- 1Novel Bacteria and Drug Discovery Research Group, School of Pharmacy, Monash University Malaysia, Bandar Sunway, Malaysia
- 2Biomedical Research Laboratory, Jeffrey Cheah School of Medicine and Health Sciences, Monash University Malaysia, Bandar Sunway, Malaysia
- 3Biochemistry Program, Faculty of Science, Institute of Biological Sciences, University of Malaya, Kuala Lumpur, Malaysia
- 4Division of Genetics and Molecular Biology, Faculty of Science, Institute of Biological Sciences, University of Malaya, Kuala Lumpur, Malaysia
- 5Center of Health Outcomes Research and Therapeutic Safety (Cohorts), School of Pharmaceutical Sciences, University of Phayao, Phayao, Thailand
A novel strain, Streptomyces antioxidans MUSC 164T was recovered from mangrove forest soil located at Tanjung Lumpur, Malaysia. The Gram-positive bacterium forms yellowish-white aerial and brilliant greenish yellow substrate mycelium on ISP 2 agar. A polyphasic approach was used to determine the taxonomy status of strain MUSC 164T. The strain showed a spectrum of phylogenetic and chemotaxonomic properties consistent with those of the members of the genus Streptomyces. The cell wall peptidoglycan was determined to contain LL-diaminopimelic acid. The predominant menaquinones were identified as MK-9(H6) and MK-9(H8), while the identified polar lipids consisted of aminolipid, diphosphatidylglycerol, glycolipid, hydroxyphosphatidylethanolamine, phospholipid, phosphatidylinositol, phosphatidylethanolamine, phosphatidylglycerol and lipid. The cell wall sugars consist of galactose, glucose and ribose. The predominant cellular fatty acids (>10.0%) were identified as iso-C15:0 (34.8%) and anteiso-C15:0(14.0%). Phylogenetic analysis identified that closely related strains for MUSC 164T as Streptomyces javensis NBRC 100777T (99.6% sequence similarity), Streptomyces yogyakartensis NBRC 100779T (99.6%) and Streptomyces violaceusniger NBRC 13459T (99.6%). The DNA–DNA relatedness values between MUSC 164T and closely related type strains ranged from 23.8 ± 0.3% to 53.1 ± 4.3%. BOX-PCR fingerprints comparison showed that MUSC 164T exhibits a unique DNA profile, with DNA G + C content determined to be 71.6 mol%. Based on the polyphasic study of MUSC 164T, it is concluded that this strain represents a novel species, for which the name Streptomyces antioxidans sp. nov. is proposed. The type strain is MUSC 164T (=DSM 101523T = MCCC 1K01590T). The extract of MUSC 164T showed potent antioxidative and neuroprotective activities against hydrogen peroxide. The chemical analysis of the extract revealed that the strain produces pyrazines and phenolic-related compounds that could explain for the observed bioactivities.
Introduction
Many therapeutic agents, such as antibiotics, anti-inflammatory and antioxidant compounds have been isolated from microorganisms (Bérdy, 2005; Williams, 2009). Discovery of these bacterial-derived bioactive compounds has a major impact on human health, helping people to live longer and reducing the mortality rate due to infectious and/or chronic diseases. In recent years, the accumulation of free radicals or oxidative stress has been identified as one of the major contribution to neuronal loss and occur early in all major neurodegenerative diseases (Lin and Beal, 2006; Fischer and Maier, 2015; Leszek et al., 2016). By reducing the presence of free radicals, increased intake of antioxidants is known to prevent and decrease the risk of these chronic diseases (Devasagayam et al., 2004; Bonda et al., 2010). Thus, continuous efforts have been directed toward searching for potent, natural antioxidants to prevent the deleterious effects of free radicals.
Over the years, exploring new taxa remains as one of the successful strategies which lead to discovery of therapeutic agents (Williams, 2009). As the most prolific producer of bioactive compounds, Streptomyces genus was initially proposed by Waksman and Henrici (1943) and metabolites isolated from these organisms have been shown to possess pharmaceutically relevant activities such as anti-inflammatory, antimicrobial, antioxidant activities (Bérdy, 2005; Wang et al., 2013; Kumar et al., 2014; Ser et al., 2015a, 2016a; Tan et al., 2016). Moreover, the metabolites derived from Streptomyces are described as potent protective agents in neuronal cells against oxidative stress induced damage. In fact, a recent study by Leiros et al. (2013) has identified seven bioactive compounds produced by Streptomyces sp. which protects against hydrogen peroxide (H2O2) challenge in primary cortical neurons. Unfortunately, many previous drug screening program focused on novel actinomycetes from terrestrial source, which in turn resulted in inefficient rediscovery of known bioactive compounds. Thus, researchers began to divert their attention to new or underexplored habitats, in hope to find new species that may yield promising bioactive compounds.
As one of the world's most dynamic environments, the mangrove ecosystem yields commercial forest products, supports coastal fisheries and protects coastlines (Alongi, 2008). Recently, there has been a renewed interest in the mangrove microorganisms' resources, considering that the changes in salinity and tidal gradient in the mangrove can trigger metabolic adaptations that could result in valuable metabolites production (Hong et al., 2009; Lee et al., 2014a; Azman et al., 2015). Several studies have discovered novel actinobacteria from the poorly explored mangrove environments, demonstrated by the isolation of Streptomyces xiamenensis (Xu et al., 2009), Streptomyces sanyensis (Sui et al., 2011), Streptomyces qinglanensis (Hu et al., 2012), Streptomyces pluripotens (Lee et al., 2014b), Streptomyces gilvigriseus (Ser et al., 2015b), and Streptomyces mangrovisoli (Ser et al., 2015a). Some of these novel strains are known to be bioactive strains as they were found to produce potent compounds with antibacterial, antifibrotic and antioxidant activities. Overall, these findings emphasized that these mangrove-derived Gram-positive filamentous bacteria could be potentially useful for discovery of new drugs or drug leads for neurodegenerative diseases which role of oxidative stress has been implicated, including Parkinson's diseases, Alzheimer's disease and multiple sclerosis.
In this study, a novel strain, MUSC 164T was discovered from a mangrove soil located in east coast of Peninsular Malaysia. A polyphasic approach determined that MUSC 164T represents a novel species of the Streptomyces genus, for which the name Streptomyces antioxidans sp. nov. is proposed. As a means to explore the bioactivities possessed by the strain, the extract of MUSC 164T was subjected to several antioxidant assays prior to in vitro neuroprotective screening against hydrogen peroxide (H2O2). Gas chromatography-mass spectrometry (GC-MS) was used to perform chemical analysis for MUSC 164T extract in order to reveal the chemical constituents present in the extract. Taken altogether, this study has implicated the potential of the mangrove-derived strain Streptomyces antioxidans sp. nov. in producing bioactive compounds, specifically with antioxidative and neuroprotective activities.
Materials and Methods
Isolation and Maintenance of Isolate
Strain MUSC 164T was recovered from a soil sample collected at site MUSC-TLS4 (3° 48′ 21.3″ N 103° 20′ 3.3″ E), located in the mangrove forest of Tanjung Lumpur in the state of Pahang, Peninsular Malaysia in December 2012. Topsoil samples of the upper 20 cm layer (after removing the top 2–3 cm) were collected and sampled into sterile plastic bags using an aseptic metal trowel, and stored at −20°C. Air-dried soil samples were ground with a mortar and pestle. Selective pretreatment of soil samples was performed using wet heat in sterilized water (15 min at 50°C; Takahashi et al., 1996). Five grams of the pretreated air-dried soil was mixed with 45 mL sterilized water and mill ground, spread onto the isolation medium ISP 2 (Shirling and Gottlieb, 1966) supplemented with cycloheximide (25 μg/mL) and nystatin (10 μg/mL), and incubated at 28°C for 14 days. Pure cultures of strain MUSC 164T were isolated and maintained on slants of ISP 2 agar and in glycerol suspensions (20% v/v).
Genomic and Phylogenetic Analyses
Extraction of DNA was performed as previously described (Hong et al., 2009), followed by 16S rRNA gene amplification carried out as stated by Lee et al. (2014b). Using CLUSTAL-X software, the 16S rRNA gene sequence of strain MUSC 164T was aligned with representative sequences of related type strains of the genus Streptomyces retrieved from the GenBank/EMBL/DDBJ databases (Thompson et al., 1997). Subsequently, the alignment was verified manually and adjusted before constructing the phylogenetic trees with the neighbor-joining (Saitou and Nei, 1987; Figure 1) and maximum-likelihood algorithms (Felsenstein, 1981; Figure S1), using the MEGA version 6.0 (Tamura et al., 2013). Evolutionary distances for the neighbor-joining algorithm were computed using Kimura's two-parameter model (Kimura, 1980). Calculations of sequence similarity was performed using EzTaxon-e server (http://eztaxon-e.ezbiocloud.net/) (Kim et al., 2012). The stability of the resultant trees topologies were evaluated by using the bootstrap resampling method of Felsenstein (1985).
