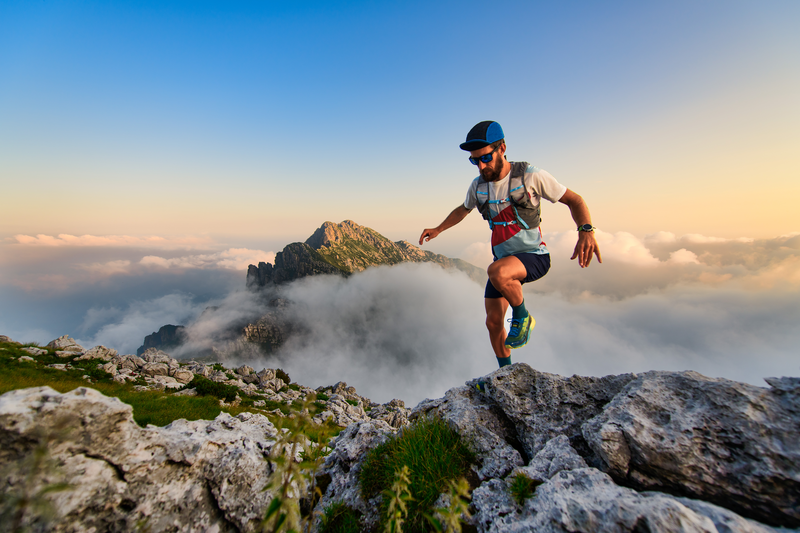
94% of researchers rate our articles as excellent or good
Learn more about the work of our research integrity team to safeguard the quality of each article we publish.
Find out more
REVIEW article
Front. Microbiol. , 09 August 2017
Sec. Infectious Agents and Disease
Volume 8 - 2017 | https://doi.org/10.3389/fmicb.2017.01473
This article is part of the Research Topic Parasitology Research in China View all 53 articles
China has set a goal to eliminate all malaria in the country by 2020, but it is unclear if current understanding of malaria vectors and transmission is sufficient to achieve this objective. Anopheles sinensis is the most widespread malaria vector specie in China, which is also responsible for vivax malaria outbreak in central China. We reviewed literature from 1954 to 2016 on An. sinensis with emphasis on biology, bionomics, and molecular biology. A total of 538 references were relevant and included. An. sienesis occurs in 29 Chinese provinces. Temperature can affect most life-history parameters. Most An. sinensis are zoophilic, but sometimes they are facultatively anthropophilic. Sporozoite analysis demonstrated An. sinensis efficacy on Plasmodium vivax transmission. An. sinensis was not stringently refractory to P. falciparum under experimental conditions, however, sporozoite was not found in salivary glands of field collected An. sinensis. The literature on An. sienesis biology and bionomics was abundant, but molecular studies, such as gene functions and mechanisms, were limited. Only 12 molecules (genes, proteins or enzymes) have been studied. In addition, there were considerable untapped omics resources for potential vector control tools. Existing information on An. sienesis could serve as a baseline for advanced research on biology, bionomics and genetics relevant to vector control strategies.
Malaria was once epidemic in China and disease levels were high. However, a significant decline of malaria incidence has occurred with reported cases declining from > 9 million cases in the 1960s to only 3078 cases in 2014 (Tang, 2010; Wang, 2014; Li et al., 2015). In 2010, the Chinese Government launched Malaria Elimination Program with a goal to eliminate malaria in the entire country by 2020 (Feng et al., 2014).
Four Anopheline species, Anopheles sinensis, Anopheles anthropophagus, Anopheles minimus, and Anopheles dirus, are considered main vectors for malaria transmission in China. Among these, An. sinensis is the most widely distributed species (Zhu et al., 2013). It is the most important malaria vector in flatlands, especially in the paddy planting regions. An. sinensis is considered to be a competent vector for Plasmodium vivax malaria since it is the only major vector in central China where P. vivax is prevalent, locally transmitted, and where several malaria epidemics have occurred (Zhou et al., 2007). Besides malaria, An. sinensis can also transmit lymphatic filariasis (Reid, 1968), JEV and Rickettsia felis (Scherer et al., 1959; Zhang et al., 2014).
The distribution, habitat, feeding behavior, and host selection of An. sinensis in China has been extensively studied. Ma (1954) published the first biology study of An. sinensis and since then, there have been many reports on its biology or bionomics. This information has contributed to the success of malaria control programs. Larval reduction by drainage, and filling, and IRS have been the main malaria control measures. The primary intervention measures for malaria elimination in China continue to target the adult vector by IRS or LLINs (Liu and Liu, 2010).
Interactions between vector and parasite are important in malaria transmission dynamics. Identification of molecules involved in multifaceted developmental cycles of parasites within the vector and the related mechanisms accounting for survival and proliferation can provide attractive targets to interfere in the disease transmission (Sreenivasamurthy et al., 2013). However, many of the molecules and mechanisms in An. sinensis are still remain unknown. Understanding the underlying details of the vector-pathogen interaction would underpin the prevention and control of parasitic diseases.
Although there are many studies on An. sinensis distribution, bionomics and molecular study in China, the information was notably dispersed in the literature. So, current studies were systematically reviewed. The objective of the present study was to review the biology, bionomics and molecules of An. sinensis in China. This could provide insights for development of novel mosquito control strategies and increase the effectiveness of the vector control interventions in elimination campaign.
An electronic search of peer-reviewed scientific and medical literature published between January 1954 and September 2016 in Chinese and English was conducted using PubMed (MEDLINE), CNKI (China National Knowledge Infrastructure), VIP (Chongqing VIP Database), and CSPD (China Science Periodical Database, Wan Fang) and Web of Science databases. Gray literature and programmatic documents were also searched using Google, Google Scholar and other search engines using the same search terms.
The following search terms (or their Chinese equivalents) were used: Anopheles sinensis, distribution, biology, bionomics, molecule, ecology and China. The decision tree for the inclusion or exclusion of articles is shown in Figure 1. Only publications reporting biology, bionomics, and molecules of An. sinensis from China were included. Articles submitting a report on morphology, development, reproductive, life cycle, vector competence, larval and adult ecology, vector capacity, molecules involved in physiology and pathology were included. Studies involving insecticides were excluded because it would be the focus of a future review. These data were extracted and processed through a series of rigorous checking procedures before classification into a database. All results were initially reviewed for mosquito bionomics (larval and adult ecology), biology and related molecular studies based on the title and abstract. Relevant publications were further reviewed using the full text to determine whether the data focused on distribution, bionomics, epidemiology, vector competence, or identified molecules.
FIGURE 1. Flow diagram of the selection procedure used for the systematic review of accessible articles.
Figure 1 illustrates the search results. The initial search strategy generated 1469 records. After first round review of titles and abstracts, a total of 824 articles were amalgamated for review. After removing 44 duplicates, 722 relevant publications were further reviewed based on the full text to find out whether primary or secondary data on the biology, bionomics, molecules. Finally, a total of 538 papers and reports met these criteria. Selected articles were saved in Endnote and their characteristics corresponding to the criteria manually entered into Microsoft Excel for ongoing data management. From these articles, we assessed risk of bias for included studies but did not exclude studies on the basis. The outcomes of analysis by study area are outlined below.
Anopheles sinensis Wiedemann (1828) is a member of the Anopheles hyrcanus species group. Adults are morphologically distinguished from sibling species by the presence of four pale bands on the palpi, a fringe spot at vein 5.2, a tuft of dark scales on the clypeus on each side in the female (Figure 2) and a T-type speckle on ventral aspect (Feng and Zhang, 1962; Yang et al., 1994). Morphometric and morphological characteristics of An. sinensis eggs were studied using scanning electron microscopy (Zhang et al., 1982, 1984; Li D. et al., 2010). Eggs of An. sinensis were black (newly laid eggs were white and blackened about an hour later (Liu, 1986). They were boat-shaped in lateral, view with a mean length of 0.5mm. Floats present on sides of the egg surface and these had 7∼10 ribs.
FIGURE 2. Typical morphological characteristics of An. sinensis. (A) A pale fringe spot at vein 5.2. (B) Four pale bands on the palpi. (C) Patch of pale scales at midcoxa. (D) Apical pale bands at hindtarsomeres.
Like all mosquitoes, An. sinensis has four life stages: egg, larva, pupa, and adult. An. sinensis female lay individual egg on water surface. According to the studies, the number of eggs laid ranges from dozens to hundreds (Lu, 1984; Li et al., 1994; Qu et al., 2000i; Zhong, 2000; Zhong and Tan, 2000). The number of eggs laid were affected by the season (Qu et al., 2000i), temperature (Zhong, 2000; Zhong and Tan, 2000), blood resources (Su and Su, 1989), and experimental factors (Luo et al., 1988; Li and Tang, 2010). Luo et al. (1988) noted that the number of eggs laid in cow sheds was greater than in human dwellings and speculated this might be an adaption related to blood preference. Lu (1984) observed that most female laid eggs during entire night, and were prone to oviposit between dusk and dawn (usually from 7 pm to 5 am). The result was consistent with studies in other provinces of China (Liu, 1986; Xiang, 1988; Qu et al., 2000d,i). Generally the female adults began to lay eggs in around 3 days (2.57–3 days) after blood feeding, and its peaks started from 5 to 16 days (Fengwen et al., 1988; Qu et al., 2000a) in July and August (Qu et al., 2000d,i). Interestingly, in An. gambiae s.s., Christiansenjucht et al. (2015) observed temperature had effect on the egg laying, the number of eggs laid was highest and lowest when adults were kept at 27°C and 31°C respectively. Differences were also observed among the successive blood meals in times to egg laying and hatching, number of eggs laid, and chances of feeding and egg laying.
Embryogenesis and hatching have been described in detail by different research teams (Wang et al., 1985; Li et al., 1994; Shen et al., 1995; Lai et al., 1995; Qu et al., 2000e). Generally it takes around 2.07–2.88 days (mean) or more for full embryonic development depending on the temperature and environment (Fengwen et al., 1988; Qu et al., 2000a; Zhong, 2000; Zhong and Tan, 2000). Hu et al. (1986) observed that the optimal temperature was between 25 and 28°C. At 25–30°C, An. sinensis hatched at 2.9 d after oviposition. At 19°C and 22°C, hatch took 6.4 and 6.7 days respectively, and below 16°C, embryonic development of the An. sinensis cannot be completed. Hatching rates ranged from 63.68 to 90.88% (Wang et al., 1985; Li et al., 1994; Lai et al., 1995; Shen et al., 1995; Qu et al., 2000e) in different provinces in China. Hatching rates were lower under natural conditions compared to under experimental conditions, and there could be diapause in embryogenesis due to the low temperature (Zhao and Zhen, 1997). Larval breeding environments largely depended on the sites where females laid their eggs, and the oviposition locations were not stringent. The environment had no effect on the sex ratio (Zhou et al., 1988). The sex ratio was very close to 1:1 which meant that the natural quantity of males and females was equal.
The life cycle duration from the egg to the adult was a popular research topic. Eight studies between 1984 and 2000, indicated that the average duration of the life cycle ranged from 14 to 20 days (Wang et al., 1985; Zhu, 1989; Xu L. et al., 1991; Xu R. et al., 1991; Lai et al., 1995; Shen et al., 1995; Qu et al., 2000d,e). The development time also varied in different studies conducted in different regions of China. The longest time was 20.9 days in the Guizhou province (Wang et al., 1985), and the shortest time was 13.74 days occurred in the Henan province (Qu et al., 2000d). The time required for the development of An. sinensis was temperature dependent. Wang et al. (2010) documented a shortened duration as the temperature increased from 16 to 31°C. The life cycle duration were 30.7, 23.3, 15.5, 13.5, and 12.5 days at 19°C, 22°C, 25°C, 28°C, and 31°C respectively. An. sinensis can complete their development at temperatures as low as 16°C (Sun et al., 1994), but cannot develop successfully at temperatures > 31°C. In contrast, An. gambiae s.s. development was fastest between 28 and 32°C; adults did not emerged at <18°C or >34°C (Kirby and Lindsay, 2009). Development time from egg to adult was also largely temperature-dependent.
