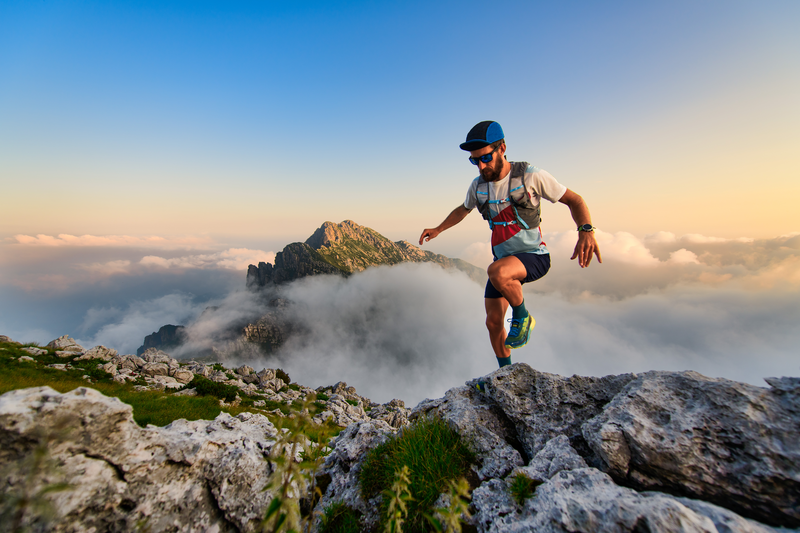
95% of researchers rate our articles as excellent or good
Learn more about the work of our research integrity team to safeguard the quality of each article we publish.
Find out more
ORIGINAL RESEARCH article
Front. Microbiol. , 20 July 2016
Sec. Aquatic Microbiology
Volume 7 - 2016 | https://doi.org/10.3389/fmicb.2016.01111
Ecological evidence suggests that heterotrophic diazotrophs fueled by organic carbon respiration in sediments play an important role in marine nitrogen fixation. However, fundamental knowledge about the identities, abundance, diversity, biogeography, and controlling environmental factors of nitrogen-fixing communities in open ocean sediments is still elusive. Surprisingly, little is known also about nitrogen-fixing communities in sediments of the more research-accessible marginal seas. Here we report on an investigation of the environmental geochemistry and putative diazotrophic microbiota in the sediments of Bohai Sea, an eutrophic marginal sea of the western Pacific Ocean. Diverse and abundant nifH gene sequences were identified and sulfate-reducing bacteria (SRB) were found to be the dominant putative nitrogen-fixing microbes. Community statistical analyses suggested bottom water temperature, bottom water chlorophyll a content (or the covarying turbidity) and sediment porewater Eh (or the covarying pH) as the most significant environmental factors controlling the structure and spatial distribution of the putative diazotrophic communities, while sediment Hg content, sulfide content, and porewater -Si content were identified as the key environmental factors correlated positively with the nifH gene abundance in Bohai Sea sediments. Comparative analyses between the Bohai Sea and the northern South China Sea (nSCS) identified a significant composition difference of the putative diazotrophic communities in sediments between the shallow-water (estuarine and nearshore) and deep-water (offshore and deep-sea) environments, and sediment porewater dissolved oxygen content, water depth and in situ temperature as the key environmental factors tentatively controlling the species composition, community structure, and spatial distribution of the marginal sea sediment nifH-harboring microbiota. This confirms the ecophysiological specialization and niche differentiation between the shallow-water and deep-water sediment diazotrophic communities and suggests that the in situ physical and geochemical conditions play a more important role than geographical contiguity in determining the community similarity of the diazotrophic microbiota in marginal sea sediments.
N2-fixing prokaryotes play a key role in marine nitrogen (N) cycling and ecosystem functioning such as carbon sequestration by providing newly fixed nitrogenous nutrients, particularly important in oligotrophic environments of the ocean (Karl et al., 1997; Dore et al., 2002; Montoya et al., 2004; Steppe and Paerl, 2005; Karl and Letelier, 2008; Sohm et al., 2011). Marine N2 fixation studies have been conducted for more than half a century, yet many diazotrophic microbes, their ecophysiology and environmental response have been revealed only in recent decades (reviewed by Zehr and Kudela, 2011; Voss et al., 2013). The marine N cycle appears to be a conundrum because the estimated N input by N2 fixation is significantly lower than the estimated N loss via denitrification and anaerobic ammonium oxidation (anammox) (Mahaffey et al., 2005). This suggested that the inventory of reactive nitrogen in the oceans is unbalanced and dwindling (Moisander et al., 2014). However, this conundrum may be an artifact caused by uncertainties in previous research results and underestimations of N2-fixation contribution to the marine N budget. These include insufficient and inaccurate measurements of the N2 fixation rate (Deutsch et al., 2007; Großkopf et al., 2012), undiscovered N2-fixing microbes (Zehr et al., 2001, 2008; Pernthaler et al., 2008; Dekas et al., 2009; Zehr, 2011; Voss et al., 2013), overlooked robustness of N2-fixing physiology of environmental diazotrophs (Knapp, 2012), and overlooked and undiscovered N2-fixing environments such as nutrient-rich estuarine and coastal seas and marine sediments (Mehta et al., 2003; Pernthaler et al., 2008; Bonnet et al., 2013; Voss et al., 2013). Historically, marine N2 fixation was thought to be carried out mainly by aggregate-forming cyanobacteria such as Trichodesmium and important only in surface and near-surface waters of the ocean (Paerl, 1990; Dore et al., 2002; Karl et al., 2002; Bergman et al., 2013). However, the water column below the photic layer has been found to harbor significant N2 fixation activities in many marine waters, which were mainly carried out by heterotrophic diazotrophs either in hypoxic and anoxic environments or in marine particle-associated microenvironments (Farnelid et al., 2011, 2013; Fernandez et al., 2011; Jayakumar et al., 2012; Bird and Wyman, 2013; Rahav et al., 2013; Dang and Lovell, 2016). Furthermore, the recent detection of numerous diazotrophic bacteria and archaea in marine sediments led to the hypothesis that marine sediments might constitute an important environment for N2 fixation in the oceans (Pernthaler et al., 2008; Dang et al., 2009a, 2013a; Dekas et al., 2009, 2014, 2016; Fulweiler, 2009; Miyazaki et al., 2009).
Hypoxic and anoxic environments can be formed and maintains more easily in marine sediments than in the water column, particularly in eutrophic coastal seas. Nitrogen gas is abundant in marine sediments, partially provided by microbial denitrification and anammox processes (Dang et al., 2009b; Trimmer and Nicholls, 2009; Shao et al., 2014). Therefore, heterotrophic rather than cyanobacterial diazotrophy may play a dominant role of N2 fixation in marine sediments (Bertics et al., 2013; Dekaezemacker et al., 2013). N2 fixation by heterotrophic diazotrophs requires a high amount of cellular energy that is mainly provided by respiration of large amounts of organic carbon (Shanmugam et al., 1978; Brill, 1980; Dang and Jiao, 2014). It has been speculated that the low N2-fixing rates of heterotrophic diazotrophs in the open ocean is caused by the lack of sufficient metabolic energy due to the scarcity of bioavailable organic carbon (Moisander et al., 2014). On the other hand, estuarine and coastal sediments may support high rates of N2 fixation by heterotrophic diazotrophs due to enhanced phytoplankton labile organic matter production under the impact of anthropogenic eutrophication and terrigenous nutrient inputs (Herbert, 1999; Boesch, 2002; Smith, 2003). Moreover, iron, phosphorus or both have been found to be the key factors limiting microbial N2 fixation and export production in many waters of the oligotrophic open oceans (Sañudo-Wilhelmy et al., 2001; Mills et al., 2004; Moore et al., 2009, 2013; Boyd and Ellwood, 2010; Sohm et al., 2011; Jacq et al., 2014). In estuarine and coastal sediments, iron and phosphorus may be sufficiently abundant to support high rates of microbial N2 fixation (Street and Paytan, 2005; Homoky et al., 2013; Karl, 2014). Furthermore, it is evident that the sediment diazotrophic communities are highly resistant to the inhibition of high environmental and concentrations (McGlathery et al., 1998; Knapp, 2012; Bertics et al., 2013). It was thus reasonably hypothesized that the marginal sea sediments may prove to be key environments of N2 fixation. However, fundamental knowledge about the identities, diversity, biogeography, and controlling environmental factors of sediment N2-fixing microbes is still lacking.
