- 1Department of Basic Sciences, College of Veterinary Medicine, Mississippi State University, Mississippi State, MS, United States
- 2Department of Nutrition and Veterinary Clinical Nutrition, Faculty of Veterinary Medicine, Damanhour University, Damanhour, Egypt
Edwardsiella ictaluri (E. ictaluri), a Gram-negative, intracellular, facultative bacterium, is the causative agent of enteric septicemia of catfish (ESC), which is one of the most significant diseases of farmed channel catfish. Macrophages have a critical role in major defense mechanisms against bacterial infections by migrating to the site of infection, engulfing and killing pathogens, and priming adaptive immune responses. Vaccination of catfish with E. ictaluri live attenuated vaccine (LAV) strains increased the efficiency of phagocytosis and bacterial killing in catfish peritoneal macrophages compared in vitro with macrophages from non-vaccinated fish. Recently, our group developed several protective LAV strains from E. ictaluri. However, their effects on the antigen uptake and bacterial killing in catfish macrophages have not been evaluated. In this study, we assessed the phagocytic and bactericidal activity of peritoneal macrophages in the uptake of E. ictaluri wild-type (WT) and two LAV strains. We found that phagocytosis of LAV strains was significantly higher compared to their WT counterpart in peritoneal macrophages. Moreover, the uptake of E. ictaluri opsonized with sera from vaccinated catfish was more efficient than when opsonized with sera from sham-vaccinated fish. Notably, catfish macrophages did not lose their phagocytic properties at 4°C, as described previously in mammalian and zebrafish models. Also, opsonization of E. ictaluri with inactivated sera from vaccinated and sham-vaccinated catfish decreased significantly phagocytic uptake of bacteria at 32°C, and virtually suppressed endocytosis at 4°C, suggesting the important role of complement-dependent mechanisms in catfish macrophage phagocytosis. In conclusion, our data on enhanced phagocytic capacity and effective killing ability in macrophages of vaccine strains suggested the LAVs’ advantage if processed and presented in the form of peptides to specific lymphocytes of an adaptive immune system and emphasize the importance of macrophage-mediated immunity against ESC. Furthermore, we showed the role of complement-dependent mechanisms in the phagocytic uptakes of E. ictaluri in catfish peritoneal macrophages at 4 and 32°C. Finally, LAV vaccine-induced bacterial phagocytosis and killing properties of peritoneal macrophages emphasized the importance of the innate immune responses in ESC.
Introduction
Edwardsiella ictaluri, a Gram-negative, intracellular, facultative bacterium, is the causative agent of ESC, which is one of the most significant diseases of farmed channel catfish (Hawke et al., 1981; Miyazaki and Plumb, 1985; Wagner et al., 2006; Zhang and Arias, 2007). Klesius and Shoemaker (1999) developed a modified live E. ictaluri vaccine against ESC (commercialized later as Aquavac-ESC) that stimulated protective immunity delivered by bath immertion in juvenile catfish. Subsequent immersion studies showed effective protection in catfish fry, fingerlings, and eyed catfish eggs (Shoemaker et al., 1999, 2002, 2007; Wise et al., 2000), which also demonstrated that E. ictaluri LAVs induced cell-mediated immunity to protect catfish against ESC (Shoemaker and Klesius, 1997; Ellis, 1999) because E. ictaluri could survive and replicate in channel catfish macrophages (Booth et al., 2006). Furthermore, vaccination of catfish with LAVs resulted in the specific antibodies production that enhanced the bactericidal activity of macrophages (Shoemaker and Klesius, 1997).
Macrophages are professional phagocytes that have multiple functions in different species including immunity, inflammation, and tissue repair (Godwin et al., 2013). New evidence has accumulated on the progenitors of adult tissue resident macrophages, embryonic macrophages (Schulz et al., 2012; Hashimoto et al., 2013; Epelman et al., 2014; Hoeffel et al., 2015; Sheng et al., 2015). Professional phagocytes, including macrophages, in fish have a significant role in major defense mechanisms against bacterial infections as these cells can migrate to the site of infection and engulf and kill pathogens (Secombes and Fletcher, 1992; Esteban et al., 2015). Multiple studies documented strong phagocytic capability and bactericidal activity of anterior kidney macrophages against intracellular pathogens including parasites, yeast, and bacteria (Bennani et al., 1995; Dieter and Katharina, 1997; Esteban et al., 1998; Muñoz et al., 2000; Qiu et al., 2016). Importantly, macrophages are present normally in the peritoneal cavity of fish; therefore, the peritoneal macrophage approach has been documented well for in vitro immunologic studies in catfish (Jenkins and Klesius, 1998). Collection of peritoneal macrophages is relatively easy and does not require special isolation and purification procedures (Jenkins and Klesius, 1998). Moreover, phagocytes response to IP inflammation is easily observed and measured both qualitatively and quantitatively (Silva et al., 1989).
Peritoneal macrophages from sea bass had significantly greater phagocytic activity against bacteria, such as Escherichia coli and Salmonella enterica serovar Typhimurium, than did monocytes and macrophages from blood and anterior kidney, respectively (Esteban and Meseguer, 1997). Furthermore, a higher number of phagocytosed bacteria was observed in macrophages than other phagocytic cells in the peritoneal cavity of sea bass (Do Vale et al., 2002). Phagocytosis of Yersinia ruckeri in peritoneal macrophages was significantly greater compared to the phagocytic activity of neutrophils in rainbow trout (Oncorhynchus mykiss) (António et al., 1998). Winter flounder (Pleuronectes americanus) peritoneal macrophages were capable of engulfing formalin-fixed bacteria (Y. ruckeri and Bacillus cereus) after a short exposure (Bodammer and Robohm, 1996). The phagocytic and microbicidal activity of peritoneal macrophages were described in rohu (Labeo rohita) and walking catfish (Clarias batrachus) (Mamnur Rashid et al., 2002; Awasthi et al., 2015). Shoemaker et al. (1997) evaluated the role of peritoneal macrophages in immunity to ESC after infection with live E. ictaluri. Phagocytic and bactericidal activity was significantly greater in macrophages from fish immunized with AL-93-75 compared to their counterparts from pathogen-free susceptible fingerling catfish (Russo et al., 2009). Interestingly, opsonization of E. ictaluri with immune serum significantly enhanced the killing ability of macrophages from susceptible fish (Shoemaker et al., 1997; Russo et al., 2009).
One of the major manifestations of immunological autophagy is the destruction and elimination of invading pathogens (Deretic et al., 2013; Mizumura et al., 2014). Recently, autophagy has been described in fish (van der Vaart et al., 2012; Yabu et al., 2012; García-Valtanen et al., 2014). Engulfment of microbial prey as part of autophagy is initiated at the plasma membrane of the macrophage where a vast repertoire of phagocytic receptors, in particular, CRs, recognize the bacterial surface directly or indirectly through deposition of serum opsonins such as IgG or the complement protein C3b (Herre et al., 2004; Patel and Harrison, 2008). Complement activation is a tightly regulated process that may proceed through three distinct pathways: the alternative pathway, classical pathway, and the lectin-dependent pathway. However, each pathway converges on the complement protein C3b to generate the bioactive components C3a and C3b (Merle et al., 2015). Similar to higher vertebrates, the complement system in teleost fish can be activated through all three pathways and shows many effector functions identified in mammalian complement system, such as opsonization, anaphylatoxic leukocyte stimulation, and target cell killing (Holland and Lambris, 2002; Nakao et al., 2011). However, some complement components in teleost fish are present in multiple active isoforms, in particular the key complement component, C3, is present in several isoforms produced by different genes (Sunyer et al., 1996, 1997; Nakao et al., 2000; Boshra et al., 2006). In addition, catalytic residues of proteins of complement are different in teleost fish (Nakao and Yano, 1998). Furthermore, unlike in mammals, fish complement components are active at low temperatures and show higher magnitude providing broader recognition of foreign substances in fish (Sunyer and Tort, 1995; Sunyer and Lambris, 1998; Boshra et al., 2006). It is well documented that opsonization of bacteria with serum proteins and fixation of complement are formidable barriers that must be overcome to establish infection (Flannagan et al., 2015). Whereas the specific signaling molecules can vary, the requirement for actin remodeling in the engulfment process is absolute (Greenberg et al., 1991; Tse et al., 2003). The lumen of phagolysosome is an inhospitable environment for intracellular pathogens (Flannagan et al., 2015). Lysosomal proteases that display a range of functions that shape the cellular immune response in macrophages, antimicrobial effectors that promote killing of phagocytosed pathogens through direct proteolytic attacks (Muller et al., 2014), and other active substances comprise part of the macrophages antimicrobial arsenal that exerts microbicidal effects by compromising directly bacterial membranes or promoting the production of immunomodulatory compounds (Weinrauch et al., 1996; Wu et al., 2010).
