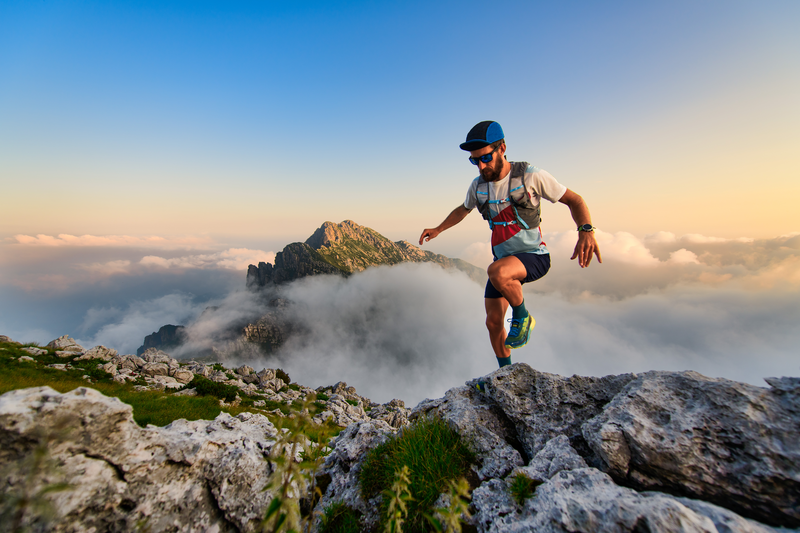
94% of researchers rate our articles as excellent or good
Learn more about the work of our research integrity team to safeguard the quality of each article we publish.
Find out more
ORIGINAL RESEARCH article
Front. Microbiol. , 11 September 2017
Sec. Microbiotechnology
Volume 8 - 2017 | https://doi.org/10.3389/fmicb.2017.01698
Pichia pastoris is widely used as a platform for heterologous protein expression because of its high volumetric productivity. Multicopy integration of the target gene is commonly used to improve the production of the target protein. Cre/lox recombination system is a powerful tool for the marker rescue during multiple integrations with one selection marker. Here we reported a novel expression vector based on the Cre/lox recombination system for multiple integrations of target gene to construct multicopy expression strain of P. pastoris. PAOX1 promoter was fused to cre to construct a methanol inducible Cre recombinase. The leakage expression of Cre recombinase in Escherichia coli was blocked by introducing the operator gene lacO. The expression vector designed pMCO-AOXα was stable in E. coli and could effectively rescue the Zeocin resistance gene for next round of integration in P. pastoris. Phytase AppA from E. coli was chosen as a reporter gene. Transformants with 2–16 copies of appA were constructed by using a single antibiotic. Expression of appA was gene dosage dependent when <12 copies were integrated. The protein yield increased 4.45-folds when 12 copies of appA were integrated comparing with the single copy integration. Our results showed that pMCO-AOXα was highly effective for rational construction of multicopy transformat in P. pastoris.
Over the past few decades, Pichia pastoris has developed into a highly popular expression host for production of recombinant protein (Spohner et al., 2015). Several approaches have been designed to improve the expression level of the target protein, such as codon optimization (Mellitzer et al., 2012; Yu et al., 2013), modification of a signal peptide (Liu et al., 2005; Li et al., 2015), selection of promoters with strong transcription strength (Çalık et al., 2015; Parashar and Satyanarayana, 2016), increasing the gene copy number and co-expression helper protein factors (Zhu et al., 2009; Yang et al., 2016). Multicopy integration of the target genes into the genome of P. pastoris was considered to be the most efficient strategy. To integrate multiple copies of a foreign gene, four methods have been employed: repeat transformations with the target gene, in vitro multimerization, direct selection using high concentrations of antibiotic and the post-transformational vector amplification (PTVA) method (Aw and Polizzi, 2013). The most frequently employed method is to directly select the transformation mixture with increasing concentrations of antibiotics. Among the transformants, some clones may be multicopy transformants (Lincereghino and Lincereghino, 2007). However, these methods should operate with antibiotics at high concentration or with multiple selection markers. The optional selection markers offered by expression hosts are limited. If they are are fully utilized, the extra selection markers required by subsequent integrations may be hardly investigated. The limited resources of selection markers that can be used in P. pastoris is the main bottleneck that hinders the arbitrary integration of the target gene or genes encoding helper protein factors into the P. pastoris genome.
Markerless manipulation in the host is desirable for multiple gene integrations or deletions (Leibig et al., 2008; Weng et al., 2009; Tuntufye and Goddeeris, 2011). Bacteriophage P1 Cre recombinase has proved to be a powerful tool for the removal of selection markers (Sauer, 1987). The Cre/lox recombination system has been used in a wide variety of eukaryotes, including yeasts (Gueldener et al., 2002). However, it has not yet been used to remove selection markers in the construction of high-copy transformants for protein expression in P. pastoris. Generally, markerless gene deletion in yeast is carried out in three steps. First, a selection marker flanked by loxP sites and recombinant arms was integrated into the target gene; then, a vector containing an inductive cre gene was introduced and induced to excise the marker gene from loxP sites; finally, the selection marker was eliminated for next cycle of manipulation. In this method, the cre gene and the disruption cassette cannot be combined in one vector because of the instability caused by possible leakage expression of Cre recombinase; the procedure is tedious and time-consuming. To overcome this disadvantage, a PCR fusion method which combined the cre gene and the disruption cassette in P. pastoris was presented in 2011 (Pan et al., 2011). Meanwhile, by introducing an intron in the cre gene, Agaphonov and Alexandrov (2014) constructed a single vector containing the cre gene and the disruption cassette. Both methods have proved to be effective in yeast genome editing.
