- 1College of Environmental Science and Engineering, Qingdao University, Qingdao, China
- 2Shandong Provincial Key Laboratory of Energy Genetics, Key Laboratory of Biofuel, Qingdao Institute of BioEnergy and Bioprocess Technology, Chinese Academy of Sciences, Qingdao, China
- 3Exploration & Production Research Institute, China Petroleum & Chemical Corporation, Beijing, China
Recalcitrance of biomass feedstock remains a challenge for microbial conversion of lignocellulose into biofuel and biochemicals. Clostridium cellulosi, one thermophilic bacterial strain dominated in compost, could hydrolyze lignocellulose at elevated temperature by secreting more than 38 glycoside hydrolases belong to 15 different families. Though one multi-modular endoglucanase CcCel9A has been identified from C. cellulosi CS-4-4, mechanism of synergistic degradation of cellulose by various cellulases from strain CS-4-4 remains elusive. In this study, CcCel9A, CcCel9B, and CcCel48A were characterized as processive endoglucanase, non-processive endoglucanase, and exoglucanase, respectively. To understand how they cooperate with each other, we estimated the approximate concentration ratio on the zymogram and optimized it using purified enzymes in vitro. Synergism between individual glycoside hydrolase during cellulose hydrolysis in the mixture was observed. CcCel9A and CcCel48A could degrade cellulose chain from non-reducing ends and reducing ends, respectively, to cello-oligosaccharide. CcCel9B could cut cellulose chain randomly and cello-oligosaccharides with varied length were released. In addition, a β-glucosidase BlgA from Caldicellulosiruptor sp. F32 which could cleave cello-oligosaccharides including G2-G6 to glucose was added to the enzyme mixture to remove the product inhibition of its partners. The combination and ratios of these cellulases were optimized based on the release rate of glucose. Hydrolysis of corn stalk was conducted by a four-component cocktail (CcCel9A:CcCel9B:CcCel48A:BlgA = 25:25:10:18), and only glucose was detected as main production by using high-performance anion-exchange chromatography. Processive endoglucanase CcCel9A, dominated in secretome of C. cellulosi, showed good potential in developing cellulase cocktail due to its exquisite cooperation with various cellulases.
Introduction
Lignocellulose is the most abundant renewable biomass for the production of biofuels and biochemicals. The bioconversion of lignocellulose to fuel and chemical is carried out via three processes: pretreatment, enzymatic hydrolysis, and fermentation (Lynd et al., 2005). Due to the recalcitrance and complexity of lignocellulose, the cost of enzymatic hydrolysis is very high, which is the bottleneck of this industry. Therefore, much effort has been made to improve the enzymatic hydrolysis efficiency, mainly focusing on reducing the enzyme amount and hydrolysis time. Development of cellulose cocktails became a mainstream strategy to enhance the process. The components of the cocktails could cooperate with each other in modifying the structure of crystalline cellulose, thus making cellulose more accessible for cellulases (Jeoh et al., 2006; Arantes and Saddler, 2010). Therefore, mining cellulases candidates which can synergize in nature was imperative.
Clostridium cellulosi is an obligately anaerobic, thermophilic Gram-positive bacterium (He et al., 1991). Although it appears not to produce cellulosome, C. cellulosi utilizes a broad range of carbon sources such as fructose, glycogen, inulin, mannitol, starch, trehalose, and xylose for generation of hydrogen, carbon dioxide, ethanol and acetic acid, which was more than C. thermocellum does. In the genomic sequence of C. cellulosi CS-4-4 a gene cluster containing five open reading frames including Ccel_3879 encoding an exoglucanase, Ccel_3880 encoding an endoglucanase, Ccel_3881 encoding a putative glycoside hydrolase, Ccel_3882 encoding a xylanase, and Ccel_3883 encoding another putative glycoside hydrolase was identified. LC-MS analysis of the bands on zymogram of spent culture from C. cellulosi CS-4-4 indicated that Ccel_3880 and Ccel_3883 played the key roles in hydrolyzing xylan and CMC (carboxymethylcellulose). Ccel_3879, as the only band appearing on the exoglucanase zymogram, was the most abundant exoglucanase in the secretome of C. cellulosi CS-4-4. Conserved domain analysis of the three ORFs showed that both Ccel_3880 and Ccel_3883 were family 9 glycoside hydrolases (CcCel9A and CcCel9B), while Ccel_3879 belonged to family 48 glycoside hydrolase (CcCel48A). Additionally, CcCel9A possessed one GH module and five CBMs (carbohydrate-binding module), which is scarce in Prokaryotes (see ESM_2.pdf in Supplementary Material) (Zhang et al., 2014).