It has been reported that the sediments of the northern South China Sea (nSCS), a large and relatively oligotrophic marginal sea of the western Pacific Ocean, might harbor the highest diazotroph diversity among all the marine environments ever investigated using molecular ecology approaches targeting the nifH gene (encoding the nitrogenase reductase subunit) (Dang et al., 2013a). The Bohai Sea, another marginal sea of the western Pacific Ocean, is conversely characterized by its eutrophic status caused by high degree of river inputs and anthropogenic pollutions and by its low water exchange with the outer ocean due to its semi-enclosed topography (Figure 1; Dang et al., 2013b). To answer the question how diazotrophic communities vary and respond to distinct environmental conditions, sediment nifH-harboring microbial assemblages in the Bohai Sea were investigated in an environmental geochemistry context and comparatively analyzed against the putative diazotrophic assemblages of the nSCS.
Figure 1. Map of the Bohai Sea and sampling station sites. The insert map shows the geographical location of the Bohai Sea in the western Pacific Ocean. Abbreviation and numerical symbols: BS, Bohai Sea; 1, Haihe River; 2, Luanhe River; 3, Dalinghe River; 4, Liaohe River; and 5, Daliaohe River (Dang et al., 2013b).
Bohai Sea is a large shallow water basin with an area of 77 × 103 km2 and an average water depth of only 18 m. It is nearly enclosed and thus its water exchange capacity with the outer Yellow Sea of the western Pacific Ocean is highly limited (Figure 1). Moreover, more than 40 rivers discharge into the Bohai Sea. Thus, the Bohai Sea receives intense and extensive terrigenous and anthropogenic impacts, especially in its estuarine and coastal bay areas (PEMSEA and BSEMP, 2005).
Samples of surface sediments in the top 0–5 cm layer were collected from 14 stations of the Bohai Sea (Figure 1) in the August of 2008 as described previously (Dang et al., 2013b). At each station, seawater physicochemical and biological parameters (Supplementary Table S1) were measured in situ at various water depths with a Compact-CTD equipped with a TCDKU sensor (Alec Electronics, Japan) as reported in a previous publication (Dang et al., 2013b), which also contained most of the sediment and sediment porewater environmental parameters, except the sediment V and S contents that were measured in the current study via a VARIAN 725-ES inductively coupled plasma-optical emission spectrometer (Varian, Palo Alto, CA, USA) (Supplementary Table S1).
Community genomic DNA of sediment microbes was extracted by using a FastPrep DNA Extraction Kit for Soil and a FastPrep-24 Cell Disrupter (MP Biomedicals, Solon, OH, USA) as previously described (Dang et al., 2013a,b). DNA concentrations were measured with dye PicoGreen (Molecular Probes, Eugene, OR, USA) and a Modulus Single Tube Multimode Reader fluorometer (Turner BioSystems, Sunnyvale, CA, USA). Partial nifH gene sequences were amplified with PCR primers nifHfw and nifHrv, which have a very broad coverage for both bacterial and archaeal nifH genes (Mehta et al., 2003). In order to test reproducibility of our experimental procedure and to identify any potential within-site variability of the sediment diazotrophic community, two separate nifH gene clone libraries (B22-1 and B22-2) were constructed for the sediments of sampling station B22, each from a distinct subcore DNA sample, respectively. PCR product cloning followed previous procedures (Dang et al., 2009a, 2013a). A miniprep method was used for recombinant plasmid extractions (Dang and Lovell, 2000). Cloned gene fragments were reamplified to check the correct size of the DNA inserts using cloning vector PCR primers M13-D and RV-M (Dang et al., 2008), which were also used for sequencing with an ABI 3770 sequencer (Applied BioSystems, Foster City, CA, USA). The obtained nifH gene sequences have been submitted to GenBank with accession numbers KM524369 to KM525656.
The nifH sequences were translated into conceptual protein sequences and the BLASTp program was used for retrieval of the top-hit NifH sequences from GenBank (last accessed 25 January 2014) (Altschul et al., 1997). The NifH protein sequences were grouped into operational taxonomic units (OTUs) using a 0.05 sequence distance cutoff calculated by using the DOTUR program (Schloss and Handelsman, 2005). These OTU sequences, along with reference sequences retrieved from GenBank, were aligned with program CLUSTAL X (version 2.1; Larkin et al., 2007), and used for phylogeny inference with the distance and neighbor-joining method implemented within the PHYLIP software package (version 3.69; Felsenstein, 1989), following a previously reported procedure (Dang et al., 2009a, 2013a).
The technique of real-time fluorescent quantitative PCR (qPCR) was employed to measure the abundance of the sediment nifH genes using primers nifHfw and nifHrv (Mehta et al., 2003; Dang et al., 2013a). Triplicate sediment DNA samples from each station were assayed with an ABI Prism 7500 Sequence Detection System (Applied Biosystems, Foster City, CA, USA), following a previously published SYBR Green qPCR protocol (Dang et al., 2013a). Plasmids containing the nifH gene inserts were extracted from Escherichia coli hosts using a Mini Plasmid Kit (Qiagen, Valencia, CA, USA), linearized with an endonuclease specific in the cloning vector region, and quantified using PicoGreen and a Modulus Single Tube Multimode Reader fluorometer (Dang et al., 2013a). A qPCR standard curve was then generated with serially diluted linearized plasmids containing the target nifH gene fragment.
The qPCR condition for nifH gene quantification was optimized based on a previous study (Dang et al., 2013a). The efficiency and sensitivity of the qPCR were shown in Supplementary Table S2, along with the range of the plasmid copy numbers used for the qPCR standard curve construction. In all experiments, negative controls lacking template DNA were run with the same qPCR procedure to prevent contamination or carryover. Agarose gel electrophoresis and melting curve analysis were performed to confirm the specificity of the qPCR reactions.
Clone library coverage (C) was calculated as C = [1 − (n1/N)] × 100, where n1 is the number of unique OTUs and N the total number of clones in a library (Mullins et al., 1995). Shannon-Wiener (H), Simpson (D), and evenness (J) indices were calculated with the OTU data (Dang et al., 2013a). The DOTUR program (Schloss and Handelsman, 2005) was used for clone library rarefaction analysis and to calculate the abundance-based coverage estimator (SACE) and the bias-corrected richness estimator Chao1 (SChao1). The Fast UniFrac program (Hamady et al., 2010) was used for nifH-harboring microbial community clustering and principal coordinates analyses (PCoA). The Canoco software (version 4.5, Microcomputer Power, Ithaca, NY, USA) was used for the canonical correspondence analysis (CCA) (ter Braak and Šmilauer, 2002; Lepš and Šmilauer, 2003), to investigate any correlations between the nifH-harboring microbial communities and environmental factors by following a previously described procedure (Dang et al., 2013a). The SPSS software (version 17.0) was used for Pearson correlation analyses (significance level α = 0.05) of the sediment nifH gene abundance with environmental factors (Dang et al., 2013a).
The Fast UniFrac program (Hamady et al., 2010) was also used for clustering analyses of the sediment nifH-harboring microbial communities in both the Bohai Sea and the previously studied nSCS (Dang et al., 2013a), to identify larger geographical-scale characteristics of the putative diazotrophic microbiota. CCA analysis (ter Braak and Šmilauer, 2002; Lepš and Šmilauer, 2003) was also performed to tentatively identify the key environmental factors that may control the biogeographical distribution of the sediment nifH-harboring microbial communities in both marginal seas.