Recently, our research group has determined that evpB gene in the Type VI secretion system (T6SS) operon is differentially regulated during in vitro iron-restricted conditions (Dumpala et al., 2015). We constructed EiΔevpB strain by in-frame deletion of evpB gene and found that E. ictaluri is completely attenuated in catfish fingerlings and fry. Vaccination with EiΔevpB did not cause mortality in fingerlings (100% survival) and low 3–4% mortality in fry catfish after WT E. ictaluri challenge (Nho et al., 2017). Our finding corroborated an earlier study showing that evpB plays a key role in E. tarda pathogenesis (Zheng and Leung, 2007). Furthermore, our laboratory reported that genes encoded tricarboxylic acid cycle (sdhCfrdA) and one-carbon metabolism (gcvP) were essential for E. ictaluri virulence (Dahal et al., 2013). Similarly, we introduced an in-frame deletion of glycine dehydrogenase (gcvP), succinate dehydrogenase (sdhC), and fumarate reductase (frdA) genes in E. ictaluri 93–146 strain and named it as ESC-NDKL1 (EiΔgcvPΔsdhCΔfrdA) (Nho et al., 2017). Vaccination of catfish fingerlings with ESC-NDKL1 showed similar 100% survival rates as EiΔevpB, however, challenge of fry with ESC-NDKL1 showed moderatly elevated 3–4% mortality rates (Nho et al., 2017). The purpose of our study was to compare the phagocytic and bacterial killing activity of channel catfish peritoneal macrophages against E. ictaluri WT and two LAV strains in the presence of sera obtained from vaccinated fish. Increased phagocytic capacity and killing ability of macrophages against opsonized LAV strains will support the importance of macrophage-mediated immunity against ESC in catfish.
Materials and Methods
Animals
The fish hatchery at the College of Veterinary Medicine, Mississippi State University, provided SPF channel catfish, which were maintained at 25–28°C. All fish experiments were performed based on a protocol approved by the Mississippi State University Institutional Animal Care and Use Committee (IACUC). Tricaine methanesulfonate (MS-222, Western, Chemical, Inc.) was used to sedate (100 mg/ml) and euthanize (400 mg/ml) the catfish. Samples were obtained as described below.
Cell Harvesting
Channel catfish (250–300 g) were used in this study. Peritoneal macrophages were harvested as described previously as a simple reliable method to obtain tissue resident macrophages that displayed high oxygen production and phagocytic ability (Jenkins and Klesius, 1998). Briefly, 1 ml squalene (Sigma–Aldrich, St. Louis, MO, United States) was injected IP in sedated catfish. After 4 days of injection, catfish were sedated, the peritoneal area wiped with 70% ethanol, and cold, sterile phosphate-buffered saline (PBS) injected IP via a three-way valve attached to a syringe, 18-gauge needle, and Tygon tubing. The valve was then closed, the fish’s abdominal region was massaged gently, and a cell suspension collected into a centrifuge tube placed on ice. Additional cold PBS was injected into the peritoneal cavity until IP fluid was clear.
Bacterial Strains and Opsonization
Bacterial strains used in this work are listed in Table 1. E. ictaluri 93–146 WT strain and vaccine strains were cultured in BHI agar or broth (Difco, Sparks, MD, United States) and incubated throughout the study at 30°C. Each vaccine strain was labeled with bioluminescence by transferring pAKgfplux1 from an E. coli donor strain (SM10aaapir) by conjugation as described previously (Karsi and Lawrence, 2007). When required, media were supplemented with following antibiotics and reagents; ampicillin (Amp: 100 mg/ml), and colistin sulfate (Col: 12.5 mg/ml, Sigma–Aldrich, St. Louis, MN, United States).
Overnight cultures of E. ictaluri and vaccine strains were grown and prepared as described above, followed by two PBS washes before opsonization with channel catfish serum for 30 min at room temperature. Opsonized bacteria were then used for antigen uptake and infection experiments.
Fish Vaccination and Serum Collection
A total of 100 SPF channel catfish fingerlings (6-month-old) with fully developed innate and adaptive immune systems (Patrie-Hanson and Ainsworth, 1999; Petrie-Hanson and Ainsworth, 2001; Rombout et al., 2005; Zapata et al., 2006) were stocked into four 40-L tanks (25 fish per tank) with a continuous water flow and aeration. Tanks were assigned randomly to EiΔevpB, ESC-NDKL1, E. ictaluri WT (positive control), and sham-vaccinated (negative control) groups. The fish were fed twice daily and acclimatized for 1 week. The water temperature was maintained at 24–26°C throughout the trial. After a week of acclimation, fish were exposed EiΔevpB, ESC-NDKL1, and WT E. ictaluri 93–146 by immersion challenge as described previously (Abdelhamed et al., 2013). Briefly, 100 ml of overnight cultures were added to 10 L water to yield infection dose of approximately 3.67 × 107 CFU/ml of water. The negative control group was immersion challenged with BHI broth.
Blood samples were collected from caudal vein of 10 fish at 14 and 21 days post-infection and were allowed to coagulate overnight at 4°C. Serum was obtained by centrifugation at 8000 rpm for 10 min.
Phagocytosis and Flow Cytometry
Harvested cells were washed three times in PBS at 2000 rpm for 10 min at 24°C and resuspended in CCMM, which included RPMI [(RPMI 1640 sans phenol red and L-glutamine, (0.407 mM magnesium sulfate and 0.424 mM calcium nitrate) Lonza, Walkersville, MD, United States) containing 1× glutamine substitute (GlutaMAX-I CTS, Gibco, Invitrogen Corporation, Carlsbad, CA, United States)], 15 mM HEPES buffer (GIBCO), in 0.18% sodium bicarbonate solution (GIBCO), 0.05 mM 2-beta-mercaptoethanol (all from Sigma Chemical Co., St. Louis, MO, United States), and 5% HI pooled channel catfish serum. Cells were counted using a hemocytometer and trypan blue exclusion. Cell suspension was transferred into a 6-well plate (Fisher Scientific, Pittsburgh, PA, United States), and the phagocytic capacities of peritoneal macrophages were determined by addition of GFP transformed bacterial strains in 1: 50 ratio and incubated at 32 (active uptake) and 4°C (background levels of endocytosis, negative control) for 2 h in the dark. Following incubation, cells were harvested with cell scrapers (Fisher Scientific, Pittsburgh, PA, United States), and washed three times by centrifugation in cold PBS and analyzed using FACSCalibur (Becton Dickinson), as follows. After setting a gate on large granular cells, the LAVs incorporation was measured and analyzed by using FlowJo 7.6.4 Software (Tree Star Inc.). To inhibit actin formation selectively, catfish macrophages were incubated for 10 min in the presence of CCD (2.5 μg/ml, Sigma–Aldrich, St. Louis, MO, United States) before the addition of LAVs bacterial strains (Watts and Marsh, 1992). To determine differences between treatments, MFI of engulfed bacteria in catfish peritoneal macrophages was analyzed using single histogram and overlay histogram statistics.
Cytospin and Light Microscopy
Peritoneal macrophages were incubated in the dark with GFP-labeled WT E. ictaluri and LAVs at 32 and 4°C for 2 h. Cells were then harvested, washed, and the cytospin preparations applied at 500 rpm for 1 min with a Cyto-Tek centrifuge machine. All samples were fixed and stained with Wright’s stain (Hemacolor, Merck) as described (Do Vale et al., 2002), analyzed, and then photographed with Olympus BX60 microscope (Olympus U-TV1 X) and Infinity software.
Bacterial Killing Assay
The bacterial killing assay was performed as described previously with some modifications (Booth et al., 2006; Russo et al., 2009). Briefly, harvested peritoneal macrophages were washed with PBS by centrifugation, resuspended in CCMM, and transferred to 96-well plates. WT strain and LAVs were added to catfish macrophages in 1:1 ratio followed by centrifugation at 1500 rpm for 5 min at 24°C to compact cells and bacteria and then incubated at 32°C for 1 h. After incubation, plates were centrifuged at 2000 rpm for 7–10 min, and supernatants removed. Next, cell pellets were resuspended in CCMM containing 100 μg/ml gentamicin (Gibco, Life Technologies, Grand Island, NY, United States) to kill extracellular bacteria, incubated at 32°C for 1 h, and washed by centrifugation in PBS. After washing, plates were incubated at 32°C for 10 and 24 h in CCMM containing 10 μg/ml gentamycin. For each time point, colony-counting method was performed, as follows. Macrophages were lysed with 1× Triton X-100 (Sigma, St. Louis, MO, United States), as described (Russo et al., 2009). Lysed macrophages were diluted in PBS and plated on a selective medium, and incubated at 32°C for 48 h.
Statistical Analysis
The significance of the differences between means was established by one-way ANOVA and two-way ANOVA procedures with Tukey’s test in SAS for Windows 9.4 (SAS Institute Inc., Cary, NC, United States) to evaluate differences in MFIs. The level of significance for all tests was set at P < 0.05.
Results
Active Phagocytic Uptake of E. ictaluri LAVs in Peritoneal Macrophages
In this study, we evaluated endocytic uptake of E. ictaluri and the LAV strains in catfish peritoneal macrophages (Figure 1). To ensure active phagocytosis in macrophages, we measured the uptake of the GFP-labeled bacteria at 32°C and background endocytosis levels at 4°C. Also, we assessed the intensity of phagocytosis in peritoneal macrophages pre-incubated with the phagocytosis inhibitor, CCD (Figures 1A,B). The phagocytic intensity levels of both LAV strains at 32°C were significantly higher compared to the endocytosis of WT E. ictaluri in catfish peritoneal macrophages (Figure 1B). However, the uptake of EiΔevpB showed a significant increase compared to its ESC-NDKL counterpart (Figure 1B). Significant decreases but not the complete inhibition of uptake of LAVs and WT E. ictaluri were evident in the presence of actin formation inhibitor CCD (Figure 1B) and at 4°C (data not shown). In conclusion, active uptake of LAV strains was significantly higher compared to their WT counterpart in peritoneal macrophages at both temperatures.