Cellulose systems exhibit higher activity than the sum of the activities of individual enzymes, which is known as synergism. Four types of synergism have been proposed: (i) endo-exo synergy between endoglucanases and exoglucanases, (ii) exo-exo synergy between reducing-end exoglucanases and non-reducing-end exoglucanases, (iii) synergy between exoglucanases, and β-glucosidases that remove cellobiose and cellodextrins as repressive end-products, and (iv) intramolecular synergy between catalytic modules and CBMs (Din et al., 1994; Teeri, 1997). The synergism between GH family 9 processive endoglucanase and GH family 48 exoglucanase has been proved in C. phytofermentans (Zhang et al., 2010) and Thermobifida fusca (Kostylev and Wilson, 2014). In T. fusca a model of synergistic cooperation between Tf Cel9A and Tf Cel48A has been proposed. Tf Cel9A provides accessible substrate for Tf Cel48A. Tf Cel48A preferentially hydrolyzes looser chains, replenishing the smooth surface for Tf Cel9A. Tf Cel9A is the first identified processive endoglucanase, which can cleave cellotetrose from the non-reducing end of a cellulose chain processively and hydrolyze cellotetrose to glucose, cellobiose, and cellotriose sequentially (Sakon et al., 1997). Thereafter, several processive endoglucanases, which possess both endo- and exoglucanases activities as Tf Cel9A, have been discovered and characterized (Riedel and Bronnenmeier, 1999; Mandelman et al., 2003; Zverlov et al., 2003, 2005; Berger et al., 2007; Zhang et al., 2010; Jeon et al., 2012). However, how the processive endoglucanase, non-processive endoglucanase, and exoglucanase cooperate with each other in the hydrolyzing process remains unknown.
In this study, we detected the cellulose hydrolyzing activities of a series of the mixture enzymes with various ratios to evaluate the cooperation between CcCel9A, CcCel9B, and CcCel48A. Furthermore, we added a β-glucosidase BlgA from Caldicellulosiruptor sp. F32 to the mixtures (Meng et al., 2015) to improve the effect of cellulose saccharification by hydrolyzing all cello-oligosaccharides to glucose, which will be beneficial for utilization by engineering strains in industrial production.
Materials and Methods
Strains and Chemicals
The Escherichia coli strains Trans1-T1 and BL21(DE3) used in plasmids construction were purchased from TransGen Biotech (Beijing, China). CMC was from Sigma-Aldrich (St. Louis, USA) and Whatman no. 1 filter paper was from GE Healthcare (Stockholm, Sweden). Cello-oligosaccharides including cellobiose (G2), cellotriose (G3), cellotetraose (G4), cellopentaose (G5), and cellohexose (G6) were all from Megazyme (Wicklow, Ireland). All the reagents were of analytical grade.
Plasmid Construction
The gene of CcCel48A was amplified from the genomic DNA of C. cellulosi CS-4-4 (GenBank accession Number: KF434246) using a forward primer (5′-GCGGATGATGGGACCTAC AAGGCGAAG-3′) and a reverse primer (5′- CGGTTCAACTCC CCAAATTAGCTCGTCGC-3′). The polymerase chain reaction (PCR) products were cloned into pEASY-E2 vector and transformed into E. coli Trans1-T1. After overnight cultivation, several clones were selected for verification by colony PCR and the DNA insert was sequenced by Sunny Bio-technology Co. Ltd. (Shanghai, China) to confirm the integrity of the gene. Then the expression vector for CcCel48A was extracted and transformed into E. coli BL21(DE3). Afterwards, a single positive clone was selected and cultivated to make competent cells, which was as a host to accept the chaperone plasmid pGro7 (Takara, Tokyo, Japan). The expression vector for CcCel9A, CcCel9B, and BlgA had been constructed in our previous study (Zhang et al., 2014; Meng et al., 2015).
Enzyme Expression and Purification
All genes were over-expressed in E. coli BL21(DE3) and the recombinant strains were incubated at 19°C overnight at 200 rpm. Except for that the expression of cel48a needed additional 4 mg ml−1 L-arabinosel, 500 mmol l−1 isopropyl β-D-1-thiogalactopyranoside (IPTG) was used to induce the cells at OD600 of 0.6. Cells were collected by centrifugation (7000 × g for 20 min at 4°C) after induction. The cell pellets were resuspended in lysis buffer (50 mmol l−1 NaH2PO4, 300 mmol l−1 NaCl, pH 8.0) and disrupted by ultrasonication after adding DNase and protease inhibitor. The target protein was purified by affinity chromatography using Ni-nitrilotriacetic acid (NTA) His-Bind resin according to the supplier's protocol (Novagen, Darmstadt, Germany). The purity was analyzed by SDS-PAGE and the purified protein was ultracentrifuged and resuspended in 50 mmol l−1 HAc-NaAc buffer (pH 5.0). Protein concentration was determined by using the Pierce BCA Protein Assay Kit (Thermo Fisher Scientific, Rockford, USA).
Enzyme Activity and Processivity Assay
For enzyme activity assay, 1 μmol l−1 of the purified enzymes were incubated with 20 mg ml−1 CMC for 30 min and 5 mg ml−1 filter paper for 4 h, respectively. All samples were prepared in 50 mmol l−1 HAc-NaAc buffer (pH 5.0) and the concentrations of soluble reducing sugars were measured using 3,5-dinitrosalicyclic acid method (Gusakov et al., 2011). The processivity was determined according to a motified protocol of Inci et al (Ozdemir et al., 2012). Single discs of filter paper with a diameter of 10 mm and a total weight of 3 mg were incubated with 9 μM purified enzymes at 60°C in 50 mmol l−1 HAc-NaAc buffer (pH 5.0) for 16 h. After incubation, the supernatant (150 μl) containing soluble reducing sugar was removed and the residual filter papers were washed with the same buffer for three times. After that, 150 μl of 50 mmol l−1 HAc-NaAc buffer was added to the filter paper. DNS reagent (200 μl) was added to the supernatant and the filter paper tubes for the measurement of the reducing sugars.