Community clustering (Supplementary Figure S1) and PCoA (Supplementary Figure S2) analyses using Fast UniFrac software revealed that the two nifH gene clone libraries (B22-1 and B22-2) constructed from separate sediment subcore samples of station B22 were highly similar. These statistical results demonstrated the reproducibility of our experimental procedures and the negligibility of within-site variability of the putative diazotrophic community detected by using the nifH gene biomarker. Therefore, in subsequent analyses, these two nifH gene clone libraries (B22-1 and B22-2) were pooled as a single B22 clone library.
Of all the 14 nifH gene clone libraries constructed, a total of 1309 clones were found to contain a valid nifH gene fragment, which were further identified as 1288 unique nifH DNA sequences yielding 619 deduced unique NifH protein sequences and 246 OTUs. Based on the obtained biodiversity index values (Table 1), the sediment nifH genes were highly diverse and their richness was heterogeneously distributed in the different sampling stations of the Bohai Sea. The high diversity (Supplementary Figure S3, Table 1) indicated that the sequences in the constructed nifH gene clone libraries might only represent the dominant putative diazotrophs in the Bohai Sea sediments. In general, the coastal site B15 had the lowest nifH gene diversity and the sites B5, B16, B20, and B22 might have the highest nifH gene diversity (Supplementary Figure S3, Table 1).
Table 1. Biodiversity and predicted richness of the sediment nifH gene sequences obtained from the sampling stations of the Bohai Sea.
The obtained 1288 unique nifH gene sequences shared 37.5–99.7% sequence identity with one another and 51–99% sequence identity with the top-match sequences obtained from GenBank. Interestingly, the majority (87.7%) of the queried Bohai Sea sediment nifH gene sequences resulted in nifH top-match sequences derived from samples obtained from nSCS marine sediments (Dang et al., 2013a). The deduced 619 unique NifH protein sequences shared 33.0–99.2% sequence identity with one another and shared 53–100% sequence identity with the top-match GenBank NifH sequences. More than half (57.4%) of the 619 unique NifH sequences shared >90% sequence identity with known bacteria, such as Alphaproteobacteria, Gammaproteobacteria, Deltaproteobacteria, Chlorobi, Firmicutes, and Verrucomicrobia (Supplementary Table S3). Importantly, most of these bacteria with top-match NifH sequences are known diazotrophs. This result indicates that diverse obligately anaerobic sulfate-reducing bacteria (SRB) in the Deltaproteobacteria class, which accounted for 18.3% of the total OTUs and 48.2% of the total clones, probably constituted the majority of taxa of diazotrophic communities in Bohai Sea sediments (Supplementary Table S3, Supplementary Figure S4).
The deduced NifH sequences were affiliated with six major groups in the constructed NifH phylogenetic tree (Figure 2, Supplementary Figure S4, Table 2), according to a recently proposed NifH sequence phylogenetic classification (Dang et al., 2009a, 2013a). Class I contained 141 OTUs and could be further divided into four clusters (Clusters I, II, III, and Cluster IV subcluster A), in which Cluster III was the largest containing 102 OTUs (Supplementary Figure S4). Clusters I and III were the only clusters that contained the NifH sequences obtained from all 14 sampling stations; therefore, these sequences tentatively represent the most prevalent diazotrophic microbes in the Bohai Sea sediments. Sequence Classes II, III, V, VI, and VIII represented 19, 32, 10, 43, and 1 OTUs (Supplementary Figure S4), respectively, and the pertinent putative diazotrophic microbes might thus be specifically distributed only in certain sediment environments of the Bohai Sea.
Figure 2. Phylogenetic tree of the major Bohai Sea sediment NifH sequences constructed with the neighbor-joining method. The NifH sequences of the most abundant OTUs (no <5 clones) were used for tree construction. The tree branch distances represent the amino acid substitution rate, and the scale bar represents the expected number of changes per homologous position. Bootstrap values higher than 50% of 100 resamplings are shown near the corresponding nodes. The chlorophyllide reductase iron protein subunit BchX sequences from Rhodobacter capsulantus and R. sphaeroides were used as outgroup.
Table 2. The detail composition of the sequences (and OTUs) in the NifH phylogenetic tree obtained from the Bohai Sea sediments.
Community classification based on Fast UniFrac clustering analysis identified three distinct clusters of the nifH-harboring microbial assemblages in Bohai Sea sediments (Figure 3). The nifH-harboring microbial assemblages of stations B8 and B15 were grouped together and represented a distinct cluster, the assemblage of station B5 represented another distinct cluster formed by a singleton member, and the assemblages of the remaining stations were grouped together and formed a third distinct cluster. This community classification pattern of nifH-harboring microbial assemblages in the Bohai Sea sediments was further supported by the PCoA analysis (Figure 4).
Figure 3. Hierarchical clustering dendrogram of the Bohai Sea sediment nifH-harboring microbial assemblages. This dendrogram was constructed by using the Fast UniFrac weighted and normalized Jackknife Environment Clusters statistical method with the use of the NifH protein sequence data. The percentage supports of the classification tested with sequence jackknifing resamplings are shown near the corresponding nodes.
Figure 4. PCoA ordination diagram of the Bohai Sea sediment nifH-harboring microbial assemblages. This diagram was produced by using the Fast UniFrac weighted and normalized PCoA method with the use of the NifH protein sequence data. The P1 and P2 show the percent variations of the diazotroph assemblages explained by the first two principal coordinates.
CCA analysis was performed to decode the putative diazotroph-environment relationship in the Bohai Sea sediments (Supplementary Figure S5). Bottom water temperature (p = 0.001; 1000 Monte Carlo permutations), bottom water chlorophyll a content (or the covarying bottom water turbidity) (p = 0.015; 1000 Monte Carlo permutations) and sediment porewater Eh (or the covarying porewater pH; p = 0.032; 1000 Monte Carlo permutations) were identified as the most significant environmental factors that might control the structure and spatial distribution of the nifH-harboring microbial communities in Bohai Sea sediments.
The qPCR results showed that the abundance of the nifH genes ranged from 2.95 × 107 copies g−1 sediment (station B3) to 2.63 × 109 copies g−1 sediment (station B19) in the Bohai Sea (Table 3). The highest nifH gene abundance occurred at the sampling station B1, B19, and B14, respectively, in Liaodong Bay, Laizhou Bay, and Bohai Bay sediments, respectively. Our previous study showed that the total bacterial abundance was also heterogeneously distributed in the sediments of the Bohai Sea, with the bacterial 16S rRNA genes ranging from 3.25 × 109 copies g−1 sediment (station B16) to 2.10 × 1010 copies g−1 sediment (station B20) (Dang et al., 2013b). The ratios of the nifH gene abundance to the bacterial 16S rRNA gene abundance ranged from 0.36% (station B1) to 14.95% (station B19).
Pearson correlation analyses indicated that the sediment Hg content (p = 0.018), sulfide content (p = 0.046), and porewater -Si content (p = 0.026) were positively correlated with the nifH gene abundance in all investigated Bohai Sea sediments.
In order to identify the difference of the diazotrophic microbial communities in sediments from distinct marine environments and to detect any general ecological characteristics of the diazotrophic microbiota in sediments of different marginal seas of the western Pacific Ocean, comparative analyses were made between sediment samples from the Bohai Sea and the previously studied nSCS (Dang et al., 2013a). Community classification analyses indicated that the nifH-harboring microbial communities could be classified into two groups based on both unweighted (Figure 5A) and weighted and normalized (Figure 5B) Fast UniFrac clustering analyses, with Group I containing exclusively the nifH-harboring microbial assemblages at the nSCS offshore and deep-sea sampling stations (water depth > 130 m) and Group II containing the nifH-harboring microbial assemblages at all the Bohai Sea sampling stations as well as the shallow-water sampling stations A3 and E501 of the nSCS (water depth < 70 m). The estuarine and nearshore sediments probably harbor N2-fixing microbial assemblages distinctly different from those of the offshore and deep-sea sediments, regardless of the large geographical distance between the Bohai Sea and the nSCS. The CCA analysis further identified the sediment porewater dissolved oxygen content (DO), water depth and in situ sediment temperature (the bottom water temperature was used to approximate the sediment temperature of the Bohai Sea) as the key environmental factors (p = 0.001, p = 0.002, and p = 0.014, respectively; 1000 Monte Carlo permutations) influencing significantly the community structure and biogeographical distribution of the putative sediment diazotrophic microbiota in Bohai Sea and nSCS sediments (Figure 6).
