- 1Division of Microbial Ecology, Department of Microbiology and Ecosystem Science, Research Network ‘Chemistry meets Microbiology’, University of Vienna, Vienna, Austria
- 2Department of Microbiology, Institute for Water and Wetland Research (IWWR), Radboud University, Nijmegen, Netherlands
Nitrification, the oxidation of ammonia via nitrite to nitrate, has always been considered to be catalyzed by the concerted activity of ammonia- and nitrite-oxidizing microorganisms. Only recently, complete ammonia oxidizers (“comammox”), which oxidize ammonia to nitrate on their own, were identified in the bacterial genus Nitrospira, previously assumed to contain only canonical nitrite oxidizers. Nitrospira are widespread in nature, but for assessments of the distribution and functional importance of comammox Nitrospira in ecosystems, cultivation-independent tools to distinguish comammox from strictly nitrite-oxidizing Nitrospira are required. Here we developed new PCR primer sets that specifically target the amoA genes coding for subunit A of the distinct ammonia monooxygenase of comammox Nitrospira. While existing primers capture only a fraction of the known comammox amoA diversity, the new primer sets cover as much as 95% of the comammox amoA clade A and 92% of the clade B sequences in a reference database containing 326 comammox amoA genes with sequence information at the primer binding sites. Application of the primers to 13 samples from engineered systems (a groundwater well, drinking water treatment and wastewater treatment plants) and other habitats (rice paddy and forest soils, rice rhizosphere, brackish lake sediment and freshwater biofilm) detected comammox Nitrospira in all samples and revealed a considerable diversity of comammox in most habitats. Excellent primer specificity for comammox amoA was achieved by avoiding the use of highly degenerate primer preparations and by using equimolar mixtures of oligonucleotides that match existing comammox amoA genes. Quantitative PCR with these equimolar primer mixtures was highly sensitive and specific, and enabled the efficient quantification of clade A and clade B comammox amoA gene copy numbers in environmental samples. The measured relative abundances of comammox Nitrospira, compared to canonical ammonia oxidizers, were highly variable across environments. The new comammox amoA-targeted primers enable more encompassing future studies of nitrifying microorganisms in diverse habitats. For example, they may be used to monitor the population dynamics of uncultured comammox organisms under changing environmental conditions and in response to altered treatments in engineered and agricultural ecosystems.
Introduction
Nitrification is an essential process of the global biogeochemical nitrogen cycle and plays a pivotal role in biological wastewater treatment and in drinking water production. The recent discovery of the first complete ammonia oxidizers (“comammox”) in the bacterial genus Nitrospira (Daims et al., 2015; van Kessel et al., 2015) was highly unexpected since Nitrospira were always regarded as canonical nitrite-oxidizing bacteria (NOB) (Watson et al., 1986; Ehrich et al., 1995; Spieck et al., 2006; Lebedeva et al., 2008, 2011). This discovery has raised a number of important questions, such as how often comammox Nitrospira occur in nitrifying microbial communities, and how relevant they are for nitrification compared to ammonia-oxidizing bacteria (AOB), archaea (AOA), and NOB.
Members of the genus Nitrospira have been assigned to at least six sublineages (Daims et al., 2001; Lebedeva et al., 2008, 2011). They are widespread in virtually all oxic natural and engineered ecosystems, and an impressively high diversity of coexisting uncultured Nitrospira strains has been detected in wastewater treatment plants and soils (Pester et al., 2014; Gruber-Dorninger et al., 2015). All known comammox organisms belong to Nitrospira sublineage II (Daims et al., 2015; van Kessel et al., 2015; Palomo et al., 2016; Pinto et al., 2016). This sublineage contains also canonical NOB, which lack the genes for ammonia oxidation (Daims et al., 2001; Koch et al., 2015). Moreover, the known comammox Nitrospira do not form a monophyletic clade within Nitrospira lineage II in phylogenies based on 16S rRNA genes or nxrB, the gene encoding subunit beta of the functional key enzyme nitrite oxidoreductase. Instead, they intersperse with the strict NOB in these phylogenetic trees (Daims et al., 2015; van Kessel et al., 2015; Pinto et al., 2016). Finally, it remains unknown whether other Nitrospira sublineages (Daims et al., 2001; Lebedeva et al., 2008, 2011) also contain comammox members. Consequently, it is impossible to infer from 16S rRNA or nxrB gene phylogenies whether yet uncharacterized Nitrospira bacteria are comammox or strict NOB, although such attempts have been published (Gonzalez-Martinez et al., 2016).
Intriguingly, comammox Nitrospira possess novel types of ammonia monooxygenase (AMO) and hydroxylamine dehydrogenase (HAO), the key enzymes of aerobic ammonia oxidation. The comammox AMO is phylogenetically distinct from the AMO forms of canonical AOB and AOA (Daims et al., 2015; van Kessel et al., 2015). The amoA gene encoding AMO subunit A has become a widely used functional and phylogenetic marker gene for bacterial and archaeal ammonia oxidizers (Rotthauwe et al., 1997; Purkhold et al., 2000; Junier et al., 2008; Pester et al., 2012). Public database mining for sequences related to the unique comammox amoA revealed the presence of putative comammox organisms in various environments including soils (paddy rice soils, other agricultural soils, forest soils, grassland soils), freshwater habitats (wetlands, rivers, lakes, groundwater basins), groundwater wells (GGWs), full-scale wastewater treatment plants (WWTPs), and drinking water treatment plants (DWTPs) (Daims et al., 2015; van Kessel et al., 2015). While this provides strong indications of a broad habitat range of comammox organisms, current knowledge about the environmental distribution and abundance of comammox Nitrospira is very limited and needs to be explored.
The amoA genes of comammox Nitrospira form two monophyletic sister clades (Daims et al., 2015; van Kessel et al., 2015), which are referred to as clade A and clade B. Clade A also contains some genes that were previously assigned to the methanotroph Crenothrix polyspora (Stoecker et al., 2006), but this assignment has recently been corrected (Oswald et al., 2017). Established PCR primer sets specifically targeting the amoA genes of AOB or AOA (Sinigalliano et al., 1995; Rotthauwe et al., 1997; Juretschko et al., 1998; Stephen et al., 1999; Nold et al., 2001; Norton et al., 2002; Webster et al., 2002; Okano et al., 2004; Francis et al., 2005; Treusch et al., 2005; Mincer et al., 2007; Junier et al., 2008; Meinhardt et al., 2015) do not amplify any clade A and clade B comammox amoA. Co-amplification of some comammox amoA genes occurs with a primer set targeting both betaproteobacterial amoA and the A subunit of the particulate methane monooxygenase (pmoA of pMMO; Holmes et al., 1995; Luesken et al., 2011). These primers, however, only target a fraction of the known comammox amoA genes. A recently established two-step PCR protocol (Wang et al., 2016) relies on the forward primer published by Holmes et al. (1995) and a new, highly degenerate reverse primer. It detects the bacterial copper-containing monooxygenase (CuMMO) genes including pmoA, betaproteobacterial amoA, and at least the comammox amoA genes that are amplified by the Holmes forward primer. However, because of its broad coverage of the CuMMOs, this primer pair does not allow a specific detection or quantification by quantitative PCR (qPCR) of comammox amoA genes. The two sets of comammox amoA targeted (q)PCR primers recently published by Bartelme et al. (2017) are, in contrast, highly specific. These primers only detect a small fraction of available comammox amoA gene sequences within clade A, and cannot be used to amplify comammox clade B amoA genes.