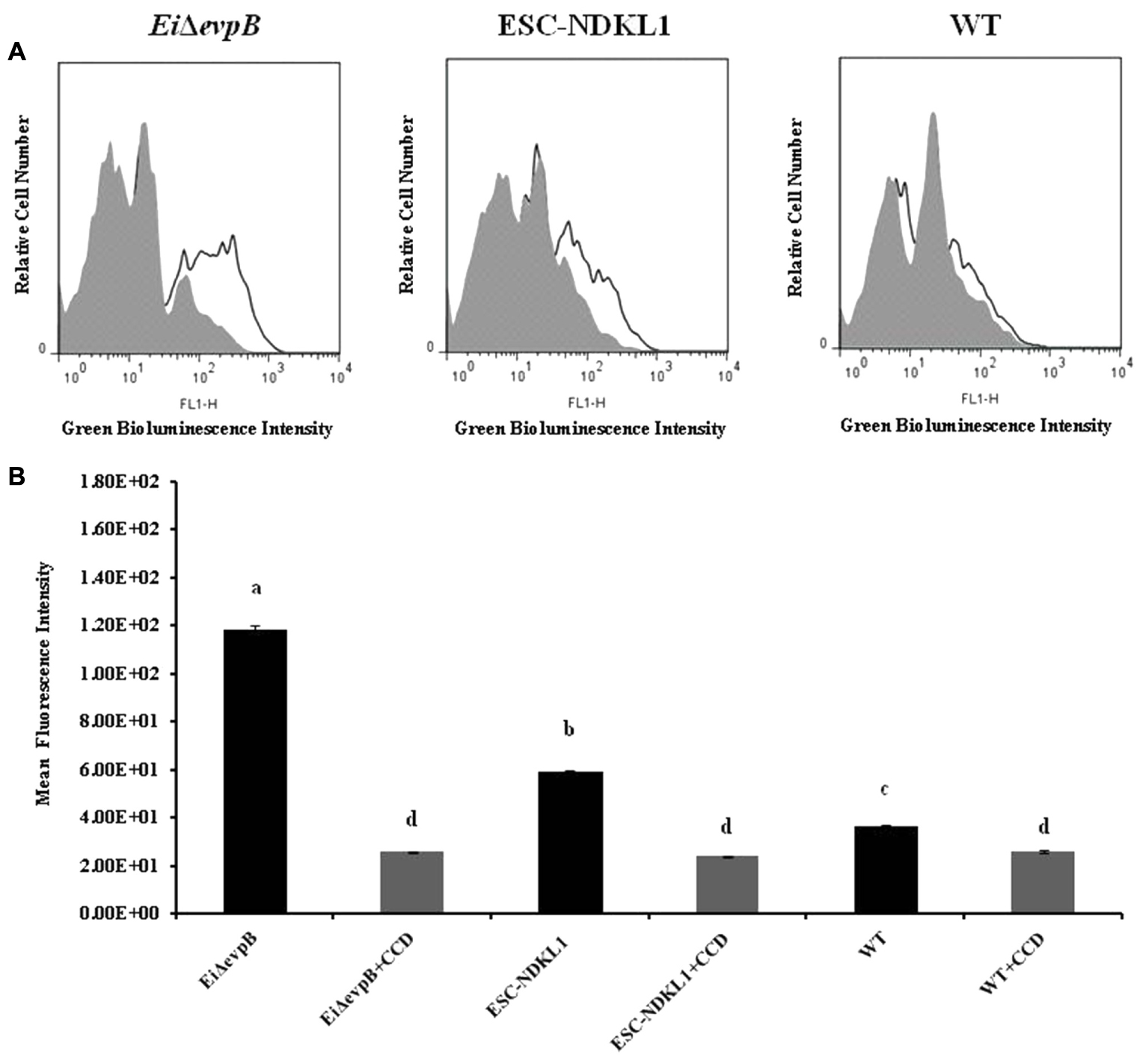
FIGURE 1. Active phagocytic uptake of EiΔevpB and ESC-NDKL1 strains in catfish peritoneal macrophages. (A) Original flow data by using overlay histogram statistics: Black line histograms indicate uptake of the LAVs and WT strains at 32°C. Gray histograms indicate antigen uptake in the presence of CCD at 32°C. (B) Statistical analysis of the phagocytic uptake mean of fluorescent intensity (MFI). Black filled columns in the graph show MFI of LAVs and WT strains uptake at 32°C. Gray filled columns show MFI of antigen uptake in the presence of CCD at 32°C. The data represent the mean of MFI of macrophage phagocytic uptake from five fish ± SD. The letters (a,b,c,d) show the significant differences between treatments (P < 0.05).
The engulfed intracellular WT and the LAV strains were evident in catfish peritoneal macrophages at both 32 and 4°C temperatures assessed, confirming our previous observation that catfish peritoneal macrophages did not lose their phagocytic properties at 4°C (Figure 2). In summary, our data showed the significant increases in bacterial uptake at 32°C compared to 4°C, the inhibitory effects of CCD, and the presence of engulfed intracellular bacteria demonstrated an active uptake of WT and LAVs in catfish peritoneal macrophages. Furthermore, both LAVs were taken more vigorously by macrophages compared to their WT counterpart, suggesting the LAVs advantage for elimination and/or processing for antigen presentation.
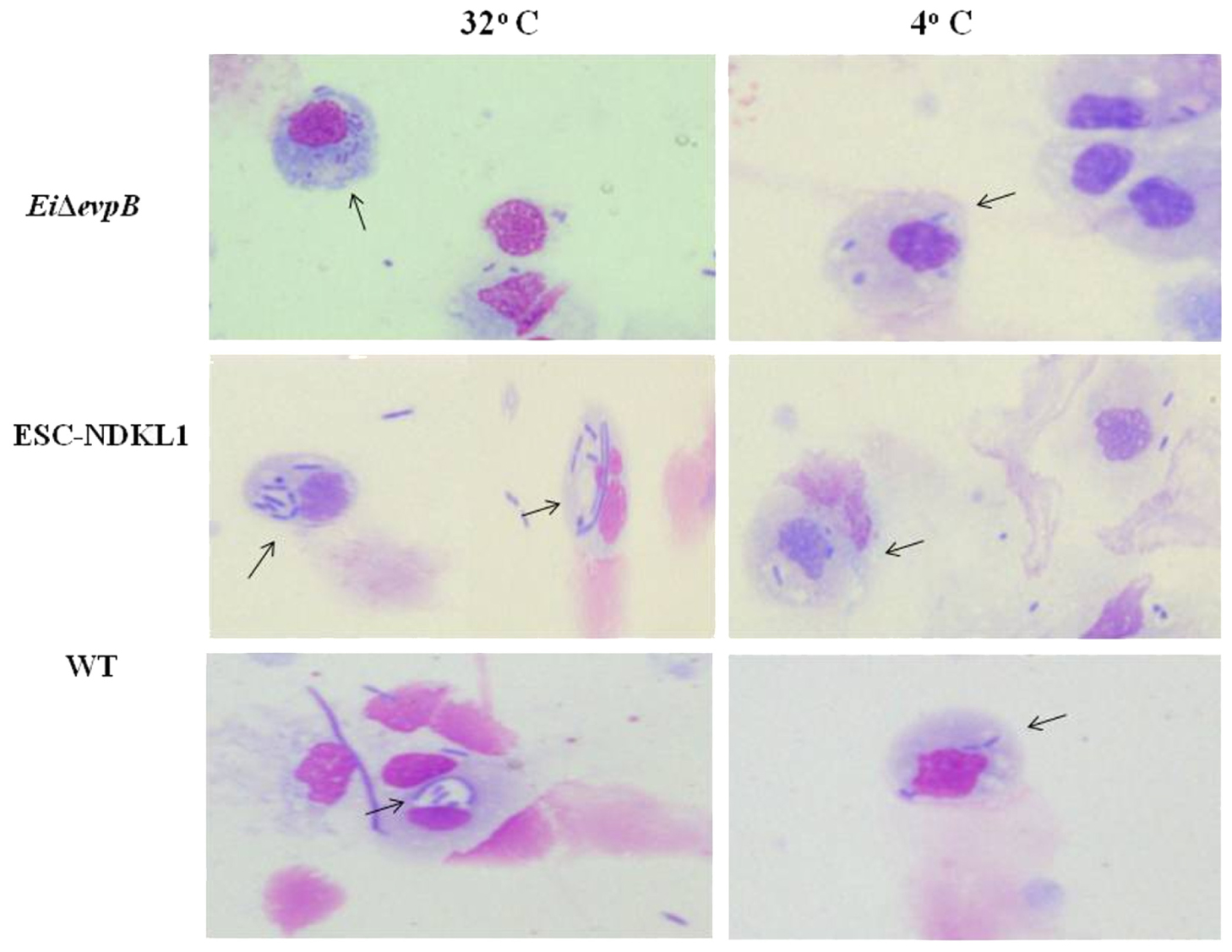
FIGURE 2. Active uptake of ESC vaccine strains in catfish peritoneal macrophages shown by light microscopy. The column on the left shows phagocytosis of E. ictaluri strains at 32°C, and the right column shows phagocytosis E. ictaluri strains at 4°C. Arrows indicate intracellular, engulfed bacterial cells in the cytoplasm and phagosomes of peritoneal macrophages.