After completion of larval development, the pupation rate was generally high except in one study. Shen et al. (1995) documented a pupation rate of only 21.28–33.68%. Li et al. (1994) found that the larvae stage needed 8.16 days to develop and the mean duration for female larvae (8.54 ± 1.49) was longer than for males (7.78 ± 0.96). The larval stage fed on organic debris and microorganisms in the water including bacteria, protozoa, pollen grains, and fungal spores (Yuan et al., 1988). The distribution of young larvae in the water was similar in different rice field locations (Chen, 1990). The strong clustering habit of larvae weakened as they were growing, spreading from the center to around (Chen et al., 1990). Larval growth was influenced by many factors such as food types, food quantity, and larval density, etc. (Dong and Liu, 1990). Xu D. et al. (1988) indicated that development would be reduced if the larval density was too great.
Larvae of An. sinensis can successfully develop under a wide range of temperatures, whereas low winter temperature (usually after October in China) restricts the development of An. sinensis (Ding et al., 1991; Wu et al., 2005). In addition, Lai et al. (1995) noted that the time required for larvae development correlated with the time required for embryogenesis and hatching. If the time required for embryogenesis and hatching increased, the length of time required for larval development would decrease accordingly to keep the life cycle duration in balance of about 14 days, and vice versa (Lai et al., 1995).
The larval molted their exoskeleton four times before becoming pupae. An. sinensis remain in the pupal phase for about 2 days until the adults emerge and disperse to seek blood or nectar (Pan et al., 1984; Wang et al., 1985; Fengwen et al., 1988; Xu L. et al., 1988; Xu R. et al., 1991; Shen et al., 1995; Qu et al., 2000a). The eclosion rate from all the studies was higher than the hatching rate and the pupation rate (Shen et al., 1995; Zhong and Tan, 2000), indicating that death in premature stage mostly occurred during the larval stage. Compared to the high mortality during the larval stage, survival was higher in the pupal stage and during eclosion (Qu et al., 2000g,h). Like other mosquito species, the An. sinensis males often emerge first and form swarms, they cannot copulate until the genitalia rotate 180° which occurs in about 1 day. Females emerge subsequently, and then enter swarms to copulate in the air (Zhu, 1989).
An. sinensis is holometabolous insect and has four different developmental stages. The developmental parameters, such as number of eggs laid, hatching rate, pupation rate, and duration of the life cycle, can influence malaria transmission. A detailed understanding of An. sinensis biology pertaining to development could help in generating novel control strategies. Investigation of An. sinensis development may reveal mosquito-specific adaptations and could provide stage-specific targets for mosquito-borne infectious disease control.
The life expectancy of An. sinensis under natural condition was 5–7 days (Xu et al., 1987; Zhang et al., 1990; Qu et al., 2000e), which was shorter than the values measured under experimental conditions (Zhou et al., 1995) in which the constant nectar and blood were available. Female and male An. sinensis from different locations in China exhibited a significant difference in life expectancy. Qu et al. (2000a) observed average female and male An. sinensis life expectancies of 13.83 and 8.33 days, and maximum longevity was 32 and 14 days respectively (Qu et al., 2000f,i). Zhou et al. (1995) observed mean female and male life expectancies of 21.63 and 17.51 days, and maximum longevity was 51 and 46 days respectively. The results of the studies on An. sinensis life expectancy between female and male in different provinces indicated that the life expectancy of females exceeded that of males. In addition, temperature had a significant influence on the life expectancy of adult mosquitoes (Zhang et al., 1990; Xu L. et al., 1991). The average life expectancy in July was longer than that in August because the temperature in August was often higher than that in July (Qu et al., 2000d). In addition, Hu et al. (1988) showed that the average life expectancy was 48.6, 27.4, 23, 20.6, 14.8, and 11.1 days at 16°C, 19°C, 22°C, 25°C, 28°C, and 31°C. The longest life expectancy was at 16°C where individuals had very low metabolic rates (Pan et al., 1984; Hu et al., 1986).
The mean gonotrophic cycle length for An. sinensis was 2.5 days (Liu, 1986; Xu et al., 1987, 1990; Wang et al., 1990; Li et al., 1994; Shi et al., 1996; Qu et al., 2000i). The shortest gonotrophic cycle for An. sinensis was 48 h (Wang et al., 1990) in Shanghai, while the longest was 2.65 days (Xu et al., 1987) in Fujian Province. The gonotrophic cycle for the different strains (Shanghai, Zhengzhou and Fujian strains) were similar (Qu et al., 2000b), but varied slightly related to the month (Zhang et al., 1990; Qu et al., 2000d), blood source (Su and Su, 1989), temperature (Xu and Zhang, 1988) and sunlight (Sun et al., 1987).
Generally, An. sinensis begin to appear in late April or May and disappear in October. When the temperature related to the about 10°C, An. sinensis tended to seek sheltered places for hibernation. On the basis of studies on the hibernation of An. sinensis (Ma, 1954), together with studies conducted in Zhejiang, Henna, Hubei, and Jiangsu provinces etc. (Liu and Chen, 1959; You et al., 1964; Xue et al., 1990), An. sinensis hibernates in the adult stage. Both male and female adults were caught in cow sheds, mountain caves, and cellars. The mosquitoes caught in hibernation sites were found to be nulliparous and have sperm in the spermathecae. Much body fat accumulated during the winter months. In northern China, An. sinensis females hibernated in sheltered places from the end of October onward (Anonymous, 1973b; Chen et al., 1979). When the weather warmed the following spring, the hibernating mosquitos became active and fly off for the new life cycle (Anonymous, 1979).
Anopheles sinensis has limited dispersal with most adults staying close to their larval sites or habitats. Dispersal distances are generally less than 1 km, but longer passive dispersal by planes, ships, or other human devices could occur. There were relatively few studies on adult flight range or dispersal. Liu et al. (2011) studied the dispersal range of An. sinensis using a mark-release-recapture technique. They marked 3000 wild An. sinensis and recaptured mosquitos for ten successive days using light traps. The recapture rate was very low and most marked An. sinensis were recaptured within a 100 m radius of the release site. The maximum flight distance was 400 m.
Anopheles sinensis has been recorded from Afghanistan, Myanmar, Cambodia, China, Indonesia, India, Japan, Korea, Malaysia, Singapore, Thailand, and Vietnam (Reid, 1953). It is distributed in 29 provinces within China and all regions except Xinjiang and Qinghai provinces (Lu et al., 1997). Xu and Feng (1975) published the first national distribution of the Anopheles hyrcanus complex in China including An. sinensis. The report primarily consisted of a brief survey of egg, larval, pupa and adult stages around the surveyed sites during 1962–1965. The results were limited to a short description of the main bionomic characteristics.
In the present review, an An. sinensis distribution map was produced by overlaying occurrence data from 332 source collected reports. An. sinensis was the mosquito taxon most commonly found and identified in China. However, this study did not document an extension to its previous distribution range. An. sinensis were found in 29 provinces and regions (Figure 3A) consistent with historical records. According to the analysis, An. sinensis has been recorded both on mainland (Chen, 1990; Pan et al., 2012) and on island areas (Figure 3B) (Zhang et al., 2001), and it was widespread across the plain areas and mountainous areas but more abundant in plain areas (Huang et al., 2015). An. sinensis was collected at 270 up to 2,300 m in elevation, but is generally restricted to elevations of 300–500 m (Liu et al., 1989). A more accurate, or a lower level distribution map is currently unavailable and the data currently consist of heterogeneous records that were not made on a national scale.
FIGURE 3. Distribution maps of An. sinensis in China. (A) Distribution of An. sinensis at province level. (B) Distribution of An. sinensis at county level based on occurrence data. Points have been georeferenced and digitized from publication maps using current departmental base map of China.
In almost all areas in China, An. sinensis was found from July to December with a population peak in August. The population density of An. sinensis in cow sheds had a June peak in most places with a second small peak in late August or September. In Heilongjiang, Jilin, Liaoning and Xizang, An. sinensis was recorded only in August and September after which no specimens were found due to cold weather. In some warmer provinces like Yunnan, active An. sinensis were present in December but in relatively small numbers. In general, the collection data showed the seasonal abundance of An. sinensis fluctuated monthly (temperature-driven model) at various study sites during different study periods. An. sinensis emerged in April, developed into large populations from July to December with a peak in August.
Anopheles sinensis larvae were found in diverse habitats. Examples of major habitats include rice-fields and ponds (Liu et al., 1962). The larvae were also collected in irrigation channels, abandoned wells, ground pools, and pools beside rivers, marshes, stream margins, ditches, seepages, shallow ponds, sumps, hoof footprints, and wheel tracks. The larvae have also been found in polluted pools and cesspits (Cai, 1959). Environmental factors associated with larval habitats have been studied. The available data (Anonymous, 1973b; You and Xu, 1979; Chen, 1990) observed that the breeding sites of An. sinensis were located within 50–200 m from irrigation wells, human or livestock settings, and the larvae were most often found in water bodies with abundant aquatic vegetation. Physicochemical properties tests of habitats showed that An. sinensis larvae had high adaptability to variable water quality (Wang, 1979).
The preferred indoor habitats (resting habits) for An. sinensis were mainly cow sheds, pigpens, sheepfolds and human dwellings (Cai, 1959; Ouyang et al., 1992; Yi et al., 1998), especially when they are seeking hosts for blood meals. About 61% of resting An. sinensis was captured from cow sheds, 37.7% from human dwellings, and 0.74% from spare houses (Cai, 1959). A report from Jaingsu province found that the average number of An. sinensis in livestock sheds was 49 times greater than that in human dwellings (Gao et al., 2004). Both studies found a higher tendency for resting mainly in cow sheds, although other resting places can be selected.
Outdoor habitats for An. sinensis were mainly grass or leaves growing near or along rice fields and streams when they are not actively seeking hosts or oviposition habitats. Typical plants were rice near permanent water or subject to regular irrigation, or sweet potatoes, vegetables, shrubs and weeds around human residences and livestock sheds. Other potential outdoor resting sites were soil cave and ravines with damp, dark and humid environments (He et al., 1962). Most An. sinensis rested at heights of 5–15 cm above the ground on the trunk of a plant or under leaves.
The habitats for An. sinensis varied among the different regions, and were under the influence of the local biotope. There was evidence for various habitats with diverse geography. For instance, An. sinensis on Hainan Island tended to rest on the grass or other nearby vegetation after a blood meal and disperse before dawn, perhaps seeking more secluded places to rest. An. sinensis in Guangdong, Guangxi, and Shanghai were more likely to stay in structure for a period after feeding, usually a human house or cow shed (Liu, 1962). This difference may be the result of different natural environments in these regions. For example, Hainan Island has a tropical climate and dense vegetation. In contrast, there is relatively less vegetation in other regions but they have more buildings with solid walls. The perennial impact of the external environment may have altered feeding habits.
The factors affecting An. sinensis habitat were more complicated. Despite clear evidence that the longitude and latitude have an influence on An. sinensis habitats, other factors such as temperature, rainfall, human activities are also involved. Human and animal activity seemed to play a critical role in habitat selection. Local residents sleeping outdoors, degree of vegetation cover, and grazing habits of livestock were all important in habitat preferences of An. sinensis.
Female An. sinensis fed throughout the night but were most active from sunset to midnight. Peaking feeding activity apparently occurred at different times depending on locality and habitats. Cai (1959) showed that An. sinensis in human dwellings fed from dusk to dawn, with two peaks one between 8:00 pm and 9:00 pm and another at 1:00 am. In cow sheds, only one peak occurred at 8:00 pm. Chen et al. (1979) observed peak activity in Henan province occurring between 8:00 pm to 9:00 pm. Peak activity in Zhejiang province was from 9:00 pm to 10:00 pm (Zhang et al., 1990). Similar peak activity has been recorded in several other areas of China and during different time periods. Considering that these data were somewhat dated, we examined the most recent studies conducted between 2013 and 2014 in Hunan Province (Zhu et al., 2015) and in 2012 in four counties in Yunnan Province (Zhang et al., 2015). By contrast, although the results differed in the bait method, selected localities, weather conditions and other factors, the similar peak activity, either one peak after the sunset (between 8:00 pm and 10:00 pm) or with a second peak before dawn (between 1:00 am and 2:00 am) could be observed in different localities.