Multicopy transformant screening with high concentrations of antibiotics is random. In this study, a vector containing the cre gene and the selection marker excision cassette was developed for construction of a P. pastoris expression strain with a designed copy number of heterologous protein genes.
The subcloning and construction of recombinant plasmids was carried out in E. coli DH10B (Invitrogen). The P. pastoris GS115 strain (Invitrogen) was used as a host for the expression of heterogenous proteins. The vectors pPIC3.5K and pPICZαA were purchased from Invitrogen. The vector pTSC (Yan et al., 2008) was stocked in this lab. pUC57-MCS7 (the original plasmid used for construction of recombinant expression vectors) was from GeneCreate Biological Engineering Co., Ltd, Wuhan, China. E. coli DH10B was cultured in Luria-Bertani medium [1% (w/v) tryptone, 0.5% (w/v) yeast extract, and 1% (w/v) NaCl, pH 7.0] at 37°C. The P. pastoris yeast strain was cultured in YPD medium [1% (w/v) yeast extract, 2% (w/v) peptone, 2% (w/v) dextrose]. YPDZ plates containing Zeocin (100 μg/mL) were used for the selection of positive P. pastoris transformants. Buffered minimal glycerol-complex medium (BMGY) was prepared with 2% (w/v) peptone, 1% (w/v) yeast extract, 1% (w/v) glycerol, 1.34% (w/v) yeast nitrogen base with ammonium sulfate but without amino acids, and 4 × 10−5% (w/v) biotin in 100 mM potassium phosphate buffer. The phosphate buffer was adjusted to pH 6.0. Buffered minimal methanol-complex medium (BMMY) was the same as BMGY, except that 0.5% (w/v) methanol was replaced with glycerol.
All primers used in the construction of the recombinant plasmid pMC are shown in Table 1; cleavage sites of restriction endonucleases are underlined. The schematic map of the construction of expression vector pMC is shown in Figure S1 (online resource) and can be briefly described as follows. A fragment with 7 multiple cloning sites was synthesized in vitro and cloned into pUC57-Kan-MCS-free, resulting in a pUC57-MCS7 plasmid. The HIS4 gene was amplified by PCR from pPIC3.5K using the primer pairs HIS4-A-F/R and HIS4-B-F/R and was integrated into the pUC57-MCS7 plasmid. The Xba I on HIS4 was synonymously mutated by overlap extension PCR. The fragment AOX1TT-PTEF1-PEM7-ZeocinR-CYC1TT-lox66 was amplified from pPICZαA using the primer pairs ATEZC-F/R and was integrated into the pUC57-MCS7 plasmid. The cre gene was amplified from pTSC using the primer pairs Cre-F/R and was integrated into the pUC57-MCS7 plasmid. The fragment lox71-PAOX1 was amplified from pPICZαA using the primer pairs AOX-F/R and was integrated into the pUC57-MCS7 plasmid. Finally, the recombinant plasmid was named pMC.
Overnight cultures of E. coli DH10B (pMC) were harvested for extraction of total RNA. Total RNA was extracted using a Bacteria total RNA Isolation Kit (Sangon Biotech, Shanghai, China) and treated with RNase-free DNase (Takara, Dalian, China) to eliminate any residual DNA. The total RNA was added to the PCR system (used as temple) to check if the total DNA was completely hydrolyzed. This PCR system was used as a negative control. The cDNA was obtained using a PrimeScript RT reagent kit (TaKaRa, Dalian, China), and 2 μl of 1:5-diluted cDNA samples was used as the template for reverse transcription-PCR. The existence of the cre gene in cDNA was identified by RT-PCR. The 16S rRNA gene was used as a positive control. Primers used in RT-PCR are shown in Table 2.
To inhibit the leakage expression of Cre recombinase in E. coli, an upstream ORF or lacO (operator gene) was integrated using the primer pairs AOX-F/R-uORF or AOX-F/R-lacO, respectively. The resulting recombinant plasmids were named pMCU and pMCO. The sites of Xho I, Not I, and Nco I on pMCO were deleted by overlap extension PCR, making them available in the heterogenous expression cassette or linearization site of HIS4. The fragments AOX1TT and PAOX1-αFactor were subsequently integrated into pMCO. The resulting recombinant plasmids were named pMCO-AOXα.
The sequence the of appA gene according to the literature (Dassa et al., 1990) was codon optimized and synthesized in vitro, then cloned into the pMCO-AOXα vector via EcoR I and Pst I. The resulting recombinant plasmid, named pMCO-AOXα-A1, was digested by Spe I and Xba I to generate a 2.7 kb expression cassette. Meanwhile, pMCO-AOXα-A1 was also linearized by Xba I. The two fragments were connected by T4 ligase to construct the plasmid pMCO-AOXα-A2; the multicopy recombinant vectors pMCO-AOXα-A4 and pMCO-AOXα-A8 were constructed in the same way.
The expression vectors pMCO-AOXα-An (n = 1, 2, 4, or 8) were linearized with Sal I and transformed into P. pastoris GS115 by electroporation. The charging voltage, resistance, and capacitance were 1,500 V, 250 Ω, and 25 μF, respectively. Positive transformants on YPDZ plates were shifted to new YPDZ plates with pre-marked serial numbers and incubated overnight at 30°C. The fresh clones were placed into 3 mL of BMGY liquid medium in test tubes and cultured at 30°C and 200 rpm for ~36 h until a stable saturation was attained; then, the cells were harvested by centrifugation and resuspended in 1 mL of BMMY. After incubation at 30°C and 200 rpm for 24 h, the enzyme assay was immediately performed.