Product Pattern of the Purified Enzyme
The enzymatic hydrolysis product of soluble cello-oligosaccharide was analyzed by thin-layer chromatography (TLC). The sample was separated on a 60 F254 silica gel plate (Merck, Darmstadt, Germany) with a developing solution of butanol/acetate/H2O (2:1:1, v/v) and the sugar was detected by charring with an acid/alcohol solution of ethanol/sulfuric acid (4:1, v/v).
Calculation of Gray Scale Value (GSV)
To realize the approximate concentration ratios of Ccel_3880:Ccel_3883 (CcCel9A:CcCel9B), the gray scale values of their bands on the endoglucanase-zymogram were calculated using ImageJ 1.42q (http://rsbweb.nih.gov/ij/) as described in Zhang et al. (2015). According to the LC-MS identification results, each pixel region of the bands 14 and 15 were extracted and transformed to GSV and the total value was noted as GSV of CcCel9A. In the same way, the GSV of CcCel9B was obtained by analyzing the bands 17 and 18, finally calculating GSVCel9A/GSVCel9B as the ratio.
Assessment of Synergy and Product Analysis
Enzyme mixtures including CcCel9A, CcCel9B, CcCel48A, and BlgA with a total concentration of 1 μmol l−1 were incubated with CMC or filter paper in 50 mmol l−1 HAc-NaAc buffer (pH 5.0) and the concentrations of soluble reducing sugars were measured as described above. The optimal ratio of each cocktail was determined according to the release of reducing sugars. All experiments were carried out in triplicate. In order to assess the synergy of each cocktail, the following formula to calculate the degree of synergy (DOS) was used: DOS = (total sugars released from enzyme mixture)/(total sugars released from incubation with individual enzymes) (Iakiviak et al., 2011).
The enzyme mixtures were incubated at 60°C with 5 mg ml−1 filter paper or 0.5 mg ml−1 of steam-exploded corn stalk and samples were withdrawn for further analysis at intervals. The products of the hydrolysate were analyzed by high-performance anion-exchange chromatography with pulsed amperometric detection (HPAEC-PAD) using Dionex ICS-3000 (Sunnyvate, USA).
Statistical Analysis
Kruskal Wallis H test was applied to ascertain significant differences using predictive analytic software (PASW 18.0). The level of statistical significance was P < 0.05.
Results and Discussion
The molecular weights of CcCel9A, CcCel9B, CcCel48A, and BlgA were 115.8 kDa, 79.4 kDa, 101.5 kDa, and 54 kDa, respectively (ESM_1.pdf and ESM_2.pdf in Supplementary Material). Basic enzymatic characters (Table 1) showed that CcCel9A had both considerable endoglucanase and exoglucanase activities, with a remarkable processivity value. CcCel9B also performed efficiently on hydrolyzing soluble substrate, which showed as one of the major bands on the endoglucanase-zymogram. Although the specific activity of CcCel48A on filter paper was poor, it was the only active band identified on the exoglucanase-zymogram, suggesting that CcCel48A was the most significant exoglucanase in C. cellulosi CS-4-4 (Zhang et al., 2014). Hydrolysis of cello-oligosaccarides by these cellulases from C. cellulosi was determined by using TLC (Figure 1). The smallest substrates for CcCel9A and CcCel9B were both cellotetraose (G4). And they could hydrolyze cellopentaose (G5) and cellohexose (G6) to glucose (G1), cellobiose (G2), and cellotriose (G3) (Figures 1A,B). As shown in Figure 1D, G4 was released by the enzymatic hydrolysis of CcCel9A initially and then G4 were further broken down to G1, G2, and G3. However, the smallest substrate for CcCel48A was cellopentaose (G5), and it was able to cleave G5 and G6 to G2, G3, and G4 (Figure 1C). All of the cello-oligosaccharides including G2-G6 could be degraded to G1 completely by BlgA (Meng et al., 2015).
