied the anti-listerial potential of a food-grade strain Staphylococcus equorum, producing a bacteriocin named as micrococcin P1, in soft cheese. A remarkable reduction of L. monocytogenes growth was achieved, but this effect was dependent on the contamination level. In addition, a regrowth of the viable Listeria could be observed after 10–16 days of maturation. Likewise, Dal Bello et al. (2012) detected a reduction of L. monocytogenes by 3 log units in cottage cheese, after incubation with a lacticin-481-producing Lc. lactis strain. However, after an initial period, L. monocytogenes counts increased to values comparable to the control.
In addition to natural bacteriocinogenic strains, the heterologous production of bacteriocin by genetically engineered LAB was tested in dairy foods (Leroy and De Vuyst, 2004). A lacticin 3147 transconjugant generated via conjugation of bacteriocin-encoding plasmids was used successfully against L. monocytogenes in cottage cheese (McAuliffe et al., 1999). Reductions of 99.9% were seen in cottage cheese held at 4°C after 5 days. In another study, a lacticin 3147 transconjugant presented also a protective effect when applied to the cheese surface, reducing L. monocytogenes by 3 log units (Ross et al., 2000). In contrast, this protective effect was not evident when a nisin-producing culture was used, possibly due to pH instability (Ross et al., 1999).
The use of Enterococcus spp. in foods may represent a risk for consumers and requires a safety assessment by the European food authorities (EFSA, 2007). However, enterococci are found naturally in some dairy foods, as they are used as starter cultures and are often part of the microbiota of artisanal cheeses (Domingos-Lopes et al., 2017). In addition, some strains of bacteriocinogenic enterococci were show to lack many of the virulence determinants (Jaouani et al., 2015). De Vuyst et al. (2003) suggested that Enterococcus species could be safely used in food if virulence genes were absent. Consequently, several studies have also employed enterocin-producing enterococcus in food systems.
The inhibitory effect of enterocin-producing enterococci against L. monocytogenes and S. aureus in dairy foods such as milk and cheeses was demonstrated by several authors (Giraffa, 1995; Giraffa and Carminati, 1997; Nunez et al., 1997; Lauková et al., 1999a,b: Lauková et al., 2001). Many enterococcal strains producing enterocins were isolated and found to be effective in controlling contamination in cheeses, without compromising the acid-producing activity of the starter and the organoleptic characteristics of the final product (Khan et al., 2010). Bacteriocinogenic E. faecalis strain was show to reduce L. monocytogenes counts in Manchego cheese by 1 and 2 log units after 7 and 60 days, respectively (Nunez et al., 1997). Although inoculation of milk with E. faecalis strain reduced the rate of acid production in the curd, the flavor and bitterness of the final product were not influenced.
Other bacteriocinogenic enterococci have also been reported to reduce L. monocytogenes in dairy foods. Pingitore et al. (2012) investigated two bacteriocinogenic enterococci strains (Enterococcus mundtii CRL35 and Enterococcus faecium ST88Ch) isolated from cheeses. Growth of L. monocytogenes was inhibited in Minas cheeses containing E. mundtii up to 12 days of storage at 8°C. This bacterium displayed a bacteriostatic effect since Listeria counts remained similar to the initial inoculum. E. faecium strain was found to be less effective, as the bacteriostatic affect occurred only after 6 days at 8°C.
Further studies also demonstrated the inhibitory effect of enterocin-producing enterococci strains against L. monocytogenes in raw milk. Vandera et al. (2017) investigated the use of a multiple enterocin-producing bacterial strains possessing the structural entA, entB, and entP enterocin genes. Some strains exhibited a bacteriostatic effect on L. monocytogenes in raw milk incubated at 37°C for 6 h. When raw milk cultures were further incubated at 18°C, viable populations of the pathogen were reduced slightly (by 0.2–0.4 log cfu ml-1) after 24 h and up to 72 h.
Achemchem et al. (2006) studied the effectiveness of an E. faecium strain in controlling L. monocytogenes in goat’s milk and goat’s traditional cheese (jben). Coculture experiments of E. faecium and L. monocytogenes in milk demonstrated that the pathogen was not eliminated, but when the bacteriocinogenic strain was previously inoculated in whole milk and left to grow for 12 h before contamination, Listeria was undetectable after 130 h of coculture. Moreover, addition of the bacteriocinogenic strain to jben cheese contaminated with L. monocytogenes prior to packaging, reduced the number of viable Listeria to undetectable levels, after 1 week of storage at 22°C.
Coelho et al. (2014) examined the inhibitory effect of enterocin-producing E. faecalis strains against L. monocytogenes in fresh cheese. Inoculation of milk with bacteriocinogenic E. faecalis strains was shown to reduce L. monocytogenes counts by 3–4 log units in fresh cheese compared to the control. The combination of two enterocin producers optimized the reduction of Listeria counts in fresh cheese, decreasing this pathogen by 4 log cfu g-1 in the first 3 days of storage and by 5 log cfu g-1 on day 7.
Bacteriocin-producing LAB strains have also been assessed to improve cheese maturation and flavor. These LAB cultures may induce controlled lysis of starter and/or non-starter LAB (NSLAB) and subsequent intracellular release of proteinases and peptidases, resulting in rapid onset of proteolysis and cheese ripening. Lc. lactis producing lacticin 3147 was shown to accelerate cheese ripening and also prevent late blowing in cheese by the inhibition of clostridia growth (Martínez-Cuesta et al., 2010).
Various bacteriocin-producing strains were shown to possess a lytic effect on starter cultures. The use of bacteriocin-producing Lc. lactis ssp. cremoris as a starter adjunct in Cheddar cheese manufacture was show to increase the rate of starter lysis. Cheese manufactured with the bacteriocinogenic adjunct exhibited increased cell lysis and higher concentrations of free amino acids, with associated higher sensory evaluation scores (Morgan et al., 1997). Another bacteriocin-producing starter Lc. lactis (a lacticin 3147 producer) was tested for controlling the proliferation of undesirable microorganisms during cheese manufacture. Cheeses made with lacticin 3147-producing starters exhibited significantly lower levels of NSLAB that remained constant over 6 months of ripening (Ryan et al., 1996).
Some strains of lacticin 481-producing Lc. lactis were also tested in cheese production and showed to cause partial lysis of starter lactococcal cultures, which continue to grow at a slower rate (O’Sullivan et al., 2003). As a direct result of starter lysis with concomitant enzyme release in the cheese matrix, these strains may be employed to increase cheese ripening. Additionally, a three-strain starter system was tested on cheese by Morgan et al. (2002). This system comprised a bacteriocin producer which causes the lysis of a second strain (sensitive to bacteriocin) and a third strain resistant to bacteriocin activity, for acid production during cheese manufacture. The experimental cheese made with this three-strain starter system showed an increase in lysis and decrease in bitterness compared to cheeses manufactured without the bacteriocin-producing adjunct (Morgan et al., 2002).
Combining Bacteriocins With Other Hurdles
One of the approaches to improve the protective action of bacteriocins is the combination with other hurdles such as chemical additives (such as EDTA, sodium lactate, potassium diacetate, and others), heating, and high-pressure treatments (Egan et al., 2016).
Narayanan and Ramana (2013) observed that the use of pediocin in combination with eugenol incorporated into polyhydroxybutyrate films worked in synergized form and provided an effective hurdle preventing food contamination. Other researchers used successfully the mixture of bacteriocins and EDTA in the sensitization of Gram-negative bacteria (Prudêncio et al., 2015). Gram-negative bacteria become sensitive to bacteriocins if the permeability of their outer membrane is compromised with chelating agents, such as EDTA (Chen and Hoover, 2003). Zapico et al. (1998) demonstrated a synergistic effect of the combined use of nisin and the lactoperoxidase system (LPS) to control L. monocytogenes in skim milk. A listericidal effect (5.6 log units lower than the control milk) was observed in treatments containing nisin (10 or 100 IU ml-1) with LPS, after 24 h at 30°C. Moreover, when the two preservatives were added in two steps (LPS was added after 3 h and nisin after 5 h of growth), the difference in L. monocytogenes counts increased by 7.4 log units.