Phytase activity was determined by the ferrous sulfate-molybdenum blue method (Huang et al., 2006). Briefly, 100 μl of 200-fold diluted enzyme solution was incubated with 900 μl of substrate solution (4 mM sodium phytate in 0.25 M sodium acetate buffer, pH 4.5) at 37°C for 10 min. The reaction was stopped by adding 1 mL of 10% (w/v) trichloroacetic acid (TCA). The amount of inorganic phosphate released was analyzed by adding 2 mL of a coloring reagent C [1% (w/v) ammonium molybdate, 3.2% (v/v) sulfuric-acid solution, and 7.2% (w/v) ferrous sulfate solution] and measuring the absorption at 700 nm. One unit (U) of phytase activity was defined as the amount of enzyme that releases 1 μmol phosphate in 1 min at 37°C.
In each group of recombinants with designed copy numbers (copy number = 1, 2, 4, or 8), the clone that exhibited the highest phytase activity was selected, and its genomic DNA was extracted using a Yeast Genomic DNA Isolation Kit (Sangon Biotech, Shanghai, China). GAPDH (PAS_chr2-1_0437), which is present as a single copy in the P. pastoris genome, was chosen as the reference gene. All primers used for real-time quantitative PCR (qPCR) are listed in Table 2. For each qPCR reaction mixture, 2 μl of 1:100 diluted genomic DNA samples was used as the template with 10 μl of SYBR Premix Ex Taq II (Takara, Dalian, China) in a total volume of 20 μl. The amplification reaction was performed according to the manufacturer's instructions. Each reaction was performed in triplicate. Relative quantification of the copy number of the target gene was performed according to the 2−ΔΔCt method (Livak and Schmittgen, 2001; Zarei et al., 2014), where ΔΔCt = ΔCt of target − ΔCt of calibrator, and ΔCt = Ct of target of calibrator − Ct of reference (GAPDH). A strain with a known copy number (one copy) of the target gene served as the calibrator strain.
The 24-h BMMY cultures were plate streaked onto YPD plates and incubated at 30°C until colonies were visible. Single clones were shifted to new YPD and YPDZ plates and incubated at 30°C overnight. The percentage of cells that lost the ZeocinR cassette after methanol induction was calculated by comparing colony numbers on YPD and YPDZ plates. Then, the phytase activity assay was performed as previously described to reconfirm the yield of phytase for colonies that were sensitive to Zeocin.
In the group of recombinants harboring 8 copies of the target gene, the clone that exhibited the highest phytase activity was induced in YPM liquid medium in test tube. The obliteration of resistance to Zeocin was performed as previously described. After reexamining the phytase activity of the clones that lost resistance to Zeocin, the linearized expression vectors pMCO-AOXα-A4 and pMCO-AOXα-A8 were transformed into markerless P. pastoris cells by electroporation, resulting in transformants with 12 or 16 copies of the target gene. The enzyme assay of positive transformants was determined in test tube-scale, and the copy number of the target gene was determined by qPCR as previously described.
In each group of recombinants harboring 1, 2, 4, 8, 12, or 16 copies of the target gene, the clone that exhibited the highest phytase activity was cultured and induced on the shake-flask scale for reexamination. Transformants were transferred to 30 mL of BMGY liquid medium in a 250 mL shake flask and were cultured ~48 h at 30°C and 200 rpm until a stable saturation was attained. Then, the cells were harvested by centrifugation, resuspended in 30 mL of BMMY and cultured at 30°C at 200 rpm for 96 h. A total of 150 μl of 100% methanol was added every 24 h to maintain induction, and 150 μl of culture was extracted at the same time for the enzyme assay. After 96 h of induction, culture supernatants were analyzed by SDS-PAGE.
A selection marker rescue vector pMC containing a lox71-Cre-ZeoR-lox66 cassette was designated for multicopy P. pastoris expression strain generation. The schematic map of the construction of pMC is shown in Figure S1. Briefly, pUC57-MCS7 was used as the original plasmid, and four DNA fragments were subsequently integrated into pUC57-MCS7. To verify the proper assembly of pMC, single-colony PCR was performed using the primer pair lox-F/R, indicated by arrows in Figure 1A. The fragment of Cre-AOX1TT-PTEF1-PEM7-ZeoR-CYC1TT-lox66 was ~2,800 bp, while the fragment plus lox71-PAOX1 was calculated as ~3,800 bp. Unexpectedly, a single DNA band of ~250 bp was clearly observed by gel electrophoresis analysis (Figure 1B), which indicated that the fragment of Cre-AOX1TT-PTEF1-PEM7-ZeoR-CYC1TT-lox66 confirmed the estimated molecular weight (2.8 kb). The smaller electrophoretic band suggests that the plasmid pMC recombined spontaneously.
Figure 1. Identification of the leakage expression of Cre recombinase. (A) The map of plasmid pMC. The primer pairs lox-F/R are indicated by arrows, and the calculated molecular weight of the two detected fragments is shown on top. (B) Single-colony PCR analysis of E. coli (pMC). Line M, DNA marker; Line 1, the detected fragment of Cre-AOX1TT-PTEF1-PEM7-ZeoR-CYC1TT-lox66; Line 2–7, the detected fragment of lox71-PAOX1-Cre-AOX1TT-PTEF1-PEM7-ZeoR-CYC1TT-lox66. (C) RT-PCR analysis of E. coli (pMC). Line M, DNA marker; Line 1, positive control of 16s rRNA gene; Line 2, cre gene; Line 3, negative control.
To further identify the leakage expression of the cre gene, the total RNA of E. coli DH10B (pMC) was extracted, and reverse transcription-PCR (RT-PCR) was performed. The negative control (Figure 1C, line 3) was not observed, demonstrating that the genome DNA and plasmid DNA were completely digested. The positive control (Figure 1C, line 1) and Cre recombinase (Figure 1C, line 2) were detected, indicating that the AOX1 promoter from P. pastoris could be partially recognized by E. coli and the downstream cre gene was transcribed. We deduced that the leakage expression of Cre recombinase caused the excision of lox71-PAOX1-Cre-AOX1TT-PTEF1-PEM7-ZeoR-CYC1TT-lox66, generating a lox72 site on the plasmid.
