- 1WHO Collaborating Centre for Reference and Research on Influenza, Victorian Infectious Diseases Reference Laboratory, Peter Doherty Institute for Infection and Immunity, Melbourne, VIC, Australia
- 2School of Applied and Biomedical Sciences, Federation University Australia, Gippsland, VIC, Australia
- 3Melbourne School of Population and Global Health, University of Melbourne, Parkville, VIC, Australia
The concern of the emergence of a pandemic influenza virus has sparked an increased effort toward the development and testing of novel influenza antivirals. Central to this is the animal model of influenza infection, which has played an important role in understanding treatment effectiveness and the effect of antivirals on host immune responses. Among the different animal models of influenza, ferrets can be considered the most suitable for antiviral studies as they display most of the human-like symptoms following influenza infections, they can be infected with human influenza virus without prior viral adaptation and have the ability to transmit influenza virus efficiently between one another. However, an accurate assessment of the effectiveness of an antiviral treatment in ferrets is dependent on three major experimental considerations encompassing firstly, the volume and titer of virus, and the route of viral inoculation. Secondly, the route and dose of drug administration, and lastly, the different methods used to assess clinical symptoms, viral shedding kinetics and host immune responses in the ferrets. A good understanding of these areas is necessary to achieve data that can accurately inform the human use of influenza antivirals. In this review, we discuss the current progress and the challenges faced in these three major areas when using the ferret model to measure influenza antiviral effectiveness.
Influenza – the Disease
Influenza is a highly contagious respiratory disease causing symptoms ranging from headache, myalgia, malaise, sore throat, sneezing, and nasal discharge (Cox and Subbarao, 1999). Influenza virus is transmitted via virus-laden secretions propelled by coughing or sneezing from an infected person. Most influenza infections are self-limiting, lasting for one to 5 days but host factors such as age, pregnancy, and underlying medical conditions can increase the severity of illness (Gavin and Thomson, 2003). Influenza causes high global mortality and morbidity annually, with United States alone experiencing approximately 95,000–172,000 hospitalizations and 21,000–41,000 deaths annually (Lowen et al., 2006). The morbidity associated with seasonal influenza has a significant economic impact due to work absenteeism and puts huge pressure on the public health system.
Influenza Antivirals
To date, the neuraminidase inhibitors (NAIs) are the only licensed class of antiviral drugs effective against currently circulating influenza viruses. Zanamivir (RelenzaTM) and oseltamivir (TamifluTM) have been licensed since 1999 while newer NAIs, such as peramivir (RapivabTM) and laninamivir (InavirTM), are approved in Japan, and in the case of peramivir also in South Korea, USA, and China (Ikematsu and Kawai, 2011; Chairat et al., 2013; ChinaBioToday, 2013). However, the therapeutic and prophylactic efficacy of NAIs against ‘seasonal’ influenza infection remains hotly debated (Jefferson et al., 2014). The continuous risks posed by the emergence of NAI-resistant viruses (Takashita et al., 2015) and the pandemic potential of avian influenza viruses, such as A(H5N1) (Nguyen et al., 2013) and A(H7N9) (Hu et al., 2013), has sparked a major effort to develop new antivirals for human use.
Typically, investigational antivirals will first undergo in vitro efficacy screening, followed by in vivo testing in animal models to look at pharmacokinetics/pharmacodynamics (PK/PD), drug toxicity and drug effectiveness prior to clinical trials. As such, the choice of the animal model for assessing the effectiveness of these influenza antivirals becomes critical as it provides pre-clinical data that can inform the decision for progression toward clinical trials. Currently, there are a large number of influenza antivirals undergoing clinical trials, a substantial increase from the limited trials in 2000 (Figure 1). In the majority of human clinical trials of influenza antivirals, the primary endpoint used to assess the drug efficacy is the time to alleviation of clinical symptoms, such as cough, fever, sore throat, myalgia, lethargy, nasal congestion, and headaches, whereas other aspects, including the ability to reduce viral shedding, are considered secondary endpoints (Hayden et al., 1997; The MIST, 1998; Makela et al., 2000; Nicholson et al., 2000; Treanor et al., 2000; Haffizulla et al., 2014).
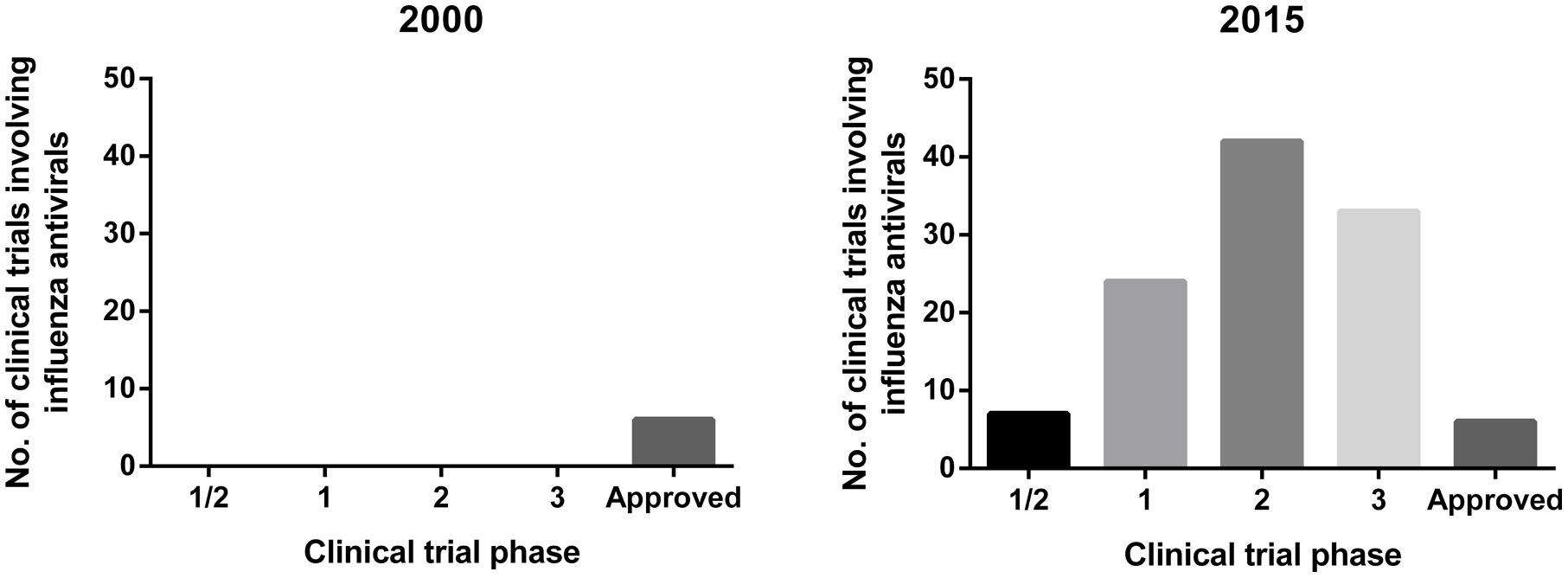
FIGURE 1. Overview of clinical trials of influenza antivirals in year 2000 and 2015. Data for 2015 extracted from clinicaltrials.gov (ClinicalTrials, 2015) using search terms: ‘Influenza’ and ‘antivirals’ and ‘antivirals treatment’.
