- 1Laboratory for Process Microbial Ecology and Bioinspirational Management, Department of Microbial and Molecular Systems, KU Leuven, Sint-Katelijne-Waver, Belgium
- 2Department of Microbial Ecology, Netherlands Institute of Ecology (KNAW), Wageningen, Netherlands
- 3Research Centre Hoogstraten vzw Meerle, Belgium
- 4Research Station for Vegetable Production vzw, Sint-Katelijne-Waver, Belgium
- 5Scientia Terrae vzw, Sint-Katelijne-Waver, Belgium
- 6Department of Microbial and Molecular Systems, Centre of Microbial and Plant Genetics, KU Leuven, Leuven, Belgium
- 7Rega Institute, KU Leuven, Leuven, Belgium
Rhizogenic Agrobacterium biovar 1 is the causative agent of hairy root disease (HRD) in the hydroponic cultivation of tomato and cucumber causing significant losses in marketable yield. In order to prevent and control the disease chemical disinfectants such as hydrogen peroxide or hypochlorite are generally applied to sanitize the hydroponic system and/or hydroponic solution. However, effective control of HRD sometimes requires high disinfectant doses that may have phytotoxic effects. Moreover, several of these chemicals may be converted to unwanted by-products with human health hazards. Here we explored the potential of beneficial bacteria as a sustainable means to control HRD. A large collection of diverse bacterial genera was screened for antagonistic activity against rhizogenic Agrobacterium biovar 1 using the agar overlay assay. Out of more than 150 strains tested, only closely related Paenibacillus strains belonging to a particular clade showed antagonistic activity, representing the species P. illinoisensis, P. pabuli, P. taichungensis, P. tundrae, P. tylopili, P. xylanexedens, and P. xylanilyticus. Assessment of the spectrum of activity revealed that some strains were able to inhibit the growth of all 35 rhizogenic agrobacteria strains tested, while others were only active against part of the collection, suggesting a different mode of action. Preliminary characterization of the compounds involved in the antagonistic activity of two closely related Paenibacillus strains, tentatively identified as P. xylanexedens, revealed that they are water-soluble and have low molecular weight. Application of a combination of these strains in greenhouse conditions resulted in a significant reduction of HRD, indicating the great potential of these strains to control HRD.
Introduction
Since the early 1990s, in several European countries hydroponically grown cucumber plants and tomato crops have been affected by a disorder called “hairy root disease” (HRD). The disease is characterized by extensive root proliferation leading to strong vegetative growth and, in severe cases, substantial losses in marketable yield (Weller et al., 2006; Ludeking et al., 2013). In hydroponic crops HRD is generally associated with rhizogenic Agrobacterium biovar 1 strains (further referred to as “rhizogenic agrobacteria”), harboring a Ri–plasmid (root-inducing plasmid; Gelvin, 2003). Symptoms arise following transfer of a portion of the Ri-plasmid (T-DNA; transferred DNA) from the bacterium to plant cells, where it is integrated in the chromosomal DNA and subsequently expressed (Hooykaas and Beijersbergen, 1994), leading to excessive root development. Once plants are infected, HRD cannot be controlled by curative means. Instead, preventative actions should be taken, such as preventing, and/or removing Agrobacterium containing biofilms that are often associated with the disease in the greenhouse irrigation system (Danhorn and Fuqua, 2007; Bosmans et al., 2015). However, to effectively prevent the disease generally high concentrations of chemical disinfectants are required, including levels that may be phytotoxic (Bosmans et al., 2016c). Moreover, several of these chemicals may be converted to unwanted by-products with human health hazards (Damstra, 2002). Therefore, there is currently a strong interest in alternative means to prevent and control HRD such as the use of biocontrol organisms (BCO).
The use of BCO has received great attention the last few decades because of the ability of such antagonistic strains to suppress plant diseases with less environmental impact than chemical pesticides, reduced off-target effects in microbiota linked to a narrow activity spectrum, and the possibility to be integrated with other control methods (Raaijmakers et al., 2002; Rubino et al., 2013). Especially rhizosphere bacteria are generally considered ideal BCO of soilborne plant pathogens because of their effective colonization of the rhizosphere, which provides a front-line defense against pathogen attack; their versatility to protect plants under different conditions; and their production of antimicrobial compounds (Sharma et al., 2009). However, so far, no bacterial antagonists have been identified to control rhizogenic Agrobacterium biovar 1.
The objectives of this study were (i) to identify potential bacterial BCO of rhizogenic agrobacteria using both laboratory and greenhouse experiments and (ii) to perform a preliminary characterization of the compounds involved in the antagonistic activity. To this end, a large collection of diverse bacterial isolates from rhizosphere soil was screened for antagonistic activity using the agar overlay assay. Antimicrobial compounds were determined using RP-HPLC and a quadrupole orthogonal acceleration time-of-flight mass spectrometer. Further, biocontrol activity of a mixture of the most promising strains was assessed under greenhouse conditions.
Materials and Methods
Culture Collection and Screening for Antagonists of Rhizogenic Agrobacteria
In a first screening, a collection of 130 phylogenetically different bacterial strains isolated from soil habitats (De Ridder-Duine et al., 2005) was used in this study and subjected to high-throughput screening for antagonists of rhizogenic agrobacteria as described previously (Tyc et al., 2014; Table S1, Supporting Information). The collection consisted of strains from different phyla and different classes (Table 1), and has previously been evaluated for antagonistic activity against two human pathogenic model organisms, including Escherichia coli and Staphylococcus aureus (Tyc et al., 2014). Additionally, Streptomyces rimosus DSM40260, a producer of oxytetracycline, was included in the study as a reference strain. Strains were stored in glycerol at −80°C in two 96-well plates until further use. To this end, first wells of the 96-well plates were filled with 150 μl lysogeny broth (LB) (10 g/L NaCl, 10 g/L Bacto™ Tryptone, 5 g/L Bacto™ Yeast extract) and inoculated with the strains. Plates were then incubated for 2 days at 25°C with gentle agitation, after which 50 μl of 50% (v/v) glycerol was added to achieve a final glycerol concentration of 12.5% (v/v).