To overcome the leakage expression of Cre in E. coli, two cis elements were integrated between PAOX1 and the cre gene on the plasmid pMC (shown in Figure 2A). One is an upstream ORF (uORF), which contains eight AGA codons. The other is the lac operator gene (lacO) of the lactose operon. The integrations were performed using primer pairs AOX-F/AOX-R-uORF and AOX-F/AOX-R-lacO, respectively. The resulting recombinant plasmids were named pMCU and pMCO.
Figure 2. Prevention of Cre-mediated plasmid recombination. (A) Schematic map of the integration of uORF and lacO. The sequences of uORF and lacO are underlined; the downstream Xho I sites are in bold. (B) Single-colony PCR analysis. Line M, DNA marker; Line 1, the detected fragment of Cre-AOX1TT-PTEF1-PEM7-ZeoR-CYC1TT-lox66; Line 2, the detected fragment between lox71 and lox66 of pMCO; Line 3, the detected fragment between lox71 and lox66 of pMCU. (C) Gel electrophoresis analysis of extracted recombinant plasmids from E. coli. Line M, DNA marker; Line 1, pMCO; Line 2, pMCU. (D) RT-PCR analysis of E. coli (pMCO). Line M, DNA marker; Line 1, positive control of 16s rRNA gene; Line 2, cre gene.
To verify the proper assembly of pMCU and pMCO, colony PCR was performed using the primer pair lox-F/R. A 3.8 kb DNA band was observed for both plasmids (Figure 2B, lane 2–3), which matches the predicted size of the whole insertion fragment, indicating the proper integration of PAOX1-uORF and PAOX1-lacO. However, when these two recombinant plasmids were extracted, gel electrophoresis analysis showed that the size of the two plasmids was clearly different. The larger band (Figure 2C, lane 1, consistent with the theoretical molecular weight) showed the outstanding stability of pMCO, while the smaller band of pMCU (Figure 2C, lane 2) indicated that recombination between lox71 and lox66 mediated by Cre recombinase still occurred. The stability of pMCO was also verified by RT-PCR using the primer pairs 16s-RT-F/R and Cre-RT-F/R. The gel electrophoresis analysis showed that no cDNA of the cre gene was detected (Figure 2D, lane 2). These results showed that the lacO operator could effectively block the leakage expression of Cre recombinase promoted by the AOX1 promoter in E. coli.
pMCO was applied to construct the full functional expression vector for P. pastoris. First, to make Xho I, Not I, and Nco I available in the heterogenous expression cassette or linearization site of HIS4, these sites on pMCO were deleted by overlap extension PCR. Then, the fragments AOX1TT and PAOX1-αFactor were subsequently integrated into pMCO. The resulting recombinant plasmid was named pMCO-AOXα (Figure 3).
The expression vector pMCO-AOXα has the potential for commercial application: it can be linearized by Nco I, Sal I, and Nhe I on HIS4, and heterologous genes can be integrated into the expression cassette by utilizing the Xho I, EcoR I, Pst I, and Not I sites. Since Spe I and Xba I were introduced into pMCO-AOXα as isocaudamers, the expression cassette can be inserted in the unique Xba I site repetitively, generating a multicopy recombinant plasmid. Briefly speaking, suitable restriction endonuclease sites facilitate the insertion of target genes, the multimerization of expression cassettes and the integration of linearized recombinant plasmids.
Phytase is generally used as an animal feed supplement to enhance the nutritive value of plant material by liberation of inorganic phosphate from phytic acid. Phytase AppA was from E. coli and can catalyze the hydrolysis of phytic acid (myo-inositol hexakisphosphate). The optimized appA gene was synthesized and successfully cloned into pMCO-AOXα; the resulting vector carrying 1 copy of the appA gene was designated as pMCO-AOXα-A1. The multimerization was performed as described in the “Materials and methods,” producing the vectors pMCO-AOXα-A2, pMCO-AOXα-A4 and pMCO-AOXα-A8, which carried 2, 4, or 8 copies of the appA gene, respectively (Figure 4A). To confirm the correct orientation of the integration of expression cassette, which was inserted in the unique Xba I site, the recombinant vectors pMCO-AOXα-A1, pMCO-AOXα-A2, pMCO-AOXα-A4, and pMCO-AOXα-A8 were digested with Spe I and Xba I (Figure 4B). Gel electrophoresis analysis showed that the molecular weight of the inserted expression cassettes increased gradually, while the molecular weight of the vector frame remained ~10 kb (arrow, Figure 4B). The result verified that pMCO-AOXα-A1, pMCO-AOXα-A2, pMCO-AOXα-A4, and pMCO-AOXα-A8 were constructed correctly.
Figure 4. Scheme for construction and identification of the expression vectors with 1, 2, 4, or 8 copies of the appA gene. (A) Schematic map of the construction of expression vectors pMCO-AOXα-A1, pMCO-AOXα-A2, pMCO-AOXα-A4, and pMCO-AOXα-A8. (B) Gel electrophoresis analysis of recombinant vectors digested with Spe I and Xba I. Line M, DNA marker; Line 1, pMCO-AOXα-A1; Line 2, pMCO-AOXα-A2; Line 3, pMCO-AOXα-A4; Line 4, pMCO-AOXα-A8.