Under normal circumstances, An. sinensis females are facultative feeders but relatively more zoophilic. An. sinensis preferred to feed on livestock in the presence of both humans and their preferred animal hosts (buffalo and cattle), and they were prone to be found inside livestock corrals. Proportion tests on blood meals to detect feeding preferences revealed that most An. sinensis were zoophilic. However, the females also fed on the blood of whatever vertebrates are available in the vicinity, and sometimes they readily fed on humans. An. sinensis mostly feed on large animals such as cows, buffalos, pigs, horses, donkeys, mules, and goats. Among them, cows were the most attractive animal. Two studies (Malaria Group, 1984; Qian et al., 1984) between 1979 and 1981 showed that the human blood proportion was 3.52 and 2.60% respectively in captured An. sinensis. Hu et al. (1988) also found a low percentage of human blood (2.90%) in An. sinensis compared with cow blood. So An. sinensisis is facultatively anthropophilic but prefers to be zoophilic.
Malaria parasites must undergo development within the mosquito before they are infectious to humans. An. sinensis is a P. vivax malaria vector in China, Indonesia, Japan, and South Korea (Rueda et al., 2010). In China, Wang et al. (1982) and Qu et al. (2000c) studied P. vivax development in An. sinensis. Within 15–20 min after mosquito ingestion, the male (microgametocytes) and female (macrogametocytes) gametocytes could be detected in the mosquito’s midgut. The fertilization event produced a zygote, then developed into an ookinete in as short as 50 min and fully developed within 26 h. The ookinete traversed the peritrophic membrane of the midgut, crossing the midgut epithelium, entered the basil lamina, and formed an oocyst in 48 h. Over a period of 3–7 days, after oocyst maturation was completed, the oocyst ruptured to release multiple immature sporozoites into the haemolymph. The sporozoites then migrated to the salivary glands in 7–8 days. The Plasmodium vivax cycle begins again when the female mosquito takes a blood meal, injecting the sporozoites from its salivary glands into the human bloodstream.
Vector competence is an indication of mosquitoes ability to obtain a disease agent (microorganism, such as parasite, arbovirus etc.) from a reservoir host and then transmit the infectious agent to another susceptible host (Min et al., 2002). Vector competence for malaria is evaluated by the susceptibility of Anopheline species to malaria parasites and the ability to transmit a susceptible host (Beier, 1998). Generally, malaria vector competence is determined through either observation of sporozoites in the salivary glands of field-caught mosquitoes or infection experiments using laboratory reared mosquitoes. An. sinensis natural infection has been extensively studied (Anonymous, 1973a, 1975; Gou et al., 1998; Zhou et al., 2005) and had sporozoite rates ranging from 0.00 to 0.33% in the salivary glands under microscope. A null sporozoite rate was detected in Hunan, Guangxi, and Shanghai (Malaria Group, 1984; Wang et al., 1987, 1990), a 0.16% rate was found in Pucheng county, Fujian province (Xu et al., 1990), and 0.33% in Xuzhou, Jiangsu province (Qiu and Zhao, 1978). An. sinensis is a confirmed malaria vector in China and has been reported naturally infected with malaria parasites in many provinces. However, the results of published studies by dissecting the salivary gland of field An. sinensis only reported the positive sporozoite rates, but failed to identify the Plasmodium species until the sporozoite ELISA kit was applied.
Experimental infection of An. sinensis against P. vivax has been done in different provinces at different times. Hu et al. (1981) found that An. sinensis oocyst rates (23.66%) were significantly higher than sporozoite rates (6.21%) in Shandong province and determined that 25–28°C was the best temperature range for P. vivax sporozoite multiplication within An. sinensis. Wang et al. (1982) found much higher P. vivax oocyst rates (38.10–95.90%) and sporozoite rates (21.90–50.80%) under experimental condition both in two An. sinensis strains in Henan province. Xiangkun et al. (1991) observed the highest P. vivax oocyst rates and sporozoite rates in the An. sinensis Guizhou strain (81.22–100.00%; 86.36–100.00%) and the Shanghai strain (85.71–100.00%; 91.67–100.00%) under experimental infection respectively. These findings suggest that there can be a large difference in the An. sinensis experimental infection rate depending on both the strain of mosquito and the parasite.
The susceptibility of different An. sinensis strains to a single P. vivax strain, together with the susceptibility of a single An. sinensis strain to different P. vivax strains was studied by Zheng et al. (1986), Xu and Ye (1987), and Zhu (1989). Xu et al. (1987) reported that the susceptibilities of Shanghai and Guangxi An. sinensis strains against the Guangxi P. vivax strain were low (0.00% and 12.3%), but significantly higher against Hainan P. vivax strain (36.40% and 43.60%). Zhu (1989) observed that the susceptibility of the Simao An. sinensis strain against P. vivax was significantly lower compared to the Shanghai An. sinensis strain. In contrast, Zheng et al. (1986) reported similar sporozoite rates in four different An. sinensis strains (Changsha, Shanghai, Zhengzhou, and Wuhan strain) against two P. vivax strains. Vector competence may vary among different An. sinensis strains for different P. vivax strains but the reasons for this are not clear.
In addition to the mosquito infection experiments, the researchers also investigated the correlation of infection rate with the patient status (Shi et al., 1983b; Qu et al., 2000c), parasite density in donor blood (Zheng et al., 1986), long incubation period strains (Xu and Ye, 1987), course of the disease (Zheng et al., 1986; Xu and Ye, 1987), and relapse or recrudesce (Zhu, 1993; Zhu et al., 2007, 2013), etc. Shi et al. (1983b) showed that An. sinensis sporozoite rate of the long incubation period P. vivax strain was higher (61.0%) than that in short incubation period P. vivax strain (33.3%), and infection rate of An. sinensis was correlated with the density and sex ratio of gametocytes. Li et al. (1998) reported that infection rate of An. sinensis for P. vivax under experimental conditions increased with parasite density and stage of the disease. An. sinensis showed a high infection rate in relapse or recrudesce malaria cases. Li et al. (1998) and Zhu et al. (2007) both reported infection rate and oocyst rates of An. sinensis to P. vivax. The blood resources from patients in the fever stage were lower than those in non-fever stage patients. Interestingly, Zhu et al. (2007) found that sporozoite rates were higher in the An. sinensis group fed on fever stage patients in the same study. However, in another study, Zhu et al. (2013) reported that the gametocytemia, asexual parasitemia, and ratio of macrogametocytes to microgametocytes, did not correlate with either oocyst or sporozoite infection, while in the oocyst-positive mosquitoes, there was a correlation between gametocytemia and the average oocyst number.
Anopheles sinensis was refractory to P. falciparum in Thailand (Rongsriyam et al., 1998). How about this situation in China? An. sinensis susceptibility to P. vivax versus P. falciparum in Guangxi province showed average infection rates of 59.80% (oocyst rate) and 12.80% (sporozoite rate) compared to no infection with P. falciparum (Xu et al., 1983). However, studies conducted by Shi et al. (1983a) and Liu et al. (1984) indicated that An. sinensis could be infected with P. falciparum but at a relatively low rate. Shi et al. (1983a) found that P. falciparum oocyst rates ranged from 2.14 to 8.6%, and sporozoite rates ranged from 0.00 to 3.54%. Liu et al. (1984) observed an average P. falciparum oocyst rate of 11.30% and average sporozoite rate of 3.00%. These provide evidence that An. sinensis in China is not totally refractory to P. falciparum. Vector competence of An. sinensis is thought to be significantly higher for P. vivax compared to P. falciparum.
In summary, An. sinensis infection experiments showed variable sporozoite infection rates with P. vivax (6.21–100.00%) and P. falciparum (0.00–3.54%). The presence of a natural infection sporozoite rate together with the relatively high value of both oocyst and sporozoite rates under laboratory infection experiments supports the potentially high vector competence for P. vivax. Although An. sinensis could be infected by P. falciparum in some studies, dissection of salivary glands in field An. sinensis failed to reveal the presence of P. falciparum sporozoites. These data support the conclusion that An. sinensis is not a vector for P. falciparum malaria in China.
Vectorial capacity is a measurement of the efficiency of vector-borne disease transmission. In addition to competence, vector capacity includes a variety of other essential traits, i.e., host preferences, biting rate, mosquito population density, longevity, and etc. The malaria transmission intensity can be characterized as the vectorial capacity (C) formulated by Macdonald (1957) and Garrett-Jones (1964), which is defined as:
where ma is the human biting rate (the number of female mosquitoes per person per night); a is the human-biting frequency per day (daily probability of a human host being bit by a vector, which is indirectly estimated as the human blood index divided by duration of gonotrophic cycle; n is the length of the sporogonic cycle; p is the daily survival rate of the vector.
In China, the An. sinensis vector capacity was measured and compared by investigating of An. sinensis at different times and in different provinces (Table 1). The first and most recent reports of An. sinensis capacity were published in 1983 and 2015, the results showed that VC was 0.24 and 0.15 respectively (Song, 2015). The vector capacities of An. sinensis varied from north to south in China, and were correlated with the season (Yao et al., 2002). Pan et al. (2008) founded that the vector capacity of An. sinensis in Huizhou and Zhuhai, Guangdong province was 0.25 and 0.66, respectively. Zhao et al. (1996) and Yu et al. (2014) reported that the vector capacities of An. sinensis were 0.01 and 0.15 in Sichuan and Shandong province (north), respectively. Differences observed in the vectorial capacity of An. sinensis may be due to variation in ecological habits or genetic background in different localities. The overall low vectorial capacity of An. sinensis may be diminished by tendencies for zoophilic feeding behavior in some study sites. In addition, vectorial capacity of An. sinensis could also serve as an indicator for annual parasite index (API) or malaria prevalence (Yang and Tian, 1994; Liu et al., 1995; Qian et al., 1996).
Vectorial capacity includes a number of factors. Human blood index (HBI) and human biting rate (ma) are important indicators for vector capacity, and would determine the capacity to transmit malaria protozoa. Two studies reported that the vector capacity of An. sinensis was highest in July or August corresponding to the highest human biting rate (Station, 1985; Yao et al., 2002). Another study conducted by Qian et al. (1996) compared the vector capacity of An. sinensis between the 1970s and the 1990s, and found a significant decrease both in human biting rate and vector capacity. In other studies conducted in different provinces a positive correlation between the HBI, human biting rate and vector capacity (Liu et al., 1995; Zhang X. et al., 2006).
Other indicators such as duration of the gonotrophic cycle (GC), length of the sporogonic cycle (n), and the daily survival rate of the vector (p) generally have stable values corresponding to the mosquito species. In different studies, the gonotrophic cycle of An. sinensis in China was 2.5–3.7 days, the length of the sporogonic cycle was 8.5–14.8 days, and the daily survival rate of the vector was 0.77–0.91. Given that the P. vivax incubation time in An. sinensis was of 9–14 days, An. sinensis would require two to three blood meals before becoming infectious agents. In addition, calculation of daily survival rate requires introduction of another parameter–the parity rate. Studies by Gao et al. (2004) found the mean parity rates of An. sinensis samples collected over years from Hunan province to be 42.86%. The parity rates varied in different habitats, months, indoor and outdoor environments, daytime and night, as well as before midnight and after midnight (Wang, 2013). This variation may be attributed to numbers of emerging mosquito, the mortality rate of larvae and sampling methods (Qian et al., 1984).