To enable the direct detection and quantification of comammox amoA genes in environmental samples, we designed in this study two new amoA-targeted primer sets specific for clade A or clade B comammox amoA genes, respectively. Subsequently, we applied these new primers to efficiently screen various habitats for the presence of comammox organisms and to rapidly quantify the abundances of comammox in selected samples.
Materials and Methods
Database Mining and Sequence Collection
The amino acid sequences of bacterial AmoA and PmoA were extracted from publicly available metagenomic datasets stored in the Integrated Microbial Genomes databases (IMG-ER and -MER) using the functional profiler tool against a specific bacterial AmoA/PmoA pfam (PF02461). For characterization of comammox AmoA, betaproteobacterial AmoA, and PmoA, sequences collected from the Integrated Microbial Genomes databases were augmented with nearly full length amino acid sequences collected from the Pfam site and from NCBI Genbank as described in Daims et al. (2015). Collected sequences were filtered against a hidden Markov model (hmm) using hmmsearch (http://hmmer.janelia.org/) with the AmoA/PmoA hmm (PF02461) requiring an expect value of <0.0001, and clustered at 90% identity using USEARCH (Edgar, 2010). Cluster centroids were aligned using Mafft (Katoh et al., 2002) and phylogenetic affiliation with comammox AmoA or betaproteobacterial AmoA was verified with phylogenetic trees calculated using FastTree (Price et al., 2009). Cluster centroids were expanded and reclustered at 99% identity with USEARCH and the phylogenetic affiliation with comammox and/or betaproteobacterial AmoA was re-assessed using FastTree.
Phylogenetic Analyses
For amino acid sequences that clustered with comammox AmoA and a selection of outgroup sequences affiliating with bacterial AmoA and PmoA, the corresponding nucleic acid sequences were recovered and aligned according to their amino acid translations using MUSCLE (Edgar, 2004). These nucleotide sequence alignments were manually corrected for frameshifts and used for calculating maximum likelihood trees with RaxmlHPC (Stamatakis et al., 2005) using the GTRCAT approximation of rate heterogeneity and 1,000 bootstrap iterations. The generated reference tree was imported into the ARB software package (Ludwig et al., 2004) and used as reference tree for the subsequent addition of short and nearly full-length comammox amoA, betaproteobacterial amoA and pmoA nucleotide sequences that were recovered from public databases. These sequences were added to the reference tree without changes of the overall tree topology by using the “parsimony interactive” tool of ARB. An ARB database including the reference tree is available for download at http://www.microbial-ecology.net/download.
Primer Design and PCR
Two degenerate PCR primer pairs (Table 1) targeting clade A or clade B comammox amoA genes, respectively, were designed and evaluated using the amoA/pmoA reference sequence dataset and the “probe design” and “probe match” functions of ARB (Ludwig et al., 2004). The oligonucleotide primers were obtained from Biomers (Ulm, Germany).
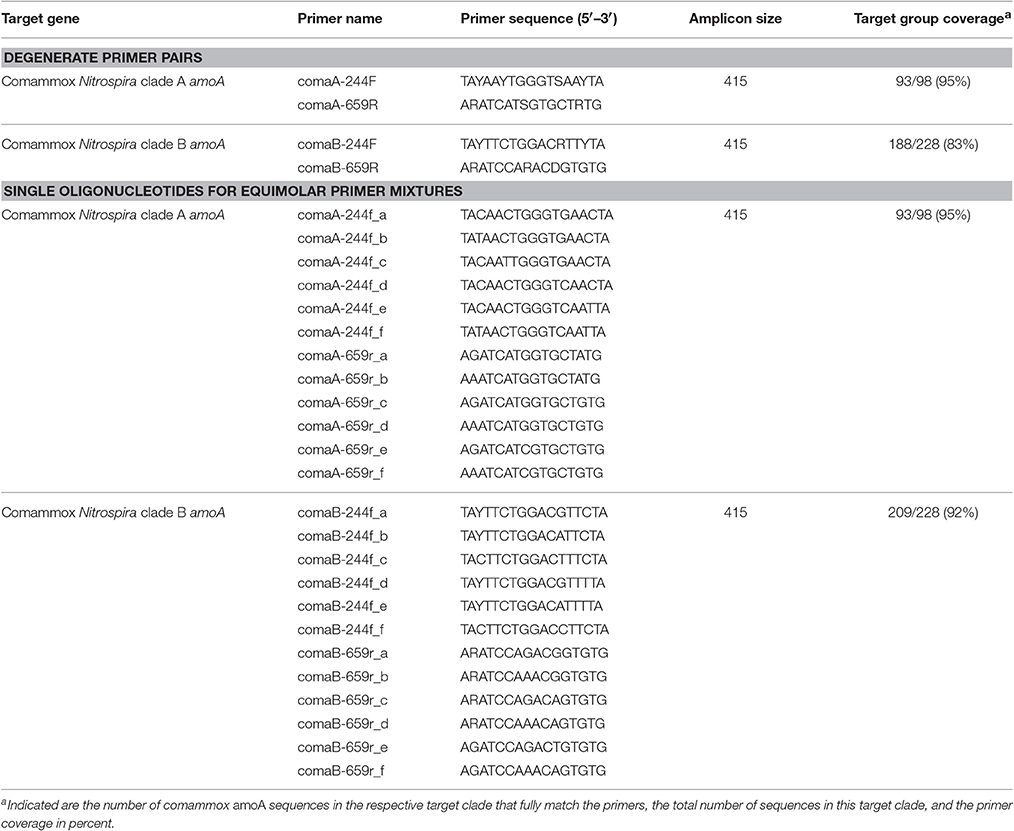
Table 1. Comammox amoA-targeted primers designed in this study, and in silico evaluation of the primer coverage of the target clades in our comammox amoA reference sequence database.
The optimal annealing temperature for the primers was determined by temperature gradient PCR using total genomic DNA extracted by a phenol-chloroform based method (details below) from a GWW sample (Wolfenbüttel, Germany), which contained both clade A and clade B comammox Nitrospira (Daims et al., 2015). An annealing temperature range from 42 to 52°C was chosen for experimental evaluation based on the theoretical melting temperature of 48°C of the designed primers (http://biotools.nubic.northwestern.edu/OligoCalc.html). Thermal cycling was carried out with an initial denaturation step at 94°C for 5 min, which was followed by 25 cycles of denaturation at 94°C for 30 s, primer annealing at 42–52°C for 45 s, and elongation at 72°C for 1 min. Cycling was completed by a final elongation step at 72°C for 10 min. The optimal annealing temperature for both primer pairs was found to be 52°C. At lower annealing temperatures, unspecific amplification of DNA fragments shorter than the expected amplicon length (415 bp) was observed. Since the amplification efficiency of correctly sized amplicons was lower at 52°C than at 48–50°C, temperatures above 52°C were not evaluated. The PCR reactions were performed in a DreamTaq Green PCR mix with 1 × Dream Taq Green Buffer containing 2 mM MgCl2, 0.025 U DreamTaq DNA polymerase, 0.2 mM dNTPs, 0.5 μM primers and 0.1 mg/mL bovine serum albumin (Fermentas, Thermo Fischer Scientific, Waltham, MA, USA).