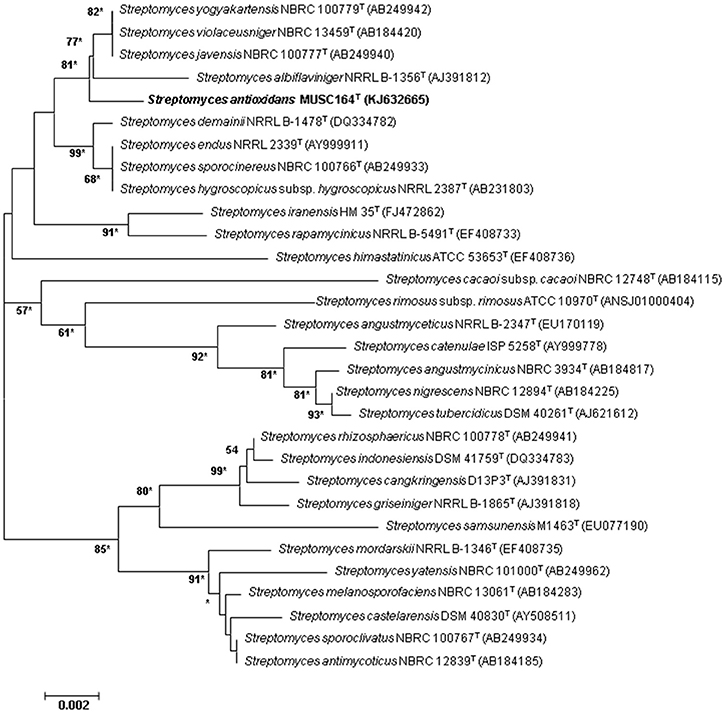
Figure 1. Neighbor-joining phylogenetic tree based on almost complete 16S rRNA sequences (1491 nucleotides) showing the relationship between strain MUSC 164T and representatives of some other related taxa. Numbers at nodes indicate percentages of 1000 bootstrap re-samplings, only values above 50% are shown. Bar, 0.002 substitutions per site. Asterisks indicate that the corresponding nodes were also recovered using the maximum-likelihood tree-making algorithm.
BOX-PCR fingerprint analysis was carried out to characterize strain MUSC 164T and the closely related strains using the primer BOX-A1R (5′-CTACGGCAAGGCGACGCTGACG-3′; Versalovic et al., 1991; Lee et al., 2014c). The PCR condition for BOX-PCR was performed as described by Lee et al. (2014d) and the PCR products were visualized by 2% agarose gel electrophoresis.
Genomic DNA extractions for DNA-DNA hybridization of strain MUSC 164T, Streptomyces javensis NBRC 100777T, Streptomyces violaceusniger NBRC 100779T and Streptomyces yogyakartensis NBRC 13459T were performed by the Identification Service of the DSMZ, Braunschweig, Germany following the protocol of Cashion et al. (1977). DNA-DNA hybridization was conducted as described by De Ley et al. (1970) with slight modifications described by Huss et al. (1983). The G + C content of strain MUSC 164T was determined by HPLC (Mesbah et al., 1989).
Chemotaxonomic Characteristics
The analyses of peptidoglycan amino acid composition and sugars of strain MUSC 164T were conducted by the Identification Service of the DSMZ using published protocols (Schumann, 2011). Analysis of respiratory quinones, polar lipids (Kates, 1986) and fatty acids (Sasser, 1990) were carried out by the Identification Service of the DSMZ. Major diagnostic cell wall sugars of strain MUSC 164T were obtained as described by Whiton et al. (1985) and analyzed by TLC on cellulose plates (Staneck and Roberts, 1974).
Phenotypic Characteristics
The cultural characteristics of strain MUSC 164T were determined following growth on ISP 2, ISP 3, ISP 4, ISP 5, ISP 6, and ISP 7 agar (Shirling and Gottlieb, 1966), actinomycetes isolation agar (AIA; Atlas, 1993), Streptomyces agar (SA; Atlas, 1993), starch casein agar (SCA; Küster and Williams, 1964) and nutrient agar (Macfaddin, 2000) for 14 days at 28°C. The colony color was examined by using the ISCC-NBS color charts (Kelly, 1964). Light microscopy (80i, Nikon) and scanning electron microscopy (JEOL-JSM 6400) were utilized to evaluate the morphology of the strain after incubation on ISP 2 medium at 28°C for 7–14 days (Figure 2). Gram staining was performed and confirmed by using KOH lysis (Cerny, 1978). The growth temperature range was tested at 12–48°C (at intervals of 4°C) on ISP 2 agar, while the pH range for growth was tested in tryptic soy broth (TSB) between pH 4.0–10.0 (at intervals of 1.0 pH unit). Tolerance of NaCl was tested in TSB with concentrations ranging from 0 to 18% (w/v) at intervals of 2%. The responses to temperature, pH and NaCl were observed for 14 days. Catalase activity and production of melanoid pigments were determined following protocols described by Lee et al. (2014e). Hemolytic activity was examined after incubation at 32°C for 7–14 days using blood agar medium containing 5% (w/v) peptone, 3% (w/v) yeast extract, 5% (w/v) NaCl, and 5% (v/v) horse blood (Carrillo et al., 1996). Amylolytic, cellulase, chitinase, lipase, protease, and xylanase activities were determined by growing cells on ISP 2 medium as described by Meena et al. (2013). The carbon-source utilization and chemical sensitivity assays were determined using Biolog GenIII MicroPlates (Biolog, USA) according to the manufacturer's instructions.
All of the phenotypic assays mentioned were performed concurrently for strain MUSC 164T, Streptomyces javensis NBRC 100777T, Streptomyces violaceusniger NBRC 100779T and Streptomyces yogyakartensis NBRC 13459T.
Extract Preparation of MUSC 164T
Seed medium was prepared by cultivating strain MUSC 164T in TSB for 14 days prior to fermentation process. Fermentation was conducted in 500 mL Erlenmeyer flask containing 200 mL of sterile FM 3 medium, shaking at 200 rpm for 7–10 days at 28°C (Hong et al., 2009; Lee et al., 2012). The cell mass was separated by centrifugation at 12000 × g for 15 min and the supernatant was filtered and freeze-dried. The freeze-dried sample was extracted repeatedly with methanol. Subsequently, the extracting solvent was removed and concentrated by rotary vacuum evaporator at 40°C. The extract of MUSC 164T was retrieved and suspended in dimethyl sulphoxide (DMSO) as vehicle reagent prior to bioactivity screening assays.
Determination of Antioxidant Activity of MUSC 164T Extract
2,2-diphenyl-1-picrylhydrazyl (DPPH) scavenging activity by MUSC 164T extract was determined using previous protocol with minor modification (Ser et al., 2015a). The reduction in radical is measured as decrease in the absorbance of 515 nm. Volume of 195 μL of 0.016% DPPH ethanolic solution was added to 5 μL of extract solution to make up final volume of 200 μL. Gallic acid was included as positive control. Reactions were carried out at room temperature in dark for 20 min before measurement with spectrophotometer at 515 nm. DPPH scavenging activity was calculated as follows:
SOD activity was determined using SOD assay Kit − WST (Sigma-Aldrich) following manufacturer's instructions (Tan et al., 2015). Twenty microliter of sample solution was added to sample and blank 2 wells, while 20 μL of ddH2O was added to blank 1 and blank 3 wells. Subsequently, WST working solution (20 μL) was then added to each well followed by 20 μL of enzyme working solution to the sample and blank 1 wells. The resultant mixtures were mixed thoroughly and incubated at 37°C for 20 min. The absorbance was read at 450 nm and superoxide anion scavenging activity was calculated as follows:
2,2′-azino-bis(3-ethylbenzothiazoline-6-sulphonic acid) (ABTS) assay was performed as previously described in published literature with some modifications (Miser-Salihoglu et al., 2013; Ser et al., 2016b). ABTS radical cation (ABTS•) was generated by reacting ABTS stock solution (7 mM) and potassium persulphate (2.45 mM) for 24 h prior to assay. The change in radical amount was indicated by decrease in absorbance at 743 nm. Metal-chelating activity was measured as described by Manivasagan et al. (2013) with slight modification. 2 mM of FeSO4was added to extract and the reaction was initiated by adding 5 mM of ferrozine before measuring at 562 nm using spectrophotometer.
Cell Lines Maintenance and Growth Condition
Neuronal SH-SY5Y cells were maintained in DMEM media (supplemented with 10% FBS) in humidified incubator (5% CO2 in air at 37°C) as described by Wong et al. (2012) with minor modification.