The plasmids containing 1, 2, 4, or 8 copies of appA were linearized and electroporated into P. pastoris. After selection of recombinants on YPDZ plates, 10 positive clones for GS115-pMCO-AOXα-An (n = 1, 2, 4, or 8) were picked (a total of 40 clones), initially cultured in BMGY, then transferred into BMMY to induce the expression of the target protein with methanol. The supernatants of 24-h BMMY cultures were collected, and the highest-yielding clones were screened by quantification of phytase activity.
In test tube culture, the transformants GS115-pMCO-AOXα-An-ZeocinR (n = 1, 2, 4, or 8) showed an increasing trend in the recombinant protein expression level, and the GS115-pMCO-AOXα-A8-ZeocinR obtained the highest phytase yield (Figure 5A). For each group of transformants GS115-pMCO-AOXα-An-ZeocinR (n = 1, 2, 4, or 8), the production of the strain exhibiting the highest yield of phytase from the 10 clones is listed in Table 3.
Figure 5. Identification and expression analysis of the transformants that were resistant or sensitive to Zeocin. (A) Expression analysis of the ZeocinR transformants. (B) Identification of the colonies that were sensitive toward Zeocin. (C) Single-colony PCR analysis of ZeocinS strains using primer pairs lox-F/αf-R. Line M, DNA marker; Line 1, ZeocinR transformants; Line 2-11, 10 randomly selected ZeocinS transformants. (D) Expression analysis of the ZeocinS transformants. The experiments were performed 10 or 20 times as described; the mean values ± SD are presented. Bars with the same letters are not significant (P < 0.05).
Since methanol could also induce the expression of Cre recombinase, the loss of Zeocin resistance gene was checked. The BMMY cultures of the clones denoted as GS115-pMCO-AOXα-A1-ZeocinR #1, GS115-pMCO-AOXα-A2-ZeocinR #7, GS115-pMCO-AOXα-A4-ZeocinR #5, and GS115-pMCO-AOXα-A8-ZeocinR #3 were streaked onto YPD plates, single colonies were transferred to new YPD and YPDZ plates, and the percentage of cells that lost the ZeocinR cassette was calculated (Figure 5B, 10 colonies for each ZeocinR transformant except 20 colonies for GS115-pMCO-AOXα-A8-ZeocinR #7). All clones were sensitive to Zeocin, indicating the excision of the resistance gene by Cre recombinase, which was verified by single-colony PCR (Figure 5C, and the schematic map of the deletion of Zeocin resistance gene was shown in Figure S2). Then, the phytase yield assay was performed for these 50 colonies (Figure 5D, in test tube-scale as well); the strain exhibiting the highest yield of phytase from each group of 10 or 20 clones is listed in Table 4. The yield of phytase and the copy number analysis are also shown in Table 4.
To obtain transformants with 12 or 16 copies of the appA gene, the vectors pMCO-AOXα-A4 or pMCO-AOXα-A8 were successfully transformed into GS115-pMCO-AOXα-A8-ZeocinS #17, and the resulting strains were denoted as GS115-pMCO-AOXα-A8A4-ZeocinR or GS115-pMCO-AOXα-A8A8-ZeocinR, respectively. The results showed that our marker rescue system worked well in P. pastoris. For each strain, 10 positive clones were cultured and induced; their phytase expression levels are shown in Figure 6A. In addition, the highest-yielding clones for GS115-pMCO-AOXα-A8A4-ZeocinR and GS115-pMCO-AOXα-A8A8-ZeocinR were screened (listed in Table 3). The highest phytase yield of the transformants harboring 12 copies of appA gene was 418.23 U/mL, which was 4.45 and 1.17 times higher than the yield of 1-copy transformants (93.97 U/mL) and 8-copy transformants (355.82 U/mL), respectively. However, the phytase yield of 16-copy transformants decreased to 369.88 U/mL, indicating that the abundant over-expression of proteins imposed a metabolic burden on the secretory pathway.
Figure 6. Expression analysis of the transformants that were resistant or sensitive to Zeocin. (A) Expression analysis of the ZeocinR transformants. (B) Expression analysis of the ZeocinS transformants. The experiments were performed 10 times as described; the mean values ± SD are presented. Bars with the same letters are not significant (P < 0.05).
Zeocin resistant genes of the P. pasrois transformants #7(12 copies) and #9(16 copies) were further deleted, the phytase yields of the 10 ZeocinS colonies were assayed (as shown in Figure 6B). The strains exhibiting the highest phytase yield from each group of 10 clones of GS115-pMCO-AOXα-A8A4-ZeocinS or GS115-pMCO-AOXα-A8A8-ZeocinS are listed in Table 4. The yield of phytase and the copy number analysis are also shown in Table 4.
After the generation and identification of a series of variant P. pastoris transformants harboring 1, 2, 4, 8, 12, or 16 copies of the appA gene, the shake-flask cultures were created to reconfirm the phytase yield for each transformant. Data shown in Figure 7 demonstrated that the recombinant phytase yield increased as the copy number of appA gene increased, and GS115-pMCO-AOXα-A8A4-ZeocinS #5 reached the highest expression level of 179.34 mg/L, based on specific activity of 3,100 U/mg protein (Huang et al., 2006). The phytase yield of GS115-pMCO-AOXα-A8A8-ZeocinS #7 decreased to 148.38 mg/L, probably because of an ERAD response to restore ER homeostasis.
Figure 7. Expression analysis of the ZeocinS transformants. (A) SDS-PAGE analysis. Line M: protein marker; Line 1: GS115-pMCO-AOXα-A1-ZeocinS #2; Line 2: GS115-pMCO-AOXα-A2-ZeocinS #3; Line 3: GS115-pMCO-AOXα-A4-ZeocinS #1; Line 4: GS115-pMCO-AOXα-A8-ZeocinS #17; Line 5: GS115-pMCO-AOXα-A8A4-ZeocinS #5; Line 6: GS115-pMCO-AOXα-A8A8-ZeocinS #7. (B) Production of phytase with different copy number of the appA gene. The experiments were performed 3 times; the mean values ± SD are presented. Bars with the same letters are not significant (P < 0.05).