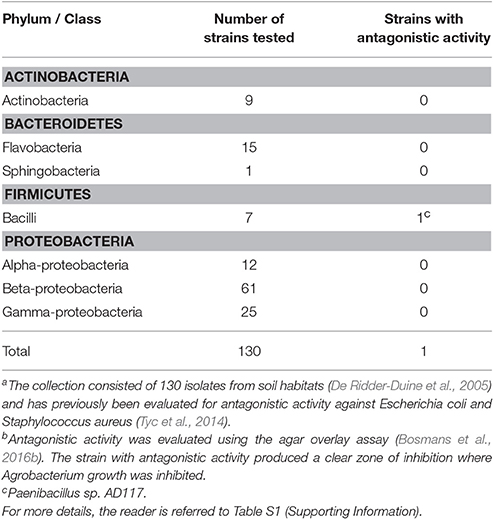
Table 1. Overview of antagonistic activity screening of 130 bacterial soil isolatesa against rhizogenic Agrobacterium biovar 1 (strain ST15.13/097)b.
For assessing the antagonistic properties of the collection, the 96-well plates were thawed and isolates were spotted using the Genetix QPix 2 colony picking robot (Molecular Devices, UK Limited, Wokingham, United Kingdom) on OmniTray-plates (size 128 × 86 mm; capacity 90 mL; Greiner Bio-One B.V., Alphen a/d Rijn, The Netherlands) with 15 mL solid bacterial growth medium [5 g/L NaCl, 1 g/L KH2PO4; 3 g/L Oxoid Tryptic Soy Broth (TSB); 20 g/L Merck Agar-agar; further referred to as “AAA” medium (Agar medium to screen for Antagonistic Activity)]. The plates were incubated for 5 days at 20°C and were used as source plates for spotting test plates containing the same medium mentioned above. Importantly, Merck agar-agar was used in our screening as this agar was shown to support bacterial antagonistic activity against rhizogenic agrobacteria, while several other agars were not (Bosmans et al., 2016b). Spot-inoculated OmniTray plates were then incubated for 1 day at 25°C. Subsequently, 15 mL melted LB agar containing Agrobacterium (about 6 × 105 cells per mL) was poured over the surface of the plate and incubated again at 25°C. After 24 h of incubation, the diameter of the inhibition visible zones surrounding spotted colonies was recorded using a digital caliper (Tyc et al., 2014; Bosmans et al., 2016b). Experiments were performed for one rhizogenic Agrobacterium biovar 1 strain (ST15.13/097, isolated from tomato; Bosmans et al., 2015), and were independently repeated twice (no replicates within the same experiment).
In a second screening, several strains from the same genus as the only strain showing antagonistic activity in the initial high-throughput screening mentioned above (i.e., Paenibacillus; Table 2) were evaluated for antagonistic activity against Agrobacterium biovar 1 strain ST15.13/097 in an agar overlay assay using 9 cm-diameter petri dishes as described by Bosmans et al. (2016b). For all strains showing antagonistic activity the spectrum of activity was evaluated using 35 rhizogenic Agrobacterium biovar 1 strains and 37 other strains from diverse phyla including Actinobacteria, Firmicutes, and Proteobacteria, among which several strains are known as plant pathogens. Besides a number of agrobacteria, test isolates also included other, non-agrobacterial strains (Table 3). Experiments were again independently repeated twice (no replicates within the same experiment).
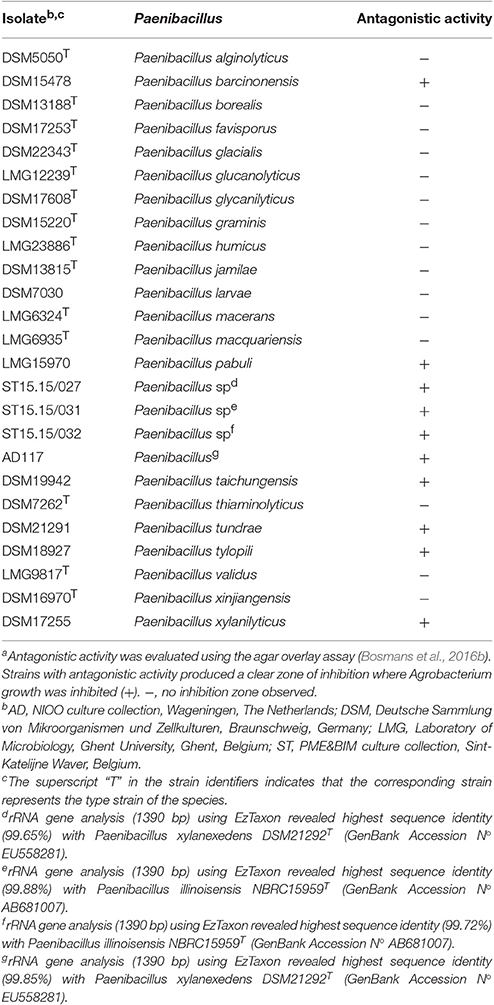
Table 2. Antagonistic activitya of diverse Paenibacillus strains against rhizogenic Agrobacterium biovar 1 (strain ST15.13/097).
Characterization of Antagonistic Strains
For all strains with antagonistic activity the 16S ribosomal RNA (rRNA) genes were partially amplified and sequenced as described by Bosmans et al. (2015). Obtained sequences were individually trimmed for quality, using a minimum Phred score of 20, and, in cases of ambiguous base calls, manually edited based on the obtained electropherograms. A maximum likelihood tree was constructed using MEGA v5.2 (Tamura et al., 2011) to assess the phylogenetic relatedness between the antagonistic strains as well as their phylogenetic relationships with previously characterized reference (type) strains for which the sequences were retrieved from EzTaxon (www.ezbiocloud.net/eztaxon).
Antagonistic strains were subjected to a Bioscreen C analysis (Oy Growth Curves Ab Ltd, Helsinki, Finland) to assess growth characteristics in different media. The working volume in the wells of the Bioscreen plate was 200 μL, comprised of 5 μL bacterial suspension (about 105 cells per mL LB medium) and 195 μL of one of the following three media: TSB (Oxoid, Basingstoke, UK), LB and a minimal broth medium (M70) containing 2 g/L Bacto™ Yeast extract and 10 g/L Mannitol (Sigma, Missouri, US). The temperature was controlled at 25°C, and the optical density of the cell suspensions was measured automatically at 600 nm in regular intervals of 15 min, for 3 days. Before each measurement, the Bioscreen plate was automatically shaken for 60 s. The experiments were performed two times independently, each with three replicates. Tested culture medium without inoculum was used as a reference. Growth curves were generated by monitoring the averaged optical density (OD600) as a function of incubation time.