Assessment of Toxicity and Neuroprotective Activity of MUSC 164T Extract
Confluence cells were harvested and seeded at a density of 3 × 104 cells/well into a sterile flat bottom 96-well plate. The seeded cells were allowed to adhere for 48 h before the experiment. Firstly, toxicity of the extract was investigated by treating the cells MUSC 164T extract (0–400 μg/mL) for 24 h prior to measurement of cell viability using MTT assay (Chan et al., 2012). On the other hand, for evaluation of neuroprotective activity, cells were pretreated with MUSC 164T (0–400 μg/mL) extract for 2 h prior to treatment with H2O2 (Wong et al., 2012). For these screenings, media with DMSO was included as negative control along with a non-inoculated extract. Subsequently, MTT assay was performed 24 h after H2O2 treatment. Tetrazolium salt solution was added into each well and incubated further for 4 h. Plates were analyzed in microplate reader at 570 nm (with a reference wavelength of 650 nm). The percentage of cell viability was calculated as follows:
Gas Chromatography-Mass Spectrometry (GC-MS) Analysis
GC-MS analysis was performed in accordance with our previous developed method with slight modification (Supriady et al., 2015; Ser et al., 2015a). The machine used was Agilent Technologies 6980N (GC) equipped with 5979 Mass Selective Detector (MS), HP-5MS (5% phenyl methyl siloxane) capillary column of dimensions 30.0 m × 250 × 0.25 μm and used helium as carrier gas at 1 mL/min. The column temperature was programmed initially at 40°C for 10 min, followed by an increase of 3°C/min to 250°C and was kept isothermally for 5 min. The MS was operating at 70 eV. The constituents were identified by comparison of their mass spectral data with those from NIST 05 Spectral Library.
Statistical Analysis
Experiments to evaluate bioactivities were performed in quadruplicate and all results were expressed as mean ± standard deviation (SD). Statistical analysis was performed using one-way analysis of variance (ANOVA) with SPSS statistical analysis software. A difference was considered statistically significant when p ≤ 0.05.
Results and Discussion
Biologically active compounds isolated from microorganisms remain to be vital for development of new drugs, particularly pharmaceutical and agricultural industry (Bérdy, 2005; Jensen et al., 2005). Oxidative stress has been implicated in pathogenesis of various chronic human disease, particularly neurodegenerative diseases (Radi et al., 2014). An imbalance in free radicals production and antioxidant mechanisms causes modifications and damages on biological macromolecules including protein, lipid and DNA. These detrimental effects of free radicals eventually lead to neuronal cell loss–a scenario which is commonly seen in neurodegenerative diseases (Uttara et al., 2009). Antioxidants are responsible of removing or reducing amount of these harmful radicals; high intake of antioxidants have been associated with reduced risk of developing neurodegenerative diseases (Bonda et al., 2010; Lassmann and van Horssen, 2015). The need to search for novel, potent antioxidative agents to combat against these diseases has called upon researchers to venture into new or underexplored habitats for the discovery of bioactive strains that could produce potent antioxidant(s) (Harvey, 2000; Penesyan et al., 2010). In current study, the mangrove forest soil-derived MUSC 164T strain shows abundant growth on ISP 2, ISP 3, ISP 5, ISP 6, ISP 7 agar, actinomycetes isolation agar, nutrient agar and starch casein agar after 7–14 days at 28°C. The strain grows moderately on Streptomyces agar, and does not grow on ISP 4 agar. The colors of the aerial and substrate mycelium were media-dependent as indicated by Table S1. Both aerial and vegetative hyphae were abundant, well developed and not fragmented as observed observed from 14-day-old culture grown on ISP 2 agar. These morphological features are consistent with assignment of the strain to the genus Streptomyces (Williams et al., 1989). Growth was found to occur at 26–36°C (optimum 28–32°C), with 0–6% NaCl tolerance (optimum 0–2%) and at pH 6.0–8.0 (optimum pH 7.0). Cells were found to be positive for catalase but lack of hemolytic activity and melanoid pigment production. Furthermore, cells were capable of hydrolyzing soluble starch and carboxymethylcellulose, but unable to hydrolyze casein, chitin, tributyrin (lipase) and xylan. Using a range of phenotypic properties, strain MUSC 164T can be differentiated from closely related members of the genus Streptomyces (Table 1). Furthermore, chemical sensitivity assays showed that the strain was resistant to aztreonam, fusidic acid, guanine HCl, lincomycin, lithium chloride, minocycline, nalidixic acid, niaproof 4, potassium tellurite, rifamycin RV, sodium bromate, sodium butyrate, 1% sodium lactate, tetrazolium blue, tetrazolium violet, troleandomycin, and vancomycin.
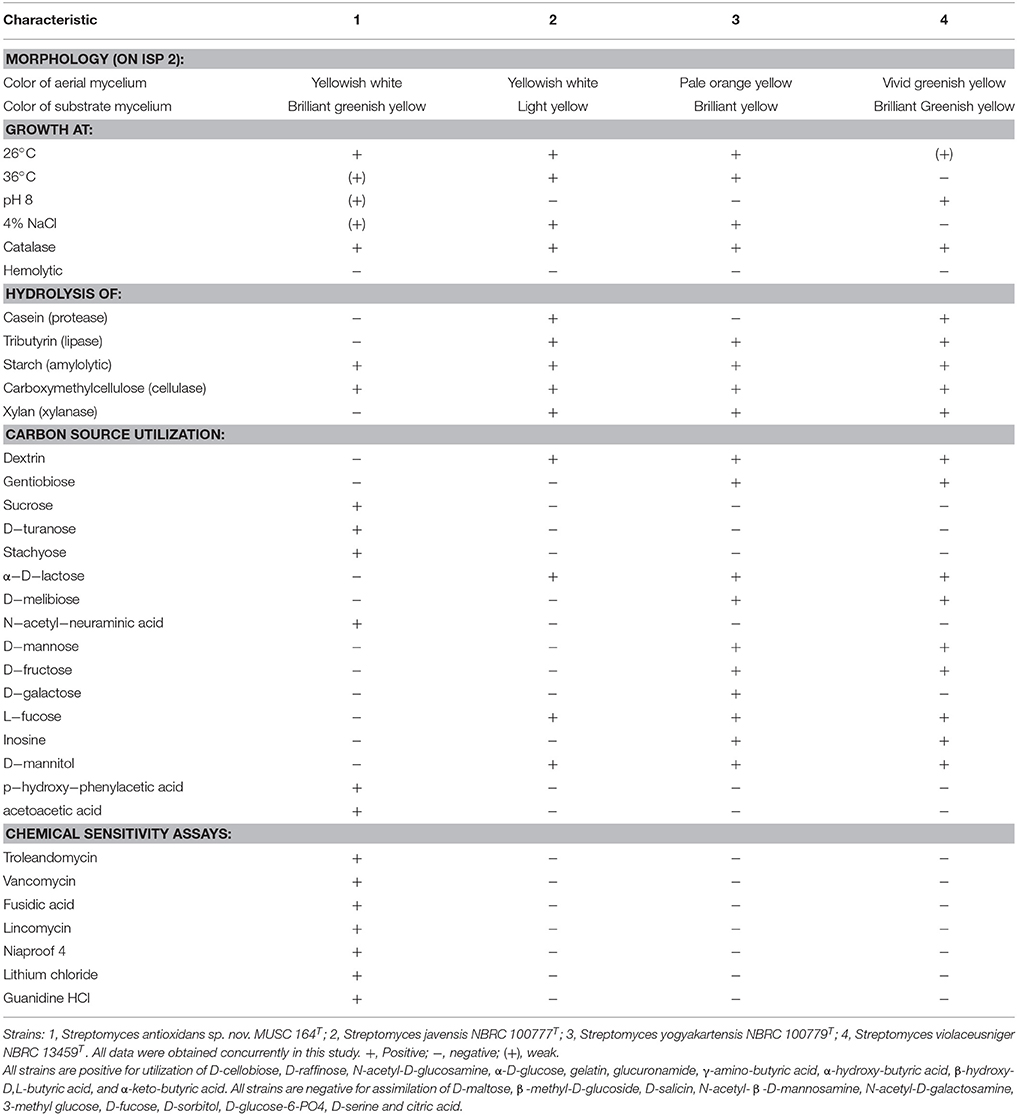
Table 1. Differentiation characteristics of strain MUSC 164T and type strains of phylogenetically closely related species of the genus Streptomyces.