Figure 5. Hierarchical clustering dendrograms of the Bohai Sea and northern South China Sea sediment nifH-harboring microbial assemblages. These dendrograms were constructed by using the Fast UniFrac unweighted (A) and weighted and normalized (B) Jackknife Environment Clusters statistical methods with the use of the NifH protein sequence data obtained from the current study and a previous study of the nSCS (Dang et al., 2013a). The percentage supports of the classifications tested with sequence jackknifing resamplings are shown near the corresponding nodes. The Bohai Sea sampling stations are labeled in red color and the northern South China Sea sampling stations are labeled in blue color.
Figure 6. CCA ordination diagram of the relationship between the sediment nifH-harboring assemblages and environmental factors in the Bohai Sea and northern South China Sea. This diagram was obtained by using the NifH OTU data obtained from the current study and a previous study of the northern South China Sea (Dang et al., 2013a).
Nitrogenous nutrient-rich environments such as estuarine and coastal seawaters and marine sediments have long been regarded as environments lacking significant diazotrophic activities, inferred previously from bacterial culture-based physiological studies. This inferred popular opinion resulted in a long-time negligence of the N2-fixing microorganisms and activities in these environments whereas newer work led to the hypothesis that marginal sea sediments may instead harbor diverse and abundant N2-fixing microorganisms (Knapp, 2012; Bertics et al., 2013; Dang et al., 2013a; Dekaezemacker et al., 2013; Voss et al., 2013). To test this hypothesis, we probed the genetic potential of N2 fixation in marine sediment microbial communities: Indeed, highly diverse and abundant nifH gene sequences were obtained from the sediments of the Bohai Sea (Supplementary Figure S4, Table 2), consistent with the results obtained recently from marine sediments of the nSCS (Dang et al., 2013a). Many of the Bohai Sea nifH gene sequences are related to known bacterial diazotrophs (Supplementary Figure S4, Supplementary Table S3). While these results revealed the N2-fixation potential of microbial communities in Bohai Sea sediments, they also suggested microbial diazotrophy as a common ecological property of marginal sea sediments.
Besides being majorly related to environmental nifH gene sequences obtained mainly from the nSCS sediments (Dang et al., 2013a) and occasionally from salt marsh sediments (Lovell et al., 2001; Lovell and Davis, 2012), many of the nifH gene sequences from Bohai Sea sediments are related to diverse known bacterial species and a small number of methanogenic archaea (Supplementary Figure S4). This is consistent with previous findings that diazotrophic bacteria may be the predominant N2-fixing microbes in shallow water sediments (Burns et al., 2002). A recently defined cluster of archaeal nifH gene sequences, called Cluster III-x or Methane Seep Group, was associated with microbes frequently found in deep-sea methane seep, gas hydrate, and mud volcano sediments of the Pacific Ocean marginal seas (Pernthaler et al., 2008; Dang et al., 2009a, 2013a; Dekas et al., 2009, 2014, 2016; Miyazaki et al., 2009). However, Cluster III-x nifH gene sequences were not detected in samples obtained from Bohai Sea sediments. Because Cluster III-x nifH gene sequences are affiliated with anaerobic methane-oxidizing archaea (ANME), they may occur only in methane-rich environments. None of our Bohai Sea sampling stations were located in methane seeps, gas hydrates or mud volcanoes. The lack of the chemoautotrophic ANME nifH gene sequences in samples from shallow coastal sediments is consistent with the in situ geochemical condition of the Bohai Sea.
More than half of the deduced NifH sequences associated with Bohai Sea sediment samples shared high (>90%) identity with NifH sequences from known bacteria, of which the majority are SRB. This result indicates that SRB may be the dominant and prevalent N2-fixing microbes in the sediments of the Bohai Sea. Many SRB harbor functional N2 fixation genetic inventories (Barton and Fauque, 2009) and SRB were previously found to be important N2-fixing bacteria in marine intertidal microbial mats (Zehr et al., 1995; Steppe and Paerl, 2002, 2005; Stal et al., 2010). It was recently reported that the benthic microbial N2 fixation rate is coupled to sulfate reduction activities in lagoons and coastal bays supporting the notion that SRB may play a key role in sediment N2 fixation and new nitrogen production (Bertics et al., 2010, 2013). Furthermore, N2 fixation activities of sediment SRB are tolerant of high (e.g., up to 0.8 or 1.2 mM) environmental concentrations (McGlathery et al., 1998; Bertics et al., 2013) suggesting that benthic N2 fixation is robust and widespread in marine sediments. In our current study, sediment sulfide, the product of microbial sulfate reduction, was found to correlate positively with nifH gene abundance in Bohai Sea sediments. This suggests that SRB may contribute substantially to N2 fixation and sulfate reduction in sediments and thus determine the abundance, spatial distribution, structure, and activity of diazotroph communities in marginal sea sediments.
SRB have been found to be the principal contributors to the accumulation and persistence of environmental organic Hg in coastal marine sediments (Sunderland et al., 2004; Schaefer et al., 2011; Parks et al., 2013). It is also known that sulfide produced by SRB facilitates precipitation and thus accumulation and persistence of Hg in anoxic marine sediments (Baeyens et al., 1998). These ecophysiological properties of SRB may explain the correlation between nifH gene abundance and Hg content in the studied Bohai Sea sediments. Hg is one of the key environmental contaminants in many estuaries and coastal bays of the Bohai Sea (Wang et al., 2010; Gao et al., 2014), where eutrophication is common, especially in summer times (Wang et al., 2009). Our data suggest that the accumulation and persistence of Hg in the sediments of the Bohai Sea may be attributable, at least partially, to the activity of nifH-harboring SRB.
The in situ bottom water temperature was putatively identified as a key environmental factor controlling the community structure and spatial distribution of the sediment putative diazotrophs in the Bohai Sea (Supplementary Figure S5). This finding agrees with the previously reported relationship of sediment in situ temperature with the community structure and spatial distribution of nifH-harboring microbial assemblages in the nSCS sediments (Dang et al., 2013a). Our current study supports the importance of temperature as a key environmental factor controlling universally the distribution of the diazotroph communities in marine water columns and sediments; also because temperature has previously been implicated as the most important environmental factor in the spatial distribution of seawater N2-fixing cyanobacteria in the oceans (Stal, 2009). Temperature influences not only nitrogenase activity but also O2 solubility in seawater and sediment porewater. Enhanced respiration is usually employed by diazotrophs under elevated O2 concentrations (such as under low temperature conditions) for providing energy to replace O2-damaged nitrogenase by de novo synthesis and/or for O2 consumption to maintain anaerobic N2-fixing activity (Stal, 2009; Großkopf and Laroche, 2012; Bandyopadhyay et al., 2013; Brauer et al., 2013). These biochemical and physiological mechanisms explain well the importance of environmental temperature on the community structure, distribution, and activity of marine diazotrophic microbiota.