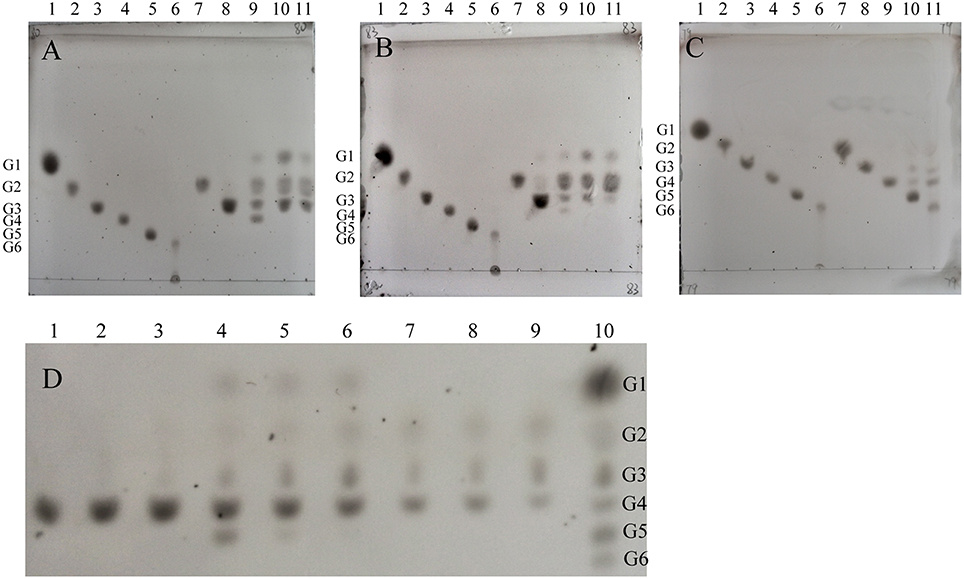
Figure 1. Product patterns of CcCel9A(A,D), CcCel9B(B) and CcCel48A(C) on cello-oligosaccharides. (A–C): G1-G6 (lanes 1-6) refer to the positions of standards (4 μg of each): glucose (G1), cellobiose (G2), cellotriose (G3), cellotetraose (G4), cellopentaose (G5), and cellohexose (G6), respectively. Reactions containing G2-G6 (0.4%) were incubated at 60°C for 16 h with 0.5 μmol enzyme. Samples loaded in lanes 7-11 were the hydrolysis products of G2 (1 μL), G3 (2 μL), G4 (2 μL), G5 (2 μL), and G6 (3 μL), respectively. (D): CcCel9A aliquots were incubated for 10, 30, and 120 min with cellotetraose (G4, lanes 1-3), cellopentaose (G5, lanes 4-6), and cellohexaose (G6, lanes 7-9). Lane 10, markers G1-G6 (from top down).
The molar ratio of synergistic enzyme was crucial for efficient saccharification of cellulose (Kim et al., 2014). The approximate ratio of CcCel9A and CcCel9B on the endoglucanase-zymogram calculated by GSV method was 1:1, consequently the ratios of the purified CcCel9A and CcCel9B were optimized based on this data (Table 2). When CcCel9A was mixed with the non-processive endoglucanase CcCel9B, CcCel9B was better at hydrolyzing CMC while CcCel9A did better in filter paper (Figure 2A). BlgA complemented CcCel9A on both CMC and filter paper when they were mixed at 2:1 and 1:2, respectively (Figure 2B). The exoglucanase CcCel48A could stimulate the activity of CcCel9A on filter paper at a ratio of 5:1, but was redundant on hydrolyzing CMC (Figure 2C), which was consistent with the results described in the previous study (Zhang et al., 2010; Kostylev and Wilson, 2014). When CcCel9A, CcCel9B, and BlgA were mixed, the highest hydrolyzing efficiency on CMC was obtained (Figure 2D and Table 3). When CcCel9A, CcCel9B, and CcCel48A were mixed, weak synergy effect was observed (Figure 2E). In the mixture of CcCel9A, CcCel48A, and BlgA, the activity on filter paper was improved significantly (Figure 2F), whereas when all of the four enzymes were mixed together, the highest synergy effect on filter paper was achieved (Figure 2G).
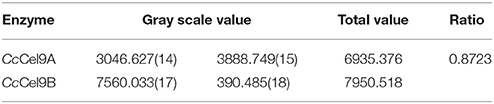
Table 2. The Gray scale value (GSV) of Ccel_3880 and Ccel_3883 on the endoglucanase-zymogram calculated by ImageJ.