Several authors also observed the synergistic effect of bacteriocins after temperature treatments (Prudêncio et al., 2015). Kalchayanand et al. (1992) exposed bacteriocin-resistant bacteria to sub-lethal stresses, either at low or high temperatures, and treated with nisin and pediocin. They found that both bacteriocins were effective in reducing the cell viability. This synergistic effect was also observed in Gram-negative bacteria, which are normally insensitive to these bacteriocins (Boziaris et al., 1998).
High pressure processing is a common technique for inactivating microorganisms at room temperature, but this treatment does not ensure the complete inactivation of microorganisms (Prudêncio et al., 2015). Several studies have demonstrated the synergistic effect of bacteriocins such as nisin with high pressure processing on the inactivation of food microorganisms (Garriga et al., 2002; Zhao et al., 2013). It is well documented that the use of bacteriocins in combination with these processing techniques enhances bacterial inactivation (Chen and Hoover, 2003). As an example, Rodriguez et al. (2005) demonstrated the efficacy of the application of reduced pressures combined with bacteriocin-producing LAB to improve cheese safety.
Incorporation of Bacteriocins in Antimicrobial Films and Coatings
A common strategy for preservation of foods that are eaten raw or without further cooking is the application of edible films or coatings containing antimicrobial substances. The incorporation of antimicrobial compounds such as bacteriocins in edible coatings and films presents as an interesting alternative for ensuring the control of pathogenic microorganisms in food products (Valdés et al., 2017).
Edible coatings and films are composed of thin layers of biopolymers that modify the surrounding atmosphere of foods, forming a barrier between the food and the environment, improve the safety, quality, and functionality of food products without changing organoleptic and nutritional properties (Han, 2003; Valdés et al., 2017). The use of purified bacteriocins or bacteriocin-producing bacteria in the packaging system may be more effective in the inhibition of the growth of pathogenic and/or deterioration microorganisms throughout the extent of the latency phase (Balciunas et al., 2013). Hydrocolloids (proteins and polysaccharides) are the most extensively investigated biopolymers in edible coatings and films applied to cheese. They facilitate the incorporation of functional compounds such as bacteriocins and bacteriocin-producing bacteria and allow an increase in the stability, safety, and shelf-life of dairy foods (Scannell et al., 2000a).
To date, few studies have investigated the effectiveness of incorporating bacteriocins and/or bacteriocin-producing LAB in coatings and films applied to dairy products. Some authors observed an inhibition of the growth of pathogenic microorganisms in foods packed with coatings and films containing antimicrobial metabolites synthesized by LAB (Cao-Hoang et al., 2010; da Silva Malheiros et al., 2010; Ercolini et al., 2010; Aguayo et al., 2016; Malheiros et al., 2016) or containing viable LAB in the film/coating matrix (Concha-Meyer et al., 2011; Barbosa et al., 2015).
Studies of the effectiveness of incorporating purified bacteriocins in edible coatings show a limited reduction of pathogens such as L. monocytogenes. Cheeses, particularly fresh cheeses, are highly perishable due to their high content in caseins, lipids, and water. The complexity of cheese composition and its manufacture support the development of pathogenic and deteriorating microorganisms that increase the risk of foodborne illness and reduce cheese quality and acceptability (Ramos et al., 2012). By acting as additional hurdle, the application of edible coatings and films with incorporation of bacteriocins may overcome problems associated with post-process contamination, therefore enhancing the safety and extending the shelf-life of the cheese.
Cao-Hoang et al. (2010) incorporated nisin in films of sodium caseinate applied in semi-soft cheese and observed a small reduction in L. innocua counts (1.1 log cfu g-1) after a week of storage at 4°C. In another study, the incorporation of nisin and lacticin in cellulose coatings applied to Cheddar cheese reduced levels of L. innocua by 2 log cycles, and S. aureus by 1.5 log cycles (Scannell et al., 2000b). However, other studies have shown an effective reduction on pathogen growth. In Ricotta cheese coated with galactomannan and nisin, the growth of L. monocytogenes was prevented for 7 days at 4°C (Martins et al., 2010). The application of a coating in Port Salut cheese, consisting of tapioca starch combined with nisin and natamycin, reduced L. innocua counts above 10 cfu ml-1 during storage, acting as a barrier to post-process contamination (Resa et al., 2014).
Recently, Marques et al. (2017) used a biodegradable film incorporated with cell-free supernatant (CFS) containing bacteriocin-like substances of Lactobacillus curvatus P99, to control the growth of L. monocytogenes in sliced “Prato” cheese. These films containing the bactericidal concentration of CFS were able to control L. monocytogenes for 10 days of storage at 4°C.
Conclusion
This review highlights the most recent trends in the use of bacteriocins and bacteriocin-producer bacteria in dairy foods. Bacteriocins, either per se or produced by live bacteria, can be successfully incorporated into dairy products to assure safety, extending shelf-life and preserve quality. The use of purified and concentrated bacteriocins as food additives has been the preferred method due to more efficacy, compared to direct application of bacteriocinogenic cultures. However, the effectiveness of bacteriocins in food systems is often low due to several factors such as adsorption to food components, enzymatic degradation, poor solubility, or uneven distribution in the food matrix. On the other hand, the application of live bacteriocin-producing bacteria into dairy foods may overcome the limitations on the use of purified bacteriocins. The bacteriocinogenic LAB added to dairy products such as yogurts and cheeses will ensure continuous production of bacteriocins throughout maturation and storage, and may be included as starter/adjunct cultures in fermentation. The main difficulty associated with this application is the lack of compatibility between the bacteriocin-producing strain and other cultures required in the fermentation of dairy foods.
Studies on application of bacteriocins and/or bacteriocin producers have been focused mainly on cheeses because most discovered LAB bacteriocins were effective against Listeria monocytogenes. This foodborne pathogen is often a great concern in traditional cheeses made from raw milk and as a post-processing contaminant in cheeses made from pasteurized milk. Cheeses are particularly vulnerable to the contamination of L. monocytogenes because they provide the appropriate growth conditions for this pathogen. Listeriosis out-breaks linked to the consumption of contaminated cheeses have been reported worldwide. Therefore, the application of bacteriocins as natural preservatives for improving cheese safety has attracted significant research interest in recent years. In contrast, very limited research has been focused on the application of bacteriocins to the preservation of milk, cream, yogurts, and other dairy products.
Although most efforts have been devoted to discover novel bacteriocins with unique properties, more studies are necessary for effective application of bacteriocins in dairy foods in order to understand bacteriocin performance in the complex environment of food matrices. In addition, bacteriocins may be combined with other protection tools as part of hurdle technologies to ensure bio-preservation and shelf-life extension of dairy foods. Their use may lie in the combination with other preservation techniques, or in the incorporation in biofilms and active packaging. In the latter case, further studies are necessary to ensure the adaptation of edible coatings and films to bacteriocin activity and efficacy in dairy products.
Author Contributions
All authors listed have made a substantial, direct and intellectual contribution to the work, and approved it for publication.
Funding
This work was supported by Instituto de Investigação e Tecnologias Agrárias e do Ambiente (IITAA). The authors are grateful to Fundação para a Ciência e Tecnologia (FCT) UID/CVT/00153/2013. SR was grateful to “Fundo Regional para a Ciência e Tecnologia” (FRCT) Project M3.1.2/F/011/2011.