Active Uptake of E. ictaluri Opsonized with the LAVs-Induced Immune Sera in Peritoneal Macrophages
Opsonization of WT E. ictaluri with sera from fingerlings vaccinated with both LAVs and non-vaccinated control was applied to determine the protective effect of antibodies and complement on E. ictaluri phagocytosis in peritoneal macrophages. The MFI of phagocytosis of WT E. ictaluri opsonized with intact (IN) and HI sera from vaccinated and control catfish was assessed by flow cytometry (Figure 3). We found significant group- and treatment-related differences in the intensity of phagocytic uptake of WT E. ictaluri compared to LAV’s induced phagocytosis in catfish peritoneal macrophages. Namely, peritoneal macrophages had increased significantly higher uptake of opsonized bacteria compared to the non-opsonized E. ictaluri (data not shown). Phagocytosis of WT E. ictaluri opsonized with ESC-NDKL1-derived serum was increased significantly compared to the EiΔevpB, and IN serum-opsonized bacteria and did not differ from the uptake of WT E. ictaluri opsonized the WT-derived serum (Figure 3). Additionally, we found significant increases in phagocytosis of opsonized bacteria at 4°C showing similar patterns to the increases at 32°C (Figure 4). These patterns of uptake at 4°C, however, had significantly less phagocytic activity compared to phagocytosis occurring at 32°C (Figure 3). As expected, our results indicated that E. ictaluri opsonized with sera from vaccinated fish were taken by peritoneal macrophages more efficiently compared to the bacterium opsonized with sera from control animals, suggesting an important role of humoral responses at the early step of E. ictaluri antigen presentation.
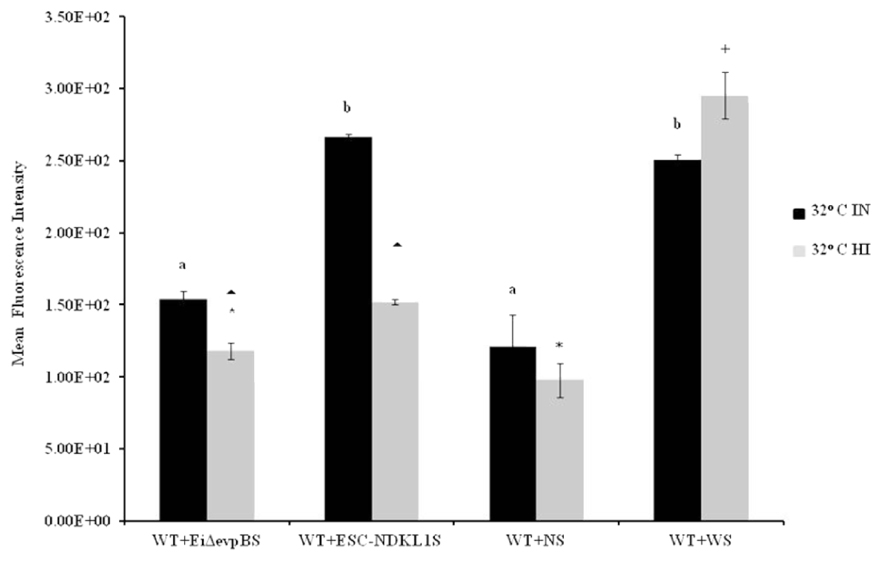
FIGURE 3. Active uptake of E. ictaluri LAVs opsonized with IN and HI sera from challenged fingerlings in catfish peritoneal macrophages at 32°C. EiΔevpB and ESC-NDKL1S indicate serum of vaccinated fish with EiΔevpB and ESC-NDKL1, respectively; WS indicates serum from fish challenged with WT, and NS indicates serum from control fish. a,bPresence of letters on top of bars indicates group differences in uptakes with bars with different letters being different from each other and from bars without a letter designation (P < 0.05). ∗Indicates treatment differences in the uptakes (P < 0.05). ∧+Indicate group differences in the uptakes (P < 0.05). The data represent the mean of MFI of macrophage phagocytic uptake from five fish ± SD.
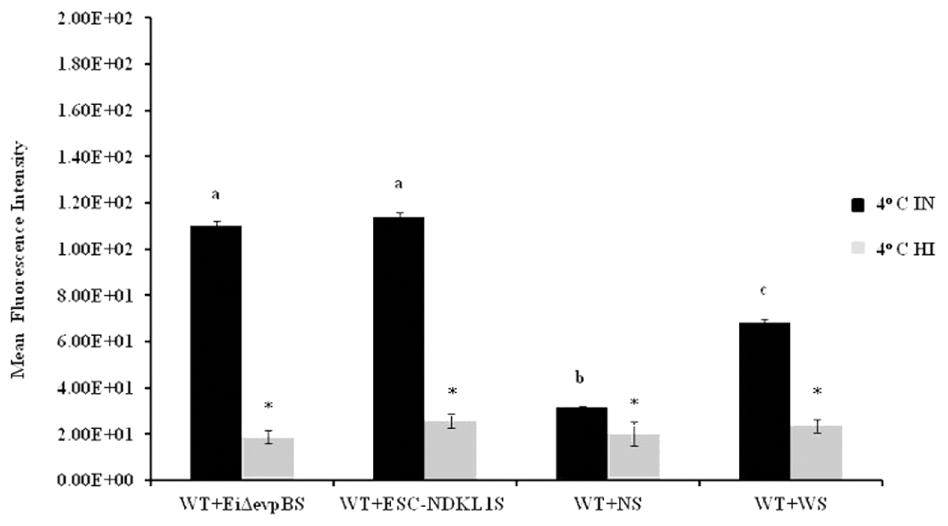
FIGURE 4. Active uptake of E. ictaluri LAVs opsonized with IN and HI sera from challenged fingerlings in catfish peritoneal macrophages at 4°C. a,b,cPresence of letters on top of bars indicates treatment differences in the uptakes with bars with different letters being different from each other and from bars without a letter designation (P < 0.05). ∗Indicates treatment differences in the uptakes (P < 0.05). The data represent the mean of MFI of macrophage phagocytic uptake from five fish ± SD.
Active Uptake of E. ictaluri Opsonized with the LAVs-Induced Heat-Inactivated Immune Sera
Opsonization of WT E. ictaluri with HI sera from fingerlings vaccinated with both LAVs and non-vaccinated controls was utilized to determine the effect of complement on E. ictaluri phagocytosis in peritoneal macrophages. E. ictaluri opsonized with complement-inactivated sera derived from normal and ESC-NDKL-challenged fingerlings was phagocytosed at significantly lower rates compared to the bacteria treated with EiΔevpB and WT treatment derived sera (Figure 3). There were no significant differences in the intensity of phagocytic uptake of bacteria treated with HI EiΔevpB and WT sera (Figure 3). Significant increases in the levels of phagocytosis were evident in all E. ictaluri treatment groups compared to the uptake of untreated bacterial cells (data not shown). However, inactivation of complement did not affect the uptake of WT serum opsonized E. ictaluri in peritoneal macrophages (Figure 3). Our results showed clearly that active uptakes of E. ictaluri and LAVs candidates in catfish peritoneal macrophages at 32°C were mediated through the complement-dependent pathway.
We assessed phagocytic activity in catfish peritoneal macrophages exposed to WT E. ictaluri treated with IN and HI sera obtained from the challenged and control fish at 4°C (Figure 4). Phagocytic uptake of bacteria opsonized with sera obtained from fish challenged with both LAVs did not show significant differences. However, they did express significant increases compared to E. ictaluri opsonized in the presence of HI sera from challenged and control fish (Figure 4). As expected, phagocytosis of E. ictaluri opsonized with sera from control animals was significantly lower compared to the uptake of bacteria opsonized with sera from all challenged fish groups (Figure 4). Notably, phagocytosis in peritoneal macrophages exposed to E. ictaluri opsonized with HI sera obtained from the challenged and control fish showed dramatically decreased background levels of phagocytic activity (Figure 4). These results indicate strongly that complement was a crucial role in phagocytosis of E. ictaluri and LAVs candidates by peritoneal macrophages.
Macrophages Killing of E. ictaluri Opsonized with the LAVs-Induced Immune Sera
To examine how effective peritoneal macrophages are at destroying ingested bacteria, we performed the bacterial killing assay with E. ictaluri opsonized with IN sera from fish challenged with E. ictaluri LAVs, the WT strain, and control non-vaccinated fish (Figure 5). Initial numbers of colonies in all groups exposed to E. ictaluri did not show significant differences. However, the bacteria-killing capacity of peritoneal macrophages exposed to non-opsonized WT E. ictaluri was significantly lower compared to their counterparts treated with opsonized bacteria (Figure 5). No significant differences in killing capacity were evident in macrophages exposed to E. ictaluri treated with control, WT, or LAV-challenged fish derived sera following 10 h exposure (Figure 5). Numbers of bacterial colonies did not differ significantly in all experimental groups exposed to opsonized E. ictaluri 24 h after infection. However, consistent numerical increases in numbers of colonies were evident in peritoneal macrophages exposed to the WT strain, E. ictaluri opsonized with sera derived from control, and the WT-infected fish compared to their counterparts treated with bacteria opsonized with both LAVs-induced immune sera (Figure 5). The virtually absent bacterial colonies in peritoneal macrophages exposed to the sera from fish challenged with E. ictaluri LAVs indicated that peritoneal macrophages efficiently killed LAVs strains after 24 h of in vitro infection. No bacterial colonies were evident in the negative control of uninfected macrophages (Figure 5). The use of HI sera in the bacterial killing assay, in general, showed the patterns that did not differ significantly from IN serum–treated bacteria (data not shown).