The nearly complete 16S rRNA gene sequence was obtained for strain MUSC 164T (1491 bp; GenBank/EMBL/DDBJ accession number KJ632665) and phylogenetic trees were reconstructed to determine the phylogenetic position of this strain (Figure 1, Figure S1). Phylogenetic analysis exhibited that closely related strains include Streptomyces javensis NBRC 100777T, Streptomyces yogyakartensis NBRC 100779T and Streptomyces violaceusniger NBRC 13459T, as they formed a distinct clade at high bootstrap value of 81% (Figure 1). The analysis of 16S rRNA gene sequence for strain MUSC 164T exhibited highest similarity to strain Streptomyces javensis NBRC 100777T (99.6% sequence similarity), Streptomyces yogyakartensis NBRC 100779T (99.6%) and Streptomyces violaceusniger NBRC 13459T (99.6%); while the type strains of other species of the genus Streptomyces showed sequences similarities below 99.3%. The DNA–DNA relatedness values between strain MUSC 164T and Streptomyces javensis NBRC 100777T (23.8 ± 0.2%), Streptomyces yogyakartensis NBRC 100779T (30.2 ± 2.7%) and Streptomyces violaceusniger NBRC 13459T (53.1 ± 4.4%) were significantly below 70% which was reported as the threshold value for the delineation of bacterial species (Wayne et al., 1987). The BOX-PCR analysis revealed a unique fingerprint pattern by strain MUSC 164T as compared with its closely related type strains (Figure S2). These results further supported the results of DNA-DNA hybridizations and phylogenetic analysis, which indicated the novel status of strain MUSC 164T in the genus Streptomyces.
The major cellular fatty acids in MUSC 164T were identified as iso-C15:0 (34.8%) and anteiso-C15:0 (14.0%) (Table 2). The fatty acids profile of MUSC 164T displayed some levels of similarities with those of closely related phylogenetic neighbors such as Streptomyces javensis NBRC 100777T, Streptomyces yogyakartensis NBRC 100779T and Streptomyces violaceusniger NBRC 13459T, as they contain iso-C15:0 (24.1–36.3%) as their predominant fatty acids. Nonetheless, the fatty acid profile of MUSC 164T was quantitatively different from those of these type strains; for instance, iso-C15:0(34.8%) was found to be predominant in strain MUSC 164T (Table 2), but the amount of the same fatty acid was much lesser in Streptomyces yogyakartensis NBRC 100779T (24.1%). The polar lipids of MUSC 164T were aminolipid, diphosphatidylglycerol, glycolipid, hydroxyphosphatidylethanolamine, phospholipid, phosphatidylinositol, phosphatidylethanolamine, phosphatidylglycerol and lipid. The differences in polar lipid profiles indicated that MUSC 164T differs from related type strains; for example, strain MUSC 164T contain aminolipid and glycolipid (Figure S3A) that were not detected in Streptomyces javensis NBRC 100777T (Figure S3B).
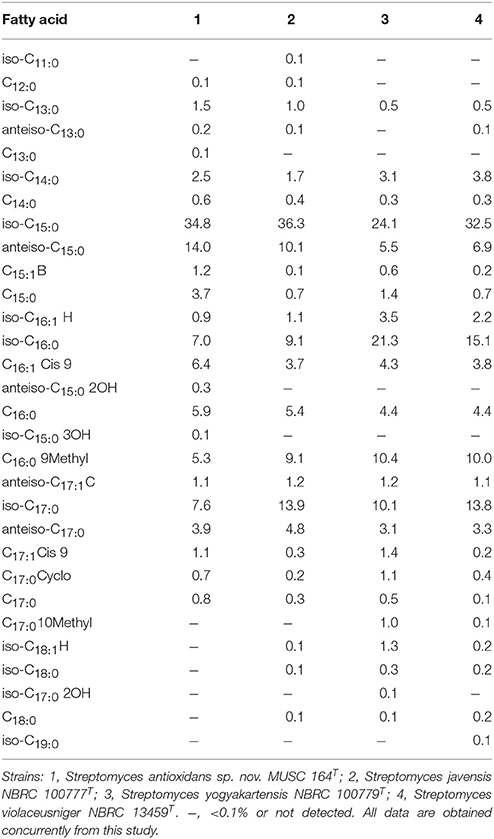
Table 2. Cellular fatty acid composition of strain MUSC 164T and its closely related Streptomyces species.
Strain MUSC 164T presented a type I cell-wall as it contains LL-diaminopimelic acid (Lechevalier and Lechevalier, 1970), an amino acid which has been found in many species of the genus Streptomyces (Lee et al., 2005, 2014b; Xu et al., 2009; Hu et al., 2012; Ser et al., 2015a, Ser et al., 2015b). The predominant menaquinones of strain MUSC 164T were detected as MK-9(H6) (51%) and MK-9(H8) (39%). These findings parallel those reported by Kim et al. (2003). The cell wall sugars detected were galactose, glucose and ribose. The G + C content of strain MUSC 164T was 71.6 mol% which falls within the range of 67.0–78.0 mol% described for species of the genus Streptomyces (Kim et al., 2003).
Based on the results of phylogenetic analysis, DNA-DNA hybridization, chemotaxonomic and phenotypic analysis, it is evident that strain MUSC 164T is different from all other species in the genus Streptomyces; the strain represents a novel species within the genus Streptomyces, for which the name Streptomyces antioxidans sp. nov. is proposed. As an attempt to explore bioactivities possessed by the strain, MUSC 164T extract was subjected to several antioxidant assays. DPPH assay revealed the ability of MUSC 164T to produce free radical scavenging compound(s) as 2 mg/mL of extract showed significant activity at 18.31 ± 2.03% (Table 3). Similarly, the extract was able to reduce ABTS radical and chelate ferrous ion significantly with highest activity recorded to be 30.38 ± 2.27 and 43.66 ± 0.98%, respectively. Additionally, SOD assay suggested antioxidant(s) in MUSC 164T extract with ranging antioxidant activity of 53.09–79.84%, depending on extract concentration. In summary, all the antioxidant assays suggested the potential of MUSC 164T to produce potent antioxidant(s) that could scavenge free radicals and may reduce occurrence of oxidative stress.
As antioxidant assays revealed presence of antioxidant(s) in MUSC 164T extract, in vitro cellular screening assay was then performed. The aim of this screening is to investigate if the extract is capable of protecting neuronal cells against the oxidative stress cellular damage elicited by an oxidative stress inducer, H2O2. Even though with short half-life, H2O2 is highly soluble in water and dissociates to hydroxyl and superoxide ions, which leads to oxidative damage to important macromolecules and ultimately cell death (Triana-Vidal and Carvajal-Varona, 2013). Thus, this molecule has been used widely in oxidative studies (Whittemore et al., 1995; Suematsu et al., 2011; Wong et al., 2012; Triana-Vidal and Carvajal-Varona, 2013). Initially, the viability of SH-SY5Y cells was examined following exposure to the extract up to 400 μg/mL and no significant toxic effect was observed in SH-SY5Y (i.e., cell viability remains >98%). In subsequent neuroprotective experiment, the obtained results have demonstrated MUSC 164T extract confers a significant level of protection on neuronal cells when challenged with H2O2. Highest cell viability was recorded at 80.62 ± 2.75% when neuronal cells were pre-treated with 400 μg/mL of extract (Figure 3) prior to induction by H2O2. As antioxidant assays suggested presence of antioxidant(s) in the extract, these compounds can possibly quench H2O2, preventing oxidative damage against the neuronal cells. Previous study has showed that compounds produced by Streptomyces sp. protect primary cortical neurons against oxidative stress, accompanied by alteration in the expression of apoptotic genes and transcription factor for antioxidant pathways (Leiros et al., 2013). Thus, further study on underlying neuroprotective mechanisms induced by MUSC 164T would be helpful for the development of new drugs in preventing the occurrence of neurodegenerative disorders.
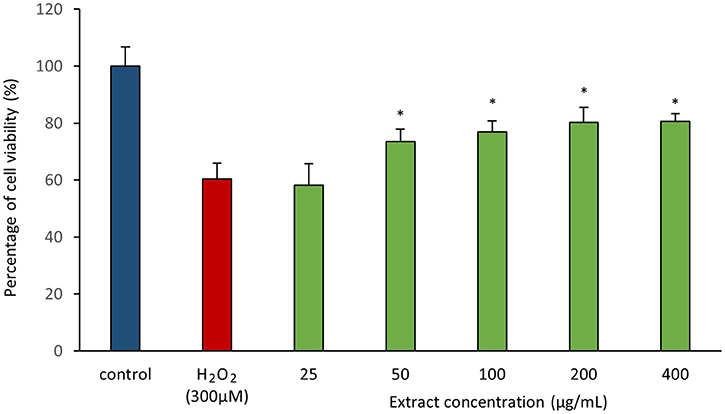
Figure 3. Neuroprotective activity of MUSC 164T extract against hydrogen peroxide (H2O2) in SH-SY5Y cells. The measurement of cell viability was done using MTT assay. Media containing 0.5% DMSO was used as control. All data are expressed as mean ± standard deviation. Symbol (*) indicates p < 0.05 significant difference between the inducer and cells treated with MUSC 164T extract.