The vectorial capacity measures efficiency of pathogen transmission, but EIR is a measure of malaria transmission intensity. It is usually interpreted as the number of infectious bites per human during a season or annually (usually annually) and is referred as a more direct way to measure transmission risk. However, although studies (Anonymous, 1975; Liu et al., 1984; Liu et al., 1986; Zhang et al., 1989) have provided estimates of EIR values ranging from 0.000031 to 0.24 (Table 1), which is conventionally computed by taking the product of biting rate multiplied by the sporozoite rate. The data still made it difficult to investigate the accuracy and precision of EIR because sporozoite rates were exceedingly low (<0.19%), while appropriate values ranging from 1 to 20% are generally suggested for EIR determination in Africa.
Scientists have unveiled the genomes of two An. sinensis strains from Korea1 and China (Zhou et al., 2014). The genome of An. sinensis (China strain) was fully sequenced in 2014. An. sinensis has six chromosomes representing two three-chromosome sets. The genome size was estimated 220.78 Mb, coding for 16, 766 genes. Approximately 14% of the putative genes were orthologous with genes in 235 known biological pathways. The genome had 3, 972 gene clusters containing 11,300 genes that were common to the genomes of three previously sequenced mosquito species, An. gambiae, An. aegypti, and Cu. quinquefasciatus. Gene orthology prediction revealed a total of 4,727 orthologous genes were shared among the mosquitoes (Zhou et al., 2014). Analysis of these orthologous genes revealed the most gene-enriched domain and family were peptidase, while KEGG pathway revealed that genes were most enriched in metabolic pathways. The results indicate that functions such as feeding behavior are central to mosquito biology. In addition to protein-coding genes, 41 microRNA, 348 tRNA and 2017 rRNA genes have also been identified.
A comprehensive reference transcriptome of An. sinensis sampled from different developmental stages of egg, larva, pupa, and adult was sequenced by Chen et al. (2014). Approximately 51.6 million clean reads were obtained, and these were assembled into 38,504 unigenes. Among them, 98.4% (37,884/38,504) of unigenes could be mapped onto the An. sinensis reference genome, and 69% (26,650) of the unigenes could be annotated with known biological functions. In addition, a total of 8,057 expressed sequence tags (ESTs) were assigned to GO and KEGG annotation. A large number of ESTs were restricted to metabolic pathways, biosynthesis of secondary metabolites, and microbial metabolism. The study also found that 2,131 ESTs were differentially expressed between deltamethrin resistant and deltamethrin susceptible mosquitoes collected from the same field site. Further, more than 2,400 microsatellite markers have also been identified (Zhu et al., 2014). These studies would definitely enhance knowledge on the An. sinensis and lay an important foundation for further functional analysis so as to provide new tools for future malaria vectors control.
A compendium of molecules are involved the interaction of the Plasmodium parasites within their host vectors (Sreenivasamurthy et al., 2013), however, little is known about the analogous process in An. sinensis. The first gene of An. sinensis was studied by Zhi et al. (2002) who attempted to clone the defensin gene (immune gene) from the main mosquito vectors of China. Sequence analysis showed that the amplified fragments from An. sinensis were homologous to the reported defensin sequence of An. gambiae. Since then, newer research has focused on cloning the defensin gene, prokaryotic expression and the product activity (Yan et al., 2005; Zheng et al., 2005). The complete defensin gene was cloned by Zhang Y.J. et al. (2006). It has a total length of 2,256 bp, including the 5′ and 3′ UTR fractions and two exons separated by an 85 bp intron. The entire cDNA sequence of An. sinensis defensin gene was 324 bp; its ORF encoded 107 amino acids, and mature peptide had 40 amino acids residues. Then, another immune gene, partial SRPN14 gene of An. sinensis, was identified and characterized (Feng et al., 2015). The SRPN14 gene was found to be located on 2L: 23C of salivary gland chromosomes of An. sinensis by in situ hybridization, which had 77% (nt) and 88% (aa) similarities with An. gambiae. Several mosquito proteins have been involved in regulating the maturation of malaria parasites. For example, HSP40 was isolated from P. yoelii infected An. sinensis and its full-length cDNA of 1,159 bp with an ORF of 1,014 bp, encoding 337 amino acids was amplified (Li Y. et al., 2010). An analysis of gene expression throughout development has been conducted for An. gambiae (Strode et al., 2006). In An. sinensis, several important homologous genes have been identified (Table 2). Liu B.Q. et al. (2016) found that there were four CPF (cuticular protein family) gene families in An. sinensis genome. Analysis of these CPFs in An. sinensis revealed that the full-length cDNA sequences ranged from 531 to 1,001 bp and coded for 148 to 345 amino acids. These AsCPFs contained a 44-amino-acid conserved region and a C-terminal region, which were secretory proteins with signal peptide sequences except for AsCPF2 that was a non-secretory protein and lacked a signal peptide sequence but contained a transmembrane region. Qiao et al. (2016) studied on the role of expression and regulation of TH (the initial enzyme in the melanin pathway) on specific physiological processes during mosquito development by silencing of AsTH. Significant disruption of cuticle in experiments strongly suggested that TH was essential for pupae tanning and immunity in Anopheles.
In addition, AQPs (water channels) are integral membrane proteins in biological cells. Some AQPs were abundantly expressed in Malpighian tubules of An. gambiae, and reduced expression could increase mosquito survival in dry environments (Liu K. et al., 2016). Tang et al. (2012) identified a full-length cDNA of AQP from An. sinensis, which consisted of 762 bp coding for 253 amino acids, with a predicted molecular mass of 63.2 kD. The AsAQP shared 76 and 78% identities with AQPs of Cu. quinquefasciatus and Ae. aegypti AQPs, respectively. To analyze the differential expression of iron responsive element binding protein genes in different strains of An. sinensis, Wang et al. (2015) identified partial sequences of IRE_BP1 by two-dimensional electrophoresis followed by corresponding PCR applications. They found that the IRE_BP1 gene expression in resistant An. sinensis strains was 9.42 times than that of susceptible strains by fluorescence quantitative PCR. The IRE_BP1 may be useful as a resistance control target and gene detection indicator.
The recently published An. sinensis genome and transcriptome provide an opportunity for advanced study of gene products. Based on the comparison between OBP conserved motifs with An. gambiae, He et al. (2016) identified 64 putative odorant-binding protein genes (OBP) in the genome of An. sinensis. The authors also investigated motif conservation, gene structure, genomic organization and classification. An. sinensis OBP genes were classified into three subfamilies, some genes might have originated from a single gene through a series of historic duplication events. Zhou et al. (2015) found 174 detoxification genes by analyzing An. sinensis genome, including 93 cytochrome P450s (P450s), 31 GSTs, and 50 choline/carboxylesterases (CCEs). Based on a combination analysis of available An. sinensis transcriptome, several candidate genes overexpressed in a deltamethrin-resistant strain were identified as belonging to the CYP4 or CYP6 family of P450s and the Delta GST class. Che et al. (2014) identified CYP6P5 gene in An. sinensis and analyzed its bioinformatics characteristics. The entire sequence was 1, 583 bp long with an ORF of 1, 527 bp, encoding 508 amino acids. Phylogenetic and similarity analyses of amino acid sequences showed that An. sinensis CYP6P5 had the closest phylogenetic relationship to the CYP6P5s of An. funestus and An. gambiae, with similarity values of 89.4 and 89.0%, respectively.
Although, the current literature on molecular biology was systematically reviewed, significant gaps in knowledge of An. sinensis basic biology remain. For instance, recent advances in molecular biology have resulted in development of a genetically modified mosquito species for the purpose of population control (Lounibos, 2004; Christophides, 2005). More inspiringly, mosquito gut microbiota have emerged as a novel target to modulate homeostasis in greater depth and to develop new paradigms for disease transmission control (Rodgers et al., 2017). Therefore, studies on vectors and their molecular biology remain important to develop possible applications for more effective vector control.
This report updates available knowledge on An. sinensis and characterizes biology, bionomics and molecules research in China through an extensive review gathered in the country from 1954 to 2016. Most of the literatures on biology and bionomics were during the early time of high incidence periods in China. In contrast, there was little recent entomology research focused on the ecology and life history study in recent years. Research conducted before 1970 was generally limited and sporadic. Entomological research increased following two events. One event occurred in 1985 and it was the start of a nationwide malaria control program activated by two decades of outbreak and pandemic transmission between 1960 and 1979 (Yin et al., 2014). Another event started in 2003 after GFATM began to support malaria control campaign in the People’s Republic of China (Wang et al., 2014). The first molecular study on An. sinensis was published in 2002, but there are still few publications on gene functions and molecular mechanisms, despite a large numbers of molecules being found using omics tools.
A detailed summary of biology information on An. sinensis was presented and represents the accumulation of many years’ studies in China. Before molecular tools were developed, the identification of An. sinensis and its sibling species depended on morphological characteristics (Reidenbach et al., 2009), which were sometimes undependable and likely to misidentify sibling species. For instance, a narrow-ovate form of An. sinensis was first described as a new species but later identified as Anopheles lesteri (Makhawi et al., 2013). PCR assays based on molecular markers have been developed to accurately distinguish in this species complex (Fanello et al., 2002) in 1990s. An. sinensis has been successfully identified from Anopheles hyrcanus complex based on specific DNA nucleotide differences in the sequences of the second internal transcribed spacer (ITS2) of the ribosomal DNA (rDNA) (Ma and Xu, 2005). Morphological characteristics together with nucleotide differences have enabled accurate identification of An. sinensis from Anopheles hyrcanus complex, which is essential for more precise knowledge of this species.
Anopheles sinensis has a complex life cycle. Current knowledge of the An. sinensis life cycle is basically complete and sustained by extensive field investigations and laboratory rearing experiments. Most egg laying of An. sinensis occurs at the night following digestion of a blood meal, however, exceptions to this behavior have also been noted. For example, egg laying is sometimes observed in the daytime (Yusheng and Xingbang, 1958). The timing and amounts of oviposition depend on local conditions, blood-feeding, and the season. Various experimental factors, such as photoperiod, substrate and bacteria, were also involved (Zhao et al., 1991; Pan et al., 1993; Li and Tang, 2010). Oviposition time and location may have important consequences for vector population dynamics and epidemiology.
Knowledge of the different stages of An. sinensis should enhance the efficacy of control strategies. An. sinensis larvae lie horizontally at the water surface and feed on microorganisms, algae, protozoa, and organic detritus. The rate of larval development is temperature dependent and correlated to the time required for embryogenesis and hatch. The pupa stage usually lasted about 2 days, and this may be extended or shortened related to temperature. The survival rate of An. sinensis pupae was highest compared to the other development stages. There was a difference between male and female sexual maturity at the time of emergence. Males usually emerge 1∼2 days before the females. An. sinensis has a limited flight range of 1 km; the species tend to stay near their breeding sites. Marked-release-recapture experiments indicate that they normally do not migrate long distances.
Studies on male and female An. sinensis life expectancy among different geographical strains indicated that male life expectancy was shorter than female. These results were consistent with the results of Suleman’s and Suman’s on Cu. quinquefasciatus. Shorter male life span may be a kind of biological rule for male and female mosquitoes. High temperatures and low humidity can also reduce An. sinensis longevity while sufficient food supply can extend the longevity, a result that is especially obvious under experimental conditions. An. sinensis overwinter as adult females. Usually, the females use the lipid reserves for overwintering survival in protected shelters when the temperature is less than 10°C.
Several studies described the complexity of P. vivax development in An. sinensis. The P. vivax gametocytes completed fertilization soon after mosquito blood-feeding on an infected host. They transformed to ookinetes and oocysts, and began to invade the salivary glands at 7∼8 days. Under experimental conditions, the sporozoite rates were lower than the oocyst rates (Wang et al., 1982; Qu et al., 2000c). Sporozoite rates were positively correlated with oocyst rates, and different An. sinensis strains showed different sporozoite rates and oocyst rates (Wang et al., 1982). The mean duration of the gonotrophic cycle for An. sinensis was 2.6 days. Assuming a P. vivax incubation time in mosquitoes of 7–8 days, An. sinensis would require three to four blood meals before being capable of transmitting sporozoites.