Animal Models in Influenza Research
Animal models of influenza infection have played an important role in the understanding of viral pathogenicity and have served as pre-clinical models for the evaluation of vaccine candidates and new therapeutics (Kiso et al., 2010; Margine and Krammer, 2014; Marjuki et al., 2014). To date, there are many different animal models of influenza infection, namely ferrets, mice, guinea pigs, swine, non-human primates (NHP), and more recently, zebrafish (Gabor et al., 2014). The pros and cons of the different animal models of influenza to investigate disease pathogenesis, transmission, and vaccine development have been well-described in several published reviews and are summarized here in Table 1 (Bouvier and Lowen, 2010; Lowen et al., 2014; Margine and Krammer, 2014; Thangavel and Bouvier, 2014; Davis et al., 2015; Enkirch and von Messling, 2015).
Animal Models in Influenza Antiviral Studies
Among all animal experimental models, mice are most commonly used for testing influenza antivirals mainly due to factors, such as lower experimental cost, ease of animal handling and the ability to use large numbers of animals to attain statistical power in a single experiment (Ryan et al., 1994; Mendel et al., 1998; Triana-Baltzer et al., 2009; Kiso et al., 2010; Bantia et al., 2011; Smee et al., 2012b; Zarogiannis et al., 2012; Marjuki et al., 2014). To date, weight loss, mortality (lethal model) and virus titer are the commonly used determinants of antiviral drug effectiveness in mice studies. Although these measurements are informative, the usefulness of mice in antiviral studies has been largely limited by the lack of clinical symptoms following influenza infection. The absence of clinical symptoms such as fever, sneezing, nasal discharge, and nasal inflammation in mice following influenza infection limits the extrapolation of mouse data to the human scenario where alleviation of symptoms are considered as the primary endpoint in clinical trials (Table 1). In contrast, the ferret is the only animal model which displays comparable clinical symptoms to that of humans following influenza infection (Table 1). In view of these factors, in this review we will discuss the current progress, limitations and the future directions of using ferrets to assess antiviral effectiveness against influenza infections.
Ferret
Since the discovery of the susceptibility of ferrets (Mustela putorius furo) to influenza virus in the 1930’s (Smith et al., 1933), they remain one of the best animal models of influenza infection as they exhibit many of the clinical symptoms observed in humans following influenza infection, can be directly infected with human influenza virus without prior viral adaptation, and have the ability to transmit influenza virus efficiently between one another (Table 1). The susceptibility of ferrets to human influenza viruses is due to the presence of α2-6-linked terminal N-acetylneuraminic sialic acids (Neu5AC) in their respiratory tract which facilitates virus binding and the initiation of viral replication (Jia et al., 2014; Ng et al., 2014).
However, the use of ferrets for influenza studies has been limited by factors such as animal availability, genetic heterogeneity (out-bred) (Margine and Krammer, 2014), the requirement of a complex husbandry facility and caging system (Mabry et al., 2013; Enkirch and von Messling, 2015), and a lack of immunological reagents and genetically modified mutants for immunological investigation (Margine and Krammer, 2014; Enkirch and von Messling, 2015). As a consequence, ferret experiments can be limited by small sample sizes (n ≤ 5) (Belser et al., 2013b; Nishiura et al., 2013; Buhnerkempe et al., 2015), where large animal-to-animal variability has resulted in the detection of non-statistically significant trends of antiviral effectiveness between the treatment groups in variables, such as weight, temperature, nasal inflammation, and virus titer (Rowe et al., 2010; Govorkova et al., 2011; Oh et al., 2014, 2015). Ideally, a larger number of ferrets should be used but limitations, such as high experimental cost, low animal availability, limited caging capacity and ethical constraint, typically restricts most studies to group sizes of five or less ferrets. Unlike the more commonly used animal models, such as rodents and guinea pigs, the use of larger animals for experimentation, such as ferrets, can be met with greater scrutiny by animal ethics governing bodies on aspects, such as the choice of animal, animal numbers and husbandry concerns. Only a small number of countries or regions (such as USA, UK, and Europe) have guidelines regarding the husbandry of ferrets for animal experimentation. Therefore in the absence of any local directives, animal ethics committees may rely on guidelines provided by other countries, particularly where the committee has little experience in the use of ferrets.
Despite these limitations, the use of ferrets in influenza research has increased considerably since 2008 (Figure 2). This increment was largely attributed to a global effort to better understand viruses with pandemic potential such as the avian influenza viruses A(H5N1) and A(H7N9), and the virus responsible for the 2009 pandemic, the A(H1N1)pdm09 virus (Govorkova et al., 2005; Yen et al., 2007; Boltz et al., 2008; Itoh et al., 2009; Maines et al., 2009; Munster et al., 2009; Belser et al., 2013a; Richard et al., 2013; Zhang et al., 2013). With the recent publication of the ferret genome (Peng et al., 2014), it is likely that the use of the ferret as a model of influenza will continue to rise.
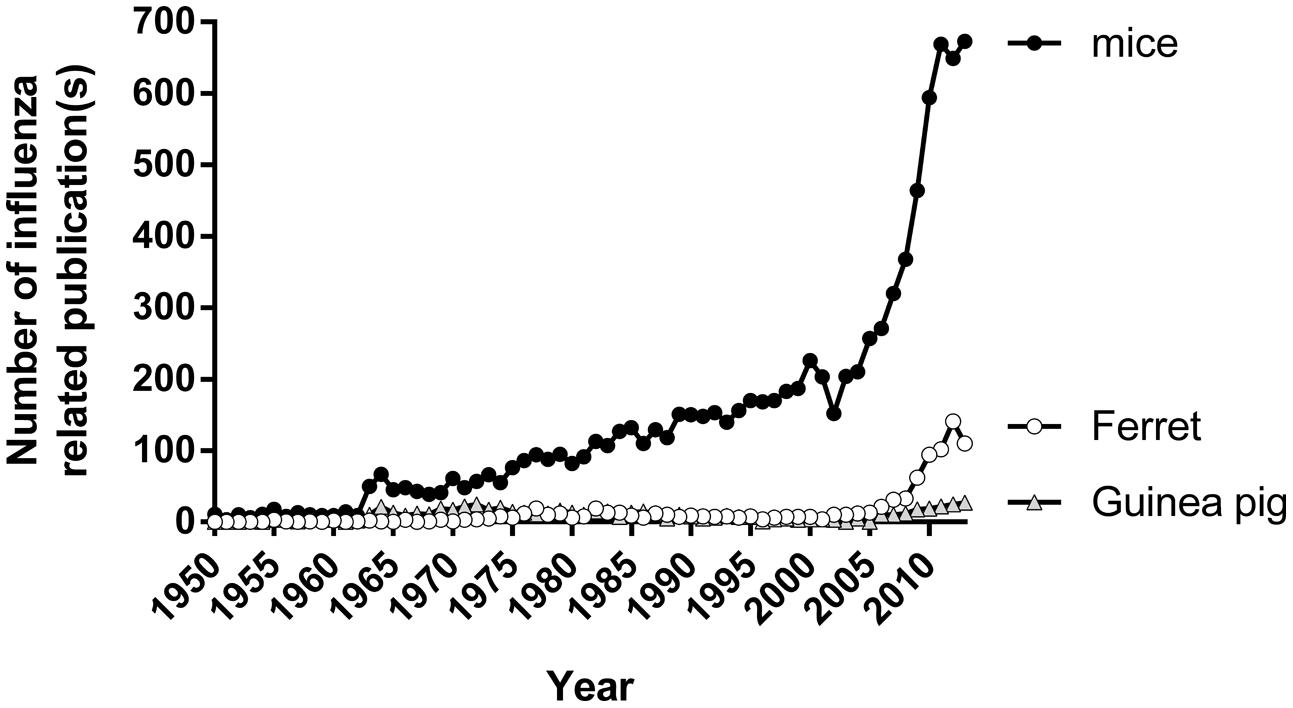
FIGURE 2. The number of publications using different animal models of influenza. Number of papers published on topics relating to influenza and mice/ferret/guinea pig from 1950 to 2013. Data tabulated by online automated yearly statistics of PubMed results (http://dan.corlan.net/medline-trend.html). Search terms used are ‘Influenza’ and ‘Mice/ferret/guinea pig.’