Conflict of Interest Statement
The authors declare that the research was conducted in the absence of any commercial or financial relationships that could be construed as a potential conflict of interest.
References
Abee, T., Krockel, L., and Hill, C. (1995). Bacteriocins: modes of action and potentials in food preservation and control of food poisoning. Int. J. Food Microbiol. 28, 169–185. doi: 10.1016/0168-1605(95)00055-0
Achemchem, F., Abrini, J., Martinez-Bueno, M., Valdivia, E., and Maqueda, M. (2006). Control of Listeria monocytogenes in goat’s milk and goat’s Jben by the bacteriocinogenic Enterococcus faecium F58 strain. J. Food Prot. 69, 2370–2376. doi: 10.4315/0362-028X-69.10.2370
Acuña, L., Corbalan, N. S., Fernandez-No, I. C., Morero, R. D., Barros-Velazquez, J., and Bellomio, A. (2015). Inhibitory effect of the hybrid bacteriocin Ent35-MccV on the growth of Escherichia coli and Listeria monocytogenes in model and food systems. Food Bioproc. Technol. 8, 1063–1075. doi: 10.1007/s11947-015-1469-0
Acuña, L., Picariello, G., Sesma, F., Morero, R. D., and Bellomio, A. (2012). A new hybrid bacteriocin, Ent35–MccV, displays antimicrobial activity against pathogenic Gram-positive and Gram-negative bacteria. FEBS Open Bio 2, 12–19. doi: 10.1016/j.fob.2012.01.002
Aguayo, M. D. C. L., Burgos, M. J. G., Pulido, R. P., Gálvez, A., and López, R. L. (2016). Effect of different activated coatings containing enterocin AS-48 against Listeria monocytogenes on apple cubes. Innov. Food Sci. Emerg. Technol. 35, 177–183. doi: 10.1016/j.ifset.2016.05.006
Ahmad, V., Khan, M. S., Jamal, Q. M. S., Alzohairy, M. A., Al Karaawi, M. A., and Siddiqui, M. U. (2017). Antimicrobial potential of bacteriocins: in therapy, agriculture and food preservation. Int. J. Antimicrob. Agents 49, 1–11. doi: 10.1016/j.ijantimicag.2016.08.016
Alvarez-Sieiro, P., Montalbán-López, M., Mu, D., and Kuipers, O. P. (2016). Bacteriocins of lactic acid bacteria: extending the family. Appl. Microbiol. Biotechnol. 100, 2939–2951. doi: 10.1007/s00253-016-7343-9
Alves, F. C. B., Barbosa, L. N., Andrade, B., Albano, M., Furtado, F. B., Pereira, A. F. M., et al. (2016). Short communication: inhibitory activities of the lantibiotic nisin combined with phenolic compounds against Staphylococcus aureus and Listeria monocytogenes in cow milk. J. Dairy Sci. 99, 1831–1836. doi: 10.3168/jds.2015-10025
Anastasiou, R., Aktypis, A., Georgalaki, M., Papadelli, M., De Vuyst, L., and Tsakalidou, E. (2009). Inhibition of Clostridium tyrobutyricum by Streptococcus macedonicus ACA-DC 198 under conditions mimicking Kasseri cheese production and ripening. Int. Dairy J. 19, 330–335. doi: 10.1016/j.idairyj.2008.12.001
Arakawa, K., Kawai, Y., Iioka, H., Tanioka, M., Nishimura, J., Kitazawa, H., et al. (2009). Effects of gassericins A and T, bacteriocins produced by Lactobacillus gasseri, with glycine on custard cream preservation. J. Dairy Sci. 92, 2365–2372. doi: 10.3168/jds.2008-1240
Arnison, P. G., Bibb, M. J., Bierbaum, G., Bowers, A. A., Bugni, T. S., Bulaj, G., et al. (2013). Ribosomally synthesized and post-translationally modified peptide natural products: overview and recommendations for a universal nomenclature. Nat. Prod. Rep. 30, 108–160. doi: 10.1039/c2np20085f
Arqués, J. L., Rodríguez, E., Nuñez, M., and Medina, M. (2011). Combined effect of reuterin and lactic acid bacteria bacteriocins on the inactivation of food-borne pathogens in milk. Food Control 22, 457–461. doi: 10.1016/j.foodcont.2010.09.027
Aspri, M., O’connor, P. M., Field, D., Cotter, P. D., Ross, P., Hill, C., et al. (2017). Application of bacteriocin-producing Enterococcus faecium isolated from donkey milk, in the bio-control of Listeria monocytogenes in fresh whey cheese. Int. Dairy J. 73, 1–9. doi: 10.1016/j.idairyj.2017.04.008
Avila, M., Garde, S., Gaya, P., Medina, M., and Nunez, M. (2005). Influence of a bacteriocin-producing lactic culture on proteolysis and texture of Hispanico cheese. Int. Dairy J. 15, 145–153. doi: 10.1016/j.idairyj.2004.06.009
Balciunas, E. M., Martinez, F. A. C., Todorov, S. D., De Melo Franco, B. D. G., Converti, A., and De Souza Oliveira, R. P. (2013). Novel biotechnological applications of bacteriocins: a review. Food Control 32, 134–142. doi: 10.1016/j.foodcont.2012.11.025
Barbosa, M. S., Todorov, S. D., Jurkiewicz, C. H., and Franco, B. D. (2015). Bacteriocin production by Lactobacillus curvatus MBSa2 entrapped in calcium alginate during ripening of salami for control of Listeria monocytogenes. Food Control 47, 147–153. doi: 10.1016/j.foodcont.2014.07.005
Benech, R.-O., Kheadr, E., Laridi, R., Lacroix, C., and Fliss, I. (2002). Inhibition of Listeria innocua in cheddar cheese by addition of nisin Z in liposomes or by in situ production in mixed culture. Appl. Environ. Microbiol. 68, 3683–3690. doi: 10.1128/AEM.68.8.3683-3690.2002
Benkerroum, N., Ghouati, Y., Ghalfi, H., Elmejdoub, T., Roblain, D., Jacques, P., et al. (2002). Biocontrol of Listeria monocytogenes in a model cultured milk (lben) by in situ bacteriocin production from Lactococcus lactis ssp. lactis. Int. J. Dairy Technol. 55, 145–151. doi: 10.1046/j.1471-0307.2002.00053.x
Beshkova, D., and Frengova, G. (2012). Bacteriocins from lactic acid bacteria: microorganisms of potential biotechnological importance for the dairy industry. Eng. Life Sci. 12, 419–432. doi: 10.1002/elsc.201100127
Bhatti, M., Veeramachaneni, A., and Shelef, L. A. (2004). Factors affecting the antilisterial effects of nisin in milk. Int. J. Food Microbiol. 97, 215–219. doi: 10.1016/j.ijfoodmicro.2004.06.010
Blin, K., Medema, M. H., Kazempour, D., Fischbach, M. A., Breitling, R., Takano, E., et al. (2013). antiSMASH 2.0—a versatile platform for genome mining of secondary metabolite producers. Nucleic acids Res. 41, W204–W212. doi: 10.1093/nar/gkt449
Bockelmann, W., Koslowsky, M., Goerges, S., Scherer, S., Franz, C., and Heller, K. J. (2017). Growth inhibition of Listeria monocytogenes by bacteriocin-producing Staphylococcus equorum SE3 in cheese models. Food Control 71, 50–56. doi: 10.1016/j.foodcont.2016.06.019
Boziaris, I., Humpheson, L., and Adams, M. (1998). Effect of nisin on heat injury and inactivation of Salmonella enteritidis PT4. Int. J. Food Microbiol. 43, 7–13. doi: 10.1016/S0168-1605(98)00083-X