Preliminary Characterization of the Antagonistic Compound(s)
The two best performing strains (based on the size of the zone of inhibition, specificity and growth in the previous assays), including AD117 (the same as ST15.13/036, Bosmans et al., 2016b) and ST15.15/027, were selected for preliminary characterization of the antagonistic compounds. First, isolates were investigated for production of volatile organic compounds (VOCs) having antagonistic activity against Agrobacterium. To this end, two bottoms of a 9 cm-diameter petri dish, one containing a freshly spot-inoculated (15 μL per spot; about 105 cells per mL in TSB) antagonistic bacterium (on AAA, see above) and the other a rhizogenic Agrobacterium biovar 1 isolate (ST15.13/097; on TSA, Oxoid, Basingstoke, UK), were sealed facing each other and incubated at 25°C with the petri-dish containing the antagonistic bacterium at the bottom. The experiments were carried out using two independent repeats, each with three replicates. After 1, 2, and 3 days of incubation, plates were checked for agrobacterial growth inhibition (formation of inhibition zones).
Secondly, to assess whether the antagonistic compounds are secreted to the extracellular space, cell-free culture filtrates were prepared and tested for antibacterial activity in a microtitre plate (Thermo Scientific™ Nunc™ MicroWell™ 96-Well Microplates). To this end, antagonistic bacteria were cultured in liquid medium (100 mL) consisting of 3 g/L tryptic soy broth (TSB; Oxoid, Basingstoke, UK), 5 g/L NaCl, and 1 g/L KH2PO4, and incubated at 25°C for 2 days. Cultures of about 104 cells per mL were then filter-sterilized (0.2-μm filter, sterile mixed cellulose ester membrane, Whatman, GE Healthcare Life Sciences, UK), and a portion of the filtrate was added to the wells of the microtiter plate. More specifically, 100, 150, and 190 μL of the cell-free filtrates were added to 100, 50, and 10 μL LB containing Agrobacterium biovar 1 isolate ST15.13/097, respectively. For each test medium, a negative control was included in which the volume of the culture filtrate was replaced by fresh TSB medium (3 g/L TSB, 5 g/L NaCl, and 1 g/L KH2PO4). Additionally, culture filtrate from a Paenibacillus strain without antagonistic activity (LMG6324) was included as another negative control (prepared as described above). Each well (200 μL test volume) contained 5 × 102 agrobacterial cells per mL. Plates were incubated with gentle agitation and growth was photospectrometrically (OD600) quantified after 24 h of incubation at 25°C. Experiments were independently repeated twice (with two replicates per experiment).
Extraction and Purification of the Antagonistic Compound(s)
For the extraction and identification of the compounds responsible for the antagonistic activity, the two best performing strains, AD117 and ST15.15/027, were selected and spot-inoculated (15 μL per spot) on AAA medium (see above) in 9 cm-diameter petri dishes (60 plates per strain). Following inoculation with Agrobacterium (isolate ST15.13/097; see above) and subsequent incubation for 1 day at 25°C, 60 agar pieces of approximately 1 cm2 were excised from the zone of inhibition, suspended in 65% methanol (65% methanol, 34.9% milliQ water and 0.1% formic acid) and shaken for 3 h at room temperature. After centrifugation at 5,000 g for 15 min, the liquid phase was transferred and the methanol was evaporated by air drying. Subsequently, the aqueous phase was frozen and freeze-dried, and the dried extract was dissolved again in 65% methanol prior to further analysis. Obtained extracts were analyzed by reversed-phase high-performance liquid chromatography (RP-HPLC; Waters Chromatography B. V., Etten-Leur, the Netherlands) equipped with a Waters 996 photodiode array detector. The separations were performed on a Waters Symmetry C18RP column (5 μm, 3.9 × 150 mm) with a mobile phase of 70% methanol and 0.1% formic acid, and operated at a flow of 0.2 mL/min for 10 min (or 60 min for improved resolution of peaks) with UV detection at 240 nm. Fractions were collected each 5 min or by collecting particular peaks. For each collected fraction, methanol was evaporated and the remaining (aqueous) phase was freeze-dried, dissolved again in 65% methanol, and 20 μL was spotted on a sterile filter paper and covered by an Agrobacterium overlay. Twenty liters methanol, spotted on filter paper was used as a control.
For those HPLC fractions that had activity against Agrobacterium, mass spectra were acquired in positive ionization mode on a quadrupole orthogonal acceleration time-of-flight mass spectrometer (Syntapt G2, Waters, Milford, MA) equipped with a standard electrospray probe and controlled by the MassLynx 4.1 software. Resolution of the instrument was set to 15,000 (resolution mode). The capillary voltage and cone voltage were set to 3 kV and 35 V, respectively. Accurate masses were obtained using the LockSpray source and leucine enkephalin (2 ng/μL in acetonitrile:water 1:1) as reference compound infused at 3 μL/min. The chromatographic system consisted of an ultra-performance liquid chromatography (UPLC) system (Acquity H-class, Waters, Milford, MA). Separations were performed on a reversed phase C18 column (Acquity HSS T3 1.8 μm 1 × 50 mm) at a flow rate of 150 μL/min. The injection volume was 5 μL. A linear gradient of acetonitrile in water (2–22% in 10 min) was applied. Mass spectra in the mass range m/z 100–700 were acquired at a rate of one spectrum per second.