To date, the ferret model has been used to investigate viral susceptibility and transmission (Yen et al., 2007; Maines et al., 2009; Zhang et al., 2013), and as a preclinical model to investigate poorly understood areas such as immunological responses to influenza in young children (newly weaned ferrets) (Huang et al., 2012), impaired immunity in older individuals (aged ferrets) (Paquette et al., 2014) and the treatment effectiveness of different antivirals to reduce influenza infection (Boltz et al., 2008; Kubo et al., 2010; Govorkova et al., 2011; Kitano et al., 2011; Marriott et al., 2014). Recently, the use of ferrets in influenza antiviral studies has extended to treatment effectiveness in an immunocompromised setting (van der Vries et al., 2013), the effectiveness of antiviral treatment or prophylaxis in preventing infections in secondary contacts (Oh et al., 2014) and the transmission of influenza between an infant and mother during breast-feeding (Paquette et al., 2015).
There are a large number of experimental variables in a typical ferret antiviral effectiveness study that can alter the study outcome and that should be carefully considered to ensure that the most reliable data is generated. These variables are discussed in detail below and are summarized in Figure 3.
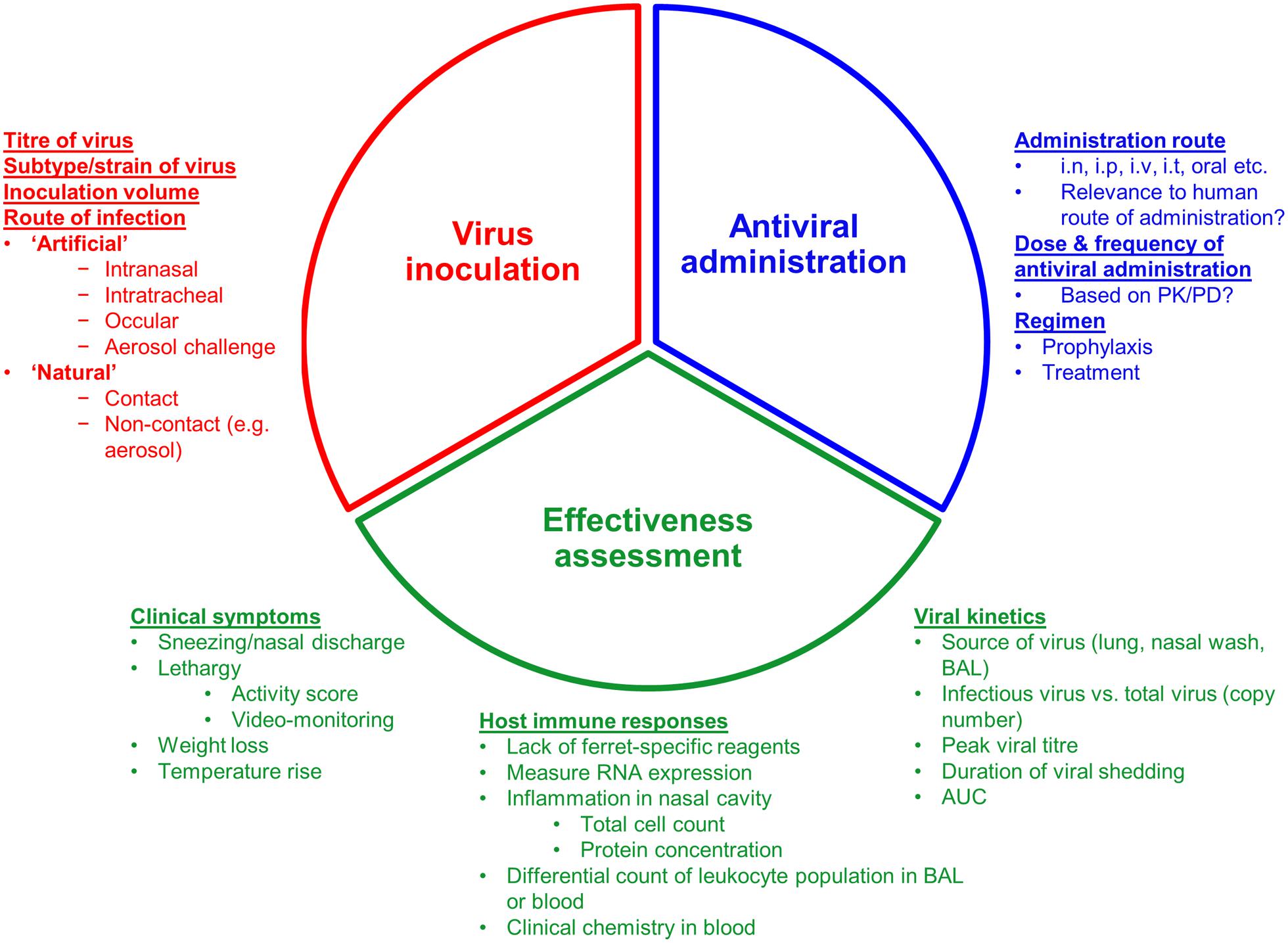
FIGURE 3. Overview of the three experimental considerations when assessing an antiviral in a ferret model of influenza infection. i.n: Intranasal; i.p, intraperitoneal; i.v, intravenous; i.t, intratracheal; PK/PD, pharmacokinetics/pharmacodynamics; BAL, bronchoalveolar lavage; AUC, area under curve.