In the context of malaria elimination, the value of understanding the bionomics of An. sinensis and its role on malaria transmission is critical for malaria reduction. Distribution maps of An. sinensis in China were produced by combining current knowledge of An. sinensis distribution, and occurrence data from open access, and published papers. The occurrence data resulted in the collation of An. sinensis occurrence from 343 records across 29 provinces. The map was limited by the available data and bias sampling on original data acquisition. The maps can still serve as an accurate representation of the ranges of An. sinensis in China. However, to increase knowledge of the biology and bionomics of the mosquitoe in China, it is important to extend entomological surveys to unexplored northern regions which may represent its northern distribution boundary. A better understanding of the full distribution range of An. sinensis and maps which could predict the distribution trend by combining climate change models is highly desirable.
Field studies on larval presence of in water bodies indicate that An. sinensis can select a wide range of water bodies in which to lay their eggs. These studies implied that larvae habitats were heterogeneous in form, space, and physical features. Indoor and outdoor habitats for adult An. sinensis were also very heterogeneous. This could be partially explained by the differences in the investigation periods and the methods used in An. sinensis collection, such as larval collection tools, light traps, and human baited trap.
Adult An. sinensis have been incriminated as a natural and experimental malaria (P. vivax) vector in China, Indonesia, Japan, and South Korea (Ree, 2005). The known published data on An. sinensis host selection in China indicated that the degree of anthropophily of An. sinensis was low, and it was generally concluded that An. sinensis is not an effective malaria vector, especially in northern China. However, since 1960, uninterrupted malaria cases have occurred in central China, where An. sinensis is the only vector in most areas, thus it is difficult to explain why An. sinensisis appears to be an effective malaria vector in these areas. According to a study in Henan province, An. sinensis not only fed on human blood, but the biting frequency was higher and natural infection of P. vavix was also present. The evidence indicated that An. sinensis served as the major vector for endemic malaria in these regions. This suggests that malaria transmission competence differs from south to north, and this is also supported by variable vector capacity. It was concluded that the vivax malaria transmission ability of An. sinensis has probably been underestimated in central China (Pan et al., 2012). In addition, the competence of An. sinensis (laboratory strain) to P. vivax was similar to An. anthropophagus when evaluated by a membrane feeding assay under experimental conditions (Zhu et al., 2013). The overall low vectorial capacity of An. sinensis may be related to tendencies for zoophilic feeding behavior in some study sites. Interestingly, the enhancement in vector capacity of An. sinensis was attributed to the local resident habits and the decline in the number of large livestock leading to the reduction of the traditional biological barriers (Pan et al., 2012). Vector capacity of An. sinensis can also depend on other factors, such as the larval environment (Moller-Jacobs et al., 2014).
Different An. sinensis populations in China exhibit variability in morphology, chromosomes, ecology, vector capacity, mitochondrial DNA, and even entire genomic DNA composition (Feng et al., 2017). Understanding the molecular and genetic mechanisms that determine variability in transmission efficiency and mosquito susceptibility could aid in the development of novel vector control strategies. A draft genome and transcriptome are currently available and many genes, including small RNA genes have also been identified. However, only 12 genes have been experimentally studied. This is a huge knowledge gap compared to the detailed analysis of variation in gene expression throughout development or related to pathogen infections which have been studied in other mosquito species. Approximately 25% of the genes showed a complex pattern of changes in gene expression during the different life stages of An. gambiae (Strode et al., 2006), and a total of 94 molecules pertaining to parasite infection have been validated (Sreenivasamurthy et al., 2013). Attention also should be focused on the new research directions such as gut-microbiome–parasite interactions, genetics of mosquito behavior, epigenetics and non-coding RNA. Identified molecules will provide useful tools for further functional analysis of the genetic, ecological and immune aspects. Determining the interactions and employing these as efficient resources for malaria intervention will require more research.
As China moves toward malaria elimination, it will be necessary to continuously update and summarize An. sinensis vector research. Climate and environmental landscapes continue to change and appropriate entomological surveillance and evaluation will alert researchers to biological and bionomic changes. The value of applying new tools generated from molecular studies of An. sinensis malaria transmission could be highly significant.
This review provides current information on biology, bionomics, and molecules relevant to An. sinensis in China. Traditional research has provided a wealth of information on An. sinensis biology and bionomics. However, there is a lack of quantitative information required to characterize mechanisms of physiology and developmental biology and interactions with parasites. Future studies should fill these knowledge gaps. An integrated understanding of biology, bionomics, and molecules may yield more effective control strategies to facilitate malaria elimination in China.
XF, ShaZ, WH, and ShuZ were involved in the conception of the idea. XF and ShaZ conducted the literature searches. XF and WH participated in writing the final manuscript. ShuZ, FH, LZ, JF, HZ, and ZX reviewed and analyzed the documents. All authors read and approved the final manuscript.
This work was supported by the National Natural Science Foundation of China (No.81271867 and 91431104), International Science and Technology Cooperation Program of China (No.2014DFA31130) and National Science and Technology Major Program (No.2009ZX10004-302).
The authors declare that the research was conducted in the absence of any commercial or financial relationships that could be construed as a potential conflict of interest.
The reviewer MDE-G and handling Editor declared their shared affiliation, and the handling Editor states that the process nevertheless met the standards of a fair and objective review.
aa, amino acid; Ae. Aegypti, Aedes aegypti; An. Gambiae, Anopheles gambia; An. Sinensis, Anopheles sinensis; AQP, aquaporin; CCEs, carboxylesterases; cDNA, complementary DNA CPF; CPF, cuticular protein family; Cu. Fatigans, Culex fatigans; EIR, entomological inoculation rate; GFATM, The Global Fund to Fight AIDS, Tuberculosis and Malaria; GO, gene ontology; GSTs, glutathione-S-transferases; HSP40, heat shock protein 40; IRE-BP1, Iron responsive element binding protein 1; IRS, indoor residual spraying; ITS2, Internal transcribed spacer 2 capacity; JEV, Japanese encephalitis virus; KEGG, Kyoto Encyclopedia of Genes and Genomes; LLINs, long-lasting insecticidal nets; miRNAs, microRNAs; NGS, Next Generation Sequencing; nt, nucleotide; OBP, odorant-binding proteins; ORF, open reading frame; P. f, Plasmodium falciparum; P. v, Plasmodium vivax; PCR, polymerase chain reaction; sRNAs, small RNAs; SRPN14, serine protease inhibitor 14; TH, tyrosine hydroxylase; UTR, Untranslated Regions; VC, vector competence; WHO, World Health Organization.
Anonymous (1973a). Investigation on natural infection rate of Anopheles sinensis in Henan province. Heinan J. Prev. Med. 1.
Anonymous (1973b). The study on activity of Anopheles sinensis in early spring in Luyi county, Henan province. Henan J. Prev. Med. 1–5.
Anonymous (1975). Investigation on malaria transmission role of Anopheles sinensis in Shangqiu, Henan province. Bull. Med. Res. 21–24.
Beier, J. C. (1998). Malaria parasite development in mosquitoes. Annu. Rev. Entomol. 43, 519–543. doi: 10.1146/annurev.ento.43.1.519
Cai, H. (1959). Malayan filariasis vector invesigation in Shucheng county, Anhui province. J. Anhui Med. Univ. 6, 163–168.
Che, Y., Zhang, Y., Tang, Y., Hong, R., and Chen, B. (2014). Bioinformatics identification and characteristics of cyp65 gene in Anopheles sinensis. Chin. J. Vector Biol. Control 25, 205–210.
Chen, B., Zhang, Y. J., He, Z., Li, W., Si, F., Tang, Y., et al. (2014). De novo transcriptome sequencing and sequence analysis of the malaria vector Anopheles sinensis (Diptera: Culicidae). Parasit Vectors 7:314. doi: 10.1186/1756-3305-7-314
Chen, H., Shi, J., Deng, M., and Bai, Y. (1979). Investigation on ecological habit of Anopheles sinensis in Feng Qiu region. Heinan J. Prev. Med. 54–58.
Chen, J. (1990). Study on the spatial distribution patterns of Anopheles Sinensis Larvae in Paddy Fields. Chin. J. Vector Biol. Control 1, 202–205.
Chen, J., He, X., Ye, L., and Zhang, S. (1990). Study on the distribution of Anopheles sinensis larvae in paddy fields. Chin. J. Vector Biol. Control 1, 132–134.
Christiansenjucht, C. D., Parham, P. E., Saddler, A., Koella, J. C., and Basáñez, M. G. (2015). Larval and adult environmental temperatures influence the adult reproductive traits of Anopheles gambiae s.s. Parasit. Vectors 8, 1–12. doi: 10.1186/s13071-015-1053-5
Christophides, G. K. (2005). Transgenic mosquitoes and malaria transmission. Cell Microbiol. 7, 325–333. doi: 10.1111/j.1462-5822.2005.00495.x
Ding, D., Zhang, X., and Zhao, Y. (1991). Influence of temperature on the generational distribution of Anopheles sinensis and its effective seasone for malaria transmission in China. Chin. J. Ecol. 10, 52–57. doi: 10.13292/j.1000-4890.1991.0030
Dong, Y., and Liu, Q. (1990). “Studies on Anopheles sinensis larvea cultivation,” in Proceedings of the 40th Conference on the Beijing Society of Insects, Beijing.
Fanello, C., Santolamazza, F., and Torre, A. D. (2002). Simultaneous identification of species and molecular forms of the Anopheles gambiae complex by PCR-RFLP. Med. Vet. Entomol. 16, 461–464. doi: 10.1046/j.1365-2915.2002.00393.x
Feng, L., and Zhang, P. (1962). “Preliminary research on the relationship between Anopheles sinensis and malaria, filariasis in Guizhou province,” in Proceedings of the Conference on the Chinese Society of Insects, Guiyang.
Feng, S., Tang, S., Zhiyong, S., Xu, Z., Xu, B., Tan, K., et al. (2002). Field-based quantitative study on feeding habit and malaria transmission effect of Anopheles sinensis and Anopheles anthropophagus. Pract. Prevent. Med. 10, 156–159.
Feng, X., Huang, L., Lin, L., Yang, M., and Ma, Y. (2017). Genetic diversity and population structure of the primary malaria vector Anopheles sinensis (Diptera: Culicidae) in China inferred by cox1 gene. Parasit Vectors 10:75. doi: 10.1186/s13071-017-2013-z
Feng, X. Y., Ma, Y. J., Xu, J. N., Liang, J. T., and Xia, A. (2015). [Genetic Polymophism and Evolution of SRPN14 Gene in Anopheles sinensis (Diptera : Culicidae)]. Chin. J. Parasitol. Parasit Dis. 33, 241–246.
Feng, X. Y., Xia, Z. G., Vong, S., Yang, W. Z., and Zhou, S. S. (2014). Chapter four–surveillance and response to drive the national malaria elimination program. Adv. Parasitol. 86, 81–108. doi: 10.1016/B978-0-12-800869-0.00004-4
Fengwen, L., Li, Y., Wang, H., Zheng, J., Tang, J., and Guo, C. (1988). Domestication of Anopheles sinensis (Guangxi strain) in laboratory. Guangxi Med. 10, 316–318.
Gao, J., Zhou, L., and He, C. (2004). Biological characteristics of Anopheles sinensis in Wujin district, Changhzou city from 1982-2002. Chin. J. Vector Biol. Control. 15, 322–323.
Garrett-Jones, C. (1964). The prognosis for interruption of malaria transmission through assessment of the mosquito’s vectorial capacity. Nature 204, 1173–1175. doi: 10.1038/2041173a0
Gou, G., Li, D., Shang, L., Guo, X., Wang, W., Sun, Q., et al. (1998). Investigation on ecology of Anopheles sinensis in 197-1996 in Luyi county. Chin. J. Vector Biol. Control 9, 133–134.
He, G., Rong, G., and Ke, X. (1962). “The study of wild habitats of Anopheles sinensis,” in Proceedings of the Conference on the Chinese Society of Insects, Beijing.