Screening of Environmental Samples for Comammox amoA
Total nucleic acids were extracted from the environmental samples (Table 2) by using (i) the Fast DNA SPIN Kit for soil (MP Biomedicals, Santa Ana, CA, USA), (ii) the PowerSoil DNA Isolation Kit (MO BIO Laboratories, Carlsbad, CA, USA), or (iii) a phenol-chloroform based total nucleic acid extraction protocol described by Angel et al. (2012). PCRs were performed with either the DreamTaq Green PCR mix as described above, or with the PerfeCTa SYBR green FastMix PCR premix containing 1.5 mM of MgCl2 (Quanta Bioscience, Beverly, MA, USA) and 0.1 μM primers. Amplicons of the expected length (415 bp) were purified by using the QIAquick PCR purification Kit (Qiagen, Hilden, Germany). Alternatively, the QIAquick Gel extraction Kit (Qiagen, Hilden, Germany) was used to purify PCR products of the correct length from agarose gels if additional, unspecific amplification products of different lengths were present. The purified PCR products were cloned in E. coli with the TOPO-TA cloning kit (Invitrogen, Karlsruhe, Germany) or the pGEM-T Easy Vector System (Promega, Mannheim, Germany) by following the manufacturer's instructions. PCR products obtained from cloned vector inserts from the TOPO-TA kit were Sanger-sequenced with the M13F primer at Microsynth (Balgach, Switzerland). For clones generated with the pGEM-TEasy Vector SysteM, plasmids were extracted with the GeneJET plasmid miniprep kit (Thermo Fischer Scientific, Waltham, MA, USA) and the inserts were Sanger-sequenced with the M13F primer at Baseclear (Leiden, the Netherlands). The recovered sequences were quality checked and vector trimmed using the Sequencher version 4.6.1 (GeneCodes Corporation, Ann Arbor, MI, USA) or the Chromas version 2.6.1 (Technelysium Pty Ltd, Brisbane, Australia) software packages, and were added as described above to the amoA/pmoA nucleotide sequence reference tree in ARB. All obtained sequences were deposited at NCBI GenBank and are available under nucleotide accession numbers MF346936-MF347377.
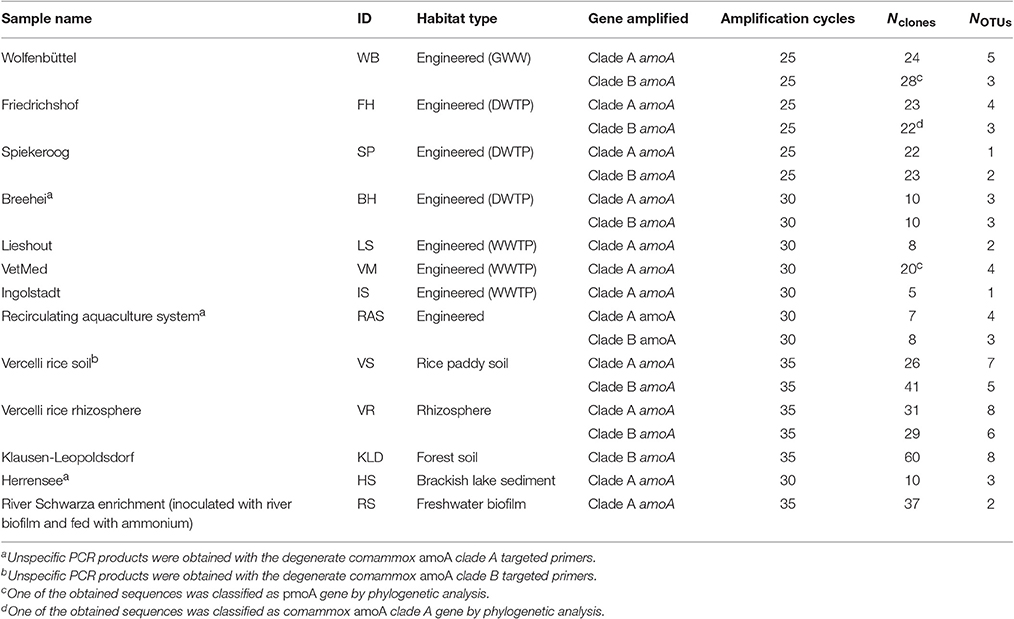
Table 2. Environmental samples analyzed in this study, PCR amplification cycles, and numbers of cloned comammox amoA sequences and OTUs retrieved from each sample.
Quantitative PCR
Quantification of comammox amoA genes was performed with individually prepared equimolar primer mixes for comammox amoA clade A and clade B genes (Table 1) on a selection of samples from different habitats (Table S2). Amplification of comammox amoA genes was performed with 3 min initial denaturation at 95°C, followed by 45 cycles of 30 s at 95°C, 45 s at 52°C, and 1 min at 72°C. Fluorescence was measured at 72°C for amplicon quantification. After amplification, an amplicon melting curve was recorded in 0.5°C steps between 38° and 96°C. Amplification of archaeal and betaproteobacterial amoA genes was performed with the GenAOA and the RottAOB primers as described by Meinhardt et al. (2015). All qPCR assays were performed on a Bio-Rad C1000 CFX96 Real-Time PCR system (Bio-Rad, Hercules, CA, USA) in Bio-Rad iQ SYBR Green Supermix (Bio-Rad, Hercules, CA, USA), containing 50 U/ml iTaq DNA polymerase, 0.4 mM dNTPs, 100 mM KCl, 40 mM Tris-HCl, 6 mM MgCl2, 20 mM fluorescein, and stabilizers. The respective equimolar comammox amoA primer mixtures (Table 1), or archaeal or bacterial amoA primers were added to a final concentration of 0.5 μM. Environmental DNA samples were added to each PCR reaction to a final volume of 20 μl reaction mix. Different template DNA dilutions (10x to 10,000x) resulting in DNA concentrations from 0.004 to 24 ng were applied to minimize possible PCR inhibition caused by excess DNA or co-extracted substances. With all samples, the two highest dilutions that yielded consistent results were used to calculate gene abundances. To furher confirm that PCR inhibition was not affecting qPCR results, 1:200 and 1:2,000 diluted DNA extracts from the GWW Wolfenbüttel sample were mixed with known concentrations of comammox clade A and clade B amoA gene standards and qPCR was carried out using the clade A or clade B amoA-targeted primers. The determined amplification efficiencies for the added standards were ~94%, demonstrating that dilution of the DNA extracts eliminated the PCR inhibition that had previously been observed with undiluted and 1:10 diluted extracts.
All assays were performed in triplicate for each dilution. For each assay, triplicate standard series were generated by tenfold serial dilutions (101–108 gene copies/μl) from purified M13-PCR products obtained from cloned vector inserts generated with the TOPO-TA cloning kit as described above. Clones of comammox amoA genes used for standard curve generation originated from this study, while the betaproteobacterial or archaeal amoA genes for the respective standard curves were obtained from pure cultures of Nitrosomonas nitrosa Nm90 and Nitrososphera gargensis, respectively. The correlation coefficient (r2) for each of the external standard curves was ≥0.98. The amplification efficiency for comammox amoA clade A and clade B genes (standard curves and environmental samples) was 88.5 and 87.0%, respectively. Amplification efficiency for archaeal amoA genes was 88.7, and 92.3% for betaproteobacterial amoA genes.