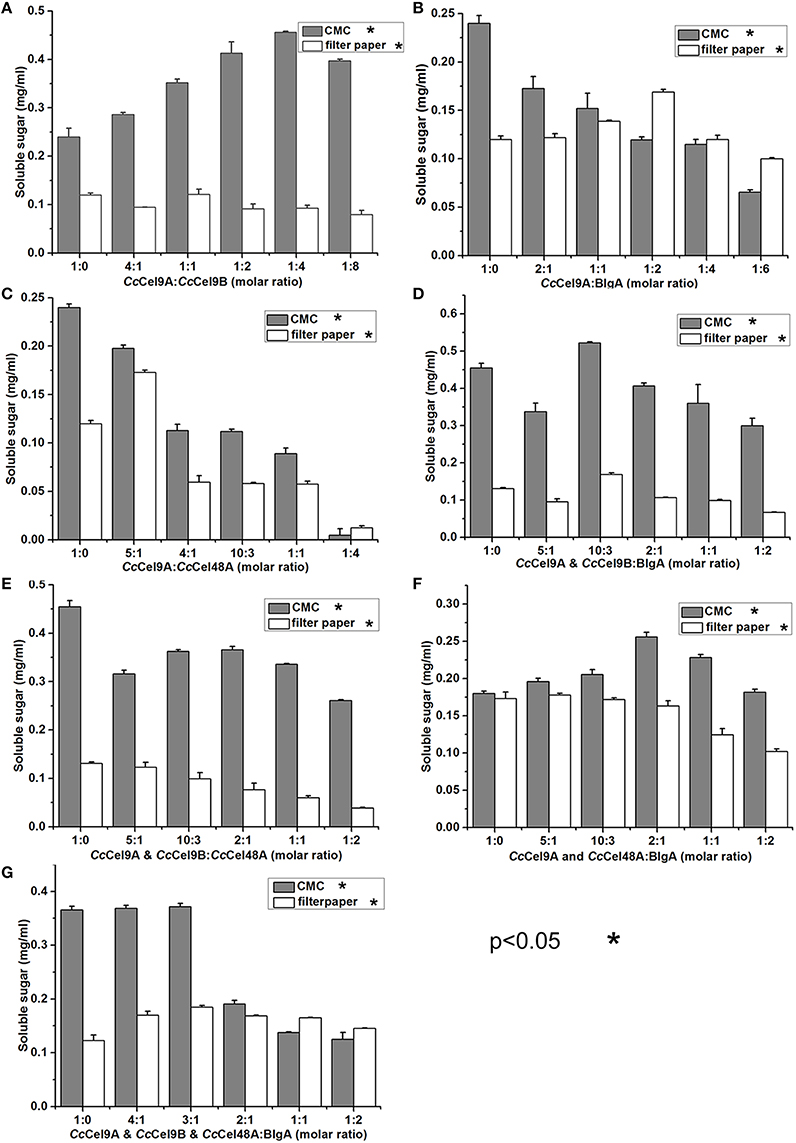
Figure 2. Synergistic effects of a series of enzyme cocktails with a total concentration of 1 μmol l−1 incubated at 60°C with 20 mg ml−1 CMC for 30 min or 5 mg ml−1 filter paper for 4 h, respectively. (A) The molar ratio of CcCel9A to CcCel9B were ranged from 1:0 to 1:8; (B) The molar ratios of CcCel9A to BlgA were ranged from 1:0 to 1:6; (C) The molar ratio of CcCel9A to CcCel48A were ranged from 1:0 to 1:4; (D) The molar ratios of the mixture (CcCel9A & CcCel9B) to BlgA were ranged from 1:0 to 1:2; (E) The molar ratios of the mixture (CcCel9A & CcCel9B) to CcCel48A were ranged from 1:0 to 1:2; (F) The molar ratios of the mixture (CcCel9A & CcCel48A) to BlgA were ranged from 1:0 to 1:2; (G) The molar ratios of the mixture (CcCel9A & CcCel9B & CcCel48A) to BlgA were ranged from 1:0 to 1:2.
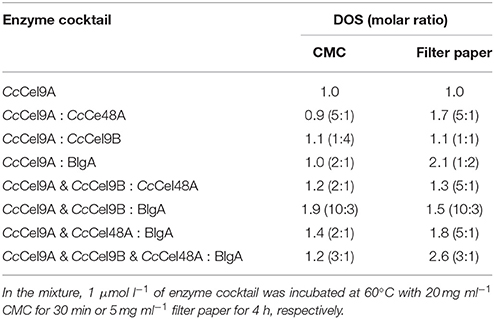
Table 3. The degree of synergy (DOS) for the enzyme cocktail hydrolyzing CMC and filter paper at the optimal ratio.
The hydrolysis processes of filter paper by five enzyme cocktails were compared (Figure 3). The final concentrations of soluble sugars from CcCel9A, CcCel9A & BlgA, CcCel9A & CcCel9B & BlgA, CcCel9A & CcCel48A & BlgA, and CcCel9A & CcCel9B & CcCel48A & BlgA were 0.787, 0.968, 1.191, 1.359, and 1.859 mg ml−1, respectively. The hydrolysis rate of the four-component cocktail was highest with a sugar conversion rate of 33.5%, indicating the best synergy effect occurred among the multiple components of mixtures. Hence, it was applied to corn stalk hydrolysis and 15.8% of the substrate was converted to soluble sugars (Figure 4).
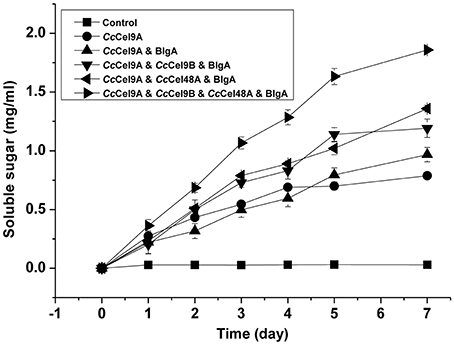
Figure 3. Hydrolysis processes on filter paper of five enzyme cocktails. Each cocktail with a total concentration of 1 μmol l−1 protein was incubated at 60°C with 5 mg ml−1 filter paper. The molar radios for each cocktail were set as follow: CcCel9A: BlgA = 2:1, CcCel9A: CcCel9B: BlgA = 5:5:3, CcCel9A: CcCel48A: BlgA = 25:5:6, and CcCel9A: CcCel9B: CcCel48A: BlgA = 25:25:10:18.