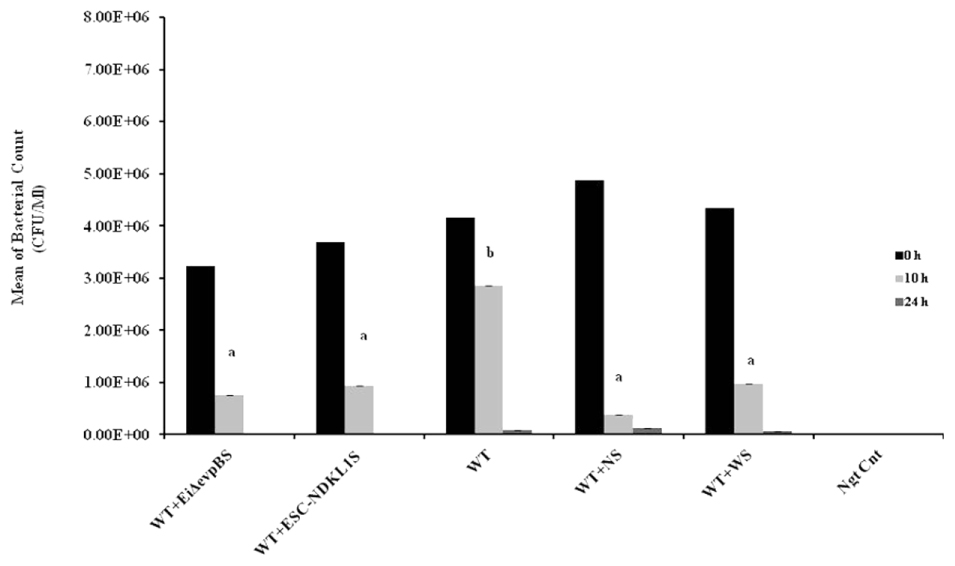
FIGURE 5. Bacterial killing of E. ictaluri LAVs opsonized with sera from challenged fingerlings in catfish peritoneal macrophages. Black columns indicate the colony numbers at 0 h, and gray columns indicate the colony numbers at 10 h, dark gray color columns indicate the colony numbers after 24 h of in vitro infection. a,bPresence of letters on top of bars indicates group differences in the uptakes with bars with different letters being different from each other and from bars without a letter designation (P < 0.05). The data represent the mean of MFI of macrophage phagocytic uptake from five fish ± SD.
Discussion
Recent studies show that monocytes and macrophages are potent APCs that prime naïve T cells, and initiate adaptive cellular and humoral immune responses (Sakaguchi et al., 1995, 1996; Asano et al., 1996). Phagocytosis, which depends on membrane–cytoskeleton interactions, is an important step that mediates innate immune recognition by professional antigen presenting cells (APCs) and triggers adaptive immune responses (Sakaguchi et al., 1995). The current research aimed to assess the phagocytic and bactericidal activity of peritoneal macrophages in the uptake of WT E. ictaluri and two LAV candidate strains. Our conclusions agree with those of multiple studies that document strong phagocytic capability and bactericidal activity of peritoneal macrophages against intracellular pathogens (Esteban and Meseguer, 1997; António et al., 1998; Do Vale et al., 2002; Awasthi et al., 2015). We documented enhanced vaccine strains phagocytic capacity and effective bacterial killing ability of catfish peritoneal macrophages in vitro. Interestingly, the intensity of EiΔevpB LAV pahgocytic uptake was significantly higher compared to the ESC-NDKL1 LAV uptake in catfish peritoneal macrophages suggesting its advantage to be destroyed and processed into peptides by the APCs compared to ESC-NDKL1 LAV strain. Our previous report showed that catfish fry vaccinated with ESC-NDKL1 had higher mortality rates compared to the fry vaccinated with in EiΔevp LAV in E. ictaluri challenge (Nho et al., 2017). However, more research should be done to establish the necessary mechanistic framework for the LAV-dependent pathogenesis in catfish fry and fingerlings. Several earlier reports in humans and other mammals showed high-intensity antigen uptake at 37°C and low background levels of endocytosis at 4°C in professional APCs (Boyd et al., 2004; Ammari et al., 2014). Hohn et al. (2009) confirmed the data obtained in the mammalian studies on the low background levels of E. ictaluri phagocytosis at 4°C by using zebrafish anterior kidney/monocyte/macrophage/granulocyte phagocytes. However, contrary to the data derived from mammals, optimal conditions of antigen uptake in fish professional APCs are not well described yet. In contrast to previous observations, we report that active bacterial uptake in catfish peritoneal macrophages was detected at 32 and 4°C with significantly higher intensity at 32°C. Differences from earlier observations in our study regarding the intensity of active phagocytosis at 4°C could be due to several factors. First, there are phenotypic and functional differences between species in particular macrophages, and between APCs. Second, there are some fish species-specific differences in APC functions. Finally, monocytes, macrophages, and other professional phagocytes differ in the antigen uptake capacity due to their different locations and functions.
Importantly, our data agree with and contribute to the previous report that phagocytosis of LAV strains and WT E. ictaluri at 32°C was inhibited significantly in the presence of the actin formation inhibitor CCD, suggesting active uptake of E. ictaluri strains in catfish peritoneal macrophages (Hohn et al., 2009; Ammari et al., 2014). In particular, similar results were obtained with the uptake of WT E. ictaluri in the presence of CCD by zebrafish kidney phagocytes in the presence of CCD (Hohn et al., 2009). Also, a recent study showed that endocytosis of FITC-OVA in bovine monocytes was decreased significantly in the presence of CCD (Tomoda et al., 1989). In addition to the significantly increased intensity of bacterial phagocytosis at 32°C and substantial inhibitory effect of CCD on the endocytic uptake, we demonstrated the presence of engulfed intracellular bacteria in the cytoplasm and phagosomes of peritoneal macrophages, thus confirming the active endocytic mechanisms of WT E. ictaluri and LAVs in catfish APCs. Furthermore, both LAVs were endocytosed more vigorously by peritoneal macrophages compared to their WT counterpart suggesting the LAVs advantage to be processed and presented in the form of peptides to specific lymphocytes, and subsequently destroyed.
Phagocytosis is a receptor-mediated process, and these receptors are classified into two groups: non-opsonic receptors (e.g., Dectin-1 and CD36) and opsonic receptors (e.g., FcγRIIA and Mac-1) (Araki et al., 1996; Schlam et al., 2015; Levin et al., 2016). Non-opsonic receptors can recognize directly and bind to chemical structures present on the surface of pathogens, whereas opsonic receptors can recognize indirectly phagocytic targets via binding to immunoglobulins (e.g., IgG) or complement C3b (Flannagan et al., 2012; Levin et al., 2016). Opsonin C3b molecules are generated by complement activation, bind covalently to the pathogen surface to create a destruction by phagocytes, which have receptors [e.g., CR1 (CD35) and CRIg] for complement C3b protein (Erdei et al., 2016). In this study, we assessed the role of complement and antibodies in active uptake of E. ictaluri by peritoneal macrophages in catfish at 4 and 32°C. As expected, our results indicated that E. ictaluri opsonized with sera from vaccinated fish was endocytosed by peritoneal macrophages more efficiently compared to the bacteria opsonized with sera from control animals, suggesting an important role of secondary humoral responses at an early stage of LAVs antigen presentation. Our results agree with previous reports on the enhanced phagocytosis of bacteria in the presence of immune sera from vaccinated fish. Namely, Russo et al. (2009) showed that opsonization of E. ictaluri with serum from vaccinated fish augmented the in vitro phagocytic ability of macrophages in catfish. Also, another study (Esteban and Meseguer, 1997) reported that macrophages from sea bass showed greater phagocytic activity against opsonized bacteria. Recently, human monocyte-derived macrophages infected with Francisella tularensis showed 40 times more phagocytic activity in the presence of serum (Dai et al., 2013).
To confirm the significant contribution of complement-dependent mechanisms in the phagocytosis of E. ictaluri, we examined the uptake of the bacteria opsonized with HI sera from fish vaccinated with ESC-LAVs. We reported significant decreases in phagocytosis activity of peritoneal macrophages at both temperatures, which suggest the importance of CR ligation in E. ictaluri phagocytosis. Notably, the intensity of bacterial endocytosis was reduced dramatically to virtually background levels at 4°C, suggesting that bacterial uptake in catfish peritoneal macrophages at low temperatures, unlike that at 32°C, is predominantly CR-mediated. In contrast, the increased phagocytic activity of bacteria opsonized with HI WT serum in macrophages was evident at 32°C, suggesting a dominant role of the opsonic Fc receptors. Our findings are in agreement with earlier reports on the role of complement opsonic receptors in the endocytic activity of professional phagocytes. Namely, phagocytic activity of rainbow trout macrophages decreased significantly when Mycobacterium marine was treated with HI serum (Shih-Chu et al., 1998). HT serum also suppressed significantly phagocytosis of Staphylococcus aureus and zymosan particles in human macrophages (Peterson et al., 1977; Shih-Chu et al., 1998; Mankovich et al., 2013). Heat inactivation disturbs the complement cascade and removes C3 opsonins thereby decreasing phagocytic activity in monocyte-derived human macrophages (Chan et al., 2001). Taken together, various data indicate the importance of complement molecules engagement for efficient phagocytosis of bacterial cells. Our results also demonstrate that complement-mediated phagocytosis is temperature dependent in catfish peritoneal macrophages. Our data are in agreement with several previous observations that complement system in teleost fish is functionally active at low temperatures suggesting the enhanced complement-dependent phagocytosis compared to the mammalian counterpart (Sunyer and Tort, 1995; Sunyer and Lambris, 1998; Boshra et al., 2006).