Following the evaluation of bioactivities possessed by MUSC 164T extract, GC-MS was performed to assist with the chemical profiling and to identify compounds present in the extract. This technique enables identification of compounds present in Streptomyces extract as GC separates the compounds and MS generates characteristic mass profile for each of the compounds present (Pollak and Berger, 1996; Karanja et al., 2010; Jog et al., 2014). Our results revealed 24 compounds present in MUSC 164T extract (Table 4) and their chemical structures (Figure 4, Figure S4) as Pyrazine, 2,5-dimethyl- (1), Pyrazine, 2,3-dimethyl- (2), Dimethyl trisulfide (3), Pyrazine, 2-ethyl-5-methyl- (4), Pyrazine, trimethyl- (5), Pyrazine, 3-ethyl-2,5-dimethyl- (6), 4-Pyridinamine, N,N,2-trimethyl- (7), 2,3-Dimethyl-5-ethylpyrazine (8), Benzoic acid, methyl ester (9), Pyrazine, 2-methyl-5-(1-propenyl)-, (E)- (10), Pyrazine, 3,5-diethyl-2-methyl- (11), 2-Piperidinone (12), Pyrazine, 2,5-dimethyl-3-(2-methylpropyl)- (13), Indolizine (14), Pyrazine, 2,5-dimethyl-3-(3-methylbutyl)- (15), Pyrazine, 3,5-dimethyl-2-propyl- (16), 2,3,5-Trimethyl-6-ethylpyrazine (17), Phenol, 2,4-bis(1,1-dimethylethyl)- (18), 1,2,3,4-Tetrahydro-cyclopenta[b]indole (19), Pyrrolo[1,2-a]pyrazine-1,4-dione, hexahydro- (20), Phenol, 3,5-dimethoxy- (21), Hexadecanoic acid, methyl ester (22), Pentadecanoic acid, 14-methyl, methyl ester (23) and Pyrrolo[1,2-a]pyrazine-1,4-dione, hexahydro-3-(2-phenylmethyl)- (24).
Through GC-MS, most of the compounds were identified to be heterocyclic organic compounds. Naturally occurring phenolic compounds have been widely accepted as potent antioxidants and these compounds are believed to play an important role in the prevention of chronic diseases resulting from oxidative stress (Soobrattee et al., 2005; Gülcin and Beydemir, 2013). The phenolic compound 18 has been detected in previously isolated Streptomyces sp. from vermicompost and mangrove forest (Narendhran et al., 2014; Tan et al., 2015; Ser et al., 2015a,c). This compound has been shown to exhibit antioxidant activity, probably owing to its hydrogen-donating ability (Brewer, 2011; Narendhran et al., 2014). On the other hand, pyrazines are heterocyclic compounds that contain two nitrogen atoms in their aromatic ring; some of these compounds are known to exhibit various bioactivities including antimicrobial, anticancer, antioxidant, neuroprotection against ischemia/reperfusion injuries and hypoxia (Premkumar and Govindarajan, 2005; Jia et al., 2009; Baldwin et al., 2013; Tan et al., 2015; Ser et al., 2015a,c). Previous studies have demonstrated that microorganisms are capable of producing compounds 1, 2, 5, 6, 15, and 17 with antioxidant activity (Sun et al., 2013; Citron et al., 2015; Pongsetkul et al., 2015). Bacillus methylotrophicus KOSM11 was used in fermentation industry for traditional soybean paste and found to produce compound 1 and 5; the presence of these pyrazines and their related compounds in various food and plants have been linked with antioxidant activities (Liu et al., 2012; Sun et al., 2013; Xu et al., 2015). Furthermore, two pyrrolopyrazines were observed in MUSC 164T extract, which are compound 20 and 24. These compounds were previously detected in several Streptomyces sp. and they have been associated with antioxidant activity exhibited by these strains (Gopi et al., 2014; Tan et al., 2015; Ser et al., 2015a,c). Gopi et al. (2014) has also reported that these compounds were highly capable of scavenging or reducing amount of free radicals when assessed with reducing power assay. Thus, the detection of these heterocyclic compounds present in MUSC 164T could account for the antioxidant activity and protect SH-SY5Y cells against H2O2 insults.
The current study described a novel streptomycete designated as MUSC 164T that produces a mixture of compounds, with some of them could be responsible for the free radical scavenging activities detected via several antioxidant assays. Furthermore, the extract has demonstrated its potential in conferring neuroprotection against oxidative insults, possibly by preventing oxidative stress and activating of antioxidant defense systems that are crucial for survival of SH-SY5Y cells. In conclusion, these preliminary studies have revealed the antioxidative and in vitro neuroprotective properties of MUSC 164T which merit for further investigations focusing on the isolation and characterization of chemical compounds using bioassay-guided purification. The identified bioactive principles could essentially be important for the development of pharmacological agents for neurodegenerative diseases.
Description of Streptomyces antioxidans sp. nov.
Streptomyces antioxidans sp. nov. (an.ti.o′xi.dans. Gr. pref. anti, against; N.L. v. oxidare, to oxidize; N.L. part. adj. antioxidans, non-oxidizing, referring to the antioxidant properties of this strain).
Gram-positive actinobacteria that forms yellowish-white aerial and brilliant greenish yellow substrate mycelium on ISP 2 agar. The colors of the aerial and substrate mycelium are media-dependent (Table S1).
Abundant growth was observed on ISP 2, ISP 3, ISP 5, ISP 6, ISP 7, actinomycetes isolation agar, starch casein agar and nutrient agar after 7–14 days at 28°C; cells grow moderately on Streptomyces agar, and does not grow on ISP 4 agar. Cells grow at 26–36°C (optimum 28–32°C), pH 6.0–8.0 (optimum pH 7.0), with 0–6% NaCl tolerance (optimum 0–2%). Cells are positive for catalase but negative for hemolytic activity and melanoid pigment production. Soluble starch and carboxymethylcellulose are hydrolyzed but casein, chitin, xylan, and tributyrin (lipase) are not. The following compounds are utilized as sole carbon sources: acetic acid, acetoacetic acid, α-D-glucose, α-hydroxy-butyric acid, α-keto-butyric acid, α-keto-glutaric acid, β-hydroxyl-D,L-butyric acid, bromo-succinic acid, D-cellobiose, D-fructose-6-phosphate, D-galactose, D-galacturonic acid, D-glucuronic acid, D-lactic acid methyl ester, D-malic acid, D-raffinose, D-saccharic acid, D-trehalose, D-turanose, formic acid, gelatin, glucuronamide, L-galactonic acid lactone, L-lactic acid, L-rhamnose, N-acetyl-D-glucosamine, N-acetyl-neuraminic acid, pectin, p-hydroxyl-phenylacetic acid, quinic acid, stachyose, sucrose, Tween 40, γ-amino-butyric acid, and myo-inositol. The following compounds are not utilized as sole carbon sources: α-D-lactose, β-methyl-D-glucoside, citric acid, D-arabitol, D-aspartic acid, Dextrin, D-fructose, D-fucose, D-glucose-6-phosphate, D-gluconic acid, D-maltose, D-mannitol, D-mannose, D-melibiose, D-salicin, D-serine, D-sorbitol, gentiobiose, glycerol, glycyl-L-proline, inosine, L-fucose, L-malic acid, methyl pyruvate, mucic acid, N-acetyl-β-D-mannosamine, N-acetyl-D-galactosamine, propionic acid, and 3-methyl glucose. The following compounds are not utilized as sole carbon sources: L-alanine, L-arginine, L-aspartic acid, L-histidine, L-pyroglutamic acid; while L-glutamic acid and L-serine are utilized as sole nitrogen sources.
The cell wall peptidoglycan contains LL-diaminopimelic acid. The predominant menaquinones are MK-9(H6) and MK-9(H8). The polar lipids consist of aminolipid, diphosphatidylglycerol, glycolipid, hydroxyphosphatidylet hanolamine, phospholipid, phosphatidylinositol, phosphatidylethanolamine, phosphatidylglycerol, and lipid. The cell wall sugars are galactose, glucose and ribose. The major cellular fatty acids are iso-C15:0 and anteiso-C15:0.
The type strain, MUSC 164T (=DSM 101523T = MCCC 1K01590T) was isolated from mangrove soil collected from the Tanjung Lumpur mangrove forest (state of Pahang, Peninsular Malaysia). The 16S rRNA gene sequence of strain MUSC 164T has been deposited in GenBank/EMBL/DDBJ under the accession number KJ632665. The G + C content of the genomic DNA of the type strain is 71.6 mol%.
Author Contributions
The experiments, data analysis and manuscript writing were performed by H-LS, LT-HT, B-HG, and L-HL, UDP, SNAM, W-FY, and K-GC provided vital guidance and technical support. L-HL and B-HG founded the research project.
Conflict of Interest Statement
The authors declare that the research was conducted in the absence of any commercial or financial relationships that could be construed as a potential conflict of interest.