Breukink, E., and de Kruijff, B. (2006). Lipid II as a target for antibiotics. Nat. Rev. Drug Discov. 5, 321–323. doi: 10.1038/nrd2004
Cao-Hoang, L., Chaine, A., Grégoire, L., and Waché, Y. (2010). Potential of nisin-incorporated sodium caseinate films to control Listeria in artificially contaminated cheese. Food Microbiol. 27, 940–944. doi: 10.1016/j.fm.2010.05.025
Carnio, M. C., Höltzel, A., Rudolf, M., Henle, T., Jung, G., and Scherer, S. (2000). The macrocyclic peptide antibiotic micrococcin P1 is secreted by the food-borne bacterium Staphylococcus equorumWS 2733 and Inhibits Listeria monocytogenes on Soft Cheese. Appl. Environ. Microbiol. 66, 2378–2384. doi: 10.1128/AEM.66.6.2378-2384.2000
Carpentier, B., and Cerf, O. (2011). Persistence of Listeria monocytogenes in food industry equipment and premises. Int. J. Food Microbiol. 145, 1–8. doi: 10.1016/j.ijfoodmicro.2011.01.005
Chen, H., and Hoover, D. (2003). Bacteriocins and their food applications. Compr. Rev. Food Sci. Food Saf. 2, 82–100. doi: 10.1111/j.1541-4337.2003.tb00016.x
Cintas, L., Casaus, P., Fernández, M., and Hernández, P. (1998). Comparative antimicrobial activity of enterocin L50, pediocin PA-1, nisin A and lactocin S against spoilage and foodborne pathogenic bacteria. Food Microbiol. 15, 289–298. doi: 10.1006/fmic.1997.0160
Cleveland, J., Montville, T. J., Nes, I. F., and Chikindas, M. L. (2001). Bacteriocins: safe, natural antimicrobials for food preservation. Int. J. Food Microbiol. 71, 1–20. doi: 10.1016/S0168-1605(01)00560-8
Cocolin, L., Foschino, R., Comi, G., and Fortina, M. G. (2007). Description of the bacteriocins produced by two strains of Enterococcus faecium isolated from Italian goat milk. Food Microbiol. 24, 752–758. doi: 10.1016/j.fm.2007.03.001
Coelho, M., Silva, C., Ribeiro, S., Dapkevicius, M., and Rosa, H. (2014). Control of Listeria monocytogenes in fresh cheese using protective lactic acid bacteria. Int. J. Food Microbiol. 191, 53–59. doi: 10.1016/j.ijfoodmicro.2014.08.029
Concha-Meyer, A., Schöbitz, R., Brito, C., and Fuentes, R. (2011). Lactic acid bacteria in an alginate film inhibit Listeria monocytogenes growth on smoked salmon. Food Control 22, 485–489. doi: 10.1016/j.foodcont.2010.09.032
Cotter, P. D., Hill, C., and Ross, R. P. (2005). Bacteriocins: developing innate immunity for food. Nat. Rev. Microbiol. 3, 777–788. doi: 10.1038/nrmicro1273
Cotter, P. D., Ross, R. P., and Hill, C. (2013). Bacteriocins—a viable alternative to antibiotics? Nat. Rev. Microbiol. 11, 95–105. doi: 10.1038/nrmicro2937
da Silva Malheiros, P., Daroit, D. J., and Brandelli, A. (2010). Food applications of liposome-encapsulated antimicrobial peptides. Trends Food Sci. Technol. 21, 284–292. doi: 10.1016/j.colsurfb.2016.05.080
da Silva Sabo, S., Vitolo, M., González, J. M. D., and De Souza Oliveira, R. P. (2014). Overview of Lactobacillus plantarum as a promising bacteriocin producer among lactic acid bacteria. Food Res. Int. 64, 527–536. doi: 10.1016/j.foodres.2014.07.041
Dal Bello, B., Cocolin, L., Zeppa, G., Field, D., Cotter, P. D., and Hill, C. (2012). Technological characterization of bacteriocin producing Lactococcus lactis strains employed to control Listeria monocytogenes in cottage cheese. Int. J. Food Microbiol. 153, 58–65. doi: 10.1016/j.ijfoodmicro.2011.10.016
Davies, E., Bevis, H., and Delves-Broughton, J. (1997). The use of the bacteriocin, nisin, as a preservative in ricotta-type cheeses to control the food-borne pathogen Listeria monocytogenes. Lett. Appl. Microbiol. 24, 343–346. doi: 10.1046/j.1472-765X.1997.00145.x
de Arauz, L. J., Jozala, A. F., Mazzola, P. G., and Penna, T. C. V. (2009). Nisin biotechnological production and application: a review. Trends Food Sci. Technol. 20, 146–154. doi: 10.1016/j.tifs.2009.01.056
de Souza Barbosa, M., Todorov, S. D., Ivanova, I., Chobert, J. -M., Haertlé, T., and De Melo Franco, B. D. G. (2015). Improving safety of salami by application of bacteriocins produced by an autochthonous Lactobacillus curvatus isolate. Food Microbiol. 46, 254–262. doi: 10.1016/j.fm.2014.08.004
De Vuyst, L., and Leroy, F. (2007). Bacteriocins from lactic acid bacteria: production, purification, and food applications. J. Mol. Microbiol. Biotechnol. 13, 194–199. doi: 10.1159/000104752
De Vuyst, L., Moreno, M. F., and Revets, H. (2003). Screening for enterocins and detection of hemolysin and vancomycin resistance in enterococci of different origins. Int. J. Food Microbiol. 84, 299–318. doi: 10.1016/S0168-1605(02)00425-7
Domingos-Lopes, M., Stanton, C., Ross, P., Dapkevicius, M., and Silva, C. (2017). Genetic diversity, safety and technological characterization of lactic acid bacteria isolated from artisanal Pico cheese. Food Microbiol. 63, 178–190. doi: 10.1016/j.fm.2016.11.014
Eaton, T. J., and Gasson, M. J. (2001). Molecular screening of Enterococcus virulence determinants and potential for genetic exchange between food and medical isolates. Appl. Environ. Microbiol. 67, 1628–1635. doi: 10.1128/AEM.67.4.1628-1635.2001
EFSA (2007). Scientific committee. introduction of a qualified presumption of safety (QPS) approach for assessment of selected microorganisms referred to EFSA1. Opinion of the Scientific Committee (Question No EFSA-Q-2005-293. EFSA J. 587, 1–16.
Egan, K., Field, D., Rea, M. C., Ross, R. P., Hill, C., and Cotter, P. D. (2016). Bacteriocins: novel solutions to age old spore-related problems? Front. Microbiol. 7:461. doi: 10.3389/fmicb.2016.00461
Eijsink, V. G., Skeie, M., Middelhoven, P. H., Brurberg, M. B., and Nes, I. F. (1998). Comparative studies of class IIa bacteriocins of lactic acid bacteria. Appl. Environ. Microbiol. 64, 3275–3281.
Ercolini, D., Ferrocino, I., La Storia, A., Mauriello, G., Gigli, S., Masi, P., et al. (2010). Development of spoilage microbiota in beef stored in nisin activated packaging. Food Microbiol. 27, 137–143. doi: 10.1016/j.fm.2009.09.006
Fagundes, P. C., De Farias, F. M., Da Silva Santos, O. C., Da Paz, J. A. S., Ceotto-Vigoder, H., Alviano, D. S., et al. (2016). The four-component aureocin A70 as a promising agent for food biopreservation. Int. J. Food Microbiol. 237, 39–46. doi: 10.1016/j.ijfoodmicro.2016.08.017
FAO and WHO (2006). Probiotics in Food: Health and Nutritional Properties and Guidelines for Evaluation. Rome: FAO.