The bottom water chlorophyll a content (or the covarying bottom water turbidity) was putatively identified as another key environmental factor controlling the community structure and spatial distribution of the putative diazotrophs in Bohai Sea sediments (Supplementary Figure S5). Chlorophyll a is related to the biomass and primary production of phytoplankton and both chlorophyll a and turbidity may indicate the potential of organic matter export from water column to marine sediments (Sobczak et al., 2002; Volkman and Tanoue, 2002). The positive correlation between nifH gene abundance with -Si content in Bohai Sea sediments suggests that diatom productivity affects size and activity of the diazotroph community in sediments (Yool and Tyrrell, 2003; Wei et al., 2004). The phylogenetic analysis of deduced NifH sequences (Supplementary Figure S4) suggests that most of the nifH-harboring microbes in Bohai Sea sediments are heterotrophic bacteria. Respiration of bioavailable organic carbon is the major process for heterotrophic diazotrophs to acquire the metabolic energy to fix N2 (Riemann et al., 2010; Moisander et al., 2014), which is highly energy demanding (Shanmugam et al., 1978; Brill, 1980). Therefore, organic carbon supplies, especially those from metabolizable phytoplankton and benthic diatom products, may constitute the major energy sources to fuel the obligately or facultatively anaerobic diazotrophic microbiota in sediments of coastal seas. In addition, obligately anaerobic bacteria such as SRB and, in particular, the process of N2 fixation, are highly sensitive to oxygen stress (Dixon and Kahn, 2004; Riemann et al., 2010; Zhou et al., 2011). Benthic respiration on organic matter consumes oxygen, which may thus help maintaining the hypoxic to anoxic state of the sediment environment and facilitate N2 fixation by anaerobic diazotrophs. In line with this, sediment porewater Eh, an indicator of the environmental redox status, was found to correlate significantly with the community structure and biogeographical distribution of nifH-harboring microbes in Bohai Sea sediments (Supplementary Figure S5). To maintain the hypoxic to anoxic condition may also be necessary for N2 supply in marine sediments: for instance, the heterotrophic N2 fixation process was linked directly to the N2 production processes by anammox and denitrifying bacteria in marine OMZ waters off the Peru coast (Loescher et al., 2014). A similar coupling of N2 fixation and N2 production microbial processes may also exist in marine sediments. Indeed, the Bohai Sea sediments harbor diverse anammox bacteria including a new species, Candidatus Scalindua pacifica (Dang et al., 2013b). Therefore, the in situ physical and geochemical condition, rather than the geographical location (Figures 3, 4, Supplementary Figure S5), may play a key role in determining the abundance, community structure, niche availability, biogeographical distribution, and activity of the diazotrophic microbiota in the sediment environments of the Bohai Sea.
In order to examine the general biogeographical and ecological characteristics of diazotrophic communities in sediments of the western Pacific Ocean, we performed a comparative analysis of nifH-harboring microbial communities in Bohai Sea and nSCS sediments (Dang et al., 2013a). The results of community classification analyses indicate that the estuarine and nearshore benthic environments harbor distinctly different putative diazotrophic assemblages from those of the offshore and deep-sea benthic environments (Figure 5). Furthermore, the consistency of the unweighted (only taking into account the NifH OTU composition for the analysis; Figure 5A) and weighted (taking into account both the composition of the NifH OTUs and their relative abundances for the analysis; Figure 5B) community classification results suggests that the difference in composition (Figure 5A) may be the key contributor to the difference between sediment diazotroph communities in the western Pacific Ocean. Although the A3 and E501 sampling stations in the nSCS are very distant and segregated from the Bohai Sea, the sediment diazotrophic assemblages of these two stations are separated from the remaining nSCS stations and clustered with the sampling stations of the Bohai Sea (Figure 5), indicating that the in situ physical and geochemical condition may play a more important role than geographical contiguity in determining the community similarity of diazotrophic microbiota in sediments. In line with this, water depth, sediment porewater DO and in situ temperature were identified as the key environmental factors tentatively controlling the composition, community structure and biogeographical distribution of the N2-fixing microbiota in the western Pacific Ocean sediments (Figure 6). The identification of sediment porewater DO as a controlling environmental factor further verifies the importance of the anaerobic heterotrophs in N2 fixation in marginal sea sediments, while the importance of water depth and in situ temperature suggests niche specialization and segregation between the shallow-water (estuarine and nearshore) and the deep-water (offshore and deep-sea) diazotrophic sediment communities. Our current investigation tentatively identified important general ecological, biogeochemical, and biogeographical characteristics and key environmental factors that influence the sediment diazotrophic microbiota and their potential activity in marginal seas.
HD conceived and designed the experiments; HZ performed the experiments and analyzed the data; HD, HZ, and MK wrote the paper.
The authors declare that the research was conducted in the absence of any commercial or financial relationships that could be construed as a potential conflict of interest.
The authors thank Prof. Zhinan Zhang from the Ocean University of China for providing the sediment samples and certain environmental parameter measurements, the crew and scientists onboard R/V “Dong Fang Hong 2” for assisting with sample collection and sharing in situ environmental parameter measurements, and Chao Cheng, Guo Chen, Lei Yang, Qiang Li, and Zhiguo Chen for their assistance in the project. This work was supported by the SOA grant GASI-03-01-02-05, China MOST 973 program grant 2013CB955700, China National Key Research and Development Plan grant 2016YFA0601303, NSFC grants 91328209, 91428308, 41076091, and 91028011, CNOOC grants CNOOC-KJ125FZDXM00TJ001-2014 and CNOOC-KJ125FZDXM00ZJ001-2014, and US-NSF grant MCB-1202648.
The Supplementary Material for this article can be found online at: http://journal.frontiersin.org/article/10.3389/fmicb.2016.01111
Altschul, S. F., Madden, T. L., Schäffer, A. A., Zhang, J., Zhang, Z., Miller, W., et al. (1997). Gapped BLAST and PSI-BLAST: a new generation of protein database search programs. Nucleic Acids Res. 25, 3389–3402. doi: 10.1093/nar/25.17.3389
Baeyens, W., Meuleman, C., Muhaya, B., and Leermakers, M. (1998). Behaviour and speciation of mercury in the Scheldt estuary (water, sediments and benthic organisms). Hydrobiologia 366, 63–79. doi: 10.1023/A:1003124310848
Bandyopadhyay, A., Elvitigala, T., Liberton, M., and Pakrasi, H. B. (2013). Variations in the rhythms of respiration and nitrogen fixation in members of the unicellular diazotrophic cyanobacterial genus Cyanothece. Plant Physiol. 161, 1334–1346. doi: 10.1104/pp.112.208231
Barton, L. L., and Fauque, G. D. (2009). Biochemistry, physiology and biotechnology of sulfate-reducing bacteria. Adv. Appl. Microbiol. 68, 41–98. doi: 10.1016/S0065-2164(09)01202-7
Bergman, B., Sandh, G., Lin, S., Larsson, J., and Carpenter, E. J. (2013). Trichodesmium - a widespread marine cyanobacterium with unusual nitrogen fixation properties. FEMS Microbiol. Rev. 37, 286–302. doi: 10.1111/j.1574-6976.2012.00352.x
Bertics, V. J., Löscher, C. R., Salonen, I., Dale, A. W., Gier, J., Schmitz, R. A., et al. (2013). Occurrence of benthic microbial nitrogen fixation coupled to sulfate reduction in the seasonally hypoxic Eckernförde Bay, Baltic Sea. Biogeosciences 10, 1243–1258. doi: 10.5194/bg-10-1243-2013
Bertics, V. J., Sohm, J. A., Treude, T., Chow, C. T., Capone, D. G., Fuhrman, J. A., et al. (2010). Burrowing deeper into benthic nitrogen cycling: the impact of bioturbation on nitrogen fixation coupled to sulfate reduction. Mar. Ecol. Prog. Ser. 409, 1–15. doi: 10.3354/meps08639