Finally, we applied a bacterial killing assay to assess the efficacy of the opsonized E. ictaluri destruction compared to the killing ability of the non-opsonized WT bacterial strain. We confirmed the previously reported observation by Shoemaker et al. (1997) on the effective killing of opsonized E. ictaluri by catfish peritoneal macrophages compared to the phagocytes exposed to the non-opsonized WT strain. Our bacterial killing data in peritoneal macrophages showed that the numbers of bacterial colonies in peritoneal macrophages exposed to the opsonized WT E. ictaluri were significantly reduced compared to the phagocytes exposed to the non-opsonized WT strain showing significant differences at time 10 h post-exposure. In our study, the intensity of the immune sera opsonized WT E. ictaluri uptakes did not correlate with the bacterial uptake in peritoneal macrophages, suggesting the different sensitivity of the experimental approaches with active uptake by flow cytometry being more sensitive. We supported the data on active bacterial uptake in peritoneal macrophages by flow cytometry with significant inhibition of phagocytosis in the presence of actin formation inhibitor, CCD (1); significantly decreased uptake at 4°C compared to the uptake at 32°C (2), and finally, with the evidence of the internalized bacterial strains by light microscopy. However, in order to assess the bactericidal properties in professional phagocytes, we performed bacterial killing assay. Absence of bacterial colonies in peritoneal macrophages exposed to the sera from fish challenged with E. ictaluri LAVs indicated that peritoneal macrophages efficiently killed WT E. ictaluri strain after 24 h of in vitro infection. Taken together, both approaches can provide valuable data on phagocytic properties and effective killing properties in professional phagocytes.
Conclusion
Efficacious E. ictaluri LAVs are expected to induce active antigen uptake by phagocytosis and antigen presentation in catfish APCs such that infected monocytes/macrophages can initiate early activation of the innate immune system and acquired immunity mediated by T and B cells. Our study demonstrated that both vaccine candidates were endocytosed efficiently by catfish peritoneal macrophages showing significant increases in the intensity of uptake compared to their WT E. ictaluri counterpart. Importantly, both LAVs induced humoral immunity in the challenged fish resulting in significant increases in WT E. ictaluri uptake and bacterial destruction in catfish peritoneal macrophages in vitro. Our data on enhanced phagocytic capacity and effective killing ability of macrophages against ESC vaccine strains suggest the LAVs advantage to be processed and presented in the form of peptides to the specific lymphocytes of the adaptive immune system and support the importance of macrophage-mediated immunity against ESC in catfish.
Author Contributions
LP and AK conceived and designed the experiments. LP and AK provided original idea of the study. AOK, HAb, HAh, and JP performed the experiments. LP and AK contributed reagents/materials/tools. AOK wrote the first draft of the manuscript and was involved in all aspects of the study. All authors were involved in critical interpretation of the data, manuscript revision, and final version approval.
Funding
This project was supported by Agriculture and Food Research Initiative Competitive grant nos. 2014-70007-22359 and 2016-67015-24909 from the USDA National Institute of Food and Agriculture.
Conflict of Interest Statement
The authors declare that the research was conducted in the absence of any commercial or financial relationships that could be construed as a potential conflict of interest.
Acknowledgments
The authors thank the Laboratory for Organismal and Cellular Imaging at the Department of Animal and Diary Sciences and the Flow Cytometry Core Facility at the College of Veterinary Medicine. They also thank Dr. John Harkness for his editorial suggestions.
Abbreviations
APCs, antigen-presenting cells; BHI, brain heart infusion; CCD, cytochalasin D; CCMM, Channel Catfish Macrophage Medium; CR, complement receptor; E. ictaluri, Edwardsiella ictaluri; ESC, enteric septicemia of catfish; HI, heat-inactivated; IN, intact serum; IP, intraperitoneal; LAV, live attenuated vaccine; MFI, mean fluorescence intensity; SPF, specific pathogen free; WT, wild-type.
References
Abdelhamed, H., Lu, J., Shaheen, A., Abbass, A., Lawrence, M. L., and Karsi, A. (2013). Construction and evaluation of an Edwardsiella ictaluri fhuC mutant. Vet. Microbiol. 162, 858–865. doi: 10.1016/j.vetmic.2012.11.006
Ammari, M. G., Harris, A. N., Stokes, J. V., Bailey, R. H., and Pinchuk, L. M. (2014). Negative regulatory effects of phosphatidylinositol3-kinase pathway on phagocytosis and macropinocytosis in bovine monocytes. J. Vet. Med. Res. 1:1008.
António, A., Susana, L., Joana, S., Anthony, E. E., and Manuel, T. S. (1998). Neutrophil and macrophage responses to inflammation in the peritoneal cavity of rainbow trout Oncorhynchus mykiss. A light and electron microscopic cytochemical study. Dis. Aquat. Organ. 34, 27–37. doi: 10.3354/dao034027
Araki, N., Johnson, M. T., and Swanson, J. A. (1996). A role for phosphoinositide 3-kinase in the completion of macropinocytosis and phagocytosis by macrophages. J. Cell Biol. 135, 1249–1260. doi: 10.1083/jcb.135.5.1249
Asano, M., Toda, M., Sakaguchi, N., and Sakaguchi, S. (1996). Autoimmune disease as a consequence of developmental abnormality of a T cell subpopulation. J. Exp. Med. 184, 387–396. doi: 10.1084/jem.184.2.387
Awasthi, A., Rathore, G., Sood, N., Khan, M. Y., and Lakra, W. S. (2015). Establishment of a leukocyte cell line derived from peritoneal macrophages of fish, Labeo rohita (Hamilton, 1822). Cytotechnology 67, 85–96. doi: 10.1007/s10616-013-9660-5
Bennani, N., Schmid-Alliana, A., and Lafaurie, M. (1995). Evaluation of phagocytic activity in a teleost fish, Dicentrarchus labrax. Fish Shellfish Immunol. 5, 237–246. doi: 10.1016/S1050-4648(05)80017-8
Bodammer, J. E., and Robohm, R. A. (1996). Ultrastructural observations on the phagocytic behavior of winter flounder Pleuronectes americanus peritoneal neutrophils and macrophages in vivo. Dis. Aquat. Organ. 25, 197–208. doi: 10.3354/dao025197
Booth, N. J., Elkamel, A., and Thune, R. L. (2006). Intracellular replication of Edwardsiella ictaluri in channel catfish macrophages. J. Aquat. Anim. Health 18, 101–108. doi: 10.1577/H05-025.1
Boshra, H., Li, J., and Sunyer, J. O. (2006). Recent advances on the complement system of teleost fish. Fish Shellfish Immunol. 20, 239–262. doi: 10.1016/j.fsi.2005.04.004
Boyd, B. L., Lee, T. M., Kruger, E. F., and Pinchuk, L. M. (2004). Cytopathic and non-cytopathic bovine viral diarrhoea virus biotypes affect fluid phase uptake and mannose receptor-mediated endocytosis in bovine monocytes. Vet. Immunol. Immunopathol. 102, 53–65. doi: 10.1016/j.vetimm.2004.06.009
Chan, H.-T., Kedzierska, K., O’Mullane, J., Crowe, S. M., and Jaworowski, A. (2001). Quantifying complement-mediated phagocytosis by human monocyte-derived macrophages. Immunol. Cell Biol. 79, 429–435. doi: 10.1046/j.1440-1711.2001.01027.x
Dahal, N., Abdelhamed, H., Lu, J., Karsi, A., and Lawrence, M. L. (2013). Tricarboxylic acid cycle and one-carbon metabolism pathways are important in Edwardsiella ictaluri virulence. PLOS ONE 8:e65973. doi: 10.1371/journal.pone.0065973
Dai, S., Rajaram, M. V. S., Curry, H. M., Leander, R., and Schlesinger, L. S. (2013). Fine tuning inflammation at the front door: macrophage complement receptor 3-mediates phagocytosis and immune suppression for Francisella tularensis. PLOS Pathog. 9:e1003114. doi: 10.1371/journal.ppat.1003114
Deretic, V., Saitoh, T., and Akira, S. (2013). Autophagy in infection, inflammation, and immunity. Nat. Rev. Immunol. 13, 722–737. doi: 10.1038/nri3532
Dieter, S., and Katharina, H. (1997). Carp coccidiosis: activity of phagocytic cells from common carp infected with Goussia carpelli. Dis. Aquat. Organ. 31, 155–159. doi: 10.3354/dao031155
Do Vale, A., Afonso, A., and Silva, M. T. (2002). The professional phagocytes of sea bass (Dicentrarchus labrax L.): cytochemical characterisation of neutrophils and macrophages in the normal and inflamed peritoneal cavity. Fish Shellfish Immunol. 13, 183–198. doi: 10.1006/fsim.2001.0394