He, X., He, Z. B., Zhang, Y. J., Zhou, Y., Xian, P. J., Qiao, L., et al. (2016). Genome-wide identification and characterization of odorant-binding protein (OBP) genes in the malaria vector Anopheles sinensis (Diptera: Culicidae). Insect Sci. 23, 366–376. doi: 10.1111/1744-7917.12333
Hu, H. (1986). Malaria control in military troops in Yunnan mountainous area. Peoples Mil. Surg. 4, 11.
Hu, Y., Miao, Y., Tianbao, F., Sun, C., and Wang, H. (1988). Biological characteristics of Anopheles sinensis in rice area in Huanghuai Plain. Chin. J. Parasitol. Parasit. Dis. 1:135.
Hu, Y., Sun, Y., and Sun, C. (1986). The development peroid and survival rate of adult Anopheles sinensis at different temperatures. Chin. J. Parasitol. Parasit. Dis. 4, 53–54.
Hu, Y., Yang, B., Sun, C., Cheng, Y., Liu, G., and Miao, Y. (1981). Observation of the propagation of the plasmodium vivax in Anopheles sinensis under different temperature. Shangdong Med. 28–29.
Huang, J. X., Xia, Z. G., Zhou, S. S., Pu, X. J., Hu, M. G., Huang, D. C., et al. (2015). Spatio-temporal analysis of malaria vectors in national malaria surveillance sites in China. Parasit Vectors 8, 146. doi: 10.1186/s13071-015-0741-5
Kirby, M. J., and Lindsay, S. W. (2009). Effect of temperature and inter-specific competition on the development and survival of Anopheles gambiae sensu stricto and An. arabiensis larvae. Acta Trop. 109, 118–123. doi: 10.1016/j.actatropica.2008.09.025
Lai, Q., Zhang, S., Wei, H., and Lei, X. (1995). Developing duration at different pre-mature stages of Anopheles sinensis and Anopheles anthropophagus under laboratory condition. J. Pract. Parasit. Dis. 3, 171–173.
Lang, P., Lang, P., Xin, D., and Li, S. (2000). Vector capacity and malaria potential of Anopheles sinensis in Huangyan district, Taizhou city. Zhejiang Prev. Med. 20, 16–19.
Li, D., Xu, S., and Jin, L. (2010). Scanning electron microscopy observation on Aedes albopictus, Culex quinquefasciatus, Anopheles sinensis and Culex barraudi. J. Shantou Med. Univ. 23, 75–77.
Li, H., Yang, B., Wang, W., Hu, H., Wang, W., Wang, X., et al. (1998). Observation on the infectivity of different densities of Plasmodium vivax to Anopheles sinensis. Chin. J. Parasitol. Parasit. Dis. 16, 368–371.
Li, J., Li, F., Guo, F., and Wang, H. (1994). Observation on ecology of Anopheles sinensis in Pubei region. J. Pract. Parasit. Dis. 2, 43.
Li, M., and Tang, L. (2010). Oviposition responses of Anopheles sinensis (Diptera:Culicidae) to three bacteria. Chin. J. Vector Biol. Control 21, 102–104.
Li, S., Hong, F., and Feng, Q. (2008). Vector capacity of Anopheles sinensis in rural village of Fenghua city. Zhejiang Prev. Med. 4, 16–19.
Li, Y., Zhu, S., Sun, Q., and Zhu, H. (2010). RACE amplification of full-length cDNA of HSP40 gene from Plasmodium yoelli infected Anopheles sinensis. Int. J. Med. Parasit. Dis. 37, 132–135. doi: 10.3760/cmn.j.issn.1673-4122.2010.03.002
Li, Z., Shui-Sen, Z., Jun, F., Wen, F., and Zhi-Gui, X. (2015). Malaria situation in the People’s republic of China in 2014. Chin. J. Parasitol. Parasit. Dis. 33, 193–196.
Liu, B. Q., Qiao, L., Xu, B., Xueling, Z., and Chen, B. (2016). Identification and characterization of the CPF family of cuticular protein genes in the genome of Anopheles sinensis (Diptera: Culicidae). Acta Entomol. Sin. 59, 622–631. doi: 10.16380/j.kcxb.2016.06.005
Liu, C., Qian, H., Gu, Z., Pan, J., Zheng, X., and Peng, Z. (1986). Quantitative study on the role of Anopheles anthropophagus in malaria transmission. Chin. J. Parasitol. Parasit. Dis. 4, 161–164.
Liu, C., Qian, H., Gu, Z., Zheng, X., Wu, Z., Chen, F., et al. (1984). The study of the role of malaria transmission in Jianghui region, Anhui province. Chin. J. Parasitol. Parasit. Dis. 2, 216–219.
Liu, E., and Chen, J. (1959). Observation on hibernation of Anopheles sinensis in Jiaxing county, Zhejiang province. Acta Entomol. Sin. 2, 77–86.
Liu, H., Sun, R., Wang, F., and Shang, J. (1995). Investigation on vectorial capacity of Anopheles sinensis in Ganyu county. Chin. J. Parasit. Dis. Con. 8, 160.
Liu, K., Tsujimoto, H., Huang, Y., Rasgon, J. L., and Agre, P. (2016). Aquaglyceroporin function in the malaria mosquito Anopheles gambiae. Biol. Cell 108, 294. doi: 10.1111/boc.201600030
Liu, Q., and Liu, X. (2010). Prevention and control of Anopheles: a key approach for malaria elimination in china. Chin. J. Vector Biol. Control 21, 409–413.
Liu, Q., Liu, X., Zhang, G., Ren, D., Jiang, J., Guo, Y., et al. (2011). Primary study on the flight range of Anopheles sinensis based on the mark-release-recapture method in Yongcheng city,Henan province. Chin. J. Vector Biol. Control 22, 201–204.
Liu, W. (1962). “The habitats difference of Anopheles sinensis in different latitude and its significance in the residual spraying,” in Proceedings of the Conference on the Chinese society of Insects, Beijing, 28–29.
Liu, Y., Wu, K., Guan, D., Shi, H., Wang, J., and Sang, B. (1983). Malaria transmission dynamics research in rice areas. Parasit Parasit. Dis. 4:7.
Liu, Z., Mo, J., and Liang, M. (1989). Anopheles distribution at different landform and elevation. Youjiang Med. 2, 41–43.
Liu, Z., Wang, J., and Li, F. (1962). Mosquito investigation in Suizhong county, Liaoning province. J. Jilin. Med. Univ. 54–58.
Lounibos, L. P. (2004). “Genetic-control trials and the ecology of Aedes aegypti at the Kenya coast,” in Ecological Aspects for Application of Genetically Modified Mosquitoes, eds W. Takken and T. W. Scott (Dordrecht: Springer), 33–43
Lu, B. L., Xu, J. J., and Dong, X. S. (1997). Fauna Sinica, Insecta, Diptera: Culicidae II, Vol. 9. Beijing: Science Press.
Lu, Z. M. (1984). Gonotrophic cycle of Anopheles sinensis in the north of Yanchen, Jiangsu. Chin. J. Parasitol. Parasit. Dis. 2, 271.
Luo, D., Shi, D., Zhang, K., Li, A., and Hu, X. (1988). Observation on Anopheles sinensis oviposition in human house and cattle pen. Heinan J. Prev. Med. doi: 10.13515/j.cnki.hnjpm.1988.02.040
Luo, S., Fan, W., and Xiaojun, Y. (2006). Quantitative study on malaria infection of anopheles hurcanussinensis in Yuwu city. Dis. Surveill. 21, 232–240.
Ma, S. (1954). The hibernation of anopheles hyrcanus var.sinenis in peking-a preliminary report. Acta Entomol. Sin. 4, 293–298.
Ma, Y., and Xu, J. (2005). The Hyrcanus group of anopheles (anopheles) in China (Diptera: Culicidae): species discrimination and phylogenetic relationships inferred by ribosomal DNA internal transcribed spacer 2 sequences. J. Med. Entomol. 42, 610–619. doi: 10.1603/0022-2585(2005)042[0610:THGOAA]2.0.CO;2
Makhawi, A. M., Liu, X. B., Yang, S. R., and Liu, Q. Y. (2013). Genetic variations of ND5 gene of mtDNA in populations of Anopheles sinensis (Diptera: Culicidae) malaria vector in China. Parasit. Vectors 6, 1–11. doi: 10.1186/1756-3305-6-290
Malaria Group (1984). Investigation of malaria epidemiology in Hunan province. J. Hunan Med. Univ. 9, 141–144.
Min, G. S., Choochote, W., Jitpakdi, A., Kim, S. J., Kim, W., Jung, J., et al. (2002). Intraspecific hybridization of Anopheles sinensis (Diptera: Culicidae) strains from Thailand and Korea. Mol. Cells 14, 198–204.
Mo, K., Lin, S., Wang, J., and Wang, H. (1988). Observation of later-period management in cattle shield. Chin. J. Parasitol. Parasit. Dis. 1, 28.
Moller-Jacobs, L. L., Murdock, C. C., and Thomas, M. B. (2014). Capacity of mosquitoes to transmit malaria depends on larval environment. Parasit. Vectors 7, 593. doi: 10.1186/s13071-014-0593-4
Nie, Z., Wang, H., Nie, J., Wen, T., Wang, X., Wang, X., et al. (1996). The potential of malaria transmission of Anopheles sinensis in Longli county. Pract. Prevent. Med. 4, 85.
Ouyang, Y., Pang, L., and Li, S. (1992). Investigation on malaria transmission and ecology of Anopheles sinensis in Hunan province. Chin. J. Parasit. Dis. Con. 5, 311–312.
Pan, B., Cheng, X., Wu, J., Zhu, T., Lin, R., and Xuguang, W. (2008). Compare the capabilities of malaria transmission between Anopheles anthropophagus and Anopheles sinensis in Guangdong province. Trop. Med. 8, 1169–1171.
Pan, J., Zheng, X., Peng, Z., Deng, D., and Qian, H. (1993). The relationship between the number of ovipositions and and ovariole dilations of Anopheles sinensis and Anopheles anthropophagus. Chin. J. Parasit. Dis. Con. 6, 121–124.
Pan, J. Y., Zhou, S. S., Zheng, X., Huang, F., Wang, D. Q., Shen, Y. Z., et al. (2012). Vector capacity of Anopheles sinensis in malaria outbreak areas of central China. Parasit. Vectors 5:136. doi: 10.1186/1756-3305-5-136
Pan, S., He, G., and Li, S. (1984). The natural population dynamics of Anopheles sinensis II: the survival rate of the Anopheles sinensis larvae. Acta Acad. Med. Zhong Shan 5, 7–15. doi: 10.13471/j.cnki.j.sun.yat-sen.univ(med.sci).1984.0018
Qian, H., Deng, D., Guan, D., Jiang, B., Zhou, S., Liu, J., et al. (1984). Investigation and quantative analysis of the components of vectorial capacity of Anopheles sinensis. Chin. J. Parasitol. Parasit. Dis. 1, 3–8.
Qian, H., Tang, L., Tang, L., and Zheng, Z. (1996). Preliminary estimate on human biting rate and vector capacity of Anopheles sinensis. Pract. Prev. Med. 3, 1–2.
Qiao, L., Du, M., Liang, X., Hao, Y., He, X., Si, F., et al. (2016). Tyrosine hydroxylase is crucial for maintaining pupal tanning and immunity in Anopheles sinensis. Sci. Rep. 6, 29835. doi: 10.1038/srep29835
Qin, Z., Ran, Y., Zhi, Z., Yan, Z., Zhang, Y., Huang, T., et al. (2014). Cloning and expression analysis of odorant binding protein gene AsinOBP1 from Anopheles sinensis (Diptera: culicidae). Acta Entomol. Sin. 57, 1289–1298.
Qiu, J., and Zhao, J. (1978). Investigation on natural infection rate of Anopheles sinensis in Xuzhou, Jiangsu province. Jiangsu Med. 10, 10–12.
Qu, C., Su, S. Z., Wang, Z., Yang, R., Yang, L., and Su, T. (2000a). Life table for experimental population of Anopheles sinensis in Zhengzhou. J. Henan Med. Univ. 35, 372–375.