Bird, C., and Wyman, M. (2013). Transcriptionally active heterotrophic diazotrophs are widespread in the upper water column of the Arabian Sea. FEMS Microbiol. Ecol. 84, 189–200. doi: 10.1111/1574-6941.12049
Boesch, D. F. (2002). Challenges and opportunities for science in reducing nutrient over-enrichment of coastal ecosystems. Estuaries 25, 886–900. doi: 10.1007/BF02804914
Bonnet, S., Dekaezemacker, J., Turk-Kubo, K. A., Moutin, T., Hamersley, R. M., Grosso, O., et al. (2013). Aphotic N2 fixation in the eastern tropical south Pacific Ocean. PLoS ONE 8:e81265. doi: 10.1371/journal.pone.0081265
Boyd, P. W., and Ellwood, M. J. (2010). The biogeochemical cycle of iron in the ocean. Nat. Geosci. 3, 675–682. doi: 10.1038/ngeo964
Brauer, V. S., Stomp, M., Rosso, C., van Beusekom, S. A., Emmerich, B., Stal, L. J., et al. (2013). Low temperature delays timing and enhances the cost of nitrogen fixation in the unicellular cyanobacterium Cyanothece. ISME J. 7, 2105–2115. doi: 10.1038/ismej.2013.103
Burns, J. A., Zehr, J. P., and Capone, D. G. (2002). Nitrogen-fixing phylotypes of Chesapeake Bay and Neuse River estuary sediments. Microb. Ecol. 44, 336–343. doi: 10.1007/s00248-002-1000-9
Dang, H., and Jiao, N. (2014). Perspectives on the microbial carbon pump with special reference to microbial respiration and ecosystem efficiency in large estuarine systems. Biogeosciences 11, 3887–3898. doi: 10.5194/bg-11-3887-2014
Dang, H., and Lovell, C. R. (2000). Bacterial primary colonization and early succession on surfaces in marine waters as determined by amplified rRNA gene restriction analysis and sequence analysis of 16S rRNA genes. Appl. Environ. Microbiol. 66, 467–475. doi: 10.1128/AEM.66.2.467-475.2000
Dang, H., and Lovell, C. R. (2016). Microbial surface colonization and biofilm development in marine environments. Microbiol. Mol. Biol. Rev. 80, 91–138. doi: 10.1128/MMBR.00037-15
Dang, H. Y., Li, T. G., Chen, M. N., and Huang, G. Q. (2008). Cross-ocean distribution of Rhodobacterales bacteria as primary surface colonizers in temperate coastal marine waters. Appl. Environ. Microbiol. 74, 52–60. doi: 10.1128/AEM.01400-07
Dang, H. Y., Luan, X. W., Zhao, J. Y., and Li, J. (2009a). Diverse and novel nifH and nifH-like gene sequences in the deep-sea methane seep sediments of the Okhotsk Sea. Appl. Environ. Microbiol. 75, 2238–2245. doi: 10.1128/AEM.02556-08
Dang, H. Y., Wang, C. Y., Li, J., Li, T. G., Tian, F., Jin, W., et al. (2009b). Diversity and distribution of sediment nirS-encoding bacterial assemblages in response to environmental gradients in the eutrophied Jiaozhou Bay, China. Microb. Ecol. 58, 161–169. doi: 10.1007/s00248-008-9469-5
Dang, H. Y., Yang, J. Y., Li, J., Luan, X. W., Zhang, Y. B., Gu, G. Z., et al. (2013a). Environment-dependent distribution of the sediment nifH-harboring microbiota in the Northern South China Sea. Appl. Environ. Microbiol. 79, 121–132. doi: 10.1128/AEM.01889-12
Dang, H. Y., Zhou, H. X., Zhang, Z. N., Yu, Z. S., Hua, E., Liu, X. S., et al. (2013b). Molecular detection of Candidatus Scalindua pacifica and environmental responses of sediment anammox bacterial community in the Bohai Sea, China. PLoS ONE 8:e61330. doi: 10.1371/journal.pone.0061330
Dekaezemacker, J., Bonnet, S., Grosso, O., Moutin, T., Bressac, M., and Capone, D. G. (2013). Evidence of active dinitrogen fixation in surface waters of the eastern tropical South Pacific during El Niño and La Niña events and evaluation of its potential nutrient controls. Global Biogeochem. Cycles 27, 768–779. doi: 10.1002/gbc.20063
Dekas, A. E., Chadwick, G. L., Bowles, M. W., Joye, S. B., and Orphan, V. J. (2014). Spatial distribution of nitrogen fixation in methane seep sediment and the role of the ANME archaea. Environ. Microbiol. 16, 3012–3029. doi: 10.1111/1462-2920.12247
Dekas, A. E., Connon, S. A., Chadwick, G. L., Trembath-Reichert, E., and Orphan, V. J. (2016). Activity and interactions of methane seep microorganisms assessed by parallel transcription and FISH-NanoSIMS analyses. ISME J. 10, 678–692. doi: 10.1038/ismej.2015.145
Dekas, A. E., Poretsky, R. S., and Orphan, V. J. (2009). Deep-sea archaea fix and share nitrogen in methane-consuming microbial consortia. Science 326, 422–426. doi: 10.1126/science.1178223
Deutsch, C., Sarmiento, J. L., Sigman, D. M., Gruber, N., and Dunne, J. P. (2007). Spatial coupling of nitrogen inputs and losses in the ocean. Nature 445, 163–167. doi: 10.1038/nature05392
Dixon, R., and Kahn, D. (2004). Genetic regulation of biological nitrogen fixation. Nat. Rev. Microbiol. 2, 621–631. doi: 10.1038/nrmicro954
Dore, J. E., Brum, J. R., Tupas, L. M., and Karl, D. M. (2002). Seasonal and interannual variability in sources of nitrogen supporting export in the oligotrophic subtropical North Pacific Ocean. Limnol. Oceanogr. 47, 1595–1607. doi: 10.4319/lo.2002.47.6.1595
Farnelid, H., Andersson, A. F., Bertilsson, S., Al-Soud, W. A., Hansen, L. H., Sørensen, S., et al. (2011). Nitrogenase gene amplicons from global marine surface waters are dominated by genes of non-cyanobacteria. PLoS ONE 6:e19223. doi: 10.1371/journal.pone.0019223
Farnelid, H., Bentzon-Tilia, M., Andersson, A. F., Bertilsson, S., Jost, G., Labrenz, M., et al. (2013). Active nitrogen-fixing heterotrophic bacteria at and below the chemocline of the central Baltic Sea. ISME J. 7, 1413–1423. doi: 10.1038/ismej.2013.26
Fernandez, C., Farías, L., and Ulloa, O. (2011). Nitrogen fixation in denitrified marine waters. PLoS ONE 6:e20539. doi: 10.1371/journal.pone.0020539
Gao, X., Zhou, F., and Chen, C. T. A. (2014). Pollution status of the Bohai Sea: an overview of the environmental quality assessment related trace metals. Environ. Int. 62, 12–30. doi: 10.1016/j.envint.2013.09.019
Großkopf, T., and Laroche, J. (2012). Direct and indirect costs of dinitrogen fixation in Crocosphaera watsonii WH8501 and possible implications for the nitrogen cycle. Front. Microbiol. 3:236. doi: 10.3389/fmicb.2012.00236
Großkopf, T., Mohr, W., Baustian, T., Schunck, H., Gill, D., Kuypers, M. M., et al. (2012). Doubling of marine dinitrogen-fixation rates based on direct measurements. Nature 488, 361–364. doi: 10.1038/nature11338
Hamady, M., Lozupone, C., and Knight, R. (2010). Fast UniFrac: facilitating high-throughput phylogenetic analyses of microbial communities including analysis of pyrosequencing and PhyloChip data. ISME J. 4, 17–27. doi: 10.1038/ismej.2009.97
Herbert, R. A. (1999). Nitrogen cycling in coastal marine ecosystems. FEMS Microbiol. Rev. 23, 563–590. doi: 10.1111/j.1574-6976.1999.tb00414.x
Homoky, W. B., John, S. G., Conway, T. M., and Mills, R. A. (2013). Distinct iron isotopic signatures and supply from marine sediment dissolution. Nat. Commun. 4, 2143. doi: 10.1038/ncomms3143
Jacq, V., Ridame, C., L'Helguen, S., Kaczmar, F., and Saliot, A. (2014). Response of the unicellular diazotrophic cyanobacterium Crocosphaera watsonii to iron limitation. PLoS ONE 9:e92655. doi: 10.1371/journal.pone.0086749
Jayakumar, A., Al-Rshaidat, M. M., Ward, B. B., and Mulholland, M. R. (2012). Diversity, distribution, and expression of diazotroph nifH genes in oxygen-deficient waters of the Arabian Sea. FEMS Microbiol. Ecol. 82, 597–606. doi: 10.1111/j.1574-6941.2012.01430.x
Karl, D., Letelier, R., Tupas, L., Diore, J., Christian, J., and Hemel, D. (1997). The role of nitrogen fixation in biogeochemical cycling in the subtropical North Pacific Ocean. Nature 388, 533–538. doi: 10.1038/41474
Karl, D., Michaels, A., Bergman, B., Capone, D., Carpenter, E., Letelier, R., et al. (2002). Dinitrogen fixation in the world's oceans. Biogeochemistry 57, 47–98. doi: 10.1023/A:1015798105851
Karl, D. M. (2014). Microbially mediated transformations of phosphorus in the sea: new views of an old cycle. Annu. Rev. Mar. Sci. 6, 279–337. doi: 10.1146/annurev-marine-010213-135046
Karl, D. M., and Letelier, R. M. (2008). Nitrogen fixation-enhanced carbon sequestration in low nitrate, low chlorophyll seascapes. Mar. Ecol. Prog. Ser. 364, 257–268. doi: 10.3354/meps07547
Knapp, A. N. (2012). The sensitivity of marine N2 fixation to dissolved inorganic nitrogen. Front. Microbiol. 3:374. doi: 10.3389/fmicb.2012.00374
Larkin, M. A., Blackshields, G., Brown, N. P., Chenna, R., McGettigan, P. A., McWilliam, H., et al. (2007). Clustal W and Clustal X version 2.0. Bioinformatics 23, 2947–2948. doi: 10.1093/bioinformatics/btm404
Lepš, J., and Šmilauer, P. (2003). Multivariate Analysis of Ecological Data Using CANOCO. New York, NY: Cambridge University Press.