Evaluation of the Antagonistic Activity in Greenhouse Conditions
A greenhouse experiment was performed to assess the biocontrol activity of a mixture of the two selected bacteria (AD117 and ST15.15/027) against Agrobacterium biovar 1 in a commercial hydroponic tomato production system in Belgium (Research Centre Hoogstraten, Belgium). Experiments were performed using the tomato cultivar “Rebelski” (De Ruiter, The Netherlands), rootstock Maxifort (De Ruiter, The Netherlands). Four plants were planted in one rockwool mat with a plant density of 2.5 plants / m2. From the start of the experiment, i.e., from the moment of planting of ~60-day-old tomato seedlings (January 2016), a set of 20 plants (5 rockwool mats) were treated by adding a mixture of 50 mL of the two candidate BCO (108 cells/mL each) to the rockwool mat daily for 10 days, while another set of 40 plants remained untreated. From day ten of the experiment, all plants were artificially infected by applying a rhizogenic Agrobacterium biovar 1 strain (isolate ST15.13/097; 50 mL of a suspension of 108 cells/mL) once a week for a total of 6 weeks to the rockwool mats. Plants were visually evaluated every 2 weeks for a total examination period of 8 weeks (until 17 weeks after infection) for development of aberrant root formation. In order to confirm that symptomatic roots were caused by Agrobacterium a qPCR analysis was performed on investigated root material to detect the presence of Agrobacterium biovar 1 DNA (Bosmans et al., 2016a). Due to capacity limitations, the experiment was conducted only once. Data were statistically analyzed using Generalized Estimating Equations (Liang and Zeger, 1986).
Results
Antagonistic Activity against Rhizogenic Agrobacteria
Out of 130 tested bacterial strains belonging to different phyla and different classes, Paenibacillus strain AD117 showed antibacterial activity against the tested rhizogenic Agrobacterium strain (ST15.13/097; Table 1, Table S1, Supporting Information). Additional screening of other Paenibacillus strains resulted in four additional antagonistic strains, including the type strain of Paenibacillus xylanilyticus (DSM17255T; the superscript “T” in the strain identifier indicates that this strain is the type strain of the species) and three Paenibacillus strains that were not yet assigned to the species level (ST15.15/027, ST15.15/031, and ST15.15/032; Table 2). Overall, for these strains the average diameter of the inhibition zones varied between 1.57 and 2.88 cm. Inhibition zones were significantly (P < 0.05) larger for strains AD117 (average diameter of inhibition zone 2.88 cm) and ST15.15/027 (2.79 cm; Figure S1, Supporting Information). 16S rRNA gene sequence analysis using the EZTaxon database showed that the strains AD117, ST15.15/027, ST15.15/031, and ST15.15/032 had highest sequence homology with Paenibacillus illinoisensis (ST15.15/031 and ST15.15/032) and P. xylanexedens (AD117 and ST15.15/027; Table 2). Examination of the growth characteristics of the five selected strains revealed highest growth rates for AD117, DSM17255T and ST15.15/027, irrespective of the growth medium used (Figure S2, Supporting Information). Phylogenetic analysis with all validly named Paenibacillus species (163 species) revealed that these five strains clustered tightly with P. illinoisensis, P. xylanilyticus, P. taichungensis, P. pabuli, P. tundra, P. tylopili, and P. xylanexedens (Figure 1). Additionally, when the type strains of these species were subjected to the agar overlay assay, all strains demonstrated antagonistic activity, while strains that were less related to this cluster did not (Table 2).
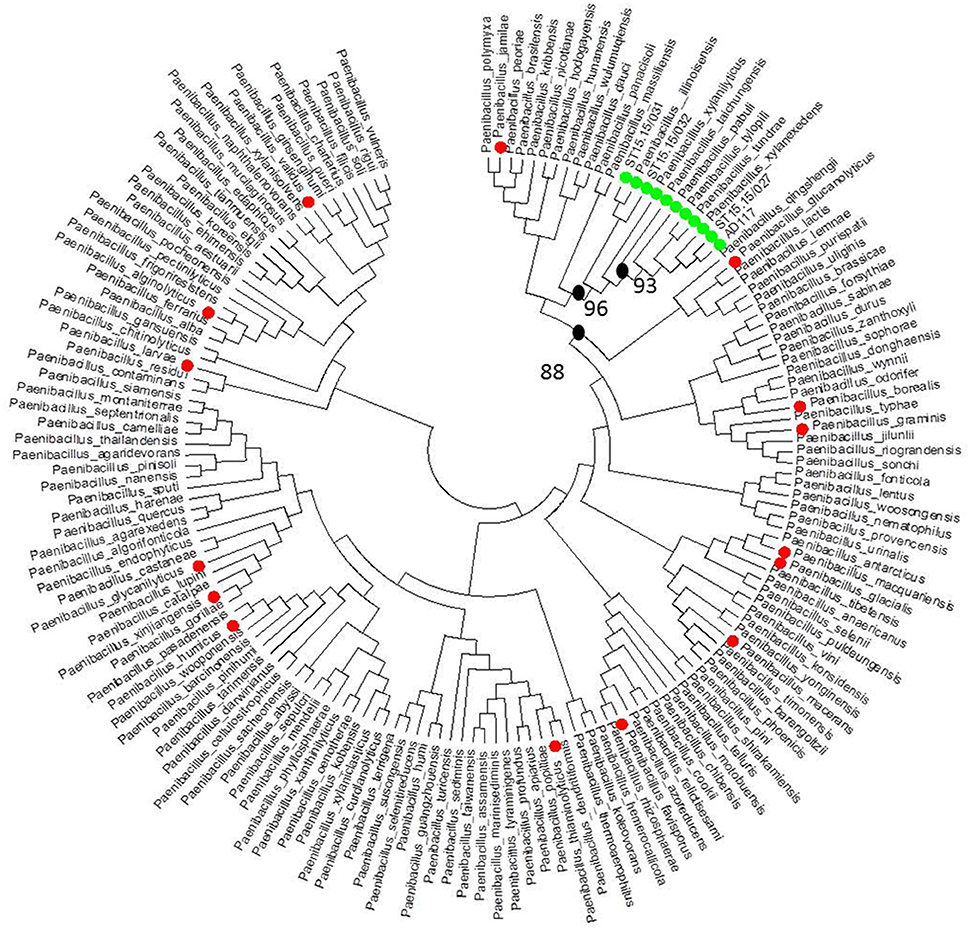
Figure 1. Phylogenetic positioning of Paenibacillus strains showing antagonistic activity against rhizogenic Agrobacterium biovar 1 strains. A maximum likelihood (ML) tree was constructed based on 16S rRNA gene sequences (1390 bp) for all reference (type) strains of all Paenibacillus species (EZtaxon) currently described (163 species) and all other Paenibacillus strains included in this study (Table 2). Only members of a tight cluster of Paenibacillus strains were found to have antagonistic activity against rhizogenic agrobacteria, while strains that were less related to this cluster were not antagonistic. Paenibacillus strains that were tested for antagonistic activity against Agrobacterium biovar 1 (isolate ST15.13/097) are marked with a green or red dot, representing antagonistic or non-antagonistic strains, respectively. Strains without colored dots were not tested for antagonistic activity against Agrobacterium biovar 1. Major bootstrap values (>85%; 1000 replications) are shown at the nodes of the tree.