Results and Discussion
Design and Evaluation of Comammox amoA-Specific PCR Primers
A principal goal of this study was to develop a specific amoA-targeted PCR assay for the efficient and cultivation-independent detection and quantification of comammox Nitrospira in environmental samples. Mining of public databases retrieved only a small number (376) of sequences related to clade A or clade B comammox amoA, and only 32 of these sequences cover almost the full length of the comammox amoA genes (840–846 bp for clade A, 885–909 bp for clade B according to the available genomic and metagenomic datasets from comammox Nitrospira). To achieve a good coverage of the known comammox amoA gene diversity, primer design was restricted to the range of aligned nucleotide positions for which sequence information exists in at least 80% of the publicly available comammox amoA sequences. Furthermore, availability of sequence information for at least one of the primer binding sites in the great majority of betaproteobacterial amoA gene sequences (14,000/14,019) and bacterial pmoA gene sequences (9,913/10,289) facilitated the design of highly comammox amoA-specific primers. Two degenerate primer pairs (ComaA-244F/659R and ComaB-244F/659R) targeted 95 and 83% of the amoA gene sequences from comammox clade A or B, respectively (Table 1). In silico analyses confirmed that each primer contained at least 4 mismatches to all sequences within the respective other comammox amoA clade. Furthermore, at least 3 mismatches to almost all sequences affiliated with betaproteobacterial amoA and with pmoA genes were present. Merely 23 out of 14,019 betaproteobacterial amoA had only two mismatches to the comammox amoA clade A-targeted primers. Further, only 22 and 2 out of 9,913 pmoA gene sequences showed only two mismatches to the comammox amoA clade A- and clade B-targeted primers, respectively. No betaproteobacterial amoA and no pmoA gene sequences had less than two mismatches to any of the comammox amoA-targeted primer pairs.
The new primer sets were first evaluated with a pasty iron sludge sample from the GWW Wolfenbüttel, which was known to harbor both clade A and clade B comammox Nitrospira according to a previous metagenomic analysis (Daims et al., 2015). A single amplicon of the expected length (415 bp) was obtained from this sample with both primer pairs after PCR with an annealing temperature of 52°C. Cloning and sequencing of the PCR products exclusively retrieved sequences of comammox amoA from clade A or clade B, respectively (Figure 1). After application of the two primer sets to 12 additional samples from various environments, comammox amoA clade A was detected in 11 samples and clade B in 7 samples (see below and Table 2). Single, correctly sized amplicons were obtained from the majority of samples (8 of the clade A-positive samples and 6 of the clade B-positive samples, Table 2). In the remaining cases, unspecific PCR products of different lengths were observed in addition to the expected amoA amplicons. These unspecific PCR products appeared irrespectively of the applied DNA extraction method and the number of PCR cycles, but were overcome by either purifying the target amoA amplicon by agarose gel excision (for the lake Herrensee sample) or by a PCR-based clone screening for the right insert size after cloning (for the remaining samples with unspecific PCR products). In summary, we retrieved 446 CuMMO sequences in this study by PCR, cloning and sequencing from the 13 environmental samples (Table 2), 444 of which were comammox amoA gene sequences. Only two sequences did not cluster with comammox amoA clades, but were classified as pmoA. Furthermore, one sequence obtained with the comammox amoA clade B-targeted primers turned out to be affiliated with comammox amoA clade A. Thus, the degenerate comammox amoA-targeted primers offer a straightforward, fast and robust approach for the detection and identification of comammox Nitrospira in complex samples. Notably, with an amplicon size of 415 bp the primers are also suitable for high-throughput amplicon sequencing by current Illumina technologies.
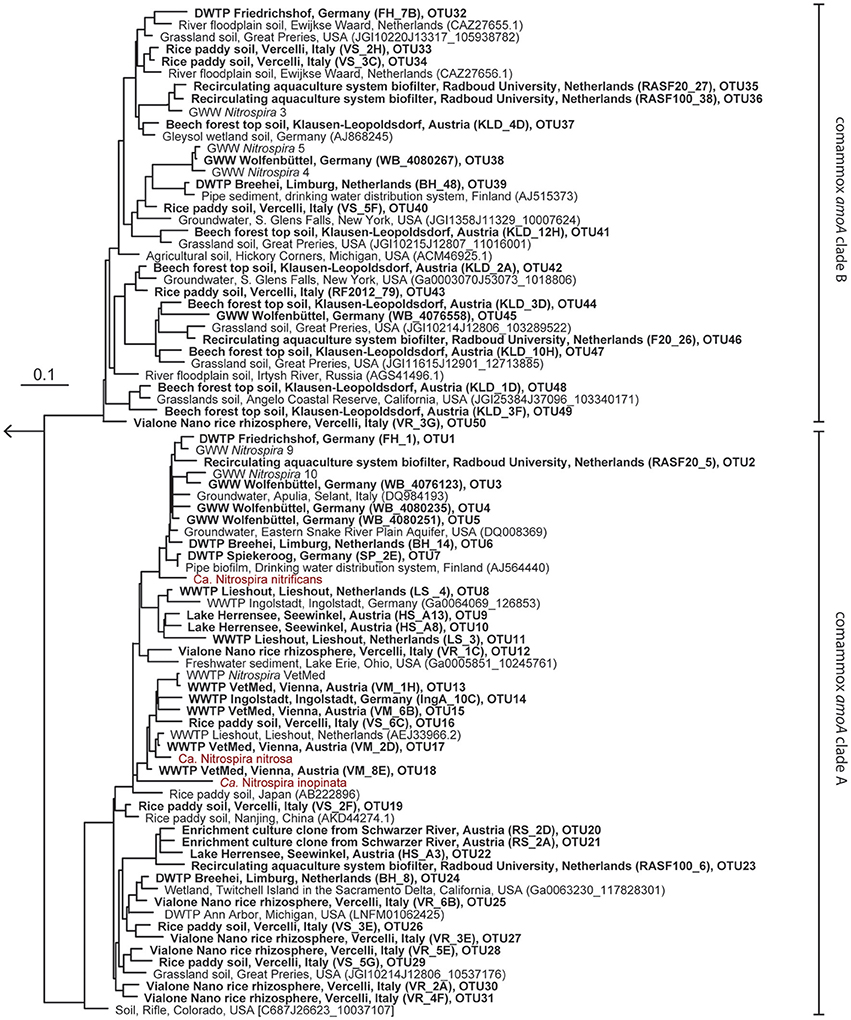
Figure 1. Maximum likelihood tree showing the phylogenetic affiliation of comammox amoA genes obtained in this study (printed in boldface) to reference amoA sequences from comammox clades A and B. One representative sequence from each OTU is shown. Identifiers of clones (in parentheses) and of OTUs are indicated. Previously described comammox Nitrospira strains are shown in red. The outgroup consisted of selected betaproteobacterial amoA and proteobacterial pmoA genes. The scale bar indicates the estimated change rate per nucleotide.
Since amplification of non-target DNA was observed with some samples, we took into account that primer degeneracy may impair PCR specificity (Linhart and Shamir, 2002). Furthermore, primer degeneracy can cause an uneven amplification of different sequence variants (Polz and Cavanaugh, 1998). In general, less degenerate, defined primer mixtures can reduce unspecific primer binding and yield cleaner amplification products from complex environmental samples (Linhart and Shamir, 2002). This is of particular importance for obtaining correct population size estimates in qPCR assays. Consequently, less degenerate primer mixtures consisting of separately synthesized versions of the respective forward and reverse primer, with each primer version containing only one or no base ambiguity (Table 1), were also tested. All selected primer versions in these mixtures matched real comammox amoA gene sequences in our database. In contrast, the original (more degenerate) primer sets inevitably contained also co-synthesized oligonucleotide versions that did not match any known target comammox amoA sequence. The less degenerate mixtures offered the same coverage of comammox amoA clade A (95%), and by adding one additional oligonucleotide to the forward primer mix we increased the coverage of comammox amoA clade B from 83 to 92%. Considerably improved PCR results were achieved by applying these primer mixtures to the samples prone to unspecific amplification, as only amplicons of the expected size were obtained after PCR (data now shown). Based on their improved specificity and the higher coverage of comammox amoA clade B, use of the defined, manually pooled equimolar oligonucleotide (primer) mixtures (Table 1) is recommended. For comammox amoA-targeted qPCR experiments that use the primer sets presented here, application of the less degenerate, equimolar primer mixtures is mandatory. In general, we recommend that the identity of PCR amplification products should be confirmed prior to downstream analyses of comammox amoA or any other functional gene diversity and abundance.