Acknowledgments
This work was supported by PVC Award Grant (Project Q7 No. PVC-ECR-2016), External Industry Grant (Biotek Abadi Vote No. GBA-808813), Fundamental Research Grant Scheme (FRGS/1/2013/SKK01/MUSM/03/3), MOSTI eScience funds (Project No. 06-02-10-SF0300) awarded to L-HL and MOSTI eScience funds (Project No. 02-02-10-SF0215) awarded to B-HG, and a University of Malaya for High Impact Research Grant (UM-MOHE HIR Nature Microbiome Grant No. H- 50001-A000027 and No. A000001-50001) awarded to K-GC. The authors are thankful to Professor Bernhard Schink for the support in the Latin etymology of the new species name.
Supplementary Material
The Supplementary Material for this article can be found online at: http://journal.frontiersin.org/article/10.3389/fmicb.2016.00899
References
Alongi, D. M. (2008). Mangrove forests: resilience, protection from tsunamis, and responses to global climate change. Estuar. Coast. Shelf Sci. 76, 1–13. doi: 10.1016/j.ecss.2007.08.024
Azman, A.-S., Othman, I., Velu, S. S., Chan, K.-G., and Lee, L.-H. (2015). Mangrove rare actinobacteria: taxonomy, natural compound, and discovery of bioactivity. Front. Microbiol. 6:856. doi: 10.3389/fmicb.2015.00856
Baldwin, M. V., Arikkatt, S. D., Sindhu, T. J., Chanran, M., Bhat, A. R., and Krishnakumar, K. (2013). A review of biological potential of pyrazine and related heterocyclic compounds. World J. Pharm. Pharmaceut. Sci. 3, 1124–1132. Available online at: http://www.wjpps.com/wjpps_controller/abstract_id/805
Bonda, D. J., Wang, X., Perry, G., Nunomura, A., Tabaton, M., Zhu, X., et al. (2010). Oxidative stress in Alzheimer disease: a possibility for prevention. Neuropharmacology 59, 290–294. doi: 10.1016/j.neuropharm.2010.04.005
Brewer, M. S. (2011). Natural antioxidants: sources, compounds, mechanisms of action, and potential applications. Comp. Rev. Food Sci. Food Safety 10, 221–247. doi: 10.1111/j.1541-4337.2011.00156.x
Carrillo, P. G., Mardaraz, C., Pitta-Alvarez, S. I., and Giulietti, A. M. (1996). Isolation and selection of biosurfactant-producing bacteria. World J. Microbiol. Biotech. 12, 82–84. doi: 10.1007/BF00327807
Cashion, P., Holder-Franklin, M. A., McCully, J., and Frankiln, M. (1977). A rapid method for the base ratio determination of bacterial DNA. Anal. Biochem. 81, 461–466. doi: 10.1016/0003-2697(77)90720-5
Cerny, G. (1978). Studies on the aminopeptidase test for the distinction of gram-negative from gram-positive bacteria. Eur. J. Appl. Microbiol. Biotech. 5, 113–122. doi: 10.1007/BF00498805
Chan, C. K., Goh, B. H., Kamarudin, M. N. A., and Kadir, H. A. (2012). Aqueous fraction of Nephelium ramboutan-ake rind induces mitochondrial-mediated apoptosis in HT-29 human colorectal adenocarcinoma cells. Molecules 17, 6633–6657. doi: 10.3390/molecules17066633
Citron, C. A., Barra, L., Wink, J., and Dickschat, J. S. (2015). Volatiles from nineteen recently genome sequenced actinomycetes. Org. Biomol. Chem. 13, 2673–2683. doi: 10.1039/C4OB02609H
De Ley, J., Cattoir, H., and Reynaerts, A. (1970). The quantitative measurement of DNA hybridization from renaturation rates. Eur. J. Biochem. 12, 133–142. doi: 10.1111/j.1432-1033.1970.tb00830.x
Devasagayam, T. P., Tilak, J. C., Boloor, K. K., Sane, K. S., Ghaskadbi, S. S., and Lele, R. D. (2004). Free radicals and antioxidants in human health: current status and future prospects. J. Assoc. Physicians India 52, 794–804.
Felsenstein, J. (1981). Evolutionary trees from DNA sequences: a maximum likelihood approach. J. Mol. Evol. 17, 368–376. doi: 10.1007/BF01734359
Felsenstein, J. (1985). Confidence limits on phylogenies: an approach using the bootstrap. Evolution 39, 783–789. doi: 10.2307/2408678
Fischer, R., and Maier, O. (2015). Interrelation of oxidative stress and inflammation in neurodegenerative disease: role of TNF. Ox. Med. Cell. longevity 2015:610813. doi: 10.1155/2015/610813
Gopi, M., Dhayanithi, N. B., Devi, K. N., and Kumar, T. T. A. (2014). Marine natural product, Pyrrolo [1,2-a] pyrazine-1,4-dione, hexahydro-(C7H10N2O2) of antioxidant properties from Bacillus species at Lakshadweep archipelago. J. Coast. Life Med. 2, 632–637. doi: 10.12980/JCLM.2.201414J40
Gülcin, I., and Beydemir, S. (2013). Phenolic compounds as antioxidants: carbonic anhydrase isoenzymes inhibitors. Mini Rev. Med. Chem. 13, 408–430. doi: 10.2174/1389557511313030009
Harvey, A. (2000). Strategies for discovering drugs from previously unexplored natural products. Drug Dis. Today 5, 294–300. doi: 10.1016/S1359-6446(00)01511-7
Hong, K., Gao, A.-H., Xie, Q.-Y., Gao, H. G., Zhuang, L., Lin, H.-P., et al. (2009). Actinomycetes for marine drug discovery isolated from mangrove soils and plants in China. Mar. Drugs 7, 24–44. doi: 10.3390/md7010024
Hu, H., Lin, H.-P., Xie, Q., Li, L., Xie, X.-Q., and Hong, K. (2012). Streptomyces qinglanensis sp. nov., isolated from mangrove sediment. Int. J. Syst. Evol. Microbiol. 62, 596–600. doi: 10.1099/ijs.0.032201-0
Huss, V. A. R., Festl, H., and Schleifer, K. H. (1983). Studies on the spectrophotometric determination of DNA hybridization from renaturation rates. Syst. Appl. Microbiol. 4, 184–192. doi: 10.1016/S0723-2020(83)80048-4
Jensen, P. R., Mincer, T. J., Williams, P. G., and Fenical, W. (2005). Marine actinomycete diversity and natural product discovery. Antonie van Leeuwenhoek 87, 43–48. doi: 10.1007/s10482-004-6540-1
Jia, J., Zhang, X., Hu, Y. S., Wu, Y., Wang, Q. Z., Li, N. N., et al. (2009). Protective effect of tetraethyl pyrazine against focal cerebral ischemia/reperfusion injury in rats: therapeutic time window and its mechanism. Throm. Res. 123, 727–730. doi: 10.1016/j.thromres.2008.11.004
Jog, R., Pandya, M., Nareshkumar, G., and Rajkumar, S. (2014). Mechanism of phosphate solubilization and antifungal activity of Streptomyces spp. isolated from wheat roots and rhizosphere and their application in improving plant growth. Microbiology 160, 778–788. doi: 10.1099/mic.0.074146-0
Karanja, E., Boga, H., Muigai, A., Wamunyokoli, F., Kinyua, J., and Nonoh, J. (2010). Growth Characteristics and production of secondary metabolites from selected novel Streptomyces species isolated from selected Kenyan national parks. Sci. Conf. Proc. 51–80. Available online at: http://journals.jkuat.ac.ke/index.php/jscp/article/view/672
Kelly, K. L. (1964). Inter-Society Color Council–National Bureau of Standards Color Name Charts Illustrated with Centroid Colors. Washington, DC: US Government Printing Office
Kim, S. B., Lonsdale, J., Seong, C. N., and Goodfellow, M. (2003). Streptacidiphilus gen. nov., acidophilic actinomycetes with wall chemotype I and emendation of the family Streptomycetaceae (Waksman and Henrici (1943)AL) emend. Rainey et al. 1997. Antonie van Leeuwenhoek 83, 107–116. doi: 10.1023/A:1023397724023
Kim, O. S., Cho, Y. J., Lee, K., Yoon, S. H., Kim, M., Na, H., Park, S. C., et al. (2012). Introducing EzTaxon-e: a prokaryotic 16S rRNA gene sequence database with phylotypes that represent uncultured species. Int. J. Syst. Evol. Microbiol. 62, 716–721. doi: 10.1099/ijs.0.038075-0
Kimura, M. (1980). A simple method for estimating evolutionary rates of base substitutions through comparative studies of nucleotide sequences. J. Mol. Evol. 16, 111–120. doi: 10.1007/BF01731581
Kumar, V., Naik, B., Gusain, O., and Bisht, G. S. (2014). An actinomycete isolate from solitary wasp mud nest having strong antibacterial activity and kills the Candida cells due to the shrinkage and the cytosolic loss. Front. Microbiol. 5:446. doi: 10.3389/fmicb.2014.00446
Küster, E., and Williams, S. (1964). Media for the isolation of streptomycetes: starch casein medium. Nature 202, 928–929. doi: 10.1038/202928a0
Lassmann, H., and van Horssen, J. (2015). Oxidative stress and its impact on neurons and glia in multiple sclerosis lesions. BBA Mol. Basis Dis. 1862, 506–510. doi: 10.1016/j.bbadis.2015.09.018