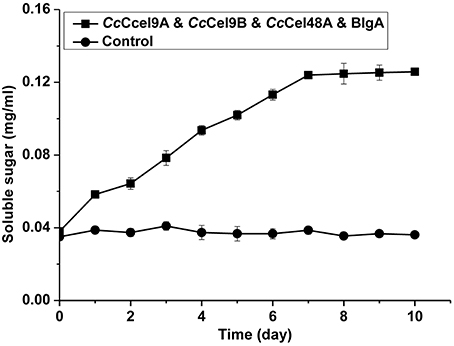
Figure 4. Hydrolysis processes on corn stalk of the four-component enzyme cocktail (CcCel9A: CcCel9B: CcCel48A: BlgA = 25:25:10:18). Totally 1 μmol l−1 enzyme was incubated at 60°C with 0.5 mg ml−1 corn stalk.
The products patterns of filter paper and corn stalk hydrolysis were detected by HPAEC-PAD after incubation at 60°C for a week (Figure 5 and ESM_3.pdf in Supplementary Material). Glucose was the only product of the mixtures, and the yield of glucose in the four-component cocktail was higher than the other cocktails. The mixture of 0.4 μM Cel9 and Cel48, from C. phytofermentans, was incubated with 10 mg ml−1 filter paper at 50°C for 72 h, and 1.620 mg ml−1 sugar containing G1, G2, G3, and G4 was obtained (Zhang et al., 2010). In our four-component cocktail, there was only glucose in the end product, which would be easily utilized by microorganisms for growth and fermentation.
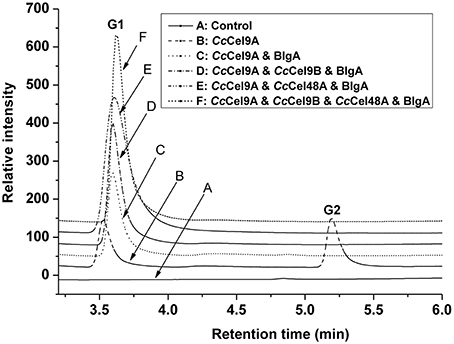
Figure 5. Analysis of end products of filter paper hydrolyzed by enzyme cocktails using high-performance anion-exchange chromatography with pulsed amperometric detection. Each cocktail with a total concentration of 1 μmol l−1 protein was incubated at 60°C with 5 mg ml−1 filter paper. The molar radios for each cocktail were set as follow: CcCel9A: BlgA = 2:1, CcCel9A: CcCel9B: BlgA = 5:5:3, CcCel9A: CcCel48A: BlgA = 25:5:6 and CcCel9A: CcCel9B: CcCel48A: BlgA = 25:25:10:18.
Thermostable bacterial cellulase is the most popular candidate in various industries since it can work effectively under extreme conditions. In the present study, four different cellulases from two thermophilic microorganisms were mixed to obtain the optimal saccharification of cellulose (Figure 6). Therein, CcCel9A, CcCel9B, and CcCel48A were the major components in the secretome of C. cellulosi CS-4-4, which was isolated from decayed corn stalk with an optimal growth at 60°C (Zhang et al., 2014). CcCel9A, a multi-modular processive endoglucanase, could provide the four types of synergisms (Din et al., 1994; Teeri, 1997). On the soluble substrate CMC, CcCel9A, cooperating with the non-processive endoglucanase CcCel9B and BlgA, could hydrolyze cellulose to glucose. However, CcCel48A was unable to act on CMC, so there was no synergism after adding CcCel48A during the hydrolysis of CMC. On the crystalline substrate filter paper, CcCel48A could collaborate with CcCel9A to destroy the crystalline structure, synergizing with CcCel9A in the rate-limiting step (Figure 2 and Table 3). In addition, CcCel9A, CcCel9B, and CcCel48A are all multi-modular cellulases, synergism between the modules of them might occur in the hydrolysis process, which needed more detailed work.
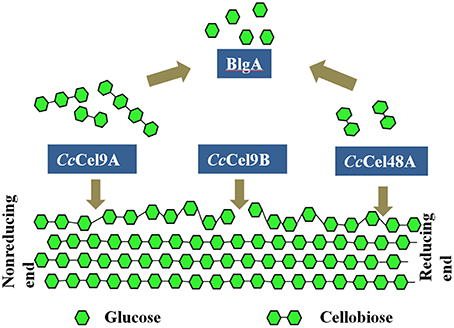
Figure 6. Synergic deconstruction model of cellulose by CcCel9A, CcCel9B, CcCel48A, and BlgA. CcCel9A and CcCel48A processively cleave cellulose from the end of a single chain, and oligosaccharides are released. CcCel9B, an endo-glucanase, cuts randomly in the cellulose chain. Oligosaccharides were hydrolyzed to glucose by BlgA.