Inoculation of Influenza Virus in Ferrets
The initiation of influenza infection in animal experimental models, and even in the human challenge model (Darton et al., 2015), is routinely carried out by instilling virus via the intranasal route. In general, intranasal instillation of virus is considered to be a simple procedure (Turner et al., 2011) compared to intratracheal (Kreijtz et al., 2013; van der Vries et al., 2013) or ocular (Belser et al., 2014), by which ferrets can also be infected in a reproducible manner. However, the decision on which inoculation route to initiate infection can be dependent on the virus strain to be tested. For avian influenza viruses, such as A(H5N1) and A(H7N9), intratracheal instillation is preferred over direct inoculation into the lower respiratory tract of ferrets, as it is found to induce a more severe pneumonia that more closely resembles pathogenesis observed in humans, compared to intranasal inoculation which causes mild to moderate pneumonia in ferrets (Bodewes et al., 2011; Kreijtz et al., 2013). For human seasonal viruses, such as influenza A(H1N1)pdm09, A(H3N2), and B viruses, intratrachel instillation has been used in only a small number of studies (van der Vries et al., 2013). Instead, ferrets can be effectively infected by intranasal instillation (Huang et al., 2011). However, the clinical severity of influenza infection via intranasal instillation is highly dependent on the volume of inoculum (Bodewes et al., 2011; Moore et al., 2014). A larger volume of inoculum (1 mL) results in the delivery of virus down into lower respiratory tract and into the lungs compared to smaller volumes (0.2 mL and 0.5 mL) which are primarily retained in the upper respiratory tract (Moore et al., 2014). As a result, ferrets intranasally infected with the larger volumes of inoculum have a more severe illness and lung histopathology compared to those infected with a smaller volume (0.2 mL) even when the same infectious titer of virus was used (Moore et al., 2014). These finding not only underscored influenza strains, inoculum route and volume to be important considerations in the ferret model of influenza infection but also highlighted the difficulties in interpreting data across studies using different inoculation protocols.
The alternatives to intranasal infection are the use of a ‘natural’ infection, that can be achieved by either contact (Roberts et al., 2012; Oh et al., 2014) or non-contact transmission (Hamelin et al., 2011; van der Vries et al., 2011; Herfst et al., 2012) of virus by exposing a naïve ferret to an infected ferret in the same or adjacent cage, or aerosolized challenge (Gustin et al., 2011). Despite ‘natural infection’ being a more clinically relevant route of transmission, few studies have used this methodology, presumably due to the additional experimental time involved, additional ferret use, the requirement for larger cages to house multiple animals for contact transmission or a more complex caging system for non-contact transmission, and the lack of transmissibility of influenza viruses, such as avian influenza A(H5N1) and A(H7N9) (Yen et al., 2007; Belser et al., 2013a) and influenza B virus (Kim et al., 2015), via the aerosol and/or contact route. Nevertheless, ‘natural’ infection has the clear benefit of infecting ferrets with a realistic infectious viral dose and results in viral replication kinetics that better mimic those of ‘natural’ influenza infections in humans (Figure 4). Unlike ‘natural’ infection, which relies on infected donor ferrets to transmit viruses to naïve recipients, a more standardized alternative is aerosol challenge which involves exposing naïve ferrets directly to known doses of aerosolized influenza viruses delivered by specific devices. Ferrets exposed to low aerosolized viral dose (1.5–2.2 FID50) have been shown to exhibit similar viral shedding kinetics and disease pathogenesis to those infected via ‘natural’ infection (Gustin et al., 2011). In contrast to ‘natural’ infection, aerosol challenges eliminates the need for donor ferrets, allows ferrets to be infected with viruses with poor aerosol transmissibility, and enables the manipulation of variables, such as dose and timing of viral exposure, to achieve a more standardized way of infecting ferrets in a ‘natural’ fashion. However, few studies have reported using this methodology presumably due to the high cost involved in requiring specialized aerosol system and cages for such inoculation protocol.
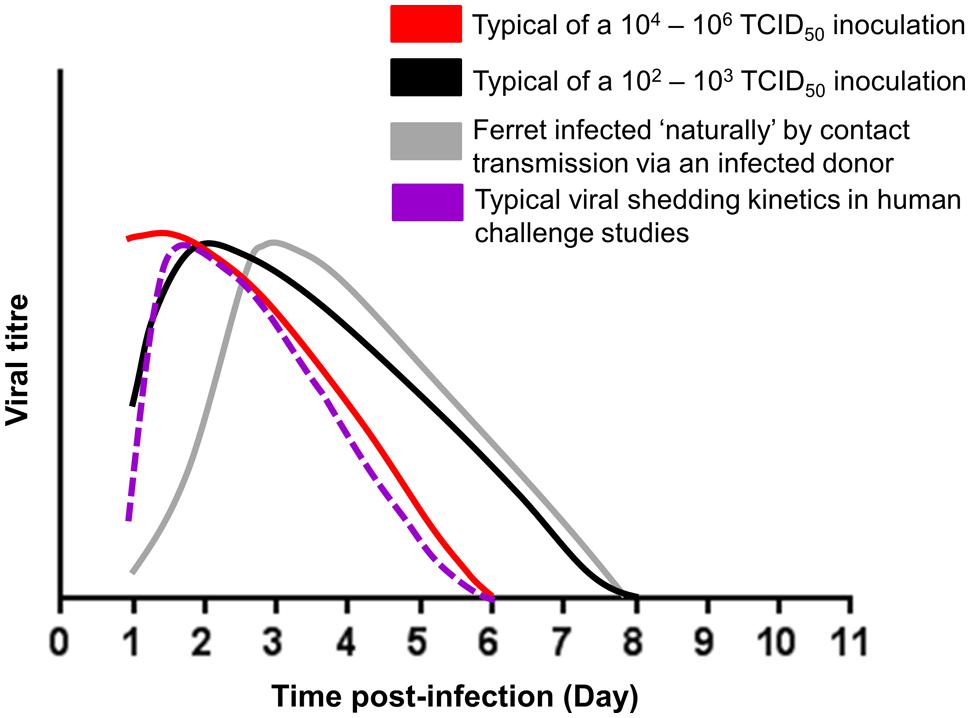
FIGURE 4. Schematic representation of the viral shedding kinetics of intranasally infected (102–103, 104–106 TCID50 viral inoculum) ferrets, ferrets naturally infected by contact transmission via an infected donor and intranasally infected human in a challenge model (Hayden et al., 1999; Baccam et al., 2006). Ferret viral data were adapted from published studies (McBrayer et al., 2010; Smith et al., 2011; Stark et al., 2013; Oh et al., 2014, 2015).
The majority of ferret infection studies have used intranasal infections with inocula of high infectious viral titers (e.g., 106 TCID50/PFU/EID50 per animal) which may result in a very large number of infectious particles infecting the nasal epithelial in a short time period (Maines et al., 2009; Govorkova et al., 2011; Kim et al., 2013; Stark et al., 2013; Paquette et al., 2014; Marjuki et al., 2015). Ferrets infected with high viral inocula (104-6 TCID50/animal) can show viral shedding kinetics where titers peak as early as 1 day post-infection (Figure 4). In contrast, ferrets infected with a lower viral inocula (102-3 TCID50/animal) can show a more ‘typical’ viral shedding curve that more closely resembles a natural infection where viral titers gradually rise, peak and then fall (Baccam et al., 2006; Figure 4). Although high titer viral inoculums have been used to increase the chances of infection or increase pathogenicity, several studies have shown that the initial titer of the inoculum does not correlate to disease severity in ferrets (Marriott et al., 2014; Oh et al., 2015).
Influenza antivirals, such as NAIs, typically act by interrupting the viral replication cycle, whereas antibiotics directly eliminate and reduce the causative pathogen (McCullers, 2011). Therefore, the common practice of infecting ferrets with a high viral inoculum could overwhelm the host (ferret) with an unrealistically large number of infectious viral particles and under such experimental conditions, the effectiveness of an antiviral treatment could be undermined as the antivirals would not be able to contain such rapid onset of viral infection. As demonstrated by Marriott et al. (2014), oseltamivir treatment significantly lowered viral shedding, lowered inflammatory nasal cell count and improved activity levels following a 102 PFU/animal dose of infection, but had no significant effect on these parameters when a high viral inoculum of 106 PFU/animal was used (Marriott et al., 2014). Therefore, in the context of antiviral testing in ferrets and in particular to intranasal inoculation, careful consideration should be given to the amount of virus used, to prevent undermining the effectiveness of antivirals in influenza infection.