P. pastoris has been widely used for the industrial production of recombination proteins because of its efficient protein secretion and high cell density cultivation. Several studies have reported that the gene copy number has a significant impact on heterogenous protein production, which is useful to achieve a high expression level of a target protein (Damasceno et al., 2012; Ahmad et al., 2014). PTVA method is the most commonly used protocol to generate multiple copy strains. High copy number strains were selected under high Zeocin concentration randomly (Aw and Polizzi, 2013). Rational design of overexpression strain with certain copy number of the target gene is difficult since the limited selection pressure offered by the host (Zhu et al., 2009). Therefore, the hypothesis that the deletion of marker genes in P. pastoris facilitates the repetitive integration of recombinant vector was investigated.
Two marker rescue (deletion) systems were compared, and the leakage expression of Cre recombinase in E. coli was examined. In the first marker rescue system (self-unexcisable method), the loxP-Marker-loxP fragment and the plasmid carrying Cre recombinase were transformed into P. pastoris successively (Gueldener et al., 2002). In the second marker rescue system (self-excisable method), the PCR fused lox71-Cre-ZeoR-lox66 fragment was transformed into P. pastoris by electroporation. Methanol then induced the expression of Cre recombinase, which excises the fragment of lox71-Cre-ZeoR-lox66, leaving a lox72 site in the P. pastoris genome (Pan et al., 2011). The self-excisable marker recycling system has the advantage of direct and easy operation, but still has the bottleneck of leakage expression of Cre recombinase, identified by single-colony PCR (Figure 1B) and RT-PCR (Figure 1C). The mechanism for spontaneous plasmid recombination may be that E. coli can recognize the AOX1 promoter and initiate the expression of Cre recombinase. Although the transcription level of Cre recombinase might be very low, the trace amount of recombinase could still cause recombination between lox71 and lox66.
The introduction of an intron in the cre gene has been shown to effectively block the leakage expression of Cre recombinase in E. coli because E. coli lacks the ability to process introns in mRNA (Agaphonov and Alexandrov, 2014). The introduced intron is from the COF1 gene of H. polymorpha. Once the intron is replaced by another eukaryotic intron (such as the intron of the xynG2 gene from Aspergillus oryzae), this method can be easily adapted for other fungal species (Zhang et al., 2016). In our work, lacO was inserted between PAOX1 and the cre gene. The operator gene lacO was bound with repressor protein LacI in E. coli; therefore, the downstream gene expression was blocked. In P. pastoris, lacO cannot be recognized because of the absence of LacI, so the downstream cre gene can be induced normally. This was demonstrated by both single-colony PCR and RT-PCR (Figure 2). Compared with the intron method, the lacO method can work in any fungal cell that does not harbor the repressor protein LacI, theoretically. The lacO method averts the screening of the intron sequences that are indispensable to be genetically manipulated, which might be laborious and time-consuming. The recombinant vector pMCO-AOXα containing the complete expression cassette for a heterogenous gene could be universally employed in the construction of a multicopy integration strain in P. pastoris by rescuing the selection marker with Cre combinase and has the potential for commercial application.
In this study, a series of P. pastoris transformants with 1, 2, 4, 8, 12, or 16 copies of the appA gene were generated. The 1-, 2-, 4-, and 8-copy transformants were constructed by transformation of pMCO-AOXα-An (n = 1, 2, 4, or 8) into GS115, followed by deleting the Cre-ZeoR cassette. The 12- and 16-copy transformants were constructed by transformation of pMCO-AOXα-An (n = 4 or 8) into GS115-pMCO-AOXα-A8-ZeocinS #17; the resulting ZeocinR clones were also treated with methanol to generate a ZeocinS strain. The ZeocinS strain can easily absorb other linearized recombinant vectors and integrate them into its genome. The qPCR analysis and the phytase yield assay showed a positive correlation between gene copy number and the recombinant protein expression level; GS115-pMCO-AOXα-A8A4-ZeocinS obtained the highest phytase yield (shown in Table 4, Figure 7). However, the phytase yield of 16-copy transformants decreased. We deduced that abundantly over-expressed protein might remain unfolded and activate the unfolded protein response (UPR) leading to endoplasmic reticulum-associated degradation (ERAD) (Malhotra and Kaufman, 2007; Vembar and Brodsky, 2008; Aw and Polizzi, 2013; Puxbaum et al., 2015).
The promoter region of Spe I-PAOX1-αfactor-EcoR I and the recombination site Xba I-HIS4-Bgl II of pMCO-AOXα can be easily changed and optimized. For instance, the recently discovered promoters such as G6 (Prielhofer et al., 2013), GCW14 (Liang et al., 2013), and THI11 (Stadlmayr et al., 2010), with their own characteristics, can be inserted into pMCO easily via Spe I and EcoR I sites. Another case is that the HIS4 gene can be replaced with other DNA fragments such as non-transcribed intergenic spacer (NTS) of the rDNA repeat locus (Marx et al., 2009), or other genes denoted as “non-essential protein of unknown function” (PAS_CHR1-1_0141, PAS_CHR1-3_0180, PAS_CHR1-3_0216 and PAS_CHR4_0177). Multiple integration of vectors into P. pastoris in a head-to-tail orientation can raise the possibility of excision of the homologous region (loop out region) (Aw and Polizzi, 2013). pMCO-AOXα can be used in the dispersed integration of the target gene by substituting the HIS4 recombination site with various homologous recombination fragments to reduce the potential genetic instability of the recombinant strain in P. pastoris.