Favaro, L., Penna, A. L. B., and Todorov, S. D. (2015). Bacteriocinogenic LAB from cheeses–application in biopreservation? Trends Food Sci. Technol. 41, 37–48. doi: 10.1016/j.tifs.2014.09.001
Felicio, B. A., Pinto, M. S., Oliveira, F. S., Lempk, M. W., Pires, A. C. S., and Lelis, C. A. (2015). Effects of nisin on Staphylococcus aureus count and physicochemical properties of Minas Frescal cheese. J. Dairy Sci. 98, 4364–4369. doi: 10.3168/jds.2015-9520
Ferreira, M., and Lund, B. (1996). The effect of nisin on Listeria monocytogenes in culture medium and long-life cottage cheese. Lett. Appl. Microbiol. 22, 433–438. doi: 10.1111/j.1472-765X.1996.tb01197.x
Galvez, A., Lopez, R. L., Abriouel, H., Valdivia, E., and Omar, N. B. (2008). Application of bacteriocins in the control of foodborne pathogenic and spoilage bacteria. Crit. Rev. Biotechnol. 28, 125–152. doi: 10.1080/07388550802107202
Garde, S., Avila, M., Arias, R., Gaya, P., and Nunez, M. (2011). Outgrowth inhibition of Clostridium beijerinckii spores by a bacteriocin-producing lactic culture in ovine milk cheese. Int. J. Food Microbiol. 150, 59–65. doi: 10.1016/j.ijfoodmicro.2011.07.018
Garriga, M., Aymerich, M., Costa, S., Monfort, J., and Hugas, M. (2002). Bactericidal synergism through bacteriocins and high pressure in a meat model system during storage. Food Microbiol. 19, 509–518. doi: 10.1006/fmic.2002.0498
Garsa, A. K., Kumariya, R., Kumar, A., Lather, P., Kapila, S., and Sood, S. (2014). Industrial cheese whey utilization for enhanced production of purified pediocin PA-1. LWT Food Sci. Technol. 59, 656–665. doi: 10.1016/j.lwt.2014.07.008
Giraffa, G. (1995). Enterococcal bacteriocins: their potential as anti-Listeria factors in dairy technology. Food Microbiol. 12, 291–299. doi: 10.1016/S0740-0020(95)80109-X
Giraffa, G., and Carminati, D. (1997). Control of Listeria monocytogenes in the rind of Taleggio, a surface-smear cheese, by a bacteriocin from Enterococcus faecium 7C5. Sci. Aliments 17, 383–391.
Gómez-Torres, N., Avila, M., Gaya, P., and Garde, S. (2014). Prevention of late blowing defect by reuterin produced in cheese by a Lactobacillus reuteri adjunct. Food Microbiol. 42, 82–88. doi: 10.1016/j.fm.2014.02.018
Gómez-Torres, N., Garde, S., Peiroten, A., and Avila, M. (2015). Impact of Clostridium spp. on cheese characteristics: microbiology, color, formation of volatile compounds and off-flavors. Food Control 56, 186–194. doi: 10.1016/j.foodcont.2015.03.025
González, L., and Zárate, V. (2015). Inhibitory activity of Lactobacillus plantarum TF711 against Clostridium sporogenes when used as adjunct culture in cheese manufacture. J. Dairy Res. 82, 236–241. doi: 10.1017/S0022029915000126
Han, J. H. (2003). “Antimicrobial food packaging,” in Novel Food Packaging Techniques, ed. R. Ahvenainen (Cambridge: Woodhead Publishing), 50–70.
Ibarguren, C., Céliz, G., Díaz, A. S., Bertuzzi, M. A., Daz, M., and Audisio, M. C. (2015). Gelatine based films added with bacteriocins and a flavonoid ester active against food-borne pathogens. Innov. Food Sci. Emerg. Technol. 28, 66–72. doi: 10.1016/j.ifset.2015.01.007
Jamuna, M., and Jeevaratnam, K. (2004). Isolation and partial characterization of bacteriocins from Pediococcus species. Appl. Microbiol. Biotechnol. 65, 433–439. doi: 10.1007/s00253-004-1576-8
Jaouani, I., Abbassi, M., Ribeiro, S., Khemiri, M., Mansouri, R., Messadi, L., et al. (2015). Safety and technological properties of bacteriocinogenic enterococci isolates from Tunisia. J. Appl. Microbiol. 119, 1089–1100. doi: 10.1111/jam.12916
Kalchayanand, N., Hanlin, M., and Ray, B. (1992). Sublethal injury makes Gram-negative and resistant Gram-positive bacteria sensitive to the bacteriocins, pediocin AcH and nisin. Lett. Appl. Microbiol. 15, 239–243. doi: 10.1111/j.1472-765X.1992.tb00773.x
Khan, H., Flint, S., and Yu, P. -L. (2010). Enterocins in food preservation. Int. J. Food Microbiol. 141, 1–10. doi: 10.1016/j.ijfoodmicro.2010.03.005
Khan, I., and Oh, D. -H. (2016). Integration of nisin into nanoparticles for application in foods. Innov. Food Sci. Emerg. Technol. 34, 376–384. doi: 10.1016/j.ifset.2015.12.013
Khay, E., Idaomar, M., El Moussaoui, N., and Abrini, J. (2014). Application of a bacteriocin-like inhibitory substance producing Enterococcus durans E204 strain, isolated from camel milk, to control Listeria monocytogenes CECT 4032 in goat jben. Ann. Microbiol. 64, 313–319. doi: 10.1007/s13213-013-0666-1
Klaenhammer, T. R. (1993). Genetics of bacteriocins produced by lactic acid bacteria. FEMS Microbiol. Rev. 12, 39–85. doi: 10.1111/j.1574-6976.1993.tb00012.x
Kondrotiene, K., Kasnauskyte, N., Serniene, L., Gölz, G., Alter, T., Kaskoniene, V., et al. (2018). Characterization and application of newly isolated nisin producing Lactococcus lactis strains for control of Listeria monocytogenes growth in fresh cheese. LWT Food Sci. Technol. 87, 507–514. doi: 10.1016/j.lwt.2017.09.021
Kykkidou, S., Pournis, N., Kostoula, O. K., and Savvaidis, I. N. (2007). Effects of treatment with nisin on the microbial flora and sensory properties of a Greek soft acid-curd cheese stored aerobically at 4 C. Int. Dairy J. 17, 1254–1258. doi: 10.1016/j.idairyj.2007.02.006
Lauková, A., and Czikková, S. (1999). The use of enterocin CCM 4231 in soy milk to control the growth of Listeria monocytogenes and Staphylococcus aureus. J. Appl. Microbiol. 87, 182–182. doi: 10.1046/j.1365-2672.1999.00810.x
Lauková, A., Czikková, S., and Burdová, O. (1999a). Anti-staphylococcal effect of enterocin in Sunar and yogurt. Folia Microbiol. 44, 707–711.
Lauková, A., Czikková, S., Dobránsky, T., and Burdová, O. (1999b). Inhibition of Listeria monocytogenes and Staphylococcus aureus by enterocin CCM 4231 in milk products. Food Microbiol. 16, 93–99.
Lauková, A., Vlaemynck, G., and Czikkova, S. (2001). Effect of enterocin CCM 4231 on Listeria monocytogenes in Saint-Paulin cheese. Folia Microbiol. 46, 157–160. doi: 10.1007/BF02873596
Leroy, F., and De Vuyst, L. (2004). Lactic acid bacteria as functional starter cultures for the food fermentation industry. Trends Food Sci. Technol. 15, 67–78. doi: 10.1016/j.tifs.2003.09.004
Leroy, F., and De Vuyst, L. (2010). Bacteriocins of lactic acid bacteria to combat undesirable bacteria in dairy products. Aust. J. Dairy Technol. 65, 143–149.