Environmental Detection and Diversity of Comammox Nitrospira
Intriguingly, comammox amoA genes were detected in all samples (Table 2) encompassing forest soil, rice paddy soils and rice rhizosphere, a freshwater biofilm and brackish lake sediment, as well as WWTPs and DWTPs. After cloning and sequencing, the obtained amoA sequences were clustered in operational taxonomic units (OTUs) based on a sequence identity threshold of 95% (Francis et al., 2003). It should be noted that these OTUs might not delineate species, as an appropriate species-level sequence identity cutoff for comammox amoA remains unknown. This would need to be determined by correlating amoA sequence identities with 16S rRNA identities or with genome-wide average nucleotide identities (Purkhold et al., 2000; Richter and Rosselló-Móra, 2009; Pester et al., 2014) once more genomic data from comammox Nitrospira become available. A phylogenetic analysis of the amoA gene OTU representatives revealed a substantial diversity of comammox Nitrospira in almost all samples (Figure 1, Table 2, Table S1). Both clade A and clade B comammox Nitrospira were detected in seven samples. Only clade A members were found in the WWTPs, the brackish lake sediment, and the river biofilm enrichment, whereas we retrieved only clade B amoA gene sequences from the forest soil (Table 2). The widespread occurrence of comammox Nitrospira in the analyzed samples is consistent with the previously reported presence of mostly uncultured and uncharacterized Nitrospira members in the respective habitat types (e.g., Daims et al., 2001; Martiny et al., 2005; Ke et al., 2013; Pester et al., 2014). Future studies should determine which fraction of these environmental Nitrospira are strict NOB or comammox, respectively. The presence of multiple comammox OTUs, and in particular the co-occurrence of both clade A and clade B comammox, in several samples (Table 2) indicates ecological niche partitioning that enables the coexistence of different comammox strains. For co-occurring Nitrospira in WWTPs, niche partitioning based on the preferred nitrite concentrations, on the capability to utilize formate as an alternative substrate, and on the tendency to co-aggregate with AOB has already been demonstrated (Maixner et al., 2006; Gruber-Dorninger et al., 2015). It is tempting to speculate that underlying mechanisms of niche differentiation of comammox Nitrospira could also be different substrate (ammonia) concentration optima and alternative energy metabolisms, such as the oxidation of formate and of hydrogen (Koch et al., 2014, 2015).
Quantification of Comammox amoA Genes by Quantitative PCR
Since the discovery of AOA (Könneke et al., 2005), numerous studies compared the abundances of AOB and AOA, and tried to assess the contributions of different groups of ammonia oxidizers to nitrification in various engineered and natural environments (e.g., Leininger et al., 2006; Chen et al., 2008; Jia and Conrad, 2009; Mußmann et al., 2011; Daebeler et al., 2012; Ke et al., 2013; Bollmann et al., 2014). To adequately investigate the niche differentiation and labor partitioning between ammonia oxidizers, it will be necessary to include comammox Nitrospira in such comparisons. This will require a robust method for the rapid and accurate quantification of comammox Nitrospira. Thus, we established qPCR assays using the equimolar primer mixtures that target comammox amoA clade A or clade B, respectively. Both assays had a high efficiency, accuracy and sensitivity, as the quantification of as few as 10 copies of comammox amoA standards was achieved (Figure S1).
As proof of applicability, we quantified comammox amoA clade A and clade B genes, alongside with archaeal and bacterial amoA genes, in five different sample types: one activated sludge sample (WWTP VetMed, Vienna); pasty iron sludge from the riser pipe of GWW Wolfenbüttel, Germany; a trickling filter sample from the DWTP Friedrichshof, Germany; paddy rice soil from Vercelli, Italy and a beech forest soil from Klausen-Leopoldsdorf, Austria (Figure 2, Table S2). Melting curve analyses confirmed the specificity of both newly established comammox amoA targeted assays (Figure S2). Amplification of non-target DNA occurred only in those samples that lacked the respective target organisms of the primers according to the aforementioned results of the end point PCR (i.e., WWTP VetMed for clade B and Klausen-Leopoldsdorf forest soil for clade A comammox) and was easily detectable based on a distinct slope of the melting curves of the unspecific amplicons (Figure S2). The copy numbers of clade A and clade B comammox amoA genes were in the same order of magnitude in the samples containing both groups (Figure 2, Table S2). Interestingly, the predominance of the different groups of ammonia oxidizers varied strongly among the analyzed samples (Figure 2). In the activated sludge from WWTP VetMed, the gene abundances of betaproteobacterial and comammox clade A amoA were significantly different (p < 0.01). Comammox clade A amoA copy numbers accounted for 14 to 34% of the estimated total amoA copy number (Table S2), indicating that comammox Nitrospira could be functionally relevant for nitrification in this system. The amoA qPCR-based detection of both comammox Nitrospira and AOB, as well as the absence of AOA, in WWTP VetMed are consistent with an earlier analysis of the same sample based on metagenomics and rRNA-targeted FISH (Daims et al., 2015). The qPCR-based dominance of clade A and B comammox Nitrospira over AOB (p < 0.01) in GWW Wolfenbüttel (Figure 2, Table S2) is in line with the previous metagenomic detection of both clades in samples from the same system (Daims et al., 2015). Altogether, the results obtained in this and other studies (Daims et al., 2015; van Kessel et al., 2015; Palomo et al., 2016; Pinto et al., 2016; Bartelme et al., 2017; Wang et al., 2017) for wastewater and drinking water treatment plants suggest that comammox Nitrospira have to be considered in future studies of nitrification in engineered systems. Likewise, the relative abundances of comammox amoA genes in comparison to those of AOA and AOB in the two soils (Figure 2) indicate that comammox organisms should not be neglected in research on terrestrial nitrifiers.
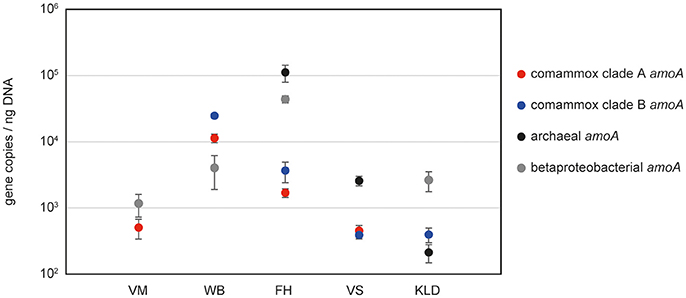
Figure 2. Abundances of amoA genes from comammox Nitrospira, AOA and AOB in selected samples from various environments. VM, WWTP VetMed; WB, GWW Wolfenbüttel; FH, DWTP Friedrichshof; VS, Vercelli rice paddy soil; KLD, Klausen-Leopoldsdorf beech forest soil.