Conclusions
CcCel9A and CcCel48A from C. cellulosi CS-4-4 cooperate in destroying the crystalline structure of cellulose, providing single cellulose chain for endoglucanase CcCel9B to cut randomly. Meanwhile, CcCel9A and CcCel48A processively cleave cellulose from the end of a single chain and release G4 and G2, respectively. BlgA from Caldicellulosiruptor sp. F32 is capable of hydrolyzing G2-G6 to G1, which might remove the product inhibition for other cellulases (Kostylev et al., 2012). The ratios and cooperation of cellulases are crucial in the hydrolysis process and the efficiency could be further improved after adding some oxidoreductases (Gardner et al., 2014) or expansins (Georgelis et al., 2015), for which the bacterial enzyme pool is so abundant to excavate in the future.
Author Contributions
FL and KZ conceived and designed research; MY and KZ performed experiments and analyzed data; PZ and XM analyzed data; XZ provided technical assistance to KZ, XM, KZ, and FL wrote the manuscript; all authors commented on the manuscript and approved the contents.
Funding
This work was supported by grants from the Shandong Province Natural Science Funds for Distinguished Young Scholar (No. JQ201507), the Key Scientific and Technological Project of Shandong Province (No. 2015ZDXX0403A01), and the Natural Science Foundation of China (No. 51561145015).
Conflict of Interest Statement
The authors declare that the research was conducted in the absence of any commercial or financial relationships that could be construed as a potential conflict of interest.
Supplementary Material
The Supplementary Material for this article can be found online at: http://journal.frontiersin.org/article/10.3389/fmicb.2016.00932
References
Arantes, V., and Saddler, J. N. (2010). Access to cellulose limits the efficiency of enzymatic hydrolysis: the role of amorphogenesis. Biotechnol. Biofuels 3:4. doi: 10.1186/1754-6834-3-4
Berger, E., Zhang, D., Zverlov, V. V., and Schwarz, W. H. (2007). Two noncellulosomal cellulases of Clostridium thermocellum, Cel9I and Cel48Y, hydrolyse crystalline cellulose synergistically. FEMS Microbiol. Lett. 268, 194–201. doi: 10.1111/j.1574-6968.2006.00583.x
Din, N., Damude, H. G., Gilkes, N. R., Miller, R. C. Jr., Warren, R. A., and Kilburn, D. G. (1994). C1-Cx revisited: intramolecular synergism in a cellulase. Proc. Natl. Acad. Sci. U.S.A. 91, 11383–11387. doi: 10.1073/pnas.91.24.11383
Gardner, J. G., Crouch, L., Labourel, A., Forsberg, Z., Bukhman, Y. V., Vaaje-Kolstad, G., et al. (2014). Systems biology defines the biological significance of redox-active proteins during cellulose degradation in an aerobic bacterium. Mol. Microbiol. 94, 1121–1133. doi: 10.1111/mmi.12821
Georgelis, N., Nikolaidis, N., and Cosgrove, D. J. (2015). Bacterial expansins and related proteins from the world of microbes. Appl. Microbiol. Biotechnol. 99, 3807–3823. doi: 10.1007/s00253-015-6534-0
Gusakov, A. V., Kondratyeva, E. G., and Sinitsyn, A. P. (2011). Comparison of two methods for assaying reducing sugars in the determination of carbohydrase activities. Int. J. Anal. Chem. 2011:283658. doi: 10.1155/2011/283658
He, Y. L., Ding, Y. F., and Long, Y. Q. (1991). Two cellulolytic Clostridium species: Clostridium cellulosi sp. nov. and Clostridium cellulofermentans sp. nov. Int. J. Syst. Bacteriol. 41, 306–309. doi: 10.1099/00207713-41-2-306
Iakiviak, M., Mackie, R. I., and Cann, I. K. (2011). Functional analyses of multiple lichenin-degrading enzymes from the rumen bacterium Ruminococcus albus 8. Appl. Environ. Microbiol. 77, 7541–7550. doi: 10.1128/AEM.06088-11
Jeoh, T., Wilson, D. B., and Walker, L. P. (2006). Effect of cellulase mole fraction and cellulose recalcitrance on synergism in cellulose hydrolysis and binding. Biotechnol. Prog. 22, 270–277. doi: 10.1021/bp050266f
Jeon, S. D., Yu, K. O., Kim, S. W., and Han, S. O. (2012). The processive endoglucanase EngZ is active in crystalline cellulose degradation as a cellulosomal subunit of Clostridium cellulovorans. N. Biotechnol. 29, 365–371. doi: 10.1016/j.nbt.2011.06.008
Kim, I. J., Lee, H. J., Choi, I. G., and Kim, K. H. (2014). Synergistic proteins for the enhanced enzymatic hydrolysis of cellulose by cellulase. Appl. Microbiol. Biotechnol. 98, 8469–8480. doi: 10.1007/s00253-014-6001-3
Kostylev, M., and Wilson, D. (2014). A distinct model of synergism between a processive endocellulase (TfCel9A) and an exocellulase (TfCel48A) from Thermobifida fusca. Appl. Environ. Microbiol. 80, 339–344. doi: 10.1128/AEM.02706-13