Qu, C., Su, S. Z., Yang, L., Yang, R., Wang, Z., Wang, M., et al. (2000b). Quantitative research of reproduction and survival of Anopheles sinensis in different areas. J. Henan Med. Univ. 5, 378–380. doi: 10.13705/j.issn.1671-6825.2000.05.004
Qu, C., Su, T., Dong, T., Shi, C., Wang, M., Wang, Z., et al. (2000c). The susceptibility and growth process of Plasmodium vivax in the body of Anopheles sinensis in Zhengzhou. J. Henan Med. Univ. 35, 383–385. doi: 10.13705/j.issn.1671-6825.2000.05.006
Qu, C., Su, T., Qi, J., Yang, L., Shi, C., Wang, J., et al. (2000d). The pattern of quantitative alternation of natural Anopheles sinensis in Zhengzhou. J. Henan Med. Univ. 35, 388–390. doi: 10.13705/j.issn.1671-6825.2000.05.008
Qu, C., Wang, J., Qi, J., Dong, T., Yang, R., Su, T., et al. (2000e). Quantitative research on natural population of Anopheles sinensis and innovation in researching tool. J. Henan Med. Univ. 35, 412–416. doi: 10.13705/j.issn.1671-6825.2000.05.014
Qu, C., Wang, J., Yang, R., Su, T., Zhang, R., Su, T., et al. (2000f). Comparisions on life table of Anopheles sinensis population in different areas. J. Henan Med. Univ. 35, 397–402. doi: 10.13705/j.issn.1671-6825.2000.05.011
Qu, C., Wang, J., Zhang, R., Shi, C., Su, T., Su, T., et al. (2000g). Establishment of ecological life tables of Anopheles sinensis in nature. J. Henan Med. Univ. 35, 416–421. doi: 10.13705/j.issn.1671-6825.2000.05.015
Qu, C., Zhang, R., Yang, L., Su, T., Wang, M., Su, T., et al. (2000h). Net reproductive rate and other population parameters of natural population of Anopheles sinensis in Zhengzhou. J. Henan Med. Univ. 35, 407–411. doi: 10.13705/j.issn.1671-6825.2000.05.013
Qu, C., Zhang, R., Yang, L., Su, T., Wang, M., Su, T., et al. (2000i). Reproduction, survival and population fluctuation pattern of natural population of Anopheles sinensis in Zhengzhou. J. Henan Med. Univ. 35, 402–406.
Ree, H. I. (2005). Studies on Anopheles sinensis, the vector species of vivax malaria in Korea. Korean J. Parasitol. 43, 75–92. doi: 10.3347/kjp.2005.43.3.75
Reid, J. A. (1953). The Anopheles hyrcanus group in South-East Asia (Diptera: Culicidae). Bull. Entomol. Res. 44, 5–76. doi: 10.1017/S0007485300022938
Reidenbach, K. R., Cook, S., Bertone, M. A., Harbach, R. E., Wiegmann, B. M., and Besansky, N. J. (2009). Phylogenetic analysis and temporal diversification of mosquitoes (Diptera: Culicidae) based on nuclear genes and morphology. BMC Evol. Biol. 9, 1–14. doi: 10.1186/1471-2148-9-298
Rodgers, F. H., Gendrin, M., Cas, W., and Christophides, G. K. (2017). Microbiota-induced peritrophic matrix regulates midgut homeostasis and prevents systemic infection of malaria vector mosquitoes. PLoS Pathog. 13:e1006391. doi: 10.1371/journal.ppat.1006391
Rongsriyam, Y., Jitpakdi, A., Choochote, W., Somboon, P., Tookyang, B., and Suwonkerd, W. (1998). Comparative susceptibility of two forms of Anopheles sinensis Wiedemann 1828 (Diptera : Culicidae) to infection with Plasmodium falciparum, P. vivax, P. yoelii and the determination of misleading factor for sporozoite identification. Southeast Asian J. Trop. Med. Public Health 29, 159–167.
Rueda, L. M., Brown, T. L., Kim, H. C., Chong, S. T., Klein, T. A., Foley, D. H., et al. (2010). Species composition, larval habitats, seasonal occurrence and distribution of potential malaria vectors and associated species of Anopheles (Diptera: Culicidae) from the Republic of Korea. Malaria J. 9, 164–175. doi: 10.1186/1475-2875-9-55
Scherer, W. F., Buescher, E. L., Flemings, M. B., Noguchi, A., and Scanlon, J. (1959). Virus in Japan. Mosquito factors. Zoatropism and vertical flight of C. tritaeniorhynchus with observations on variations in collections from animal-baited traps in different habitats. Am. J. Trop. Med. Hyg. 8, 665–677. doi: 10.4269/ajtmh.1959.8.665
Shen, B., Fang, H., and Zhou, H. (1995). Comparative observation on development of Anopheles sinensis and Anopheles anthropophagus. Chin. J. Schistosomiasis Control 7, 318.
Shi, D., Shang, L., Li, A., Gou, G., Huang, Z., and Fu, Z. (1983a). The susceptibility of Anopheles sinensis to plasmodium falciparum. Heinan J. Prev. Med. 49–50.
Shi, D., You, X., Chen, J., Li, A., Gou, G., Shang, L., et al. (1983b). Study of Anopheles sinensis artificial infection with Plasmodium vivax in Henan province. Heinan J. Prev. Med. 45–48.
Shi, H., Jiang, M., Wu, K., Liu, Y., Guan, D., Sang, B., et al. (1996). Malaria transmission dynamics in Deqing county, Zhejiang province. Chin. J. Parasitol. Parasit. Dis. 14, 322–323.
Song, S. (2015). Malaria transmission in Xiangshan peninsula. Chin. Rural Health Serv. Admin 35, 67–68.
Sreenivasamurthy, S. K., Dey, G., Ramu, M., Kumar, M., Gupta, M. K., Mohanty, A. K., et al. (2013). A compendium of molecules involved in vector-pathogen interactions pertaining to malaria. Malaria J. 12:216. doi: 10.1186/1475-2875-12-216
Station, A.-E. (1985). Seven year’s malaria surveillance in Guidong county, Hunan province. Chin. J. Parasitol. Parasit. Dis. 3, 170–173.
Strode, C., Steen, K., Ortelli, F., and Ranson, H. (2006). Differential expression of the detoxification genes in the different life stages of the malaria vector Anopheles gambiae. Insect Mol. Biol. 15, 523–530. doi: 10.1111/j.1365-2583.2006.00667.x
Su, T., and Su, S. Z. (1989). Effect of different blood sources on reproductive capacity, gonotrophic cycle, longevity and biochemical aspects of Anopheles sinensis. Chin. J. Parasit. Dis. Con. 2, 15–18.
Sun, Y., Deng, S., and Liu, L. (1994). The developmental temperature and effective accumulated temperature of Anopheles sinensis. J. Pract. Parasit. Dis. 2, 41–42.
Sun, Y., Hu, Y., and Sun, C. (1987). Investigation on the role of light cycle in Anopheles sinensis diapause. Chin. J. Parasitol. Parasit. Dis. 5, 158.
Tang, J. X., Zhang, C., Bai, L., Li, J. L., Liu, K., Zhou, H. Y., et al. (2012). Cloning and sequence analysis of cDNA encoding aquaporin (AQP) gene from Anopheles sinensis. Chin. J. Schistosomiasis Control 24, 663–667.
Tang, L. (2010). Diagnose, Treatment and Management of Imported Malaria Cases. Shanghai: Shanghai Science and Technology Press.
Wang, H., Guo, C., Huang, H., Liu, C., Qian, H., Liu, Y., et al. (1987). Geographical distribution of Anopheles anthropophagus and its role in malaria transmission in Guangxi. Chin. J. Parasitol. Parasit. Dis. 5, 104–106.
Wang, H., Jiang, Q., Zhang, S., Jiang, J., and Huang, J. (1982). Observation of the development and incubation period of the Plasmodium vivax in Anopheles sinensis in Kaifeng, Henan province. Henan Med. 2, 58.
Wang, J., Wang, Y., and Yao, B. (1985). Studies on the population dynamics of Anopheles sinensis in Guizhou province, developmental characeristics of the immature stages. J. Guiyang Med. Univ. 2, 86–91.
Wang, J., Yiliang, Y., Hong, G., Zhang, Y., Chen, Y., and Ji, S. (1990). Investigation on malaria vector in Shanghai. Chin. J. Parasitol. Parasit. Dis. 8, 216.
Wang, M., Tang, L., Zhengcheng, G., Jiang, W., Zhu, J., Zhao, Z., et al. (2007). Study on threshold density of Anopheles sinensis for transmission of malar ia in the Northern Anhui Province. J. Trop. Med. 7, 597–599.
Wang, R. B., Zhang, Q. F., Zheng, B., Xia, Z. G., Zhou, S. S., Tang, L. H., et al. (2014). Transition from control to elimination: impact of the 10-year global fund project on malaria control and elimination in China. Adv. Parasitol. 86, 289–318. doi: 10.1016/B978-0-12-800869-0.00011-1
Wang, W., Li, J., Zhou, H., Cao, J., Yang, K., Gu, Y., et al. (2010). Impact of different temperature on development of Anopheles sinensis. Chin. J. Schistosomiasis Control 22, 260–263.
Wang, X. (2013). Observation on the parous rate of Anopheles sinensis in different time period at night in Zhang jia gang city. Chin. Trop. Med. 13, 367–368. doi: 10.13604/j.cnki.46-1064/r.2013.03.002
Wang, X., Yan, D. U., Yang, L., Cheng, P., Liu, L., Gong, M., et al. (2015). Study on the relationship between IRE_BP1 gene expression and cypermethrin resistance of Anopheles sinensis. Chin. Trop. Med. 15, 1037–1039.
Wu, X., Wu, J., Pan, B., and Ruan, F. (2005). The effect of low temperature in winter on the development of Anopheles sinensis in the littoral zone of Guangdong. J. Trop. Med. 5, 77–779.
Xiangkun, L., Zheng, X., Xie, J., Chen, Z., Chen, Y., Zhou, Y., et al. (1991). Experimental study on the susceptibility of Anopheles sinensis to Plasmodium vivax in Guizhou. Chin. J. Parasit. Dis. Con. 4, 176–178.
Xu, D., and Zhang, T. (1988). Effects of various illuminations on the reproduction capacity of Anopheles sinensis. J. Zunyi Med. Univ. 11, 36–38.
Xu, D., Zhang, T., and Zhang, Q. (1988). The influence of Anopheles sinensis larvae density on the growth. J. Zunyi Med. Univ. 11, 62–63.
Xu, J. J., and Feng, L. C. (1975). Studies on the Anopheles hyrcanus group of mosquitoes in China. Acta Entomol. Sin. 18, 77–98.
Xu, L., He, X., and Yu, J. (1988). The experimental observation on the development and reproduetion of Anopheles. Chin. J. Parasitol. Parasit. Dis. 1:136.
Xu, L., He, X., and Yu, J. (1991). Life table of Anopheles sinensis Fuzhou Strain. Chin. J. Pest Control 7, 48–49.
Xu, L., Wu, J., Huang, B., He, X., and Xu, B. (1990). Comparative analysis of malaria transmission of Anopheles sinensis and Anopheles anthropophagus. Chin. J. Parasit. Dis. Con. 3, 69–70.
Xu, L., Wu, J., Xu, B., Zheng, C., and Wang, H. (1987). Quantitative analysis of malaria transmission of Anopheles sinensis and Anopheles anthropophagus. J. Fujian Med. Univ. 21, 281.
Xu, R., Zhang, J., Hu, Y., Du, X., and Wang, X. (1991). Imamature development and survivorship of ricefield-breeding mosquito:Anopheles sinensis and Culex tritaeniorhynchus. Chin. J. Pest. Control 7, 181–183.