Summary and Outlook
The newly developed primer sets were designed for maximal coverage of the known comammox Nitrospira lineages, high specificity for comammox amoA genes, and a broad range of applications including high-throughput amplicon sequencing and qPCR. By using these primer sets, we detected the presence of comammox Nitrospira in a variety of habitats and found their amoA genes to be of comparable abundance as the amoA of other ammonia oxidizers. In this methodological study, our primary goal was to evaluate the applicability of the newly designed primers for end point PCR and qPCR with a selection of different sample types. A much broader census of comammox Nitrospira in the environment is needed before general conclusions on their distribution and importance for nitrification can be drawn. However, as was previously shown for AOA in a WWTP (Mußmann et al., 2011), high amoA gene copy numbers do not always indicate that the respective population is functionally important for ammonia oxidation. Aside from the possibility that abundant putative nitrifiers grow on alternative substrates, one can also not exclude amoA gene quantification biases caused by extracellular DNA, which may for example be secreted to a different extent by the phylogenetically diverse nitrifiers. Moreover, at least in AOA a basal amoA transcription can occur even in the absence of detectable ammonia-oxidizing activity (Mußmann et al., 2011; Ke et al., 2013). In contrast, pronounced shifts in the amoA transcription levels upon certain environmental stimuli, such as a changing availability of ammonium, likely are useful activity indicators for AOA, AOB, and comammox Nitrospira. Although not tested yet in this context, the new comammox amoA-specific primers should in principle be suitable for reverse transcription-qPCR of amoA and should thus allow the quantification of comammox amoA transcripts in environmental samples and during incubation experiments. Thus, the primers can serve as a tool for a broad range of study designs to elucidate the ecological significance of comammox Nitrospira in comparison to other ammonia oxidizers in natural and engineered ecosystems.
Author Contributions
PP, SL, and HD conceived the research idea. PP, SL, HD, Mv, MJ, and MW contributed to the development of the research plan and project goals. CH performed database mining, and CH and PP compiled the pmoA/amoA database used for primer design by PP, CS, LP, PP, AD, and MS performed laboratory work, and contributed to data analysis. SL, HD, Mv, MJ, and MW contributed sources of project funding. PP and HD were the primary authors writing the manuscript, while all other co-authors were involved in writing and editing of the manuscript.
Funding
This research was supported by the Austrian Science Fund (FWF) projects P27319-B21 and P25231-B21 to HD, the Netherlands Organization for Scientific Research (NWO VENI grant 863.14.019) to SL, European Research Council Advanced Grant projects NITRICARE 294343 to MW and Eco_MoM 339880 to MJ, the Technology Foundation STW (grant 13146) to Mv and MJ, and the Netherlands Earth System Science Centre (NESSC), and through financing from the Ministry of Education, Culture and Science (OCW) of The Netherlands. The Radboud Excellence Initiative is acknowledged for support to SL.
Conflict of Interest Statement
The authors declare that the research was conducted in the absence of any commercial or financial relationships that could be construed as a potential conflict of interest.
Acknowledgments
The authors would like to thank Bernd Bendinger, Kay Bouts, Stephanie Eichorst, Mohammad Ghashghavi, Hannes Schmidt, Jasmin Schwarz, Annika Vaksmaa, Giampiero Valè, Weren de Vet, Julia Vierheilig, Dagmar Woebken, and Thomas Zechmeister for providing sample material. Ping Han, Bela Hausmann, and Roey Angel are acknowledged for valuable discussion and advice with the qPCR assay setup. Andreas Pommerening-Röser is acknowledged for providing the N. nitrosa Nm90 isolate. A preprint of this article was made available at bioRxiv (https://doi.org/10.1101/096891).
Supplementary Material
The Supplementary Material for this article can be found online at: http://journal.frontiersin.org/article/10.3389/fmicb.2017.01508/full#supplementary-material
References
Angel, R., Claus, P., and Conrad, R. (2012). Methanogenic archaea are globally ubiquitous in aerated soils and become active under wet anoxic conditions. ISME J. 6, 847–862. doi: 10.1038/ismej.2011.141
Bartelme, R. P., McLellan, S. L., and Newton, R. J. (2017). Freshwater recirculating aquaculture system operations drive biofilter bacterial community shifts around a stable nitrifying consortium of ammonia-oxidizing archaea and comammox Nitrospira. Front. Microbiol. 8:101. doi: 10.3389/fmicb.2017.00101
Bollmann, A., Bullerjahn, G. S., and McKay, R. M. (2014). Abundance and diversity of ammonia-oxidizing archaea and bacteria in sediments of trophic end members of the Laurentian Great Lakes, Erie and Superior. PLoS ONE 9:e97068. doi: 10.1371/journal.pone.0097068
Chen, X., Zhu, Y. G., Xia, Y., Shen, J. P., and He, J. Z. (2008). Ammonia-oxidizing archaea: important players in paddy rhizosphere soil?. Environ. Microbiol. 10, 1978–1987. doi: 10.1111/j.1462-2920.2008.01613.x
Daebeler, A., Abell, G. C., Bodelier, P. L., Bodrossy, L., Frampton, D. M., Hefting, M. M., et al. (2012). Archaeal dominated ammonia-oxidizing communities in Icelandic grassland soils are moderately affected by long-term N fertilization and geothermal heating. Front. Microbiol. 3:352. doi: 10.3389/fmicb.2012.00352
Daims, H., Lebedeva, E. V., Pjevac, P., Han, P., Herbold, C., Albertsen, M., et al. (2015). Complete nitrification by Nitrospira bacteria. Nature 528, 504–509. doi: 10.1038/nature16461
Daims, H., Nielsen, J. L., Nielsen, P. H., Schleifer, K. H., and Wagner, M. (2001). In Situ Characterization of Nitrospira-Like Nitrite-Oxidizing Bacteria Active in Wastewater Treatment Plants. Appl. Environ. Microbiol. 67, 5273–5284. doi: 10.1128/AEM.67.11.5273-5284.2001
Edgar, R. C. (2004). MUSCLE: multiple sequence alignment with high accuracy and high throughput. Nucleic Acids Res. 32, 1792–1797. doi: 10.1093/nar/gkh340
Edgar, R. C. (2010). Search and clustering orders of magnitude faster than BLAST. Bioinformatics 26, 2460–2461. doi: 10.1093/bioinformatics/btq461
Ehrich, S., Behrens, D., Lebedeva, E., Ludwig, W., and Bock, E. (1995). A new obligately chemolithoautotrophic, nitrite-oxidizing bacterium, Nitrospira moscoviensis sp. nov. and its phylogenetic relationship. Arch. Microbiol. 164, 16–23. doi: 10.1007/BF02568729
Francis, C. A., O'Mullan, G. D., and Ward, B. B. (2003). Diversity of ammonia monooxygenase (amoA) genes across environmental gradients in Chesapeake Bay sediments. Geobiology 1, 129–140. doi: 10.1046/j.1472-4669.2003.00010.x
Francis, C. A., Roberts, K. J., Beman, J. M., Santoro, A. E., and Oakley, B. B. (2005). Ubiquity and diversity of ammonia-oxidizing archaea in water columns and sediments of the ocean. Proc. Nat. Acad. Sci. U.S.A. 102, 14683–14688. doi: 10.1073/pnas.0506625102
Gonzalez-Martinez, A., Rodriguez-Sanchez, A., van Loosdrecht, M. M., Gonzalez-Lopez, J., and Vahala, R. (2016). Detection of comammox bacteria in full-scale wastewater treatment bioreactors using tag-454-pyrosequencing. Environ. Sci. Pollut. R. 23, 25501–25511. doi: 10.1007/s11356-016-7914-4
Gruber-Dorninger, C., Pester, M., Kitzinger, K., Savio, D. F., Loy, A., Rattei, T., et al. (2015). Functionally relevant diversity of closely related Nitrospira in activated sludge. ISME J. 9, 643–655. doi: 10.1038/ismej.2014.156
Holmes, A. J., Costello, A., Lidstrom, M. E., and Murrell, J. C. (1995). Evidence that participate methane monooxygenase and ammonia monooxygenase may be evolutionarily related. FEMS Microbiol. Lett. 132, 203–208. doi: 10.1111/j.1574-6968.1995.tb07834.x
Jia, Z., and Conrad, R. (2009). Bacteria rather than Archaea dominate microbial ammonia oxidation in an agricultural soil. Environ. Microbiol. 11, 1658–1671. doi: 10.1111/j.1462-2920.2009.01891.x
Junier, P., Kim, O. S., Molina, V., Limburg, P., Junier, T., Imhoff, J. F., et al. (2008). Comparative in silico analysis of PCR primers suited for diagnostics and cloning of ammonia monooxygenase genes from ammonia-oxidizing bacteria. FEMS Microbiol. Ecol. 64, 141–152. doi: 10.1111/j.1574-6941.2007.00437.x
Juretschko, S., Timmermann, G., Schmid, M., Schleifer, K. H., Pommerening-Röser, A., Koops, H. P., et al. (1998). Combined molecular and conventional analyses of nitrifying bacterium diversity in activated sludge: Nitrosococcus mobilis and Nitrospira-like bacteria as dominant populations. Appl. Environ. Microbiol. 64, 3042–3051.