Loescher, C. R., Großkopf, T., Desai, F. D., Gill, D., Schunck, H., Croot, P. L., et al. (2014). Facets of diazotrophy in the oxygen minimum zone waters off Peru. ISME J. 8, 2180–2192. doi: 10.1038/ismej.2014.71
Lovell, C. R., and Davis, D. A. (2012). Specificity of salt marsh diazotrophs for vegetation zones and plant hosts: results from a North American marsh. Front. Microbiol. 3:84. doi: 10.3389/fmicb.2012.00084
Lovell, C. R., Friez, M. J., Longshore, J. W., and Bagwell, C. E. (2001). Recovery and phylogenetic analysis of nifH sequences from diazotrophic bacteria associated with dead aboveground biomass of Spartina alterniflora. Appl. Environ. Microbiol. 67, 5308–5314. doi: 10.1128/AEM.67.11.5308-5314.2001
Mahaffey, C., Michaels, A. F., and Capone, D. G. (2005). The conundrum of marine N2 fixation. Am. J. Sci. 305, 546–595. doi: 10.2475/ajs.305.6-8.546
McGlathery, K. J., Risgaard-Petersen, N., and Christensen, P. B. (1998). Temporal and spatial variation in nitrogen fixation activity in the eelgrass Zostera marina rhizosphere. Mar. Ecol. Prog. Ser. 168, 245–258. doi: 10.3354/meps168245
Mehta, M. P., Butterfield, D. A., and Baross, J. A. (2003). Phylogenetic diversity of nitrogenase (nifH) genes in deep-sea and hydrothermal vent environments of the Juan de Fuca Ridge. Appl. Environ. Microbiol. 69, 960–970. doi: 10.1128/AEM.69.2.960-970.2003
Mills, M. M., Ridame, C., Davey, M., La Roche, J., and Geider, R. J. (2004). Iron and phosphorus co-limit nitrogen fixation in the eastern tropical North Atlantic. Nature 429, 292–294. doi: 10.1038/nature02550
Miyazaki, J., Higa, R., Toki, T., Ashi, J., Tsunogai, U., Nunoura, T., et al. (2009). Molecular characterization of potential nitrogen fixation by anaerobic methane-oxidizing archaea in the methane seep sediments at the number 8 Kumano Knoll in the Kumano Basin, offshore of Japan. Appl. Environ. Microbiol. 75, 7153–7162. doi: 10.1128/AEM.01184-09
Moisander, P. H., Serros, T., Paerl, R. W., Beinart, R. A., and Zehr, J. P. (2014). Gammaproteobacterial diazotrophs and nifH gene expression in surface waters of the South Pacific Ocean. ISME J. 8, 1962–1973. doi: 10.1038/ismej.2014.49
Montoya, J. P., Holl, C. M., Zehr, J. P., Hansen, A., Villareal, T. A., and Capone, D. G. (2004). High rates of N2 fixation by unicellular diazotrophs in the oligotrophic Pacific Ocean. Nature 430, 1027–1032. doi: 10.1038/nature02824
Moore, C. M., Mills, M. M., Achterberg, E. P., Geider, R. J., LaRoche, J., Lucas, M. I., et al. (2009). Large-scale distribution of Atlantic nitrogen fixation controlled by iron availability. Nat. Geosci. 2, 867–871. doi: 10.1038/ngeo667
Moore, C. M., Mills, M. M., Arrigo, K. R., Berman-Frank, I., Bopp, L., Boyd, P. W., et al. (2013). Processes and patterns of oceanic nutrient limitation. Nat. Geosci. 6, 701–710. doi: 10.1038/ngeo1765
Mullins, T. D., Britschgi, T. B., Krest, R. L., and Giovannoni, S. J. (1995). Genetic comparisons reveal the same unknown bacterial lineages in Atlantic and Pacific bacterioplankton communities. Limnol. Oceanogr. 40, 148–158. doi: 10.4319/lo.1995.40.1.0148
PEMSEA and BSEMP (2005). Bohai Sea Environmental Risk Assessment. PEMSEA Technical Report No. 12. 114. Partnerships in Environmental Management for the Seas of East Asia, Bohai Sea Environmental Management Project of the People's Republic of China.