Lechevalier, M. P., and Lechevalier, H. (1970). Chemical composition as a criterion in the classification of aerobic actinomycetes. Int. J. Syst. Evol. Microbiol. 20, 435–443. doi: 10.1099/00207713-20-4-435
Lee, J. Y., Lee, J. Y., Jung, H. W., and Hwang, B. K. (2005). Streptomyces koyangensis sp. nov., a novel actinomycete that produces 4-phenyl-3-butenoic acid. Int. J. Syst. Evol. Microbiol. 55, 257–262. doi: 10.1099/ijs.0.63168-0
Lee, L.-H., Azman, A.-S., Zainal, N., Eng, S.-K., Ab Mutalib, N.-S., Yin, W.-F., et al. (2014d). Microbacterium mangrovi sp. nov., an amylotytic actinobacterium isolated from mangrove forest soil. Int. J. Syst. Evol. Microbiol. 64, 3513–3519. doi: 10.1099/ijs.0.062414-0
Lee, L. H., Azman, A. S., Zainal, N., Eng, S. K., Fang, C. M., Hong, K., et al. (2014c). Novosphingobium malaysiense sp. nov. isolated from mangrove sediment. Int. J. Syst. Evol. Microbiol. 64, 1194–1201. doi: 10.1099/ijs.0.059014-0
Lee, L.-H., Cheah, Y.-K., Sidik, S. M., Ab Mutalib, N.-S., Tang, Y.-L., Lin, H.-P., et al. (2012). Molecular characterization of Antarctic actinobacteria and screening for antimicrobial metabolite production. World J. Microbiol. Biotech. 28, 2125–2137. doi: 10.1007/s11274-012-1018-1
Lee, L.-H., Zainal, N., Azman, A.-S., Ab Mutalib, N.-S., Hong, K., and Chan, K.-G. (2014e). Mumia flava gen. nov., sp. nov., an actinobacterium of the family Nocardioidaceae. Int. J. Syst. Evol. Microbiol. 64, 1461–1467. doi: 10.1099/ijs.0.058701-0
Lee, L.-H., Zainal, N., Azman, A.-S., Eng, S.-K., Ab Mutalib, N.-S., Yin, W.-F., et al. (2014b). Streptomyces pluripotens sp. nov., a bacteriocin-producing streptomycete that inhibits meticillin-resistant Staphylococcus aureus. Int. J. Syst. Evol. Microbiol. 64, 3297–3306. doi: 10.1099/ijs.0.065045-0
Lee, L. H., Zainal, N., Azman, A. S., Eng, S. K., Goh, B. H., Yin, W. F., et al. (2014a). Diversity and antimicrobial activities of actinobacteria isolated from tropical mangrove sediments in Malaysia. Sci. World J. 2014:698178. doi: 10.1155/2014/698178
Leiros, M., Alonso, E., Sanchez, J. A., Rateb, M. E., Ebel, R., Houssen, W. E., et al. (2013). Mitigation of ROS insults by Streptomyces secondary metabolites in primary cortical neurons. ACS Chem. Neurosci. 5, 71–80. doi: 10.1021/cn4001878
Leszek, J., E., Barreto, G. E., Gasiorowski, K., and Koutsouraki, E., Aliev, G. (2016). Inflammatory mechanisms and oxidative stress as key factors responsible for progression of neurodegeneration: role of brain innate immune system. CNS Neurol. Dis. Drug Targets 15, 329–336. doi: 10.2174/1871527315666160202125914
Lin, M. T., and Beal, M. F. (2006). Mitochondrial dysfunction and oxidative stress in neurodegenerative diseases. Nature 443, 787–795. doi: 10.1038/nature05292
Liu, P., Huang, M., Song, S., Hayat, K., Zhang, X., Xia, S., et al. (2012). Sensory characteristics and antioxidant activities of Maillard reaction products from soy protein hydrolysates with different molecular weight distribution. Food Bioproc. Tech. 5, 1775–1789. doi: 10.1007/s11947-010-0440-3
Macfaddin, J. (2000). Biochemical Tests for Identification of Medical Bacteria. Philadelphia, PA: Lippincott Williams and Wilkins.
Manivasagan, P., Venkatesan, J., Sivakumar, K., and Kim, S. K. (2013). Production, characterization and antioxidant potential of protease from Streptomyces sp. MAB18 using poultry wastes. BioMed. Res. Int. 2013:496586. doi: 10.1155/2013/496586
Meena, B., Rajan, L. A., Vinithkumar, N. V., and Kirubagaran, R. (2013). Novel marine actinobacteria from emerald Andaman & Nicobar Islands: a prospective source for industrial and pharmaceutical byproducts. BMC Microbiol. 13:145. doi: 10.1186/1471-2180-13-145
Mesbah, M., Premachandran, U., and Whitman, W. B. (1989). Precise measurement of the G+ C content of deoxyribonucleic acid by high-performance liquid chromatography. Int. J. Syst. Bacteriol. 39, 159–167. doi: 10.1099/00207713-39-2-159
Miser-Salihoglu, E., Akaydin, G., Caliskan-Can, E., and Yardim-Akaydin, S. (2013). Evalution of antioxidant activity of various herbal folk medicines. J. Nutri. Food Sci. 3:222. doi: 10.4172/2155-9600.1000222
Narendhran, S., Rajiv, P., Vanathi, P., and Sivaraj, R. (2014). Spectroscopic analysis of bioactive compounds from Streptomyces cavouresis KU-V39: evaluation of antioxidant and cytotoxicity activity. Int. J. Pharm. Pharmaceut. Sci. 6, 319–322. Available online at: http://innovareacademics.in/journals/index.php/ijpps/article/view/2009
Penesyan, A., Kjelleberg, S., and Egan, S. (2010). Development of novel drugs from marine surface associated microorganisms. Mar. Drugs 8, 438–459. doi: 10.3390/md8030438
Pollak, F. C., and Berger, R. G. (1996). Geosmin and related volatiles in bioreactor-cultured Streptomyces citreus CBS 109.60. Appl. Environ. Microbiol. 62, 1295–1299.
Pongsetkul, J., Benjakul, S., Sampavapol, P., Osako, K., and Faithong, N. (2015). Chemical compositions, sensory and antioxidative properties of salted shrimp paste (Ka-pi) in Thailand. Int. Food Res. J. 22, 155–166. doi: 10.1007/s40071-014-0076-4
Premkumar, T., and Govindarajan, S. (2005). Antimicrobial study of pyrazine, pyrazole and imidazole carboxylic acids and their hydrazinium salts. World J. Microbiol. Biotech. 21, 479–480. doi: 10.1007/s11274-004-2041-7
Radi, E., Formichi, P., Battisti, C., and Federico, A. (2014). Apoptosis and oxidative stress in neurodegenerative diseases. JAD 42, S125–S152. doi: 10.3233/JAD-132738
Saitou, N., and Nei, M. (1987). The neighbor-joining method: a new method for reconstructing phylogenetic trees. Mol. Biol. Evol. 4, 406–425.
Sasser, M. (1990). Identification of Bacteria by Gas Chromatography of Cellular Fatty Acids. Newark, DE: DEMIDI Inc. MIDI Technical Note 101.
Schumann, P. (2011). 5- Peptidoglycan structure. Met. Microbiol. 38, 101–129. doi: 10.1016/b978-0-12-387730-7.00005-x
Ser, H.-L., Palanisamy, U. D., Yin, W.-F., Abd Malek, S. N., Chan, K.-G., Goh, B.-H., et al. (2015a). Presence of antioxidative agent, Pyrrolo[1,2-a]pyrazine-1,4-dione,hexahydro- in newly isolated Streptomyces mangrovisoli sp. nov. Front. Microbiol. 6:854. doi: 10.3389/fmicb.2015.00854
Ser, H.-L., Zainal, N., Palanisamy, U. D., Goh, B.-H., Yin, W.-F., Chan, K.-G., et al. (2015b). Streptomyces gilvigriseus sp. nov., a novel actinobacterium isolated from mangrove forest soil. Antonie van Leeuwenhoek 107, 1369–1378. doi: 10.1007/s10482-015-0431-5
Ser, H.-L., Ab Mutalib, N.-S., Yin, W.-F., Chan, K.-G., Goh, B. H., and Lee, L. H. (2015c). Evaluation of antioxidative and cytotoxic activities of Streptomyces pluripotens MUSC 137 isolated from mangrove soil in Malaysia. Front. Microbiol. 6:1398. doi: 10.3389/fmicb.2015.01398
Ser, H.-L., Law, J. W.-F., Chaiyakunapruk, N., Jacob, S. A., Palanisamy, U. D., Chan, K.-G., et al. (2016a). Fermentation conditions that affect clavulanic acid production in Streptomyces clavuligerus: a systematic review. Front. Microbiol. 7:522. doi: 10.3389/fmicb.2016.00522
Ser, H.-L., Palanisamy, U. D., Yin, W.-F., Chan, K.-G., Goh, B.-H., and Lee, L.-H. (2016b). Streptomyces malaysiense sp. nov.: a novel Malaysian mangrove soil actinobacterium with antioxidative activity and cytotoxic potential against human cancer cell lines. Sci. Rep. 6:24247. doi: 10.1038/srep24247
Shirling, E. B., and Gottlieb, D. (1966). Methods for characterization of Streptomyces species. Int. J. Syst. Evol. Microbiol. 16, 313–340. doi: 10.1099/00207713-16-3-313
Soobrattee, M. A., Neergheen, V. S., Luximon-Ramma, A., Aruoma, O. I., and Bahorun, T. (2005). Phenolics as potential antioxidant therapeutic agents: mechanism and actions. Mut. Res./Fund. Mol. Mech. Mutagen. 579, 200–213. doi: 10.1016/j.mrfmmm.2005.03.023
Staneck, J. L., and Roberts, G. D. (1974). Simplified approach to identification of aerobic actinomycetes by thin-layer chromatography. Appl. Microbiol. 28, 226–231.