DL performed all the experiments, coordinated the data analysis, and prepared the manuscript. BZ, SL, and JZ contributed in the preparation of the manuscript and data analysis. HC and YH provided the research work suggestion. ZC supervised the whole study.
The authors declare that the research was conducted in the absence of any commercial or financial relationships that could be construed as a potential conflict of interest.
The reviewer XZ and handling Editor declared their shared affiliation.
This work was supported by grants from the National Natural Science Foundation of China (No. 31570059 and No. 31400056), the Natural Science Foundation of Jiangsu Province (No. BK20140687).
The Supplementary Material for this article can be found online at: http://journal.frontiersin.org/article/10.3389/fmicb.2017.01698/full#supplementary-material
Figure S1. Schematic map of the construction of expression vector pMC.
Figure S2. Schematic map of the excision of the resistance gene.
Agaphonov, M., and Alexandrov, A. (2014). Self-excising integrative yeast plasmid vectors containing an intronated recombinase gene. FEMS Yeast Res. 14, 1048–1054. doi: 10.1111/1567-1364.12197
Ahmad, M., Hirz, M., Pichler, H., and Schwab, H. (2014). Protein expression in Pichia pastoris: recent achievements and perspectives for heterologous protein production. Appl. Microbiol. Biotechnol. 98, 5301–5317. doi: 10.1007/s00253-014-5732-5
Aw, R., and Polizzi, K. M. (2013). Can too many copies spoil the broth? Microb. Cell Fact. 12, 128–128. doi: 10.1186/1475-2859-12-128
Çalık, P., Ata, Ö., Güneş, H., Massahi, A., Boy, E., Keskin, A., et al. (2015). Recombinant protein production in Pichia pastoris under glyceraldehyde-3-phosphate dehydrogenase promoter: from carbon source metabolism to bioreactor operation parameters. Biochem. Eng. J. 95, 20–36. doi: 10.1016/j.bej.2014.12.003
Damasceno, L. M., Huang, C. J., and Batt, C. A. (2012). Protein secretion in Pichia pastoris and advances in protein production. Appl. Microbiol. Biotechnol. 93, 31–39. doi: 10.1007/s00253-011-3654-z
Dassa, J., Marck, C., and Boquet, P. L. (1990). The complete nucleotide sequence of the Escherichia coli gene appA reveals significant homology between pH 2.5 acid phosphatase and glucose-1-phosphatase. J. Bacteriol. 172, 5497–5500. doi: 10.1128/jb.172.9.5497-5500.1990
Gueldener, U., Heinisch, J., Koehler, G. J., Voss, D., and Hegemann, J. H. (2002). A second set of loxP marker cassettes for Cre-mediated multiple gene knockouts in budding yeast. Nucleic Acids Res. 30:e23. doi: 10.1093/nar/30.6.e23
Huang, H., Luo, H., Yang, P., Meng, K., Wang, Y., Yuan, T., et al. (2006). A novel phytase with preferable characteristics from Yersinia intermedia. Biochem. Biophys. Res. Commun. 350, 884–889. doi: 10.1016/j.bbrc.2006.09.118
Leibig, M., Krismer, B., Kolb, M., Friede, A., Götz, F., and Bertram, R. (2008). Marker removal in staphylococci via Cre recombinase and different lox sites. Appl. Environ. Microbiol. 74, 1316–1323. doi: 10.1128/AEM.02424-07
Li, C., Lin, Y., Zheng, X., Pang, N., Liao, X., Liu, X., et al. (2015). Combined strategies for improving expression of Citrobacter amalonaticus phytase in Pichia pastoris. BMC Biotechnol. 15:88. doi: 10.1186/s12896-015-0204-2
Liang, S., Zou, C., Ying, L., Zhang, X., and Ye, Y. (2013). Identification and characterization of P GCW14: a novel, strong constitutive promoter of Pichia pastoris. Biotechnol. Lett. 35, 1865–1871. doi: 10.1007/s10529-013-1265-8
Lincereghino, J., and Lincereghino, G. P. (2007). Vectors and strains for expression. Methods Mol. Biol. 389, 11–26. doi: 10.1007/978-1-59745-456-8_2
Liu, S. H., Chou, W. I., Sheu, C. C., and Chang, M. D. (2005). Improved secretory production of glucoamylase in Pichia pastoris by combination of genetic manipulations. Biochem. Biophys. Res. Commun. 326, 817–824. doi: 10.1016/j.bbrc.2004.11.112
Livak, K. J., and Schmittgen, T. D. (2001). Analysis of relative gene expression data using real-time quantitative PCR and the 2(-Delta Delta C(T)) Method. Methods 25, 402–408. doi: 10.1006/meth.2001.1262
Malhotra, J. D., and Kaufman, R. J. (2007). The endoplasmic reticulum and the unfolded protein response. Semin. Cell Dev. Biol. 18, 716–731. doi: 10.1016/j.semcdb.2007.09.003
Marx, H., Mecklenbräuker, A., Gasser, B., Sauer, M., and Mattanovich, D. (2009). Directed gene copy number amplification in Pichia pastoris by vector integration into the ribosomal DNA locus. FEMS Yeast Res. 9, 1260–1270. doi: 10.1111/j.1567-1364.2009.00561.x
Mellitzer, A., Weis, R., Glieder, A., and Flicker, K. (2012). Expression of lignocellulolytic enzymes in Pichia pastoris. Microb. Cell Fact. 11, 172–183. doi: 10.1186/1475-2859-11-61
Pan, R., Zhang, J., Shen, W.-L., Tao, Z.-Q., Li, S.-P., and Yan, X. (2011). Sequential deletion of Pichia pastoris genes by a self-excisable cassette. FEMS Yeast Res. 11, 292–298. doi: 10.1111/j.1567-1364.2011.00716.x