Paerl, H. W. (1990). Physiological ecology and regulation of N2 fixation in natural waters. Adv. Microb. Ecol. 11, 305–344. doi: 10.1007/978-1-4684-7612-5_8
Parks, J. M., Johs, A., Podar, M., Bridou, R., Hurt, R. A. Jr., Smith, S. D., et al. (2013). The genetic basis for bacterial mercury methylation. Science 339, 1332–1335. doi: 10.1126/science.1230667
Pernthaler, A., Dekas, A. E., Brown, C. T., Goffredi, S. K., Embaye, T., and Orphan, V. J. (2008). Diverse syntrophic partnerships from deep-sea methane vents revealed by direct cell capture and metagenomics. Proc. Natl. Acad. Sci. U.S.A. 105, 7052–7057. doi: 10.1073/pnas.0711303105
Rahav, E., Bar-Zeev, E., Ohayon, S., Elifantz, H., Belkin, N., Herut, B., et al. (2013). Dinitrogen fixation in aphotic oxygenated marine environments. Front. Microbiol. 4:227. doi: 10.3389/fmicb.2013.00227
Riemann, L., Farnelid, H., and Steward, G. F. (2010). Nitrogenase genes in non- cyanobacterial plankton: prevalence, diversity and regulation in marine waters. Aquat. Microb. Ecol. 61, 235–247. doi: 10.3354/ame01431
Sañudo-Wilhelmy, S. A., Kustka, A. B., Gobler, C. J., Hutchins, D. A., Yang, M., Lwiza, K., et al. (2001). Phosphorus limitation of nitrogen fixation by Trichodesmium in the central Atlantic Ocean. Nature 411, 66–69. doi: 10.1038/35075041
Schaefer, J. K., Rocks, S. S., Zheng, W., Liang, L., Gu, B., and Morel, F. M. M. (2011). Active transport, substrate specificity, and methylation of Hg(II) in anaerobic bacteria. Proc. Natl. Acad. Sci. U.S.A. 108, 8714–8719. doi: 10.1073/pnas.1105781108
Schloss, P. D., and Handelsman, J. (2005). Introducing DOTUR, a computer program for defining operational taxonomic units and estimating species richness. Appl. Environ. Microbiol. 71, 1501–1506. doi: 10.1128/AEM.71.3.1501-1506.2005
Shanmugam, K. T., O'Gara, F., Andersen, K., and Valentine, R. C. (1978). Biological nitrogen fixation. Annu. Rev. Plant Physiol. 29, 263–276. doi: 10.1146/annurev.pp.29.060178.001403
Shao, S. D., Luan, X. W., Dang, H. Y., Zhou, H. X., Zhao, Y. K., Liu, H. T., et al. (2014). Deep-sea methane seep sediments in the Okhotsk Sea sustain diverse and abundant anammox bacteria. FEMS Microbiol. Ecol. 87, 503–516. doi: 10.1111/1574-6941.12241
Smith, V. H. (2003). Eutrophication of freshwater and coastal marine ecosystems: a global problem. Environ. Sci. Pollut. Res. Int. 10, 126–139. doi: 10.1065/espr2002.12.142
Sobczak, W. V., Cloern, J. E., Jassby, A. D., and Müller-Solger, A. B. (2002). Bioavailability of organic matter in a highly disturbed estuary: the role of detrital and algal resources. Proc. Natl. Acad. Sci. U.S.A. 99, 8101–8105. doi: 10.1073/pnas.122614399
Sohm, J. A., Webb, E. A., and Capone, D. G. (2011). Emerging patterns of marine nitrogen fixation. Nat. Rev. Microbiol. 9, 499–508. doi: 10.1038/nrmicro2594
Stal, L. J. (2009). Is the distribution of nitrogen-fixing cyanobacteria in the oceans related to temperature? Environ. Microbiol. 11, 1632–1645. doi: 10.1111/j.1758-2229.2009.00016.x
Stal, L. J., Severin, I., and Bolhuis, H. (2010). The ecology of nitrogen fixation in cyanobacterial mats. Adv. Exp. Med. Biol. 675, 31–45. doi: 10.1007/978-1-4419-1528-3_3
Steppe, T. F., and Paerl, H. W. (2002). Potential N2 fixation by sulfate-reducing bacteria in a marine intertidal microbial mat. Aquat. Microb. Ecol. 28, 1–12. doi: 10.3354/ame028001
Steppe, T. F., and Paerl, H. W. (2005). Nitrogenase activity and nifH expression in a marine intertidal microbial mat. Microb. Ecol. 49, 315–324. doi: 10.1007/s00248-004-0245-x
Street, J. H., and Paytan, A. (2005). Iron, phytoplankton growth, and the carbon cycle. Met. Ions. Biol. Syst. 43, 153–193. doi: 10.1201/9780824751999.ch7
Sunderland, E. M., Gobas, F. A. P. C., Heyes, A., Branfireun, B. A., Bayer, A. K., Cranston, R. E., et al. (2004). Speciation and bioavailability of mercury in well-mixed estuarine sediments. Mar. Chem. 90, 91–105. doi: 10.1016/j.marchem.2004.02.021
ter Braak, C. J. F., and Šmilauer, P. (2002). CANOCO Reference Manual and CanoDraw for Windows User's Guide: Software for Canonical Community Ordination (Version 4.5). Ithaca, NY: Microcomputer Power.
Trimmer, M., and Nicholls, J. C. (2009). Production of nitrogen gas via anammox and denitrification in intact sediment cores along a continental shelf to slope transect in the North Atlantic. Limnol. Oceanogr. 54, 577–589. doi: 10.4319/lo.2009.54.2.0577
Volkman, J. K., and Tanoue, E. (2002). Chemical and biological studies of particulate organic matter in the ocean. J. Oceanogr. 58, 265–279. doi: 10.1023/A:1015809708632
Voss, M., Bange, H. W., Dippner, J. W., Middelburg, J. J., Montoya, J. P., and Ward, B. (2013). The marine nitrogen cycle: recent discoveries, uncertainties and the potential relevance of climate change. Philos. Trans. R. Soc. B. 368:20130121. doi: 10.1098/rstb.2013.0121
Wang, J., Chen, S., and Xia, T. (2010). Environmental risk assessment of heavy metals in Bohai Sea, North China. Procedia Environ. Sci. 2, 1632–1642. doi: 10.1016/j.proenv.2010.10.174
Wang, X. L., Cui, Z. G., Guo, Q., Han, X. R., and Wang, J. T. (2009). Distribution of nutrients and eutrophication assessment in the Bohai Sea of China. Chin. J. Oceanol. Limnol. 27, 177–183. doi: 10.1007/s00343-009-0177-x
Wei, H., Sun, J., Moll, A., and Zhao, L. (2004). Phytoplankton dynamics in the Bohai Sea-observations and modelling. J. Mar. Syst. 44, 233–251. doi: 10.1016/j.jmarsys.2003.09.012
Yool, A., and Tyrrell, T. (2003). Role of diatoms in regulating the ocean's silicon cycle. Global Biogeochem. Cycles 17, 1103–1121. doi: 10.1029/2002GB002018
Zehr, J. P. (2011). Nitrogen fixation by marine cyanobacteria. Trends Microbiol. 19, 162–173. doi: 10.1016/j.tim.2010.12.004
Zehr, J. P., Bench, S. R., Carter, B. J., Hewson, I., Niazi, F., Shi, T., et al. (2008). Globally distributed uncultivated oceanic N2-fixing cyanobacteria lack oxygenic photosystem II. Science 322, 1110–1112. doi: 10.1126/science.1165340
Zehr, J. P., and Kudela, R. M. (2011). Nitrogen cycle of the open ocean: from genes to ecosystems. Annu. Rev. Mar. Sci. 3, 197–225. doi: 10.1146/annurev-marine-120709-142819
Zehr, J. P., Mellon, M., Braun, S., and Litaker, W. (1995). Diversity of heterotrophic nitrogen fixation genes in a marine cyanobacterial mat. Appl. Environ. Microbiol. 61, 2527–2532.
Zehr, J. P., Waterbury, J. B., Turner, P. J., Montoya, J. P., Omoregie, E., Steward, G. F., et al. (2001). Unicellular cyanobacteria fix N2 in the subtropical Pacific Ocean. Nature 412, 635–638. doi: 10.1038/news010809-11
Keywords: coastal sediments, ecophysiological specialization, estuary, marginal sea, niche differentiation, nifH, nitrogen fixation, sulfate-reducing bacteria
Citation: Zhou H, Dang H and Klotz MG (2016) Environmental Conditions Outweigh Geographical Contiguity in Determining the Similarity of nifH-Harboring Microbial Communities in Sediments of Two Disconnected Marginal Seas. Front. Microbiol. 7:1111. doi: 10.3389/fmicb.2016.01111
Received: 09 March 2016; Accepted: 04 July 2016;
Published: 20 July 2016.
Edited by:
Dennis A. Bazylinski, University of Nevada at Las Vegas, USAReviewed by:
Zhe-Xue Quan, Fudan University, ChinaCopyright © 2016 Zhou, Dang and Klotz. This is an open-access article distributed under the terms of the Creative Commons Attribution License (CC BY). The use, distribution or reproduction in other forums is permitted, provided the original author(s) or licensor are credited and that the original publication in this journal is cited, in accordance with accepted academic practice. No use, distribution or reproduction is permitted which does not comply with these terms.
*Correspondence: Hongyue Dang, ZGFuZ2h5QHhtdS5lZHUuY24=
Disclaimer: All claims expressed in this article are solely those of the authors and do not necessarily represent those of their affiliated organizations, or those of the publisher, the editors and the reviewers. Any product that may be evaluated in this article or claim that may be made by its manufacturer is not guaranteed or endorsed by the publisher.
Research integrity at Frontiers
Learn more about the work of our research integrity team to safeguard the quality of each article we publish.