Assessment of the spectrum of antagonistic activity of strains AD117, DSM15255T, ST15.15/027, ST15.15/031, and ST15.15/032 revealed that three strains (AD117, DSM17255T and ST15.15/027) showed antagonistic activity against all rhizogenic Agrobacterium biovar 1 strains (35) tested (Table 3). In contrast, the isolates corresponding to P. illinoisensis, ST15.15/031, and ST15.15/032, showed a different activity spectrum and were only able to inhibit the growth of 19 and 17 Agrobacterium biovar 1 strains, respectively (Table 3). Furthermore, strains AD117, DSM17255T and ST15.15/027 were able to supress the growth of one or more rhizogenic Agrobacterium biovar 2 strains causing HRD on Rosaceae. Additionally, strain ST15.15/027 showed antagonistic activity against Rhizobium vitis LMG256, a plant pathogen causing crown gall of grapevine (Table 3).
Preliminary Characterization of the Antagonistic Compound(s)
Based on the results described above (size of the zone of inhibition, spectrum of activity and general growth characteristics), both AD117 and ST15.15/027 were selected for further experiments to identify the active substances mediating the antagonistic effects observed. First, strains were evaluated for the production of volatile organic compounds (VOCs) with antagonistic activity against rhizogenic agrobacteria, but no VOC-dependent activity could be detected (agrobacterial growth was recorded daily for three consecutive days). In contrast, when the cell-free culture filtrates were tested, a dose-dependent growth inhibition of Agrobacterium was observed (Figure 2), suggesting that the selected bacteria secrete water-soluble antibacterial compounds. HPLC fractionation of an extract from the agar cut from the inhibition zones in the agar overlay assay was performed and gave one fraction with antagonistic activity (Figure S3, Supporting Information). For each isolate, mass spectrometry analysis of this HPLC fraction showed the presence of four specific peaks having a mass number of m/z = 463.2030, 477.1830, 504.2669, and 578.2324.
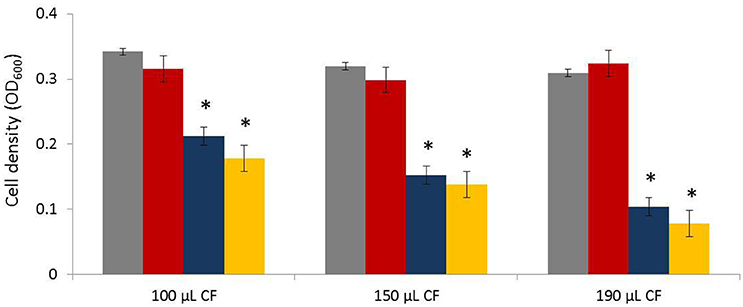
Figure 2. Antagonistic activity of cell-free culture filtrates of selected Paenibacillus strains with biocontrol potential against rhizogenic Agrobacterium biovar 1 [AD117 (blue) and ST15.15/027 (yellow)]. Paenibacillus strains were grown in liquid TSB-based medium (3 g/L TSB, 5 g/L NaCl and 1 g/L KH2PO4). Subsequently, cultures of 104 cells per mL were filter-sterilized and 100 μL, 150 μL and 190 μL of the cell-free filtrates (CF) were added to 100, 50, and 10 μL Agrobacterium-containing LB (isolate ST15.13/097), respectively. For each test medium, a negative control (gray) was included in which the volume of the culture filtrate was replaced by fresh TSB-based medium. Further, the cell-free culture filtrate of a Paenibacillus strain without antagonistic activity (LMG6324) was included as a control (red). For each condition (200 μL test volume), an agrobacterial cell concentration of 5 × 102 cells per mL was tested. Bacterial growth (OD600) was measured after 24 h of incubation at 25°C. Presented data are means of two independent experiments (two replicates per experiment) and error bars represent standard error of the mean. The asterisk indicates a statistically significant difference (Student t-test) with the corresponding control in which the culture filtrate was replaced by fresh TSB-medium (p < 0.05).
Greenhouse Experiments
A mixture of AD117 and ST15.15/027 was evaluated for its biocontrol potential of rhizogenic agrobacteria in greenhouse conditions. To this end, two sets of 20 plants were scored weekly for development of excessive root formation. Nine weeks after artificial infection with Agrobacterium, the first symptoms of HRD were observed. After 17 weeks about 75% of all control plants artificially infected with Agrobacterium showed HRD. When plants were treated with a mixture of AD117 and ST15.15/027 incidence of HRD dropped to 45% (Figure 3), which was significantly different from the control treatment. Observation of HRD symptoms was always confirmed by a positive qPCR analysis targeting Agrobacterium biovar 1 DNA.
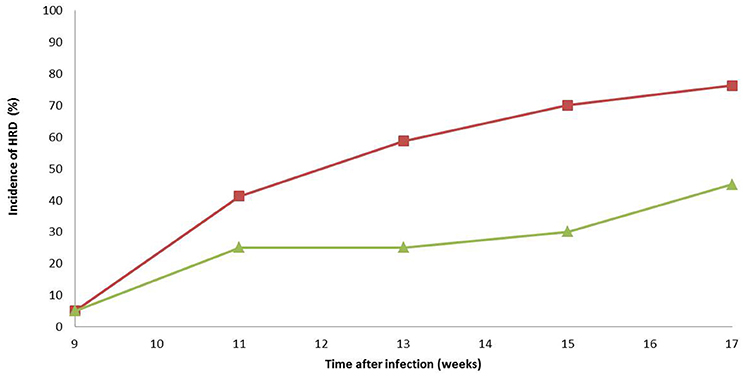
Figure 3. Biocontrol activity of a mixture of Paenibacillus strains (AD117 and ST15.15/027) against rhizogenic agrobacteria causing HRD (isolate ST15.13/097) in greenhouse conditions. Incidence of HRD (calculated as the ratio of infected tomato plants) is plotted as a function of time (weeks after initial infection with Agrobacterium): red, control plants (n = 20); green, plants treated with the BCO mixture (n = 20). Starting on day 10 of the experiment, all hydroponically grown plants were weekly infected with Agrobacterium (isolate ST15.13/097) for 6 weeks in total. Plants were visually evaluated every 2 weeks for development of excessive root formation. Observation of symptoms was confirmed by a positive qPCR analysis specifically targeting Agrobacterium biovar 1 DNA. The experiment was conducted once. Statistical analysis using Generalized Estimating Equations revealed that both treatments were significantly different at week 17.