Kostylev, M., Moran-Mirabal, J. M., Walker, L. P., and Wilson, D. B. (2012). Determination of the molecular states of the processive endocellulase Thermobifida fusca Cel9A during crystalline cellulose depolymerization. Biotechnol. Bioeng. 109, 295–299. doi: 10.1002/bit.23299
Lynd, L. R., van Zyl, W. H., McBride, J. E., and Laser, M. (2005). Consolidated bioprocessing of cellulosic biomass: an update. Curr. Opin. Biotechnol. 16, 577–583. doi: 10.1016/j.copbio.2005.08.009
Mandelman, D., Belaich, A., Belaich, J. P., Aghajari, N., Driguez, H., and Haser, R. (2003). X-ray crystal structure of the multidomain endoglucanase Cel9G from Clostridium cellulolyticum complexed with natural and synthetic cello-oligosaccharides. J. Bacteriol. 185, 4127–4135. doi: 10.1128/JB.185.14.4127-4135.2003
Meng, D. D., Ying, Y., Zhang, K. D., Lu, M., and Li, F. L. (2015). Depiction of carbohydrate-active enzyme diversity in Caldicellulosiruptor sp. F32 at the genome level reveals insights into distinct polysaccharide degradation features. Mol. Biosyst. 11, 3164–3173. doi: 10.1039/C5MB00409H
Ozdemir, I., Blumer-Schuette, S. E., and Kelly, R. M. (2012). S-layer homology domain proteins Csac_0678 and Csac_2722 are implicated in plant polysaccharide deconstruction by the extremely thermophilic bacterium Caldicellulosiruptor saccharolyticus. Appl. Environ. Microbiol. 78, 768–777. doi: 10.1128/AEM.07031-11
Riedel, K., and Bronnenmeier, K. (1999). Active-site mutations which change the substrate specificity of the Clostridium stercorarium cellulase CelZ implications for synergism. Eur. J. Biochem. 262, 218–223. doi: 10.1046/j.1432-1327.1999.00374.x
Sakon, J., Irwin, D., Wilson, D. B., and Karplus, P. A. (1997). Structure and mechanism of endo/exocellulase E4 from Thermomonospora fusca. Nat. Struct. Biol. 4, 810–818. doi: 10.1038/nsb1097-810
Teeri, T. T. (1997). Crystalline cellulose degradation: new insight into the function of cellobiohydrolases. Trends Biotechnol. 15, 160–167. doi: 10.1016/S0167-7799(97)01032-9
Zhang, K., Chen, X., Schwarz, W. H., and Li, F. (2014). Synergism of glycoside hydrolase secretomes from two thermophilic bacteria cocultivated on lignocellulose. Appl. Environ. Microbiol. 80, 2592–2601. doi: 10.1128/AEM.00295-14
Zhang, Q., Zhang, X., Wang, P., Li, D., Chen, G., Gao, P., et al. (2015). Determination of the action modes of cellulases from hydrolytic profiles over a time course using fluorescence-assisted carbohydrate electrophoresis. Electrophoresis 36, 910–917. doi: 10.1002/elps.201400563
Zhang, X. Z., Sathitsuksanoh, N., and Zhang, Y. H. (2010). Glycoside hydrolase family 9 processive endoglucanase from Clostridium phytofermentans: heterologous expression, characterization, and synergy with family 48 cellobiohydrolase. Bioresour. Technol. 101, 5534–5538. doi: 10.1016/j.biortech.2010.01.152
Zverlov, V. V., Schantz, N., and Schwarz, W. H. (2005). A major new component in the cellulosome of Clostridium thermocellum is a processive endo-beta-1, 4-glucanase producing cellotetraose. FEMS Microbiol. Lett. 249, 353–358. doi: 10.1016/j.femsle.2005.06.037
Zverlov, V. V., Velikodvorskaya, G. A., and Schwarz, W. H. (2003). Two new cellulosome components encoded downstream of celI in the genome of Clostridium thermocellum: the non-processive endoglucanase CelN and the possibly structural protein CseP. Microbiology 149(Pt 2), 515–524. doi: 10.1099/mic.0.25959-0
Keywords: cellulose, secretome, synergism, processive endoglucanase, β-glucosidase
Citation: Yang M, Zhang K-D, Zhang P-Y, Zhou X, Ma X-Q and Li F-L (2016) Synergistic Cellulose Hydrolysis Dominated by a Multi-Modular Processive Endoglucanase from Clostridium cellulosi. Front. Microbiol. 7:932. doi: 10.3389/fmicb.2016.00932
Received: 28 February 2016; Accepted: 31 May 2016;
Published: 15 June 2016.
Edited by:
Weiwen Zhang, Tianjin University, ChinaReviewed by:
Christopher L. Hemme, University of Rhode Island, USAYejun Han, Chinese Academy of Sciences, China
Copyright © 2016 Yang, Zhang, Zhang, Zhou, Ma and Li. This is an open-access article distributed under the terms of the Creative Commons Attribution License (CC BY). The use, distribution or reproduction in other forums is permitted, provided the original author(s) or licensor are credited and that the original publication in this journal is cited, in accordance with accepted academic practice. No use, distribution or reproduction is permitted which does not comply with these terms.
*Correspondence: Xiao-Qing Ma, bWF4cUBxaWJlYnQuYWMuY24=;
Fu-Li Li, bGlmbEBxaWJlYnQuYWMuY24=
†These authors have contributed equally to this work.