Katoh, K., Misawa, K., Kuma, K. I., and Miyata, T. (2002). MAFFT: a novel method for rapid multiple sequence alignment based on fast Fourier transform. Nucleic Acids Res. 30, 3059–3066. doi: 10.1093/nar/gkf436
Ke, X., Angel, R., Lu, Y., and Conrad, R. (2013). Niche differentiation of ammonia oxidizers and nitrite oxidizers in rice paddy soil. Environ. Microbiol. 15, 2275–2292. doi: 10.1111/1462-2920.12098
Koch, H., Galushko, A., Albertsen, M., Schintlmeister, A., Gruber-Dorninger, C., Lücker, S., et al. (2014). Growth of nitrite-oxidizing bacteria by aerobic hydrogen oxidation. Science 345, 1052–1054. doi: 10.1126/science.1256985
Koch, H., Lücker, S., Albertsen, M., Kitzinger, K., Herbold, C., Spieck, E., et al. (2015). Expanded metabolic versatility of ubiquitous nitrite-oxidizing bacteria from the genus Nitrospira. Proc. Nat. Acad. Sci. U.S.A. 112, 11371–11376. doi: 10.1073/pnas.1506533112
Könneke, M., Bernhard, A. E., José, R., Walker, C. B., Waterbury, J. B., and Stahl, D. A. (2005). Isolation of an autotrophic ammonia-oxidizing marine archaeon. Nature 437, 543–546. doi: 10.1038/nature03911
Lebedeva, E. V., Alawi, M., Maixner, F., Jozsa, P. G., Daims, H., and Spieck, E. (2008). Physiological and phylogenetic characterization of a novel lithoautotrophic nitrite-oxidizing bacterium, “Candidatus Nitrospira bockiana.” IJSEM 58, 242–250. doi: 10.1099/ijs.0.65379-0
Lebedeva, E. V., Off, S., Zumbrägel, S., Kruse, M., Shagzhina, A., Lücker, S., et al. (2011). Isolation and characterization of a moderately thermophilic nitrite-oxidizing bacterium from a geothermal spring. FEMS Microbiol. Ecol. 75, 195–204. doi: 10.1111/j.1574-6941.2010.01006.x
Leininger, S., Urich, T., Schloter, M., Schwark, L., Qi, J., Nicol, G. W., et al. (2006). Archaea predominate among ammonia-oxidizing prokaryotes in soils. Nature 442, 806–809. doi: 10.1038/nature04983
Linhart, C., and Shamir, R. (2002). The degenerate primer design problem. Bioinformatics 18, S172–S181. doi: 10.1093/bioinformatics/18.suppl_1.S172
Ludwig, W., Strunk, O., Westram, R., Richter, L., Meier, H., Buchner, A., et al. (2004). ARB: a software environment for sequence data. Nucleic Acids Res. 32, 1363–1371. doi: 10.1093/nar/gkh293
Luesken, F. A., van Alen, T. A., van der Biezen, E., Frijters, C., Toonen, G., Kampman, C., et al. (2011). Diversity and enrichment of nitrite-dependent anaerobic methane oxidizing bacteria from wastewater sludge. Appl. Microbiol. Biot. 92, 845–854. doi: 10.1007/s00253-011-3361-9
Maixner, F., Noguera, D. R., Anneser, B., Stoecker, K., Wegl, G., Wagner, M., et al. (2006). Nitrite concentration influences the population structure of Nitrospira-like bacteria. Environ. Microbiol. 8, 1487–1495. doi: 10.1111/j.1462-2920.2006.01033.x
Martiny, A. C., Albrechtsen, H. J., Arvin, E., and Molin, S. (2005). Identification of bacteria in biofilm and bulk water samples from a nonchlorinated model drinking water distribution system: detection of a large nitrite-oxidizing population associated with Nitrospira spp. Appl. Environ. Microbiol. 71, 8611–8617. doi: 10.1128/AEM.71.12.8611-8617.2005
Meinhardt, K. A., Bertagnolli, A., Pannu, M. W., Strand, S. E., Brown, S. L., et al. (2015). Evaluation of revised polymerase chain reaction primers for more inclusive quantification of ammonia-oxidizing archaea and bacteria. Environ. Microbiol. Rep. 7, 354–363. doi: 10.1111/1758-2229.12259
Mincer, T. J., Church, M. J., Taylor, L. T., Preston, C., Karl, D. M., and DeLong, E. F. (2007). Quantitative distribution of presumptive archaeal and bacterial nitrifiers in Monterey Bay and the North Pacific Subtropical Gyre. Environ. Microbiol. 9, 1162–1175. doi: 10.1111/j.1462-2920.2007.01239.x
Mußmann, M., Brito, I., Pitcher, A., Damsté, J. S. S., Hatzenpichler, R., Richter, A., et al. (2011). Thaumarchaeotes abundant in refinery nitrifying sludges express amoA but are not obligate autotrophic ammonia oxidizers. Proc. Nat. Acad. Sci. U.S.A. 108, 16771–16776. doi: 10.1073/pnas.1106427108
Nold, S. C., Zhou, J., Devol, A. H., and Tiedje, J. M. (2001). Pacific Northwest Marine Sediments Contain Ammonia-Oxidizing Bacteria in the β Subdivision of the Proteobacteria. Appl. Environ. Microbiol. 66, 4532–4535. doi: 10.1128/AEM.66.10.4532-4535.2000
Norton, J. M., Alzerreca, J. J., Suwa, Y., and Klotz, M. G. (2002). Diversity of ammonia monooxygenase operon in autotrophic ammonia-oxidizing bacteria. Arch. Microbiol. 17, 139–149. doi: 10.1007/s00203-001-0369-z
Okano, Y., Hristova, K. R., Leutenegger, C. M., Jackson, L. E., Denison, R. F., Gebreyesus, B., et al. (2004). Application of real-time PCR to study effects of ammonium on population size of ammonia-oxidizing bacteria in soil. Appl. Environ. Microbiol. 70, 1008–1016. doi: 10.1128/AEM.70.2.1008-1016.2004
Oswald, K., Graf, J. S., Littmann, S., Tieken, D., Brand, A., Wehrli, B., et al. (2017). Crenothrix are major methane consumers in stratified lakes. ISME J. doi: 10.1038/ismej.2017.77. [Epub ahead of print].