Drug Administration
Administration of therapeutics into animals requires the careful consideration of many factors including the drug pharmacology, concentration, volume, timing, frequency of dose and route of administration (Urso et al., 2002). The antivirals for influenza treatment that are currently approved or in late-phase clinical trials in humans encompass various routes of administration including oral, inhaled and intravenous (Table 2). Where possible the route of administration in animals should follow the same route of delivery as in humans so that the results can be better extrapolated to the findings in man (Turner et al., 2011; Table 2).
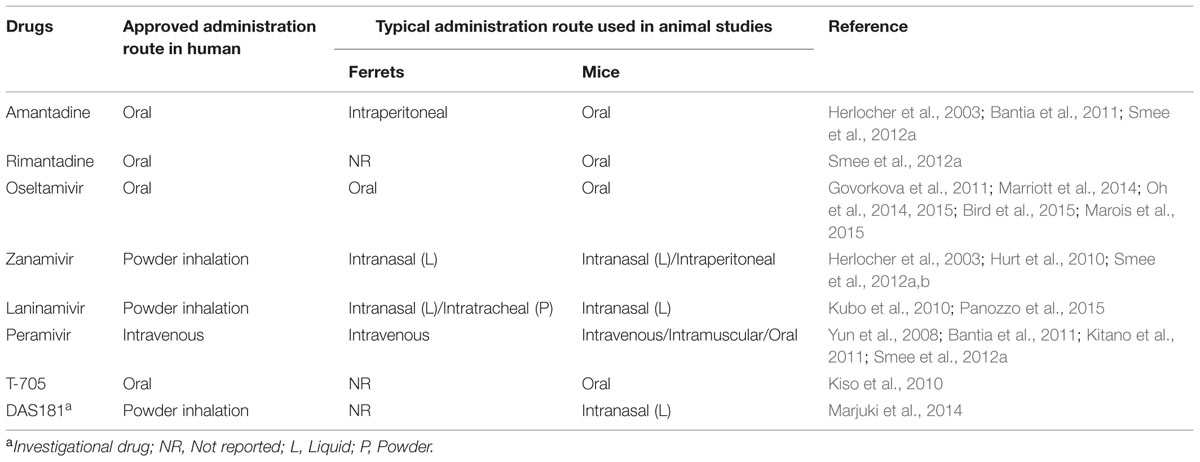
TABLE 2. The differences in antiviral drug administration route between human and animal model of influenza infection.
The delivery of parenterally administered antivirals, such as peramivir, in ferrets is relatively straight-forward and can be easily administered by intravenous injection (Yun et al., 2008; Kitano et al., 2011). Administering drugs via the enteral (oral) route in ferrets can be limited by many factors such as the requirement for multiple dosing regimens, poor drug solubility and palatability of the drug to animals. In ferrets, direct feeding of orally administered compounds is preferred over oral gavage (which is commonly used in mice) as it does not require anesthesia, which can impact on the animal’s health and behavior if given repeatedly, such as twice daily for 5 days. For oseltamivir administration, ferrets can be fed oseltamivir phosphate dissolved in sugar solution to achieve an accurate dosing (Oh et al., 2014, 2015). The drug in sugar solution is well-tolerated by ferrets allowing multiple dosing without the need for sedation, although higher doses of the drug (e.g., 25 mg/kg) are less readily swallowed than the standard 5 mg/kg dose. In contrast, oral antivirals with poor solubility, such as nitazoxanide (Haffizulla et al., 2014; Rossignol, 2014), pose difficulties when administering to ferrets, and therefore alternative methods, such as spiking the drug into food or in special ‘treats’, may be necessary (Turner et al., 2011).
The delivery of inhaled drugs, such as zanamivir and laninamivir, to ferrets poses additional challenges. Both zanamivir and laninamivir are delivered to humans as a dry powder formulation that is actively inhaled by the patient via specially designed inhalers (Chairat et al., 2013). Mimicking this type of administration in animals is difficult, and is presumably why the majority of animal studies investigating zanamivir (Herlocher et al., 2003; Hurt et al., 2010; Smee et al., 2012a,b) and laninamivir (Kubo et al., 2010) have dissolved the compounds in saline or water and administered intranasally (Table 2). While intranasal instillation of drugs is a simple technique that can be easily adopted by most laboratories, the relevance of delivering the drug in this manner when it is designed to be inhaled is questionable (Turner et al., 2011). In addition, it has been demonstrated in mice that intranasal instillation of drug administration can exacerbate viral infection leading to lower drug effectiveness (Smee et al., 2012b). Insufflators are devices that have been widely used as a non-invasive method to administer powdered drugs for drug deposition studies in small animals such as mice or rats (Nahar et al., 2013), but have been less commonly used in larger animals such as ferrets. To bridge this unmet need for a delivery system for powdered antivirals such as laninamivir, we recently characterized the usage of a dry powder insufflator to deliver laninamivir octanoate (LO) to ferrets prior to influenza infection (Panozzo et al., 2015). In vitro laser diffraction analysis showed that ∼80% LO together with its lactose carrier can be effectively discharged from the device and intratracheal administration of LO in anesthetized ferrets can be easily performed (Panozzo et al., 2015). For LO where a single dose is sufficient, the method is highly applicable, but repeated dosing would be difficult due to the need for regular anesthesia. The use of the insufflator device may be useful to investigate other inhaled drugs in ferrets, such as DAS181.
Drug Dosage
Besides route of administration, accurate evaluation of the effectiveness of antivirals in animals is also dependent on the dose of drug being administered. In humans, the optimal dose is determined by pharmacokinetic/pharmacodynamic (PK/PD) analysis. The PK/PD of influenza antivirals is often determined during pre-clinical testing in animals by pharmaceutical companies (Urso et al., 2002), but data from these studies is often not in the public domain. For example, the majority of studies have used 5 mg/kg oseltamivir phosphate in ferrets as being ‘equivalent’ to the 75 mg dose in humans, although limited publicly available PK/PD data is available to support this (Li et al., 1998; Mendel et al., 1998; Reddy et al., 2015). At the time of writing this review, we have found only a small number of PK studies of the influenza antivirals in animals such as mice, rats, and ferrets (Table 3). As the generation and analysis of PK/PD data can involve a large number of animals and requires specialized modeling expertise, it is not always a viable option for research laboratories to complete such studies. Therefore, if PK/PD data of approved drugs in ferrets or other animals was made publicly available in open access databases, then this would greatly assist and encourage the use of a consensus dose in an animal to allow a better assessment and comparison of the effectiveness of the drugs across different studies.