Machaidze, G., and Seelig, J. (2003). Specific binding of cinnamycin (Ro 09-0198) to phosphatidylethanolamine. Comparison between micellar and membrane environments. Biochemistry 42, 12570–12576. doi: 10.1021/bi035225b
Maisnier-Patin, S., Deschamps, N., Tatini, S., and Richard, J. (1992). Inhibition of Listeria monocytogenes in Camembert cheese made with a nisin-producing starter. Le Lait 72, 249–263. doi: 10.1051/lait:1992318
Malheiros, P. S., Cuccovia, I. M., and Franco, B. (2016). Inhibition of Listeria monocytogenes in vitro and in goat milk by liposomal nanovesicles containing bacteriocins produced by Lactobacillus sakei subsp sakei 2a. Food Control 63, 158–164. doi: 10.1016/j.foodcont.2015.11.037
Marques, J. D., Funck, G. D., Dannenberg, G. D., Cruxen, C. E. D., El Halal, S. L. M., Dias, A. R. G., et al. (2017). Bacteriocin-like substances of Lactobacillus curvatus P99: characterization and application in biodegradable films for control of Listeria monocytogenes in cheese. Food Microbiol. 63, 159–163. doi: 10.1016/j.fm.2016.11.008
Martinez, R. C. R., Staliano, C. D., Vieira, A. D. S., Villarreal, M. L. M., Todorov, S. D., Saad, S. M. I., et al. (2015). Bacteriocin production and inhibition of Listeria monocytogenes by Lactobacillus sakei subsp sakei 2a in a potentially synbiotic cheese spread. Food Microbiol. 48, 143–152. doi: 10.1016/j.fm.2014.12.010
Martínez-Cuesta, M. C., Bengoechea, J., Bustos, I., Rodríguez, B., Requena, T., and Peláez, C. (2010). Control of late blowing in cheese by adding lacticin 3147-producing Lactococcus lactis IFPL 3593 to the starter. Int. Dairy J. 20, 18–24. doi: 10.1016/j.idairyj.2009.07.005
Martinez-Cuesta, M. C., Requena, T., and Pelaez, C. (2001). Use of a bacteriocin-producing transconjugant as starter in acceleration of cheese ripening. Int. J. Food Microbiol. 70, 79–88. doi: 10.1016/S0168-1605(01)00516-5
Martins, J. T., Cerqueira, M. A., Souza, B. W., Carmo Avides, M. D., and Vicente, A. A. (2010). Shelf life extension of ricotta cheese using coatings of galactomannans from nonconventional sources incorporating nisin against Listeria monocytogenes. J. Agric. Food Chem. 58, 1884–1891. doi: 10.1021/jf902774z
Mathot, A. G., Beliard, E., and Thuault, D. (2003). Streptococcus thermophilus 580 produces a bacteriocin potentially suitable for inhibition of Clostridium tyrobutyricum in hard cheese. J. Dairy Sci. 86, 3068–3074. doi: 10.3168/jds.S0022-0302(03)73906-X
Matijasic, B. B., Rajsp, M. K., Perko, B., and Rogelj, I. (2007). Inhibition of Clostridium tyrobutyricum in cheese by Lactobacillus gasseri. Int. Dairy J. 17, 157–166. doi: 10.1016/j.idairyj.2006.01.011
McAuliffe, O., Hill, C., and Ross, R. (1999). Inhibition of Listeria monocytogenes in cottage cheese manufactured with a lacticin 3147-producing starter culture. J. Appl. Microbiol. 86, 251–256. doi: 10.1046/j.1365-2672.1999.00663.x
McAuliffe, O., Ryan, M. P., Ross, R. P., Hill, C., Breeuwer, P., and Abee, T. (1998). Lacticin 3147, a broad-spectrum bacteriocin which selectively dissipates the membrane potential. Appl. Environ. Microbiol. 64, 439–445.
Melo, J., Andrew, P., and Faleiro, M. (2015). Listeria monocytogenes in cheese and the dairy environment remains a food safety challenge: the role of stress responses. Food Res. Int. 67, 75–90. doi: 10.1016/j.foodres.2014.10.031
Metlitskaya, A., Kazakov, T., Kommer, A., Pavlova, O., Praetorius-Ibba, M., Ibba, M., et al. (2006). Aspartyl-tRNA synthetase is the target of peptide nucleotide antibiotic Microcin C. J. Biol. Chem. 281, 18033–18042. doi: 10.1074/jbc.M513174200
Mills, S., Serrano, L., Griffin, C., O’connor, P. M., Schaad, G., Bruining, C., et al. (2011). Inhibitory activity of Lactobacillus plantarum LMG P-26358 against Listeria innocua when used as an adjunct starter in the manufacture of cheese. Microbial Cell Factories 10, S7. doi: 10.1186/1475-2859-10-S1-S7
Mitra, S., Mukhopadhyay, B. C., and Biswas, S. R. (2011). Potential application of the nisin Z preparation of Lactococcus lactis W8 in preservation of milk. Lett. Appl. Microbiol. 53, 98–105. doi: 10.1111/j.1472-765X.2011.03075.x
Moreno, M. F., Sarantinopoulos, P., Tsakalidou, E., and De Vuyst, L. (2006). The role and application of enterococci in food and health. Int. J. Food Microbiol. 106, 1–24. doi: 10.1016/j.ijfoodmicro.2005.06.026
Morgan, S., Galvin, M., Ross, R., and Hill, C. (2001). Evaluation of a spray-dried lacticin 3147 powder for the control of Listeria monocytogenes and Bacillus cereus in a range of food systems. Lett. Appl. Microbiol. 33, 387–391. doi: 10.1046/j.1472-765X.2001.01016.x
Morgan, S., O’sullivan, L., Ross, R., and Hill, C. (2002). The design of a three strain starter system for Cheddar cheese manufacture exploiting bacteriocin-induced starter lysis. Int. Dairy J. 12, 985–993. doi: 10.1016/S0958-6946(02)00123-1
Morgan, S., Ross, R., and Hill, C. (1997). Increasing starter cell lysis in Cheddar cheese using a bacteriocin-producing adjunct. J. Dairy Sci. 80, 1–10. doi: 10.3168/jds.S0022-0302(97)75906-X
Muñoz, A., Ananou, S., Gálvez, A., Martínez-Bueno, M., Rodríguez, A., Maqueda, M., et al. (2007). Inhibition of Staphylococcus aureus in dairy products by enterocin AS-48 produced in situ and ex situ: bactericidal synergism with heat. Int. Dairy J. 17, 760–769. doi: 10.1016/j.idairyj.2006.09.006
Muñoz, A., Maqueda, M., Galvez, A., Martinez-Bueno, M., Rodriguez, A., and Valdivia, E. (2004). Biocontrol of psychrotrophic enterotoxigenic Bacillus cereus in a nonfat hard cheese by an enterococcal strain-producing enterocin AS-48. J. Food Prot. 67, 1517–1521. doi: 10.4315/0362-028X-67.7.1517
Narayanan, A., and Ramana, K. V. (2013). Synergized antimicrobial activity of eugenol incorporated polyhydroxybutyrate films against food spoilage microorganisms in conjunction with pediocin. Appl. Biochem. Biotechnol. 170, 1379–1388. doi: 10.1007/s12010-013-0267-2
Narsaiah, K., Wilson, R. A., Gokul, K., Mandge, H., Jha, S., Bhadwal, S., et al. (2015). Effect of bacteriocin-incorporated alginate coating on shelf-life of minimally processed papaya (Carica papaya L.). Postharvest Biol. Technol. 100, 212–218. doi: 10.1016/j.postharvbio.2014.10.003