Palomo, A., Fowler, S. J., Gülay, A., Rasmussen, S., Sicheritz-Ponten, T., and Smets, B. F. (2016). Metagenomic analysis of rapid gravity sand filter microbial communities suggests novel physiology of Nitrospira spp. ISME J. 10, 2569–2580. doi: 10.1038/ismej.2016.63
Pester, M., Maixner, F., Berry, D., Rattei, T., Koch, H., Lücker, S., et al. (2014). NxrB encoding the beta subunit of nitrite oxidoreductase as functional and phylogenetic marker for nitrite-oxidizing Nitrospira. Environ. Microbiol. 16, 3055–3071. doi: 10.1111/1462-2920.12300
Pester, M., Rattei, T., Flechl, S., Gröngröft, A., Richter, A., Overmann, J., et al. (2012). AmoA-based consensus phylogeny of ammonia-oxidizing archaea and deep sequencing of amoA genes from soils of four different geographic regions. Environ. Microbiol. 14, 525–539. doi: 10.1111/j.1462-2920.2011.02666.x
Pinto, A. J., Marcus, D. N., Ijaz, U. Z., Bautista-de lose Santos, Q. M., Dick, G. J., and Raskin, L. (2016). Metagenomic evidence for the presence of comammox Nitrospira-like bacteria in a drinking water system. mSphere 1:e00054-15. doi: 10.1128/mSphere.00054-15
Polz, M. F., and Cavanaugh, C. M. (1998). Bias in template-to-product ratios in multitemplate PCR. Appl. Environ. Microbiol. 64, 3724–3730.
Price, M. N., Dehal, P. S., and Arkin, A. P. (2009). FastTree: computing large minimum evolution trees with profiles instead of a distance matrix. Mol. Biol. Evol. 26, 1641–1650. doi: 10.1093/molbev/msp077
Purkhold, U., Pommerening-Röser, A., Juretschko, S., Schmid, M. C., Koops, H. P., and Wagner, M. (2000). Phylogeny of all recognized species of ammonia oxidizers based on comparative 16S rRNA and amoA sequence analysis: implications for molecular diversity surveys. Appl. Environ. Microbiol. 66, 5368–5382. doi: 10.1128/AEM.66.12.5368-5382.2000
Richter, M., and Rosselló-Móra, R. (2009). Shifting the genomic gold standard for the prokaryotic species definition. Proc. Nat. Acad. Sci. U.S.A. 106, 19126–19131. doi: 10.1073/pnas.0906412106
Rotthauwe, J. H., Witzel, K. P., and Liesack, W. (1997). The ammonia monooxygenase structural gene amoA as a functional marker: molecular fine-scale analysis of natural ammonia-oxidizing populations. Appl. Environ. Microbiol. 63, 4704–4712.
Sinigalliano, C. D., Kuhn, D. N., and Jones, R. D. (1995). Amplification of the amoA gene from diverse species of ammonium-oxidizing bacteria and from an indigenous bacterial population from seawater. Appl. Environ. Microbiol. 61, 2702–2706.
Spieck, E., Hartwig, C., McCormack, I., Maixner, F., Wagner, M., Lipski, A., et al. (2006). Selective enrichment and molecular characterization of a previously uncultured Nitrospira-like bacterium from activated sludge. Environ. Microbiol. 8, 405–415. doi: 10.1111/j.1462-2920.2005.00905.x
Stamatakis, A., Ludwig, T., and Meier, H. (2005). RAxML-III: a fast program for maximum likelihood-based inference of large phylogenetic trees. Bioinformatics 21, 456–463. doi: 10.1093/bioinformatics/bti191
Stephen, J. R., Chang, Y. J., Macnaughton, S. J., Kowalchuk, G. A., Leung, K. T., Flemming, C. A., et al. (1999). Effect of toxic metals on indigenous soil β-subgroup proteobacterium ammonia oxidizer community structure and protection against toxicity by inoculated metal-resistant bacteria. Appl. Environ. Microbiol. 65, 95–101.
Stoecker, K., Bendinger, B., Schöning, B., Nielsen, P. H., Nielsen, J. L., Baranyi, C., et al. (2006). Cohn's Crenothrix is a filamentous methane oxidizer with an unusual methane monooxygenase. Proc. Nat. Acad. Sci. U.S.A. 103, 2363–2367. doi: 10.1073/pnas.0506361103
Treusch, A. H., Leininger, S., Kletzin, A., Schuster, S. C., Klenk, H. P., and Schleper, C. (2005). Novel genes for nitrite reductase and Amo-related proteins indicate a role of uncultivated mesophilic crenarchaeota in nitrogen cycling. Environ. Microbiol. 7, 1985–1995. doi: 10.1111/j.1462-2920.2005.00906.x
van Kessel, M. A., Speth, D. R., Albertsen, M., Nielsen, P. H., den Camp, H. J. O., Kartal, B., et al. (2015). Complete nitrification by a single microorganism. Nature 528, 555–559. doi: 10.1038/nature16459
Wang, J. G., Xia, F., Zeleke, J., Zou, B., Rhee, S. K., and Quan, Z. X. (2016). An improved protocol with a highly degenerate primer targeting copper-containing membrane-bound monooxygenase (CuMMO) genes for community analysis of methane-and ammonia-oxidizing bacteria. FEMS Microbiol. Ecol. 93:fiw244. doi: 10.1093/femsec/fiw244
Wang, Y., Ma, L., Mao, Y., Jiang, X., Xia, Y., Yu, K., et al. (2017). Comammox in drinking water systems. Water Res. 116, 332–341. doi: 10.1016/j.watres.2017.03.042
Watson, S. W., Bock, E., Valois, F. W., Waterbury, J. B., and Schlosser, U. (1986). Nitrospira marina gen. nov. sp. nov.: a chemolithotrophic nitrite-oxidizing bacterium. Arch. Microbiol. 144, 1–7. doi: 10.1007/BF00454947
Keywords: nitrification, comammox, Nitrospira, amoA, marker gene, PCR
Citation: Pjevac P, Schauberger C, Poghosyan L, Herbold CW, van Kessel MAHJ, Daebeler A, Steinberger M, Jetten MSM, Lücker S, Wagner M and Daims H (2017) AmoA-Targeted Polymerase Chain Reaction Primers for the Specific Detection and Quantification of Comammox Nitrospira in the Environment. Front. Microbiol. 8:1508. doi: 10.3389/fmicb.2017.01508
Received: 04 April 2017; Accepted: 27 July 2017;
Published: 04 August 2017.
Edited by:
Martin G. Klotz, Washington State University Tri-Cities, United StatesReviewed by:
Lisa Y. Stein, University of Alberta, CanadaMee-Rye Park, Columbia University, United States
Hongkeun Park, Columbia University, United States
Copyright © 2017 Pjevac, Schauberger, Poghosyan, Herbold, van Kessel, Daebeler, Steinberger, Jetten, Lücker, Wagner and Daims. This is an open-access article distributed under the terms of the Creative Commons Attribution License (CC BY). The use, distribution or reproduction in other forums is permitted, provided the original author(s) or licensor are credited and that the original publication in this journal is cited, in accordance with accepted academic practice. No use, distribution or reproduction is permitted which does not comply with these terms.
*Correspondence: Petra Pjevac, cGpldmFjQG1pY3JvYmlhbC1lY29sb2d5Lm5ldA==
Sebastian Lücker, cy5sdWVja2VyQHNjaWVuY2UucnUubmw=
Holger Daims, ZGFpbXNAbWljcm9iaWFsLWVjb2xvZ3kubmV0
†These authors have contributed equally to this work.