Suematsu, N., Hosoda, M., and Fujimori, K. (2011). Protective effects of quercetin against hydrogen peroxide-induced apoptosis in human neuronal SH-SY5Y cells. Neurosci. Lett. 504, 223–227. doi: 10.1016/j.neulet.2011.09.028
Sui, J.-L., Xu, X.-X., Qu, Z., Wang, H.-L., Lin, H.-P., Xie, Q.-Y., et al. (2011). Streptomyces sanyensis sp. nov., isolated from mangrove sediment. Int. J. Syst. Evol. Microbiol. 61, 1632–1637. doi: 10.1099/ijs.0.023515-0
Sun, X.-S., Li, W.-H., Li, R., Gao, C.-Q., and Cui, G.-Y. (2013). Antioxidative activity of sulfide and pyrazine compositions in sesame-flavor liquor. Liquor Making 40, 57–60.
Supriady, H., Kamarudin, M. N. A., Chan, C. K., Goh, B. H., and Kadir, H. A. (2015). SMEAF attenuates the production of pro-inflammatory mediators through the inactivation of Akt-dependent NF-κB, p38 and ERK1/2 pathways in LPS-stimulated BV-2 microglial cells. J. Func. Foods 17, 434–448. doi: 10.1016/j.jff.2015.05.042
Takahashi, Y., Matsumoto, A., Seino, A., Iwai, Y., and Omura, S. (1996). Rare actinomycetes isolated from desert soils. Actinomycetologica 10, 91–97. doi: 10.3209/saj.10_91
Tamura, K., Stecher, G., Peterson, D., Filipski, A., and Kumar, S. (2013). MEGA6: molecular evolutionary genetics analysis version 6.0. Mol. Biol. Evol. 30, 2725–2729. doi: 10.1093/molbev/mst197
Tan, L. T. H., Chan, K. G., Lee, L. H., and Goh, B. H. (2016). Streptomyces bacteria as potential probiotics in aquaculture. Front. Microbiol. 7:79. doi: 10.3389/fmicb.2016.00079
Tan, L. T. H., Ser, H. L., Yin, W. F., Chan, K. G., Lee, L. H., and Goh, B. H. (2015). Investigation of antioxidative and anticancer potentials of Streptomyces sp. MUM256 isolated from Malaysia mangrove soil. Front. Microbiol. 6:1316. doi: 10.3389/fmicb.2015.01316
Thompson, J. D., Gibson, T. J., Plewniak, F., Jeanmougin, F., and Higgins, D. G. (1997). The CLUSTAL_X windows interface: flexible strategies for multiple sequence alignment aided by quality analysis tools. Nucleic Acids Res. 25, 4876–4882. doi: 10.1093/nar/25.24.4876
Triana-Vidal, L. E., and Carvajal-Varona, S. M. (2013). Protective effect of galantamine against oxidative damage using human lymphocytes: a novel in vitro model. Arch. Med. Res. 44, 85–92. doi: 10.1016/j.arcmed.2013.01.001
Uttara, B., Singh, A. V., Zamboni, P., and Mahajan, R. T. (2009). Oxidative stress and neurodegenerative diseases: a review of upstream and downstream antioxidant therapeutic options. Curr. Neuropharmacol. 7, 65. doi: 10.2174/157015909787602823
Versalovic, J., Koeuth, T., and Lupski, J. R. (1991). Distribution of repetitive DNA sequences in eubacteria and application to fingerprinting of bacterial genomes. Nucleic Acids Res. 19, 6823–6831. doi: 10.1093/nar/19.24.6823
Waksman, S. A., and Henrici, A. T. (1943). The nomenclature and classification of the actinomycetes. J. Bacteriol. 46, 337–341.
Wang, C., Wang, Z., Qiao, X., Li, Z., Li, F., Chen, M., et al. (2013). Antifungal activity of volatile organic compounds from Streptomyces alboflavus TD-1. FEMS Microbiol. Lett. 341, 45–51. doi: 10.1111/1574-6968.12088
Wayne, L. G., Brenner, D. J., Colwell, R. R., Grimont, P. A. D., Kandler, O., Krichevsky, M. I., et al. (1987). Report of the ad hoc committee on reconciliation of approaches to bacterial systematics. Int. J. Syst. Bacteriol. 37, 463–464. doi: 10.1099/00207713-37-4-463
Whiton, R. S., Lau, P., Morgan, S. L., Gilbart, J., and Fox, A. (1985). Modifications in the alditol acetate method for analysis of muramic acid and other neutral and amino sugars by capillary gas chromatography-mass spectrometry with selected ion monitoring. J. Chromato. A 347, 109–120. doi: 10.1016/S0021-9673(01)95474-3
Whittemore, E. R., Loo, D. T., Watt, J. A., and Cotmans, C. W. (1995). A detailed analysis of hydrogen peroxide-induced cell death in primary neuronal culture. Neuroscience 67, 921–932. doi: 10.1016/0306-4522(95)00108-U
Williams, P. G. (2009). Panning for chemical gold: marine bacteria as a source of new therapeutics. Trends Biotech. 27, 45–52. doi: 10.1016/j.tibtech.2008.10.005
Williams, S. T., Goodfellow, M., and Alderson, G. (1989). “Genus Streptomyces Waksman and Henrici 1943, 339AL,” in: Bergey's Manual of Systematic Bacteriology, eds S. T. Williams, M. E. Sharpe, J. G. Holt (Baltimore: Williams & Wilkins), 2452–2492
Wong, D. Z. H., Kadir, H. A., Lee, C. L., and Goh, B. H. (2012). Neuroprotective properties of Loranthus parasiticus aqueous fraction against oxidative stress-induced damage in NG108-15 cells. J. Nat. Med. 66, 544–551. doi: 10.1007/s11418-011-0622-y
Xu, C.-P., Liu, Y., Zhao, S., Zeng, Y., and Liu, S. (2015). Chemical composition and in vitro antioxidant activity of the essential oil of Rehmannia Glutinosa libosch using ultrasound as a pretreatment. J. Biol. Active Prod. from Nat. 5, 276–282. doi: 10.1080/22311866.2015.1073628
Keywords: Streptomyces antioxidans, actinobacteria, mangrove, neuroprotective, antioxidative
Citation: Ser H-L, Tan LT-H, Palanisamy UD, Abd Malek SN, Yin W-F, Chan K-G, Goh B-H and Lee L-H (2016) Streptomyces antioxidans sp. nov., a Novel Mangrove Soil Actinobacterium with Antioxidative and Neuroprotective Potentials. Front. Microbiol. 7:899. doi: 10.3389/fmicb.2016.00899
Received: 15 March 2016; Accepted: 26 May 2016;
Published: 16 June 2016.
Edited by:
Yuji Morita, Aichi Gakuin University, JapanReviewed by:
Jem Stach, University of Newcastle, UKHuda Mahmoud Mahmoud, Kuwait University, Kuwait
Janice Lorraine Strap, University of Ontario Institute of Technology, Canada
Copyright © 2016 Ser, Tan, Palanisamy, Abd Malek, Yin, Chan, Goh and Lee. This is an open-access article distributed under the terms of the Creative Commons Attribution License (CC BY). The use, distribution or reproduction in other forums is permitted, provided the original author(s) or licensor are credited and that the original publication in this journal is cited, in accordance with accepted academic practice. No use, distribution or reproduction is permitted which does not comply with these terms.
*Correspondence: Bey-Hing Goh, goh.bey.hing@monash.edu;
Learn-Han Lee, lee.learn.han@monash.edu; leelearnhan@yahoo.com