Dumpala, P. R., Peterson, B. C., Lawrence, M. L., and Karsi, A. (2015). Identification of differentially abundant proteins of Edwardsiella ictaluri during iron restriction. PLOS ONE 10:e0132504. doi: 10.1371/journal.pone.0132504
Ellis, A. E. (1999). Immunity to bacteria in fish. Fish Shellfish Immunol. 9, 291–308. doi: 10.1006/fsim.1998.0192
Epelman, S., Lavine Kory, J., Beaudin Anna, E., Sojka Dorothy, K., Carrero Javier, A., Calderon, B., et al. (2014). Embryonic and adult-derived resident cardiac macrophages are maintained through distinct mechanisms at steady state and during inflammation. Immunity 40, 91–104. doi: 10.1016/j.immuni.2013.11.019
Erdei, A., Sándor, N., Mácsik-Valent, B., Lukácsi, S., Kremlitzka, M., and Bajtay, Z. (2016). The versatile functions of complement C3-derived ligands. Immunol. Rev. 274, 127–140. doi: 10.1111/imr.12498
Esteban, M. A., and Meseguer, J. (1997). Factors influencing phagocytic response of macrophages from the sea bass (Dicentrarchus labrax L.): an ultrastructural and quantitative study. Anat. Rec. 248, 533–541. doi: 10.1002/(SICI)1097-0185(199708)248:4<533::AID-AR5>3.0.CO;2-M
Esteban, M. A., Mulero, V., Munoz, J., and Meseguer, J. (1998). Methodological aspects of assessing phagocytosis of Vibrio anguillarum by leucocytes of gilthead seabream (Sparus aurata L.) by flow cytometry and electron microscopy. Cell Tissue Res. 293, 133–141. doi: 10.1007/s004410051105
Esteban, M. Á., Cuesta, A., Chaves-Pozo, E., and Meseguer, J. (2015). Phagocytosis in teleosts. Implications of the new cells involved. Biology 4, 907–922. doi: 10.3390/biology4040907
Flannagan, R. S., Heit, B., and Heinrichs, D. E. (2015). Antimicrobial mechanisms of macrophages and the immune evasion strategies of Staphylococcus aureus. Pathogens 4, 826–868. doi: 10.3390/pathogens4040826
Flannagan, R. S., Jaumouillé, V., and Grinstein, S. (2012). The cell biology of phagocytosis. Annu. Rev. Pathol. 7, 61–98. doi: 10.1146/annurev-pathol-011811-132445
García-Valtanen, P., Ortega-Villaizán Mdel, M., Martínez-López, A., Medina-Gali, R., Pérez, L., Mackenzie, S., et al. (2014). Autophagy-inducing peptides from mammalian VSV and fish VHSV rhabdoviral G glycoproteins (G) as models for the development of new therapeutic molecules. Autophagy 10, 1666–1680. doi: 10.4161/auto.29557
Godwin, J. W., Pinto, A. R., and Rosenthal, N. A. (2013). Macrophages are required for adult salamander limb regeneration. Proc. Natl. Acad. Sci. U.S.A. 110, 9415–9420. doi: 10.1073/pnas.1300290110
Greenberg, S., el Khoury, J., di Virgilio, F., Kaplan, E. M., and Silverstein, S. C. (1991). Ca2+-independent F-actin assembly and disassembly during Fc receptor-mediated phagocytosis in mouse macrophages. J. Cell Biol. 113, 757–767. doi: 10.1083/jcb.113.4.757
Hashimoto, D., Chow, A., Noizat, C., Teo, P., Beasley Mary, B., Leboeuf, M., et al. (2013). Tissue-resident macrophages self-maintain locally throughout adult life with minimal contribution from circulating monocytes. Immunity 38, 792–804. doi: 10.1016/j.immuni.2013.04.004
Hawke, J. P. M., Alma, C., Steigerwalt, A. G., and Brenner, D. J. (1981). Edwardsiella ictaluri sp. nov., the causative agent of enteric septicemia of catfish. Int. J. Syst. Evol. Microbiol. 31, 396–400. doi: 10.1099/00207713-31-4-396
Herre, J., Marshall, A. S. J., Caron, E., Edwards, A. D., Williams, D. L., Schweighoffer, E., et al. (2004). Dectin-1 uses novel mechanisms for yeast phagocytosis in macrophages. Blood 104, 4038–4045. doi: 10.1182/blood-2004-03-1140
Hoeffel, G., Chen, J., Lavin, Y., Low, D., Almeida Francisca, F., See, P., et al. (2015). C-Myb+ erythro-myeloid progenitor-derived fetal monocytes give rise to adult tissue-resident macrophages. Immunity 42, 665–678. doi: 10.1016/j.immuni.2015.03.011
Hohn, C., Lee, S.-R., Pinchuk, L. M., and Petrie-Hanson, L. (2009). Zebrafish kidney phagocytes utilize macropinocytosis and Ca2+-dependent endocytic mechanisms. PLOS ONE 4:e4314. doi: 10.1371/journal.pone.0004314
Holland, M. C. H., and Lambris, J. D. (2002). The complement system in teleosts. Fish Shellfish Immunol. 12, 399–420. doi: 10.1006/fsim.2001.0408
Jenkins, J. A., and Klesius, P. H. (1998). Elicitation of macrophages from the peritoneal cavity of channel catfish. J. Aquat. Anim. Health 10, 69–74. doi: 10.1577/1548-8667(1998)010<0069:EOMFTP>2.0.CO;2
Karsi, A., and Lawrence, M. L. (2007). Broad host range fluorescence and bioluminescence expression vectors for Gram-negative bacteria. Plasmid 57, 286–295. doi: 10.1016/j.plasmid.2006.11.002
Klesius, P. H., and Shoemaker, C. A. (1999). “Development and use of modified live Edwardsiella ictaluri vaccine against enteric septicemia of catfish,” in Advances in Veterinary Medicine, ed. R. D. Schultz (San Diego, CA: Academic Press), 523–537.
Lawrence, M. L., Cooper, R. K., and Thune, R. L. (1997). Attenuation, persistence, and vaccine potential of an Edwardsiella ictaluri purA mutant. Infect. Immun. 65, 4642–4651.
Levin, R., Grinstein, S., and Canton, J. (2016). The life cycle of phagosomes: formation, maturation, and resolution. Immunol. Rev. 273, 156–179. doi: 10.1111/imr.12439
Mamnur Rashid, M., Sardar, M., and Islam, M. R. (2002). In vitro phagocytic study of blood leucocytes and peritoneal macrophages of walking catfish Clarias batrachus against Aeromonas hydrophila and Escherichia coli. Bangladesh J. Fish. Res. 6, 35–41.
Mankovich, A. R., Lee, C.-Y., and Heinrich, V. (2013). Differential effects of serum heat treatment on chemotaxis and phagocytosis by human neutrophils. PLOS ONE 8:e54735. doi: 10.1371/journal.pone.0054735
Merle, N. S., Church, S. E., Fremeaux-Bacchi, V., and Roumenina, L. T. (2015). Complement system part I – molecular mechanisms of activation and regulation. Front. Immunol. 6:262. doi: 10.3389/fimmu.2015.00262
Miyazaki, T., and Plumb, J. A. (1985). Histopathology of Edwardsiella ictaluri in channel catfish, Ictalurus punctatus (Rafinesque). J. Fish Dis. 8, 389–392. doi: 10.1111/j.1365-2761.1985.tb00961.x
Mizumura, K., Choi, A. M. K., and Ryter, S. W. (2014). Emerging role of selective autophagy in human diseases. Front. Pharmacol. 5:244. doi: 10.3389/fphar.2014.00244
Muller, S., Faulhaber, A., Sieber, C., Pfeifer, D., Hochberg, T., Gansz, M., et al. (2014). The endolysosomal cysteine cathepsins L and K are involved in macrophage-mediated clearance of Staphylococcus aureus and the concomitant cytokine induction. FASEB J. 28, 162–175. doi: 10.1096/fj.13-232272
Muñoz, P., Álvarez-Pellitero, P., and Sitjà-Bobadilla, A. (2000). Modulation of the in vitro activity of European sea bass (Dicentrarchus labrax L.) phagocytes by the myxosporean parasite Sphaerospora dicentrarchi (Myxosporea: Bivalvulida). Fish Shellfish Immunol. 10, 567–581. doi: 10.1006/fsim.2000.0272
Nakao, M., Mutsuro, J., Obo, R., Fujiki, K., Nonaka, M., and Yano, T. (2000). Molecular cloning and protein analysis of divergent forms of the complement component C3 from a bony fish, the common carp (Cyprinus carpio): presence of variants lacking the catalytic histidine. Eur. J. Immunol. 30, 858–866. doi: 10.1002/1521-4141(200003)30:3<858::AID-IMMU858>3.0.CO;2-M
Nakao, M., Tsujikura, M., Ichiki, S., Vo, T. K., and Somamoto, T. (2011). The complement system in teleost fish: progress of post-homolog-hunting researches. Dev. Comp. Immunol. 35, 1296–1308. doi: 10.1016/j.dci.2011.03.003
Nakao, M., and Yano, T. (1998). Structural and functional identification of complement components of the bony fish, carp (Cyprinus carpio). Immunol. Rev. 166, 27–38. doi: 10.1111/j.1600-065X.1998.tb01250.x
Nho, S. W., Abdelhamed, H., Karsi, A., and Lawrence, M. L. (2017). Improving safety of a live attenuated Edwardsiella ictaluri vaccine against enteric septicemia of catfish and evaluation of efficacy. Vet. Microbiol. 210, 83–90. doi: 10.1016/j.vetmic.2017.09.004
Patel, P. C., and Harrison, R. E. (2008). Membrane ruffles capture C3bi-opsonized particles in activated macrophages. Mol. Biol. Cell 19, 4628–4639. doi: 10.1091/mbc.E08-02-0223
Patrie-Hanson, L., and Ainsworth, A. J. (1999). Humoral immune responses of channel catfish (Ictalurus punctatus) fry and fingerlings exposed to Edwardsiella ictaluri. Fish Shellfish Immunol. 9, 579–589. doi: 10.1006/fsim.1999.0215
Peterson, P. K., Verhoef, J., and Quie, P. G. (1977). Influence of temperature on opsonization and phagocytosis of staphylococci. Infect. Immun. 15, 175–179.