Discussion
HRD caused by rhizogenic Agrobacterium biovar 1 strains is an economically important disease in the hydroponic cultivation of cucurbits and tomato leading to significant losses in marketable yield. As different lineages of rhizogenic Agrobacterium strains are able to form biofilms in which they can be protected from chemical disinfectants (Bosmans et al., 2015), or are able to tolerate high disinfectant concentrations (Bosmans et al., 2016c) or even diverse antibiotics (Khodykina et al., 2014), there is an urgent need for alternative, effective means to prevent, and control the disease including the use of biocontrol organisms.
After an extensive evaluation of a diverse bacterial collection several Paenibacillus strains were found to have antagonistic activity against rhizogenic Agrobacterium biovar 1 strains. Antagonistic strains included the type strain of P. xylanilyticus (DSM17255T), two strains putatively identified as P. illinoisensis (ST15.15/031 and ST15.15/032) and two strains putatively identified as P. xylanexedens (AD117 and ST15.15/027). Paenibacillus species have been isolated from various ecological habitats including soil, air, rhizosphere, and extreme environments such as floral plant nectar, warm water springs, and glaciers (McSpadden Gardener, 2004; Jacquemyn et al., 2013). The wide range of habitats from which the identified strains have been previously isolated include air (DSM17255T), rhizosphere (AD117; De Ridder-Duine et al., 2005), malting wheat kernels (ST15.15/031, ST15.15/032; Malfliet et al., 2013), and oak bourbon casks used to age beer (ST15.15/027), which suggests that antagonistic activity against rhizogenic agrobacteria is not related to the original (natural) habitat of the strains, and that antagonistic activity is not dependent on a history of previous contact with the pathogen (see also Duffy et al., 2003). However, positioning of these strains in a phylogenetic tree containing 16S rRNA gene sequences of the reference (type) strains of all validly named Paenibacillus species revealed that these five strains clustered tightly together with the type strains of P. illinoisensis, P. xylanilyticus, P. taichungensis, P. pabuli, P. tundra, P. tylopili, and P. xylanexedens. Similar results were obtained when a phylogenetic analysis was performed using rpoB sequences (encoding the β subunit of the bacterial RNA polymerase; although fewer sequences were available for type strains; Figure S4, Supporting Information), confirming their close phylogenetic relatedness. Furthermore, when these strains were subjected to the agar overlay assay, they all exhibited antagonistic activity against rhizogenic agrobacteria, suggesting phylogenetic conservation in antagonistic activity. However, further analysis with more (antagonistic and agrobacterial) strains should be performed to draw strong conclusions. Other studies have also reported on a correlation between antimicrobial activity and phylogeny. For example, Satheeja and Jebakumar (2011) showed that the antimicrobial activities of Streptomyces isolates were linked to their phylogenetic position. Likewise, Wilson et al. (2010) found a correlation between the antimicrobial activities of marine bacteria and the phylogeny of the isolates investigated. Several studies have shown antagonistic properties of Paenibacillus species or demonstrated their potential as biocontrol agents to control plant diseases caused by bacteria, fungi and oomycetes (Tjamos et al., 2004; Jung et al., 2005; Haggag and Timmusk, 2008; Timmusk et al., 2009; Algam et al., 2010; Sato et al., 2014). However, to the best of our knowledge, our study is the first in which a correlation was found between a distinct phylogenetic clade and antagonistic activity against a particular bacterial pathogen. All antagonistic strains were found to have the following 16S rRNA gene signature sequence differentiating antagonistic from non-antagonistic strains: 5′-TTGGGACAACTACCGGAAACGGTAGCTAATACCGAATA-3′.
Strikingly, differences were observed between the activity spectrum of the phylogenetically-clustered antagonistic Paenibacillus strains. More particularly, while isolates AD117, DSM17255T and ST15.15/027 showed antagonistic activity against all rhizogenic Agrobacterium biovar 1 isolates tested (35 isolates), the two isolates identified as P. illinoisensis, (ST15.15/031 and ST15.15/032) showed a different activity spectrum inhibiting the growth of different Agrobacterium strains and were only antagonistic against some (approximately 50%) of the strains tested. Rhizogenic Agrobacterium biovar 1 comprises a group of different genetic lineages exhibiting substantial genetic diversity (Bosmans et al., 2015). Nevertheless, no correlation could be found between the genetic background of the tested Agrobacterium strains and their vulnerability/resistance to these Paenibacillus strains. This also suggests that different modes of action are at play explaining antagonistic activity against rhizogenic Agrobacterium biovar 1. Interestingly, strains AD117, DSM17255T, and ST15.15/027 were also able to supress the growth of one or more rhizogenic Agrobacterium biovar 2 strains, which cause HRD on other crops such as Rosaceae (Cervera et al., 1998). Additionally, strain ST15.15/027 showed antagonistic activity against Rhizobium vitis. Preliminary characterization of the antagonistic compounds of AD117 and ST15.15/027 revealed that the compounds are water-soluble molecules of low molecular weight (<600 Da). There also seems to be an important role of Ca2+ to produce and/or secrete potential toxins/antibiotics against rhizogenic agrobacteria (Bosmans et al., 2016c). Further research, however, is necessary to structurally identify and characterize these compounds. The fact that they are water-soluble opens the possibility of their application and efficacy in hydroponic systems. Indeed, when the paenibacilli were evaluated in a commercial hydroponic tomato production system, a significant reduction in incidence of HRD (45 vs. 75% for the control treatment) was obtained when plants were evaluated over a period of about 4 months. Although these results are highly promising, it has to be noted that plants were not tested until the fruit bearing stage. Therefore, in order to draw firm conclusions, additional research over a longer period is needed, in which also a negative control without Agrobacterium is included. Additionally, it would be of interest to know the effect of each strain without mixing as sometimes antagonistic reactions happen between biological control agents (Xu et al., 2011). Further, it is reasonable to assume that biocontrol efficacy can be enhanced by frequent application of the BCO.