Nissen, H., Holo, H., Axelsson, L., and Blom, H. (2001). Characterization and growth of Bacillus spp. in heat-treated cream with and without nisin. J. Appl. Microbiol. 90, 530–534. doi: 10.1046/j.1365-2672.2001.01279.x
Nunez, M., Rodriguez, J., Garcia, E., Gaya, P., and Medina, M. (1997). Inhibition of Listeria monocytogenes by enterocin 4 during the manufacture andripening of Manchego cheese. J. Appl. Microbiol. 83, 671–677. doi: 10.1046/j.1365-2672.1997.00275.x
O’Connor, P. M., Ross, R. P., Hill, C., and Cotter, P. D. (2015). Antimicrobial antagonists against food pathogens: a bacteriocin perspective. Curr. Opin. Food Sci. 2, 51–57. doi: 10.1016/j.cofs.2015.01.004
O’Mahony, T., Rekhif, N., Cavadini, C., and Fitzgerald, G. F. (2001). The application of a fermented food ingredient containing ‘variacin’, a novel antimicrobial produced by Kocuria varians, to control the growth of Bacillus cereus in chilled dairy products. J. Appl. Microbiol. 90, 106–114. doi: 10.1046/j.1365-2672.2001.01222.x
Oshima, S., Hirano, A., Kamikado, H., Nishimura, J., Kawai, Y., and Saito, T. (2014). Nisin A extends the shelf life of high-fat chilled dairy dessert, a milk-based pudding. J. Appl. Microbiol. 116, 1218–1228. doi: 10.1111/jam.12454
O’Sullivan, L., Ryan, M. P., Ross, R. P., and Hill, C. (2003). Generation of food-grade lactococcal starters which produce the lantibiotics lacticin 3147 and lacticin 481. Appl. Environ. Microbiol. 69, 3681–3685. doi: 10.1128/AEM.69.6.3681-3685.2003
Oumer, A., Garde, S., Gaya, P., Medina, M., and Nunez, M. (2001). The effects of cultivating lactic starter cultures with bacteriocin-producing lactic acid bacteria. J. Food Prot. 64, 81–86. doi: 10.4315/0362-028X-64.1.81
Parks, W. M., Bottrill, A. R., Pierrat, O. A., Durrant, M. C., and Maxwell, A. (2007). The action of the bacterial toxin, microcin B17, on DNA gyrase. Biochimie 89, 500–507. doi: 10.1016/j.biochi.2006.12.005
Patrovský, M., Kouřimská, L., Havlíková,Š., Marková, J., Pechar, R., and Rada, V. (2016). Utilization of bacteriocin-producing bacteria in dairy products. Mljekarstvo 66, 215–224.
Perez, R. H., Zendo, T., and Sonomoto, K. (2014). Novel bacteriocins from lactic acid bacteria (LAB): various structures and applications. Microb. Cell Fact. 13:S3. doi: 10.1186/1475-2859-13-S1-S3
Piard, J. -C., Muriana, P., Desmazeaud, M., and Klaenhammer, T. (1992). Purification and partial characterization of lacticin 481, a lanthionine-containing bacteriocin produced by Lactococcus lactis subsp. lactis CNRZ 481. Appl. Environ. Microbiol. 58, 279–284.
Pimentel-Filho, N. D. J., Mantovani, H. C., Carvalho, A. F., Dias, R. S., and Vanetti, M. C. D. (2014). Efficacy of bovicin HC5 and nisin combination against Listeria monocytogenes and Staphylococcus aureus in fresh cheese. Int. J. Food Sci. Technol. 49, 416–422. doi: 10.1111/ijfs.12316
Pingitore, E. V., Todorov, S. D., Sesma, F., and De Melo Franco, B. D. G. (2012). Application of bacteriocinogenic Enterococcus mundtii CRL35 and Enterococcus faecium ST88Ch in the control of Listeria monocytogenes in fresh Minas cheese. Food Microbiol. 32, 38–47. doi: 10.1016/j.fm.2012.04.005
Prudêncio, C. V., Dos Santos, M. T., and Vanetti, M. C. D. (2015). Strategies for the use of bacteriocins in Gram-negative bacteria: relevance in food microbiology. J. Food Sci. Technol. 52, 5408–5417. doi: 10.1007/s13197-014-1666-2
Pucci, M. J., Vedamuthu, E. R., Kunka, B. S., and Vandenbergh, P. A. (1988). Inhibition of Listeria monocytogenes by using bacteriocin PA-1 produced by Pediococcus acidilactici PAC 1.0. Appl. Environ. Microbiol. 54, 2349–2353.
Ramos,Ó. L., Pereira, J., Silva, S. I., Fernandes, J. C., Franco, M., Lopes-Da-Silva, J., et al. (2012). Evaluation of antimicrobial edible coatings from a whey protein isolate base to improve the shelf life of cheese. J. Dairy Sci. 95, 6282–6292. doi: 10.3168/jds.2012-5478
Ramsaran, H., Chen, J., Brunke, B., Hill, A., and Griffiths, M. (1998). Survivial of bioluminescent Listeria monocytogenes and Escherichia coli 0157: H7 in soft cheeses. J. Dairy Sci. 81, 1810–1817. doi: 10.3168/jds.S0022-0302(98)75750-9
Ramu, R., Shirahatti, P. S., Devi, A. T., and Prasad, A. (2015). Bacteriocins and their applications in food preservation. Crit. Rev. Food Sci. Nutr. doi: 10.1080/10408398.2015.1020918 [Epub ahead of print].
Renye, J., Somkuti, G., Garabal, J., and Du, L. (2011). Heterologous production of pediocin for the control of Listeria monocytogenes in dairy foods. Food Control 22, 1887–1892. doi: 10.1016/j.foodcont.2011.04.031
Resa, C. P. O., Gerschenson, L. N., and Jagus, R. J. (2014). Natamycin and nisin supported on starch edible films for controlling mixed culture growth on model systems and Port Salut cheese. Food Control 44, 146–151. doi: 10.1016/j.foodcont.2014.03.054
Ribeiro, S. C., O’Connor, P. M., Ross, R. P., Stanton, C., and Silva, C. C. (2016). An anti-listerial Lactococcus lactis strain isolated from Azorean Pico cheese produces lacticin 481. Int. Dairy J. 63, 18–28. doi: 10.1016/j.idairyj.2016.07.017
Ribeiro, S. C., Ross, R. P., Stanton, C., and Silva, C. C. (2017). Characterization and application of antilisterial enterocins on model fresh cheese. J. Food Prot. 80, 1303–1316. doi: 10.4315/0362-028X.JFP-17-031
Rilla, N., Martinez, B., Delgado, T., and Rodriguez, A. (2003). Inhibition of Clostridium tyrobutyricum in Vidiago cheese by Lactococcus lactis ssp lactis IPLA 729, a nisin Z producer. Int. J. Food Microbiol. 85, 23–33. doi: 10.1016/S0168-1605(02)00478-6
Rodrígez, E., Arqués, J. L., Gaya, P., Nunez, M., and Medina, M. (2001). Control of Listeria monocytogenes by bacteriocins and monitoring of bacteriocin-producing lactic acid bacteria by colony hybridization in semi-hard raw milk cheese. J. Dairy Res. 68, 131–137. doi: 10.1017/S0022029900004660
Rodriguez, E., Arques, J. L., Nunez, M., Gaya, P., and Medina, M. (2005). Combined effect of high-pressure treatments and bacteriocin-producing lactic acid bacteria on inactivation of Escherichia coli O157: H7 in raw-milk cheese. Appl. Environ. Microbiol. 71, 3399–3404. doi: 10.1128/AEM.71.7.3399-3404.2005
Ross, R. P., Galvin, M., Mcauliffe, O., Morgan, S. M., Ryan, M. P., Twomey, D. P., et al. (1999). Developing applications for lactococcal bacteriocins. Antonie Van Leeuwenhoek 76, 337–346.