Parameters to Assess the Effectiveness of Antiviral Treatment
Pathology
One advantage of using the ferret is the ability to track viral shedding kinetics from both the upper and/or lower respiratory tract via nasal washing or lower bronchoalveolar lavage (BAL), although the latter is rarely used (Lee et al., 2014). In addition, ferrets can be monitored for clinical symptoms associated with human influenza infections including weight loss, rise in body temperature, sneezing, nasal discharge, and lethargy (Table 4). Whilst weight, temperature and symptoms such as sneezing and nasal discharge can be easily assessed, lethargy is more challenging to quantitate accurately. Conventionally, the activity of ferrets has been determined by visually assessing movement of the animal and assigning an arbitrary score (Reuman et al., 1989; Govorkova et al., 2011; Kim et al., 2013; Stark et al., 2013; Marriott et al., 2014; Oh et al., 2014). However, we have recently reported on the use of video-tracking methodology, which allows the activity level of ferrets to be quantitated by computer software analysis (Oh et al., 2015). Not only did video-tracking improve the sensitivity of detecting activity changes post-infection, it also enabled the assessment process to be simplified and less prone to bias. To date, many studies including ours have shown that treatment with an antiviral, such as oseltamivir, can improve the activity level of ferrets infected with influenza (Govorkova et al., 2011; Marriott et al., 2014; Oh et al., 2014, 2015). This improved methodology allows a more accurate assessment and extrapolation of the associated effect of antivirals on activity or ‘wellness’.
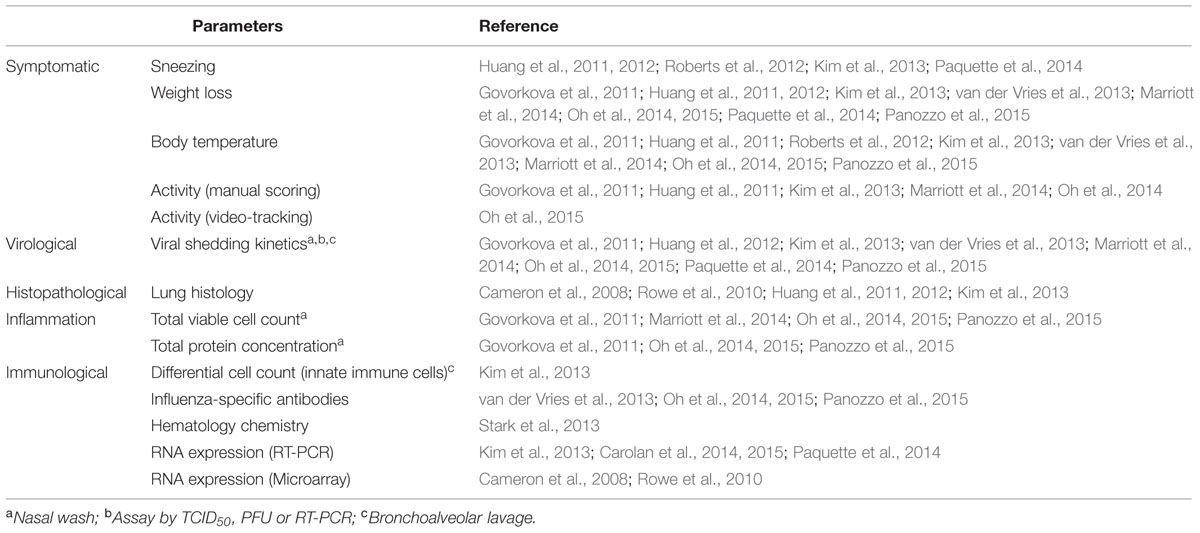
TABLE 4. Different types of clinical and symptomatic parameters measured in influenza studies in ferrets.
Immunology
The major disadvantage of using ferrets as a model for studying influenza (or influenza antiviral effectiveness) is the lack of validated ferret-specific reagents, such as antibodies, to study the immunology of disease pathogenesis. However, because of the significant benefits of the model many laboratories are now attempting to validate cross-reactive antibodies and develop novel reagents for ferret research (Rutigliano et al., 2008; Martel and Aasted, 2009; Music et al., 2014). One such collaborative effort is chaired by the United States Centre of Excellence for Influenza Research and Surveillance group at St. Jude Children’s Research Hospital in Memphis, USA. To date, several groups have assessed ferret host immune responses to influenza infection based on RNA expression by either quantitative real-time PCR in either nasal washes, blood or lung (Maines et al., 2012; Carolan et al., 2014, 2015; Paquette et al., 2014; Vidana et al., 2014), or by using a canine microarray platform (Cameron et al., 2008; Rowe et al., 2010; Table 4). Recently, the report of an annotated ferret transcriptome during influenza infection has also opened up an avenue for interrogating the global host gene expression to further understand host immunity toward influenza infection (Leon et al., 2013). In addition, simple immunological analysis such as measuring total cell count or protein concentration in nasal wash samples can also be useful as a determinant of inflammation (Govorkova et al., 2007, 2011; Marriott et al., 2014; Oh et al., 2015; Panozzo et al., 2015). Other basic immune measurements include differential staining of various leukocytes populations in the BAL or blood, and also clinical chemistry of different proteins in blood post influenza infection (Kim et al., 2013; Stark et al., 2013; Table 4).
Despite a great effort to study the interplay between influenza infection and immunity in ferrets, the effect of antivirals on immunity in this model is still poorly understood. To date, the majority of the immunity-related studies on influenza antivirals have used mice as the animal model (Burger et al., 2000; Wong et al., 2011; Bird et al., 2015; Marois et al., 2015). Oseltamivir was found to reduce innate cell populations in BAL, such as neutrophils and macrophages, when given prophylactically, and reduced pro-inflammatory cytokines when given pre- and post-infection (Wong et al., 2011). In contrast, the effect of oseltamivir on the adaptive immune cell population, such as cytotoxic CD8+ T cells, is still unclear. While Burger et al. (2000) reported that oseltamivir had minimal impact on the splenic population of T and B cells, and had no effect on the cytolytic activity of CD8+ T cells and natural killer cells in A(H1N1) influenza-infected mice (Burger et al., 2000), a recent study by Marois et al. (2015), found oseltamivir reduced circulating and tissue-resident effector and memory CD8+ T cells in A(H1N1) influenza-infected mice and significantly reduced the protective effect in a subsequent infection episode (Marois et al., 2015). In addition, a study by Bird et al. (2015) showed that although oseltamivir treatment reduced the number of CD8+ T cells in mice, they had normal recall responses and conferred protection against subsequent infection (Bird et al., 2015).
Future Directions for the Use of Ferrets to Assess Antiviral Effectiveness
To date, the ferret remains as one of the preferred models for assessing influenza infection and as such has become an important model for antiviral testing. Although the ferret poses many advantages compared to other animal models, this review has highlighted some of the challenges of using the ferret to assess antiviral effectiveness. Researchers conducting studies in the ferret model of influenza infection need to consider progressing toward a more ‘natural’ infection methodology by either contact or non-contact transmission, or aerosol challenge, using drug doses that are based on PK/PD analysis and to deliver the drugs via a relevant route (Figure 3). The development of more robust parameters to measure antiviral effectiveness in ferrets, such as computational analysis of behavior/activity and the development of ferret-specific reagents to explore the immunological effects of antiviral treatment, will both greatly improve our understanding of antiviral modes of action and the effect on viral pathogenesis. In our opinion, the application of ferrets in antiviral studies should not be limited to just understanding therapeutic effectiveness. Instead, the ferret model can be applied to address other questions such as the in vivo effectiveness of antivirals against drug ‘resistant’ viruses, and the effectiveness of different antiviral treatment or prophylaxis strategies on preventing or minimizing transmission. Therefore, we anticipate that with continuous development and refinement of the ferret model for influenza antiviral testing, it has the potential to provide more meaningful data to better inform human use of influenza antivirals.