Altogether, we have shown that Paenibacillus holds great potential to control HRD. Furthermore, we have shown that its antagonistic activity against rhizogenic agrobacteria is correlated with the phylogeny of the Paenibacillus strains, but not with the phylogeny of the agrobacteria. Together with its plant-growth promoting traits (Lamsal et al., 2013), this makes Paenibacillus an excellent candidate for practical applications in the hydroponic cultivation of cucurbits and tomato crops.
Author Contributions
All authors listed, have made substantial, direct and intellectual contribution to the work, and approved it for publication.
Conflict of Interest Statement
The authors declare that the research was conducted in the absence of any commercial or financial relationships that could be construed as a potential conflict of interest.
Acknowledgments
We thank VLAIO (Flanders Innovation & Entrepreneurship) for support of this research (project IWT-LA: 120761).
Supplementary Material
The Supplementary Material for this article can be found online at: http://journal.frontiersin.org/article/10.3389/fmicb.2017.00447/full#supplementary-material
References
Algam, S. A. E., Xie, G., Li, B., Yu, S., Su, T., and Larsen, J. (2010). Effects of Paenibacillus strains and chitosan on plant growth promotion and control of Ralstonia wilt in tomato. J. Plant. Pathol. 92, 593–600. doi: 10.4454/jpp.v92i3.303
Bosmans, L., De Bruijn, I., De Mot, R., Rediers, H., and Lievens, B. (2016b). Agar composition affects in vitro screening of biocontrol activity of antagonistic microorganisms. J. Microbiol. Meth. 127, 7–9. doi: 10.1016/j.mimet.2016.05.004
Bosmans, L., Paeleman, A., Moerkens, R., Wittemans, L., Van Calenberge, B., Van Kerckhove, S., et al. (2016a). Development of a qPCR assay for detection and quantification of rhizogenic Agrobacterium biovar 1 strains. Eur. J. Plant. Pathol. 145, 719–730. doi: 10.1007/s10658-016-0861-6
Bosmans, L., Álvarez-Pérez, S., Moerkens, R., Wittemans, L., Van Calenberge, B., Van Kerckhove, S., et al. (2015). Assessment of the genetic and phenotypic diversity among rhizogenic Agrobacterium biovar 1 strains infecting solanaceous and cucurbit crops. FEMS Microbiol. Ecol. 91:fiv081. doi: 10.1093/femsec/fiv081
Bosmans, L., Van Calenberge, B., Paeleman, A., Moerkens, R., Wittemans, L., Van Kerckhove, S., et al. (2016c). Efficacy of hydrogen peroxide treatment for control of hairy roots disease caused by rhizogenic agrobacteria. J. Appl. Microbiol. 121, 519–527. doi: 10.1111/jam.13187
Cervera, M., López, M. M., Navarro, L., and Pe-a, L. (1998). Virulence and supervirulence of Agrobacterium tumefaciens in woody fruit plants. Physiol. Mol. Plant Pathol. 52, 67–78. doi: 10.1006/pmpp.1997.0135
Damstra, T. (2002). Potential effects of certain persistent organic pollutants and endocrine disrupting chemicals on the health of children. J. Toxicol. Clin. Toxicol. 40, 457–465. doi: 10.1081/clt-120006748
Danhorn, T., and Fuqua, C. (2007). Biofilm formation by plant-associated bacteria. Annu. Rev. Microbiol. 61, 401–422. doi: 10.1146/annurev.micro.61.080706.093316
De Ridder-Duine, A. S., Kowalchuk, G. A., Klein Gunnewiek, P. J. A., Smant, W., van Veen, J. A., and De Boer, W. (2005). Rhizosphere bacterial community composition in natural stands of Carex arenaria (sand sedge) is determined by bulk soil community composition. Soil Biol. Biochem. 37, 349–357. doi: 10.1016/j.soilbio.2004.08.005
Duffy, B., Schouten, A., and Raaijmakers, J. M. (2003). Pathogen self-defense: mechanisms to counteract microbial antagonism. Annu. Rev. Phytopathol. 41, 501–538. doi: 10.1146/annurev.phyto.41.052002.095606
Gelvin, S. B. (2003). Agrobacterium-mediated plant transformation: the biology behind the ‘gene-jockeying’ tool. Microbiol. Mol. Biol. Rev. 67, 16–37. doi: 10.1128/MMBR.67.1.16-37.2003
Haggag, W. M., and Timmusk, S. (2008). Colonization of peanut roots by biofilm-forming Paenibacillus polymyxa initiates biocontrol against crown rot disease. J. Appl. Microbiol. 104, 961–969. doi: 10.1111/j.1365-2672.2007.03611.x
Hooykaas, P. J. J., and Beijersbergen, A. G. (1994). The virulence system of Agrobacterium tumefaciens. Annu. Rev. Phytopathol. 32, 157–181. doi: 10.1146/annurev.py.32.090194.001105
Jacquemyn, H., Lenaerts, M., Tyteca, D., and Lievens, B. (2013). Microbial diversity in the floral nectar of seven Epipactis (Orchidaceae) species. Microbiol. Open 2, 644–658. doi: 10.1002/mbo3.103
Jung, W. J., Jin, Y. L., Kim, K. Y., Park, R. D., and Kim, T. H. (2005). Changes in pathogenesis-related proteins in pepper plants with regard to biological control of phytophthora blight with Paenibacillus illinoisensis. Biol. Control 50, 165–178. doi: 10.1007/s10526-004-0451-y
Khodykina, M. V., Polityko, V. A., Kyrova, E. I., Krutyakov, Y. A., Zherebin, P. M., and Ignatov, A. N. (2014). Antibacterial activity of antibiotics combined with silver agent “Zeroks” against causing agents of bacterial plant diseases. Potato Prot. 2, 83–86.