Ross, R. P., Morgan, S., and Hill, C. (2002). Preservation and fermentation: past, present and future. Int. J. Food Microbiol. 79, 3–16. doi: 10.1016/S0168-1605(02)00174-5
Ross, R. P., Stanton, C., Hill, C., Fitzgerald, G. F., and Coffey, A. (2000). Novel cultures for cheese improvement. Trends Food Sci. Technol. 11, 96–104. doi: 10.1016/S0924-2244(00)00057-1
Ryan, M. P., Rea, M. C., Hill, C., and Ross, R. P. (1996). An application in cheddar cheese manufacture for a strain of Lactococcus lactis producing a novel broad-spectrum bacteriocin, lacticin 3147. Appl. Environ. Microbiol. 62, 612–619.
Şahingil, D., İsleogl, H., Yildirim, Z., Akcelik, M., and Yildirim, M. (2011). Characterization of lactococcin BZ produced by Lactococcus lactis subsp. lactis BZ isolated from boza. Turk. J. Biol. 35, 21–33.
Salgado, P. R., Ortiz, C. M., Musso, Y. S., Di Giorgio, L., and Mauri, A. N. (2015). Edible films and coatings containing bioactives. Curr. Opin. Food Sci. 5, 86–92. doi: 10.1016/j.cofs.2015.09.004
Sánchez-González, L., Saavedra, J. I. Q., and Chiralt, A. (2013). Physical properties and antilisterial activity of bioactive edible films containing Lactobacillus plantarum. Food Hydrocoll. 33, 92–98. doi: 10.1016/j.foodhyd.2013.02.011
Sánchez-Hidalgo, M., Montalbán-López, M., Cebrián, R., Valdivia, E., Martínez-Bueno, M., and Maqueda, M. (2011). AS-48 bacteriocin: close to perfection. Cell. Mol. Life Sci. 68, 2845–2857. doi: 10.1007/s00018-011-0724-4
Scannell, A. G., Hill, C., Ross, R., Marx, S., Hartmeier, W., and Arendt, E. (2000a). Continuous production of lacticin 3147 and nisin using cells immobilized in calcium alginate. J. Appl. Microbiol. 89, 573–579.
Scannell, A. G., Hill, C., Ross, R., Marx, S., Hartmeier, W., and Arendt, E. K. (2000b). Development of bioactive food packaging materials using immobilised bacteriocins Lacticin 3147 and Nisaplin. Int. J. Food Microbiol. 60, 241–249.
Shi, F., Wang, Y. W., Li, Y. F., and Wang, X. Y. (2016). Mode of action of leucocin K7 produced by Leuconostoc mesenteroides K7 against Listeria monocytogenes and its potential in milk preservation. Biotechnol. Lett. 38, 1551–1557. doi: 10.1007/s10529-016-2127-y
Simha, B. V., Sood, S., Kumariya, R., and Garsa, A. K. (2012). Simple and rapid purification of pediocin PA-1 from Pediococcus pentosaceous NCDC 273 suitable for industrial application. Microbiol. Res. 167, 544–549. doi: 10.1016/j.micres.2012.01.001
Sobrino-López, A., and Martín-Belloso, O. (2008). Use of nisin and other bacteriocins for preservation of dairy products. Int. Dairy J. 18, 329–343. doi: 10.1016/j.idairyj.2007.11.009
Suda, S., Cotter, P. D, Hill, C., and Paul Ross, R. (2012). Lacticin 3147-biosynthesis, molecular analysis, immunity, bioengineering and applications. Curr. Protein Pept. Sci. 13, 193–204. doi: 10.2174/138920312800785021
Valdés, A., Ramos, M., Beltrán, A., Jiménez, A., and Garrigós, M. C. (2017). State of the art of antimicrobial edible coatings for food packaging applications. Coatings 7:56. doi: 10.3390/coatings7040056
van Heel, A. J., De Jong, A., Montalban-Lopez, M., Kok, J., and Kuipers, O. P. (2013). BAGEL3: automated identification of genes encoding bacteriocins and (non-) bactericidal posttranslationally modified peptides. Nucleic Acids Res. 41, W448–W453. doi: 10.1093/nar/gkt391
Vandera, E., Lianou, A., Kakouri, A., Feng, J., Koukkou, A. -I., and Samelis, J. (2017). Enhanced control of Listeria monocytogenes by Enterococcus faecium KE82, a multiple enterocin–producing strain, in different milk environments. J. Food Prot. 80, 74–85. doi: 10.4315/0362-028X.JFP-16-082
Verma, S. K., Sood, S. K., Saini, R. K., and Saini, N. (2017). Pediocin PA-1 containing fermented cheese whey reduces total viable count of raw buffalo (Bubalis bubalus) milk. LWT Food Sci. Technol. 83, 193–200. doi: 10.1016/j.lwt.2017.02.031
Vincent, P. A., and Morero, R. D. (2009). The structure and biological aspects of peptide antibiotic microcin J25. Curr. Med. Chem. 16, 538–549. doi: 10.2174/092986709787458461
Yamauchi, Y., Ishii, S., Toyoda, S., and Ahiko, K. (1996). Process for the manufacture of fermented milk. U. S. Patent 5,527,505. Washington, DC: U.S. Patent and Trademark Office.
Yildirim, Z., Öncül, N., Yildirim, M., and Karabiyikli, Ş. (2016). Application of lactococcin BZ and enterocin KP against Listeria monocytogenes in milk as biopreservation agents. Acta Aliment. 45, 486–492. doi: 10.1556/066.2016.45.4.4
Zacharof, M., and Lovitt, R. (2012). Bacteriocins produced by lactic acid bacteria a review article. APCBEE Procedia 2, 50–56. doi: 10.1016/j.apcbee.2012.06.010
Zapico, P., Medina, M., Gaya, P., and Nuñez, M. (1998). Synergistic effect of nisin and the lactoperoxidase system on Listeria monocytogenes in skim milk. Int. J. Food Microbiol. 40, 35–42. doi: 10.1016/S0168-1605(98)00008-7
Zhao, L., Wang, S., Liu, F., Dong, P., Huang, W., Xiong, L., et al. (2013). Comparing the effects of high hydrostatic pressure and thermal pasteurization combined with nisin on the quality of cucumber juice drinks. Innov. Food Science & Emerg. Technol. 17, 27–36. doi: 10.1016/j.ifset.2012.10.004
Keywords: bacteriocins, lactic acid bacteria, dairy products, biopreservation, edible coatings
Citation: Silva CCG, Silva SPM and Ribeiro SC (2018) Application of Bacteriocins and Protective Cultures in Dairy Food Preservation. Front. Microbiol. 9:594. doi: 10.3389/fmicb.2018.00594
Received: 28 December 2017; Accepted: 15 March 2018;
Published: 09 April 2018.
Edited by:
Riadh Hammami, University of Ottawa, CanadaReviewed by:
M. Y. Sreenivasa, University of Mysore, IndiaGiuseppe Spano, University of Foggia, Italy
Ehab Eissa Kheadr, Alexandria University, Egypt
Copyright © 2018 Silva, Silva and Ribeiro. This is an open-access article distributed under the terms of the Creative Commons Attribution License (CC BY). The use, distribution or reproduction in other forums is permitted, provided the original author(s) and the copyright owner are credited and that the original publication in this journal is cited, in accordance with accepted academic practice. No use, distribution or reproduction is permitted which does not comply with these terms.
*Correspondence: Célia C. G. Silva Y2VsaWEuY2cuc2lsdmFAdWFjLnB0; Y2VsaWFAdWFjLnB0