Author Contributions
Both authors made substantial, direct and intellectual contribution to the work, and approved it for publication.
Funding
DO is supported by a National Health and Medical Research Grant, Australia (APP 1055793). The Melbourne WHO Collaborating Centre for Reference and Research on Influenza is supported by the Australian Government Department of Health.
Conflict of Interest Statement
The authors declare that the research was conducted in the absence of any commercial or financial relationships that could be construed as a potential conflict of interest.
Acknowledgment
We thank Chantal Baas for contributing ferret viral load data.
References
Baccam, P., Beauchemin, C., Macken, C. A., Hayden, F. G., and Perelson, A. S. (2006). Kinetics of influenza A virus infection in humans. J. Virol. 80, 7590–7599. doi: 10.1128/jvi.01623-05
Bantia, S., Kellogg, D., Parker, C., Upshaw, R., Ilyushina, N. A., and Babu, Y. S. (2011). A single intramuscular injection of neuraminidase inhibitor peramivir demonstrates antiviral activity against novel pandemic A/California/04/2009 (H1N1) influenza virus infection in mice. Antiviral Res. 90, 17–21. doi: 10.1016/j.antiviral.2011.02.001
Belser, J. A., Gustin, K. M., Katz, J. M., Maines, T. R., and Tumpey, T. M. (2014). Influenza virus infectivity and virulence following ocular-only aerosol inoculation of ferrets. J. Virol. 88, 9647–9654. doi: 10.1128/jvi.01067-14
Belser, J. A., Gustin, K. M., Pearce, M. B., Maines, T. R., Zeng, H., Pappas, C., et al. (2013a). Pathogenesis and transmission of avian influenza A (H7N9) virus in ferrets and mice. Nature 501, 556–559. doi: 10.1038/nature12391
Belser, J. A., Maines, T. R., Katz, J. M., and Tumpey, T. M. (2013b). Considerations regarding appropriate sample size for conducting ferret transmission experiments. Future Microbiol. 8, 961–965. doi: 10.2217/fmb.13.64
Bird, N. L., Olson, M. R., Hurt, A. C., Oshansky, C. M., Oh, D. Y., Reading, P. C., et al. (2015). Oseltamivir prophylaxis reduces inflammation and facilitates establishment of cross-strain protective T cell memory to influenza viruses. PLoS ONE 10:e0129768. doi: 10.1371/journal.pone.0129768
Bodewes, R., Kreijtz, J. H., van Amerongen, G., Fouchier, R. A., Osterhaus, A. D., Rimmelzwaan, G. F., et al. (2011). Pathogenesis of Influenza A/H5N1 virus infection in ferrets differs between intranasal and intratracheal routes of inoculation. Am. J. Pathol. 179, 30–36. doi: 10.1016/j.ajpath.2011.03.026
Boltz, D. A., Rehg, J. E., McClaren, J., Webster, R. G., and Govorkova, E. A. (2008). Oseltamivir prophylactic regimens prevent H5N1 influenza morbidity and mortality in a ferret model. J. Infect. Dis. 197, 1315–1323. doi: 10.1086/586711
Bouvier, N. M., and Lowen, A. C. (2010). Animal models for influenza virus pathogenesis and transmission. Viruses 2, 1530–1563. doi: 10.3390/v20801530
Buhnerkempe, M. G., Gostic, K., Park, M., Ahsan, P., Belser, J. A., and Lloyd-Smith, J. O. (2015). Mapping influenza transmission in the ferret model to transmission in humans. Elife 4:e07969. doi: 10.7554/eLife.07969
Burger, R. A., Billingsley, J. L., Huffman, J. H., Bailey, K. W., Kim, C. U., and Sidwell, R. W. (2000). Immunological effects of the orally administered neuraminidase inhibitor oseltamivir in influenza virus-infected and uninfected mice. Immunopharmacology 47, 45–52. doi: 10.1016/S0162-3109(99)00184-8
Cameron, C. M., Cameron, M. J., Bermejo-Martin, J. F., Ran, L., Xu, L., Turner, P. V., et al. (2008). Gene expression analysis of host innate immune responses during Lethal H5N1 infection in ferrets. J. Virol. 82, 11308–11317. doi: 10.1128/jvi.00691-08
Carolan, L. A., Butler, J., Rockman, S., Guarnaccia, T., Hurt, A. C., Reading, P., et al. (2014). TaqMan real time RT-PCR assays for detecting ferret innate and adaptive immune responses. J. Virol. Methods 205C, 38–52. doi: 10.1016/j.jviromet.2014.04.014
Carolan, L. A., Rockman, S., Borg, K., Guarnaccia, T., Reading, P., Mosse, J., et al. (2015). Characterisation of the localised immune response in the respiratory tract of ferrets following infection with influenza A and B viruses. J. Virol. doi: 10.1128/jvi.02797-15 [Epub ahead of print].
Chairat, K., Tarning, J., White, N. J., and Lindegardh, N. (2013). Pharmacokinetic properties of anti-influenza neuraminidase inhibitors. J. Clin. Pharmacol. 53, 119–139. doi: 10.1177/0091270012440280
ChinaBioToday (2013). China’s SFDA Ready to Fast-Track Approvals of Peramivir, a Flu Treatment. Available at: http://www.chinabiotoday.com/articles/20130408_1 [accessed March 13, 2014].
ClinicalTrials (2015). National Institute of Health. Available at: https://clinicaltrials.gov/ [accessed July 13, 2015].