Xu, Z., and Ye, Y. (1987). The susceptibility of Anopheles sinensis to different Plasmodium vivax geographic strains. Chin. J. Parasitol. Parasit. Dis. 5, 161. doi: 10.16190/j.cnki.45-1211/r.1987.04.013
Xu, Z., Ye, Y., He, D., and Long, Z. (1983). The susceptibility of Anopheles sinensis to Plasmodium falciparum and Plasmodium vivax. Chin. J. Parasitol. Parasit. Dis. 2, 9–16. doi: 10.16190/j.cnki.45-1211/r.1985.02.003
Xue, R., Lu, B., and Jing, M. (1990). Investigation on hibernation of Anopheles sinensis. Acta Entomol. Sin. 3, 444–449.
Yan, X., Chen, X., Peng, H., and Zheng, X. (2005). Cloning and prokaryotic expression of Anopheles sinensis defensin gene and preliminary bioactivity evaluation of the recombinant product. J. First Mil Meal Univ. 25, 371–376.
Yan, Z., Zhang, Y., Zhou, Y., and Chen, B. (2015). Identification and bioinformatics analysis of CYP4G17 gene of in Anopheles sinensis. J. Chongqing Nor. Univ. 3, 35–41.
Yang, F., and Tian, Z. (1994). Investigation on vectorial capacity of Anopheles sinensis in Yanhe county, Guizhou province. Endem. Dis. Bull. 9, 58–59. doi: 10.13215/j.cnki.jbyfkztb.1994.02.027
Yang, G., Shao, M., Li, D., and Yu, H. (2013). Malaria transmission threshold value of Anopheles sinensis in Shuangliu county. J. Parasit. Infect. Dis. 11, 26–28.
Yang, Q., Wang, Y., and Shi, J. (1994). Morphological characteristics of Anopheles sinensis and Anopheles anthropophagus. Hubei J. Prev. Med. 5, 19–21.
Yao, L., Pan, P., Ji, X., and Yang, W. (2002). Investigation on vectorial capacity of Anopheles sinensis in Haining city, Zhejiang province. J. Pract. Parasit. Dis. 10, 67.
Yi, Z., Song, S., and Pan, H. (1998). Malaria epidemiology investigation in the three gorges reservoir area in hubei province. Hubei J. Prev. Med. 9, 1–3.
Yin, J. H., Zhou, S. S., Xia, Z. G., Wang, R. B., Qian, Y. J., Yang, W. Z., et al. (2014). Historical patterns of malaria transmission in China. Adv. Parasitol. 86, 1–19. doi: 10.1016/B978-0-12-800869-0.00001-9
You, X., Shi, D., Ge, F., Qu, Q., and Su, S. (1964). Investigation on hibernation of Anopheles sinensis in different regions in Henan province. J. Zhengzhou Univ. 4, 17.
You, X., and Xu, Y. (1979). Investigation on ecological habit of Anopheles sinensis in irrigation well in Yuanyou and Yuanzhai village in Kaifeng county. Heinan J. Prev. Med. 40–41.
Yu, H., Tan, K., Yang, G., Tao Wang, Song, Z., Chen, G., et al. (2014). Threshold density of Anopheles sinensis for transmission of malaria in Chengdu city. J. Prev. Med. Inf. 30, 817–820.
Yuan, F., Xu, B., Yu, P., and Ming, G. (1988). Larva feeding habit and poison effect of Bacillus thuringiensis on Anopheles sinensis and Aedes albopictus. Chin. J. Parasitol. Parasit. Dis. 1, 142.
Yusheng, T., and Xingbang, C. (1958). Preliminary observations on the effect of atmospheric temperature on the oviposition metamorphosis of Anopheles hyrcanus sinensis in West Hunan. Acta Entomol. Sin. 8, 371–372.
Zhang, G., Dong, X., Wang, X., and Lu, Y. (1989). Quantitative study on transmission of malaria by Anopheles kunmingensis. Chin. J. Parasitol. Parasit. Dis. 7, 100–102.
Zhang, G., and Zhang, Z. (1997). Comparative analysis of role of malaria transmission by Anopheles sinensis and Anopheles kunmingensis. Chin. J. Pest Control 13, 149–151.
Zhang, J., Jiang, J., Ceng, X., Zheng, Y., and Zhou, H. (2015). Study of ecological habits of important malaria vectors in epidemicprone regions of malaria in Yunnan province, China. Chin. J. Vector Biol. Control 26, 47–54. doi: 10.11853/j.issn.1003.4692.2015.01.012
Zhang, J., Jianyue, W., Han, S., and Wang, H. (1990). Observation on ecology of Anopheles sinensis in Zhoushan island. Chin. J. Pub. Heal 9, 270.
Zhang, J., Jianyue, W., Le, Z., and Jiang, Y. (2001). Studies on surveillance of vector of malaria in the controlled areas in Zhoushan island. Chin. J. Vector Biol. Control 12, 127–129.
Zhang, J., Lu, G., Kelly, P., Zhang, Z., Wei, L., Yu, D., et al. (2014). First report of Rickettsia felis in China. BMC Infect. Dis. 14:682. doi: 10.1186/s12879-014-0682-1
Zhang, X., Xie, H., Zhao, D., and Wen, M. (2006). Investigation on vectorial capacity of Anopheles sinensis in Wenzhou city. Zhejiang J. Prev. Med. 18, 29–30.
Zhang, Y., Xu, X., Wang, Z., and Su, S. (1982). Observation of eggs of Anopheles sinensis by electron microscope scanning. J. Henan Med. Univ. 1, 4.
Zhang, Y., Xu, X., Wang, Z., and Su, S. (1984). The study of eggs of different Anopheles sinensis strains by scanning electron microscope. Chin. J. Parasitol. Parasit. Dis. 48–49.
Zhang, Y. J., Chen, X. G., Zheng, X. L., and Wang, C. M. (2006). Cloning and identification of the cDNA and genomic DNA sequences of the defensin gene of Anopheles sinensis. Chin. J. Parasitol. Parasit. Dis. 24, 35–40.
Zhao, C., Yang, B., Du, X., Li, J., Fu, Z., Han, G., et al. (1996). Investigation on vectorial capacity of Anopheles sinensis in urban region of Jining city. Chin. J. Parasit. Dis. Con. 9, 18.
Zhao, T., Xue, R., and Lu, B. (1991). Experimental study on effect of photoperiod on blood-feeding and ovary development of Anopheles sinensis. Chin. J. Pub. Health 10, 93–95.
Zhao, Y., and Zhen, T. (1997). Egg hatching rate of Anopheles sinensis at different temperatures. Chin. J. Parasit. Dis. Con. 10, 319–320.
Zheng, X., Chen, X., and Wang, C. (2005). Cloning and sequence analysis of cDNA encoding the antibacterial peptide, defensin from the mosquito, Anopheles sinensis. Chin. J. Parasit. Dis. Con. 18, 404–406.
Zheng, Y., Liu, D., Xie, C., and Nie, C. (1986). Comparative study on susceptibility of Anopheles sinensis to two Plasmodium vivax strains. J. Hunan Med. Univ. 11, 337–340.
Zhi, G. Z., Chen, X. G., Zhou, X. H., Peng, H. J., Lin, L. F., Yi, J. R., et al. (2002). Cloning and sequence analysis of mosquito defensin genes. J. Dali Meal Univ. 22, 499–502.
Zhi, Z. J., Qin, Z., Huang, T., Ran, Y. H., Yan, Z. T., Si, F., et al. (2015). Molecular characterization and expression profiles post blood feeding of heme oxygenase gene asho-1 in Anopheles sinensis (diptera: culicidae). Acta Entomol. Sin. 58, 950–958.
Zhong, G. (2000). Experimental study on the biological traits of Anopheles sinensis and Anopheles anthropophagus. Chin. J. Pest Control 16, 4–7.
Zhong, G., and Tan, Y. (2000). Experimental observation on the fecundity and longevity of Anopheles sinensis. Chin. J. Vector Biol. Control 11, 265–267.
Zhou, D., Liu, X., Sun, Y., Ma, L., Shen, B., and Zhu, C. (2015). Genomic analysis of detoxification supergene families in the mosquito Anopheles sinensis. PLoS ONE 10:e0143387. doi: 10.1371/journal.pone.0143387
Zhou, D., Zhang, D., Ding, G., Shi, L., Hou, Q., Ye, Y., et al. (2014). Genome sequence of Anopheles sinensis provides insight into genetics basis of mosquito competence for malaria parasites. BMC Genomics 15:42. doi: 10.1186/1471-2164-15-42
Zhou, H., Fang, H., and Shen, B. (1995). Observation of the life expectancy of Anopheles and Anopheles anthropophagus. Chin. J. Schistosomiasis Control 7, 349–351.
Zhou, H., Zhang, Z., Li, C., Wu, C., Wang, P., Curtis, C., et al. (2005). The role of the malaria vectors in the upper valley of Mekong river in Yunnan. J. Pathog. Biol. 18, 407–411.
Zhou, S. S., Wang, Y., and Tang, L. H. (2007). Malaria situation in the People’s Republic of China in 2006. Chin. J. Parasitol. Parasit. Dis. 25, 439–441.
Zhou, W., Peng, J., and Shen, Z. (1988). The relationship of the color of the Anopheles sinensis larve, enviroment and adult sex. Chin. J. Zoonoses 4, 24–26.
Zhu, G., Gao, Q., Zhou, H., Li, J., Jin, X., Lu, F., et al. (2007). Growth difference of Plasmodium vivax in Anopheles sinensis and an Anopheles anthropophagus during the periods of clinic attack and diapause of vivax malaria patients. Chin. J. Schistosomiasis Control 19, 274–277.
Zhu, G., Xia, H., Zhou, H., Li, J., Lu, F., Liu, Y., et al. (2013). Susceptibility of Anopheles sinensis to Plasmodium vivax in malarial outbreak areas of central China. Parasit Vectors 6, 176. doi: 10.1186/1756-3305-6-176
Zhu, G., Zhong, D., Cao, J., Zhou, H., Li, J., Liu, Y., et al. (2014). Transcriptome profiling of pyrethroid resistant and susceptible mosquitoes in the malaria vector, Anopheles sinensis. BMC Genomics 15:448. doi: 10.1186/1471-2164-15-448
Zhu, H., Li, C., Duan, L., Liu, Y., Wang, G., Fan, K., et al. (2015). Investigation on species and density of malaria vector anopheles in Chenzhou from 2013 to 2014. J. Commun. Med. 13, 25–27.
Keywords: Anopheles sinensis, biology, bionomics, gene, protein, molecule, China, vector
Citation: Feng X, Zhang S, Huang F, Zhang L, Feng J, Xia Z, Zhou H, Hu W and Zhou S (2017) Biology, Bionomics and Molecular Biology of Anopheles sinensis Wiedemann 1828 (Diptera: Culicidae), Main Malaria Vector in China. Front. Microbiol. 8:1473. doi: 10.3389/fmicb.2017.01473
Received: 11 May 2017; Accepted: 20 July 2017;
Published: 09 August 2017.
Edited by:
Guan Zhu, Texas A&M University, United StatesReviewed by:
Berlin L. Londono-Renteria, Kansas State University, United StatesCopyright © 2017 Feng, Zhang, Huang, Zhang, Feng, Xia, Zhou, Hu and Zhou. This is an open-access article distributed under the terms of the Creative Commons Attribution License (CC BY). The use, distribution or reproduction in other forums is permitted, provided the original author(s) or licensor are credited and that the original publication in this journal is cited, in accordance with accepted academic practice. No use, distribution or reproduction is permitted which does not comply with these terms.
*Correspondence: Wei Hu, aHV3d3l6QDE2My5jb20= Shuisen Zhou, enNzMTYzQGhvdG1haWwuY29t
Disclaimer: All claims expressed in this article are solely those of the authors and do not necessarily represent those of their affiliated organizations, or those of the publisher, the editors and the reviewers. Any product that may be evaluated in this article or claim that may be made by its manufacturer is not guaranteed or endorsed by the publisher.
Research integrity at Frontiers
Learn more about the work of our research integrity team to safeguard the quality of each article we publish.