Petrie-Hanson, L., and Ainsworth, A. J. (2001). Ontogeny of channel catfish lymphoid organs. Vet. Immunol. Immunopathol. 81, 113–127. doi: 10.1016/S0165-2427(01)00331-2
Qiu, W., Liu, S., Chen, J., Hu, L., Wu, M., and Yang, M. (2016). The primary culture of carp (Cyprinus carpio) macrophages and the verification of its phagocytosis activity. In Vitro Cell. Dev. Biol. Anim. 52, 10–19. doi: 10.1007/s11626-015-9942-7
Rombout, J. H., Huttenhuis, H. B. T., Picchietti, S., and Scapigliati, G. (2005). Phylogeny and ontogeny of fish leucocytes. Fish Shellfish Immunol. 19, 441–455. doi: 10.1016/j.fsi.2005.03.007
Russo, R., Shoemaker, C. A., Panangala, V. S., and Klesius, P. H. (2009). In vitro and in vivo interaction of macrophages from vaccinated and non-vaccinated channel catfish (Ictalurus punctatus) to Edwardsiella ictaluri. Fish Shellfish Immunol. 26, 543–552. doi: 10.1016/j.fsi.2009.02.011
Sakaguchi, S., Sakaguchi, N., Asano, M., Itoh, M., and Toda, M. (1995). Immunologic self-tolerance maintained by activated T cells expressing IL-2 receptor alpha-chains (CD25). Breakdown of a single mechanism of self-tolerance causes various autoimmune diseases. J. Immunol. 155, 1151–1164.
Sakaguchi, S., Toda, M., Asano, M., Itoh, M., Morse, S. S., and Sakaguchi, N. (1996). T cell-mediated maintenance of natural self-tolerance: its breakdown as a possible cause of various autoimmune diseases. J. Autoimmun. 9, 211–220. doi: 10.1006/jaut.1996.0026
Schlam, D., Bagshaw, R. D., Freeman, S. A., Collins, R. F., Pawson, T., Fairn, G. D., et al. (2015). Phosphoinositide 3-kinase enables phagocytosis of large particles by terminating actin assembly through Rac/Cdc42 GTPase-activating proteins. Nat. Commun. 6:8623. doi: 10.1038/ncomms9623
Schulz, C., Perdiguero, E. G., Chorro, L., Szabo-Rogers, H., Cagnard, N., Kierdorf, K., et al. (2012). A lineage of myeloid cells independent of Myb and hematopoietic stem cells. Science 336, 86–90. doi: 10.1126/science.1219179
Secombes, C. J., and Fletcher, T. C. (1992). The role of phagocytes in the protective mechanisms of fish. Annu. Rev. Fish Dis. 2, 53–71. doi: 10.1016/0959-8030(92)90056-4
Sheng, J., Ruedl, C., and Karjalainen, K. (2015). Most tissue-resident macrophages except microglia are derived from fetal hematopoietic stem cells. Immunity 43, 382–393. doi: 10.1016/j.immuni.2015.07.016
Shih-Chu, C., Alexandra, A., Kim, D. T., and Randolph, H. R. (1998). Electron microscope studies of the in vitro phagocytosis of Mycobacterium spp. by rainbow trout Oncorhynchus mykiss head kidney macrophages. Dis. Aquat. Organ. 32, 99–110. doi: 10.3354/dao032099
Shoemaker, C. A., and Klesius, P. H. (1997). Protective immunity against enteric septicaemia in channel catfish, Ictalurus punctatus (Rafinesque), following controlled exposure to Edwardsiella ictaluri. J. Fish Dis. 20, 361–368. doi: 10.1046/j.1365-2761.1997.00310.x
Shoemaker, C. A., Klesius, P. H., and Bricker, J. M. (1999). Efficacy of a modified live Edwardsiella ictaluri vaccine in channel catfish as young as seven days post hatch. Aquaculture 176, 189–193. doi: 10.1016/S0044-8486(99)00116-7
Shoemaker, C. A., Klesius, P. H., and Evans, J. J. (2002). In ovo methods for utilizing the modified live Edwardsiella ictaluri vaccine against enteric septicemia in channel catfish. Aquaculture 203, 221–227. doi: 10.1016/S0044-8486(01)00631-7
Shoemaker, C. A., Klesius, P. H., and Evans, J. J. (2007). Immunization of eyed channel catfish, Ictalurus punctatus, eggs with monovalent Flavobacterium columnare vaccine and bivalent F. columnare and Edwardsiella ictaluri vaccine. Vaccine 25, 1126–1131. doi: 10.1016/j.vaccine.2006.09.055
Shoemaker, C. A., Klesius, P. H., and Plumb, J. A. (1997). Killing of Edwardsiella ictaluri by macrophages from channel catfish immune and susceptible to enteric septicemia of catfish. Vet. Immunol. Immunopathol. 58, 181–190. doi: 10.1016/S0165-2427(97)00026-3
Silva, M. T., Silva, M. N., and Appelberg, R. (1989). Neutrophil-macrophage cooperation in the host defence against mycobacterial infections. Microb. Pathog. 6, 369–380. doi: 10.1016/0882-4010(89)90079-X
Sunyer, J. O., and Lambris, J. D. (1998). Evolution and diversity of the complement system of poikilothermic vertebrates. Immunol. Rev. 166, 39–57. doi: 10.1111/j.1600-065X.1998.tb01251.x
Sunyer, J. O., and Tort, L. (1995). Natural hemolytic and bactericidal activities of sea bream Sparus aurata serum are effected by the alternative complement pathway. Vet. Immunol. Immunopathol. 45, 333–345. doi: 10.1016/0165-2427(94)05430-Z
Sunyer, J. O., Tort, L., and Lambris, J. D. (1997). Diversity of the third form of complement, C3, in fish: functional characterization of five forms of C3 in the diploid fish Sparus aurata. Biochem. J. 326, 877–881. doi: 10.1042/bj3260877
Sunyer, J. O., Zarkadis, I. K., Sahu, A., and Lambris, J. D. (1996). Multiple forms of complement C3 in trout that differ in binding to complement activators. Proc. Natl. Acad. Sci. U.S.A. 93, 8546–8551. doi: 10.1073/pnas.93.16.8546
Tomoda, H., Kishimoto, Y., and Lee, Y. C. (1989). Temperature effect on endocytosis and exocytosis by rabbit alveolar macrophages. J. Biol. Chem. 264, 15445–15450.
Tse, S. M., Furuya, W., Gold, E., Schreiber, A. D., Sandvig, K., Inman, R. D., et al. (2003). Differential role of actin, clathrin, and dynamin in Fc gamma receptor-mediated endocytosis and phagocytosis. J. Biol. Chem. 278, 3331–3338. Epub 2002/11/09., doi: 10.1074/jbc.M207966200
van der Vaart, M., Spaink, H. P., and Meijer, A. H. (2012). Pathogen recognition and activation of the innate immune response in zebrafish. Adv. Hematol. 2012:159807. doi: 10.1155/2012/159807
Wagner, B. A., Wise, D. J., Khoo, L. H., and Terhune, J. S. (2006). The epidemiology of bacterial diseases in food-size channel catfish. J. Aquat. Anim. Health 18, 263–272. doi: 10.1577/1548-86672002014
Weinrauch, Y., Elsbach, P., Madsen, L. M., Foreman, A., and Weiss, J. (1996). The potent anti-Staphylococcus aureus activity of a sterile rabbit inflammatory fluid is due to a 14-kD phospholipase A2. J. Clin. Investig. 97, 250–257. doi: 10.1172/JCI118399
Wise, D. J., Klesius, P. H., Shoemaker, C. A., and Wolters, W. R. (2000). Vaccination of mixed and full-sib families of channel catfish Ictalurus punctatus against enteric septicemia of catfish with a live attenuated Edwardsiella ictaluri Isolate (RE-33). J. World Aquac. Soc. 31, 206–212. doi: 10.1111/j.1749-7345.2000.tb00354.x
Wu, Y., Raymond, B., Goossens, P. L., Njamkepo, E., Guiso, N., Paya, M., et al. (2010). Type-IIA secreted phospholipase A2 is an endogenous antibiotic-like protein of the host. Biochimie 92, 583–587. doi: 10.1016/j.biochi.2010.01.024
Yabu, T., Imamura, S., Mizusawa, N., Touhata, K., and Yamashita, M. (2012). Induction of autophagy by amino acid starvation in fish cells. Mar. Biotechnol. 14, 491–501. doi: 10.1007/s10126-012-9432-9
Zapata, A., Diez, B., Cejalvo, T., Gutiérrez-de Frías, C., and Cortés, A. (2006). Ontogeny of the immune system of fish. Fish Shellfish Immunol. 20, 126–136. doi: 10.1016/j.fsi.2004.09.005
Zhang, Y., and Arias, C. R. (2007). Identification and characterization of an intervening sequence within the 23S ribosomal RNA genes of Edwardsiella ictaluri. Syst. Appl. Microbiol. 30, 93–101. doi: 10.1016/j.syapm.2006.04.004
Keywords: Edwardsiella ictaluri, live attenuated vaccines, catfish macrophages, phagocytosis, bacterial killing, complement
Citation: Kordon AO, Abdelhamed H, Ahmed H, Park JY, Karsi A and Pinchuk LM (2018) Phagocytic and Bactericidal Properties of Channel Catfish Peritoneal Macrophages Exposed to Edwardsiella ictaluri Live Attenuated Vaccine and Wild-Type Strains. Front. Microbiol. 8:2638. doi: 10.3389/fmicb.2017.02638
Received: 20 September 2017; Accepted: 18 December 2017;
Published: 09 January 2018.
Edited by:
Jaime Romero, Universidad de Chile, ChileReviewed by:
Javier Santander, Memorial University of Newfoundland, CanadaJorge Galindo-Villegas, Universidad de Murcia, Spain
Copyright © 2018 Kordon, Abdelhamed, Ahmed, Park, Karsi and Pinchuk. This is an open-access article distributed under the terms of the Creative Commons Attribution License (CC BY). The use, distribution or reproduction in other forums is permitted, provided the original author(s) or licensor are credited and that the original publication in this journal is cited, in accordance with accepted academic practice. No use, distribution or reproduction is permitted which does not comply with these terms.
*Correspondence: Lesya M. Pinchuk, pinchuk@cvm.msstate.edu