Lamsal, K., Kim, S. W., Kim, Y. S., and Lee, Y. S. (2013). Biocontrol of late blight and plant growth promotion in tomato using rhizobacterial isolates. J. Microbiol. Biotechnol. 23, 897–904. doi: 10.4014/jmb.1209.09069
Liang, K.-Y., and Zeger, S. L. (1986). Longitudinal data analysis using generalized linear models. Biometrika 73, 13–22. doi: 10.1093/biomet/73.1.13
Ludeking, D., Hamelink, R., Wubben, J. P., Wubben, J., and Schenk, M. F. (2013). Aanpak van overmatige wortelgroei in vruchtgroentegewassen. Wageningen: UR Glastuinbouw 1244.
Malfliet, S., Justé, A., Crauwels, S., Willems, K. A., De Cooman, L., Lievens, B., et al. (2013). Assessing the xylanolytic bacterial diversity during the malting process. Food Microbiol. 36, 406–415. doi: 10.1016/j.fm.2013.06.025
McSpadden Gardener, B. B. (2004). Ecology of Bacillus and Paenibacillus spp. in agricultural systems. Phytopathology 94, 1252–1258. doi: 10.1094/PHYTO.2004.94.11.1252
Raaijmakers, J. M., Vlami, M., and De Souza, J. T. (2002). Antibiotic production by bacterial biocontrol agents. Antonie Van Leeuwenhoek 81, 537–547. doi: 10.1023/A:1020501420831
Rubino, F. M., Mandic-Rajcevic, S., Mrema, E. J., and Colosio, C. (2013). “Principles and application of the integrated pest management approach. biological pesticides,” in Environmental Security Assessment and Management of Obsolete Pesticides in Southeast Europe, eds L. Simeonov, F. Macae, and B. Simeonova (Dordrecht: Springer Netherlands), 413–432.
Satheeja, S. V., and Jebakumar, S. R. (2011). Phylogenetic analysis and antimicrobial activities of Streptomyces isolates from mangrove sediment. J. Basic Microbiol. 51, 71–79. doi: 10.1002/jobm.201000107
Sato, I., Yoshida, S., Iwamoto, Y., Aino, M., Hyakumachi, M., Shimizu, M., et al. (2014). Suppressive potential of Paenibacillus strains isolated from the tomato phyllosphere against Fusarium crown and root rot of tomato. Microbes Environ. 29, 168–177. doi: 10.1264/jsme2.ME13172
Sharma, R. R., Singh, D., and Singh, R. (2009). Biological control of postharvest diseases of fruits and vegetables by microbial antagonists: a review. Biol. Control 50, 205–220. doi: 10.1016/j.biocontrol.2009.05.001
Tamura, K., Peterson, D., Peterson, N., Stecher, G., and Nei, M. (2011). MEGA5: Molecular Evolutionary Genetics Analysis using maximum likelihood, evolutionary distance, and maximum parsimony methods. Mol. Biol. Evol. 28, 2731–2739. doi: 10.1093/molbev/msr121
Timmusk, S., Van West, P., Gow, N. A. R., and Paul Huffstutler, R. (2009). Paenibacillus polymyxa antagonizes oomycete plant pathogens Phytophthora palmivora and Pythium aphanidermatum. J. Appl. Microbiol. 106, 1473–1481. doi: 10.1111/j.1365-2672.2009.04123.x
Tjamos, E. C., Tsitsigiannis, D. I., Tjamos, S. E., Antoniou, P. P., and Katinakis, P. (2004). Selection and screening of endorhizosphere bacteria from solarized soils as biocontrol agents against Verticillium dahliae of solanaceous hosts. Eur. J. Plant Pathol. 110, 35–44. doi: 10.1023/B:EJPP.0000010132.91241.cb
Tyc, O., van den Berg, M., Gerards, S., van Veen, J. A., Raaijmakers, J. M., De Boer, W., et al. (2014). Impact of interspecific interactions on antagonistic activity among soil bacteria. Front. Microbiol. 5:567. doi: 10.3389/fmicb.2014.00567
Weller, S. A., Stead, D. E., and Young, J. P. W. (2006). Recurrent outbreaks of root mat in cucumber and tomato are associated with a monomorphic, cucumopine, Ri-plasmid harboured by various Alphaproteobacteria. FEMS Microbiol. Lett. 258, 136–143. doi: 10.1111/j.1574-6968.2006.00214.x
Wilson, G. S., Raftos, D. A., Corrigan, S. L., and Nair, S. V. (2010). Diversity and antimicrobial activities of surface-attached marine bacteria from Sydney Harbour, Australia. Microbiol. Res. 165, 300–311. doi: 10.1016/j.micres.2009.05.007
Keywords: Agrobacterium, antagonistic activity, biological control, high-throughput screening, Paenibacillus
Citation: Bosmans L, De Bruijn I, Gerards S, Moerkens R, Van Looveren L, Wittemans L, Van Calenberge B, Paeleman A, Van Kerckhove S, De Mot R, Rozenski J, Rediers H, Raaijmakers JM and Lievens B (2017) Potential for Biocontrol of Hairy Root Disease by a Paenibacillus Clade. Front. Microbiol. 8:447. doi: 10.3389/fmicb.2017.00447
Received: 16 November 2016; Accepted: 03 March 2017;
Published: 22 March 2017.
Edited by:
Brigitte Mauch-Mani, University of Neuchâtel, SwitzerlandReviewed by:
Lois Banta, Williams College, USADavid Ruano-Rosa, Instituto de Agricultura Sostenible (CSIC), Spain
Copyright © 2017 Bosmans, De Bruijn, Gerards, Moerkens, Van Looveren, Wittemans, Van Calenberge, Paeleman, Van Kerckhove, De Mot, Rozenski, Rediers, Raaijmakers and Lievens. This is an open-access article distributed under the terms of the Creative Commons Attribution License (CC BY). The use, distribution or reproduction in other forums is permitted, provided the original author(s) or licensor are credited and that the original publication in this journal is cited, in accordance with accepted academic practice. No use, distribution or reproduction is permitted which does not comply with these terms.
*Correspondence: Bart Lievens, YmFydC5saWV2ZW5zQGt1bGV1dmVuLmJl
†Shared last co-authorship.