Parashar, D., and Satyanarayana, T. (2016). Enhancing the production of recombinant acidic α-amylase and phytase in Pichia pastoris under dual promoters [constitutive (GAP) and inducible (AOX)] in mixed fed batch high cell density cultivation. Process Biochem. 51, 1315–1322. doi: 10.1016/j.procbio.2016.07.027
Prielhofer, R., Maurer, M., Klein, J., Wenger, J., Kiziak, C., Gasser, B., et al. (2013). Induction without methanol: novel regulated promoters enable high-level expression in Pichia pastoris. Microb. Cell Fact. 12:5. doi: 10.1186/1475-2859-12-5
Puxbaum, V., Mattanovich, D., and Gasser, B. (2015). Quo vadis? The challenges of recombinant protein folding and secretion in Pichia pastoris. Appl. Microbiol. Biotechnol. 99, 2925–2938. doi: 10.1007/s00253-015-6470-z
Sauer, B. (1987). Functional expression of the cre-lox site-specific recombination system in the yeast Saccharomyces cerevisiae. Mol. Cell. Biol. 7, 2087–2096. doi: 10.1128/MCB.7.6.2087
Spohner, S. C., Müller, H., Quitmann, H., and Czermak, P. (2015). Expression of enzymes for the usage in food and feed industry with Pichia pastoris. J. Biotechnol. 202, 118–134. doi: 10.1016/j.jbiotec.2015.01.027
Stadlmayr, G., Mecklenbräuker, A., Rothmüller, M., Maurer, M., Sauer, M., Mattanovich, D., et al. (2010). Identification and characterisation of novel Pichia pastoris promoters for heterologous protein production. J. Biotechnol. 150, 519–529. doi: 10.1016/j.jbiotec.2010.09.957
Tuntufye, H. N., and Goddeeris, B. M. (2011). Use of lambda Red-mediated recombineering and Cre/lox for generation of markerless chromosomal deletions in avian pathogenic Escherichia coli. FEMS Microbiol. Lett. 325, 140–147. doi: 10.1111/j.1574-6968.2011.02421.x
Vembar, S. S., and Brodsky, J. L. (2008). One step at a time: endoplasmic reticulum-associated degradation. Nat. Rev. Mol. Cell Biol. 9, 944–957. doi: 10.1038/nrm2546
Weng, L., Biswas, I., and Morrison, D. A. (2009). A self-deleting Cre–lox–ermAM cassette, Cheshire, for marker-less gene deletion in Streptococcus pneumoniae. J. Microbiol. Methods 79, 353–357. doi: 10.1016/j.mimet.2009.10.007
Yan, X., Yu, H. J., Hong, Q., and Li, S. P. (2008). Cre/lox System and PCR-based genome engineering in Bacillus subtilis. Appl. Environ. Microbiol. 74, 5556–5562. doi: 10.1128/AEM.01156-08
Yang, J., Lu, Z., Chen, J., Chu, P., Cheng, Q., Jie, L., et al. (2016). Effect of cooperation of chaperones and gene dosage on the expression of porcine PGLYRP-1 in Pichia pastoris. Appl. Microbiol. Biotechnol. 100, 5453–5465. doi: 10.1007/s00253-016-7372-4
Yu, P., Yan, Y., Gu, Q., and Wang, X. (2013). Codon optimisation improves the expression of Trichoderma viride sp. endochitinase in Pichia pastoris. Sci. Rep. 3:3043. doi: 10.1038/srep03043
Zarei, N., Vaziri, B., Shokrgozar, M. A., Mahdian, R., Fazel, R., and Khalaj, V. (2014). High efficient expression of a functional humanized single-chain variable fragment (scFv) antibody against CD22 in Pichia pastoris. Appl. Microbiol. Biotechnol. 98, 10023–10039. doi: 10.1007/s00253-014-6071-2
Zhang, S., Ban, A., Ebara, N., Mizutani, O., Tanaka, M., Shintani, T., et al. (2016). Self-excising Cre/mutant lox marker recycling system for multiple gene integrations and consecutive gene deletions in Aspergillus oryzae. J. Biosci. Bioeng. 123, 403–411. doi: 10.1016/j.jbiosc.2016.11.001.
Keywords: markerless genetic manipulation, novel expression vector, dosage effect, phytase appA, Pichia pastoris expression system
Citation: Li D, Zhang B, Li S, Zhou J, Cao H, Huang Y and Cui Z (2017) A Novel Vector for Construction of Markerless Multicopy Overexpression Transformants in Pichia pastoris. Front. Microbiol. 8:1698. doi: 10.3389/fmicb.2017.01698
Received: 28 February 2017; Accepted: 23 August 2017;
Published: 11 September 2017.
Edited by:
Ning-Yi Zhou, Shanghai Jiao Tong University, ChinaReviewed by:
Jun-Jie Zhang, Indiana University School of Medicine, United StatesCopyright © 2017 Li, Zhang, Li, Zhou, Cao, Huang and Cui. This is an open-access article distributed under the terms of the Creative Commons Attribution License (CC BY). The use, distribution or reproduction in other forums is permitted, provided the original author(s) or licensor are credited and that the original publication in this journal is cited, in accordance with accepted academic practice. No use, distribution or reproduction is permitted which does not comply with these terms.
*Correspondence: Zhongli Cui, Y3psQG5qYXUuZWR1LmNu
Disclaimer: All claims expressed in this article are solely those of the authors and do not necessarily represent those of their affiliated organizations, or those of the publisher, the editors and the reviewers. Any product that may be evaluated in this article or claim that may be made by its manufacturer is not guaranteed or endorsed by the publisher.
Research integrity at Frontiers
Learn more about the work of our research integrity team to safeguard the quality of each article we publish.