Cox, N., and Subbarao, K. (1999). Influenza. Lancet 354, 1277–1282. doi: 10.1016/S0140-6736(99)01241-6
Darton, T. C., Blohmke, C. J., Moorthy, V. S., Altmann, D. M., Hayden, F. G., Clutterbuck, E. A., et al. (2015). Design, recruitment, and microbiological considerations in human challenge studies. Lancet Infect Dis. 15, 840–851. doi: 10.1016/s1473-3099(15)00068-7
Davis, A. S., Taubenberger, J. K., and Bray, M. (2015). The use of nonhuman primates in research on seasonal, pandemic and avian influenza, 1893-2014. Antiviral Res. 117, 75–98. doi: 10.1016/j.antiviral.2015.02.011
Enkirch, T., and von Messling, V. (2015). Ferret models of viral pathogenesis. Virology 479–480, 259–270. doi: 10.1016/j.virol.2015.03.017
Gabor, K. A., Goody, M. F., Mowel, W. K., Breitbach, M. E., Gratacap, R. L., Witten, P. E., et al. (2014). Influenza A virus infection in zebrafish recapitulates mammalian infection and sensitivity to anti-influenza drug treatment. Dis. Model Mech. 7, 1227–1237. doi: 10.1242/dmm.014746
Gavin, P., and Thomson, R. (2003). Review of rapid diagnosis tests for influenza. Clin. Applied Immunol. Rev. 4, 151–172. doi: 10.1016/S1529-1049(03)00064-3
Govorkova, E. A., Ilyushina, N. A., Boltz, D. A., Douglas, A., Yilmaz, N., and Webster, R. G. (2007). Efficacy of oseltamivir therapy in ferrets inoculated with different clades of H5N1 influenza virus. Antimicrob. Agents Chemother. 51, 1414–1424. doi: 10.1128/aac.01312-06
Govorkova, E. A., Marathe, B. M., Prevost, A., Rehg, J. E., and Webster, R. G. (2011). Assessment of the efficacy of the neuraminidase inhibitor oseltamivir against 2009 pandemic H1N1 influenza virus in ferrets. Antiviral Res. 91, 81–88. doi: 10.1016/j.antiviral.2011.05.008
Govorkova, E. A., Rehg, J. E., Krauss, S., Yen, H. L., Guan, Y., Peiris, M., et al. (2005). Lethality to ferrets of H5N1 influenza viruses isolated from humans and poultry in 2004. J. Virol. 79, 2191–2198. doi: 10.1128/jvi.79.4.2191-2198.2005
Gustin, K. M., Belser, J. A., Wadford, D. A., Pearce, M. B., Katz, J. M., Tumpey, T. M., et al. (2011). Influenza virus aerosol exposure and analytical system for ferrets. Proc. Natl. Acad. Sci. U.S.A. 108, 8432–8437. doi: 10.1073/pnas.1100768108
Haffizulla, J., Hartman, A., Hoppers, M., Resnick, H., Samudrala, S., Ginocchio, C., et al. (2014). Effect of nitazoxanide in adults and adolescents with acute uncomplicated influenza: a double-blind, randomised, placebo-controlled, phase 2b/3 trial. Lancet Infect. Dis. 14, 609–618. doi: 10.1016/s1473-3099(14)70717-0
Hamelin, M. E., Baz, M., Bouhy, X., Beaulieu, E., Dube, K., Mallett, C., et al. (2011). Reduced airborne transmission of oseltamivir-resistant pandemic A/H1N1 virus in ferrets. Antivir. Ther. 16, 775–779. doi: 10.3851/imp1794
Hayden, F. G., Osterhaus, A. D., Treanor, J. J., Fleming, D. M., Aoki, F. Y., Nicholson, K. G., et al. (1997). Efficacy and safety of the neuraminidase inhibitor zanamivir in the treatment of influenzavirus infections, GG167 Influenza Study Group. N. Engl. J. Med. 337, 874–880. doi: 10.1056/nejm199709253371302
Hayden, F. G., Treanor, J. J., Fritz, R. S., Lobo, M., Betts, R. F., Miller, M., et al. (1999). Use of the oral neuraminidase inhibitor oseltamivir in experimental human influenza: randomized controlled trials for prevention and treatment. JAMA 282, 1240–1246. doi: 10.1001/jama.282.13.1240
Herfst, S., Schrauwen, E. J., Linster, M., Chutinimitkul, S., de Wit, E., Munster, V. J., et al. (2012). Airborne transmission of influenza A/H5N1 virus between ferrets. Science 336, 1534–1541. doi: 10.1126/science.1213362
Herlocher, M. L., Truscon, R., Fenton, R., Klimov, A., Elias, S., Ohmit, S. E., et al. (2003). Assessment of development of resistance to antivirals in the ferret model of influenza virus infection. J. Infect. Dis. 188, 1355–1361. doi: 10.1086/379049
Hoffman, H. E., Gaylord, J. C., Blasecki, J. W., Shalaby, L. M., and Whitney, C. C. Jr. (1988). Pharmacokinetics and metabolism of rimantadine hydrochloride in mice and dogs. Antimicrob. Agents Chemother. 32, 1699–1704. doi: 10.1128/AAC.32.11.1699
Hu, Y., Lu, S., Song, Z., Wang, W., Hao, P., Li, J., et al. (2013). Association between adverse clinical outcome in human disease caused by novel influenza A H7N9 virus and sustained viral shedding and emergence of antiviral resistance. Lancet 381, 2273–2279. doi: 10.1016/s0140-6736(13)61125-3
Huang, S. S., Banner, D., Degousee, N., Leon, A. J., Xu, L., Paquette, S. G., et al. (2012). Differential pathological and immune responses in newly weaned ferrets are associated with a mild clinical outcome of pandemic 2009 H1N1 infection. J. Virol. 86, 13187–13201. doi: 10.1128/jvi.01456-12
Huang, S. S., Banner, D., Fang, Y., Ng, D. C., Kanagasabai, T., Kelvin, D. J., et al. (2011). Comparative analyses of pandemic H1N1 and seasonal H1N1, H3N2, and influenza B infections depict distinct clinical pictures in ferrets. PLoS ONE 6:e27512. doi: 10.1371/journal.pone.0027512
Hurt, A. C., Lowther, S., Middleton, D., and Barr, I. G. (2010). Assessing the development of oseltamivir and zanamivir resistance in A(H5N1) influenza viruses using a ferret model. Antiviral Res. 87, 361–366. doi: 10.1016/j.antiviral.2010.06.009
Ikematsu, H., and Kawai, N. (2011). Laninamivir octanoate: a new long-acting neuraminidase inhibitor for the treatment of influenza. Exp. Rev. Anti Infect Ther. 9, 851–857. doi: 10.1586/eri.11.112
Itoh, Y., Shinya, K., Kiso, M., Watanabe, T., Sakoda, Y., Hatta, M., et al. (2009). In vitro and in vivo characterization of new swine-origin H1N1 influenza viruses. Nature 460, 1021–1025. doi: 10.1038/nature08260
Jefferson, T., Jones, M., Doshi, P., Spencer, E. A., Onakpoya, I., and Heneghan, C. J. (2014). Oseltamivir for influenza in adults and children: systematic review of clinical study reports and summary of regulatory comments. BMJ 348:2545. doi: 10.1136/bmj.g2545
Jia, N., Barclay, W. S., Roberts, K., Yen, H. L., Chan, R. W., Lam, A. K., et al. (2014). Glycomic characterization of respiratory tract tissues of ferrets: implications for its use in influenza virus infection studies. J. Biol. Chem. 289, 28489–28504. doi: 10.1074/jbc.M114.588541
Kim, E. H., Park, S. J., Kwon, H. I., Kim, S. M., Kim, Y. I., Song, M. S., et al. (2015). Mouse adaptation of influenza B virus increases replication in the upper respiratory tract and results in droplet transmissibility in ferrets. Sci. Rep. 5:15940. doi: 10.1038/srep15940
Kim, H. M., Kang, Y. M., Ku, K. B., Park, E. H., Yum, J., Kim, J. C., et al. (2013). The severe pathogenicity of alveolar macrophage-depleted ferrets infected with 2009 pandemic H1N1 influenza virus. Virology 444, 394–403. doi: 10.1016/j.virol.2013.07.006
Kiso, M., Takahashi, K., Sakai-Tagawa, Y., Shinya, K., Sakabe, S., Le, Q. M., et al. (2010). T-705 (favipiravir) activity against lethal H5N1 influenza A viruses. Proc. Natl. Acad. Sci. U.S.A. 107, 882–887. doi: 10.1073/pnas.0909603107
Kitano, M., Itoh, Y., Kodama, M., Ishigaki, H., Nakayama, M., Ishida, H., et al. (2011). Efficacy of single intravenous injection of peramivir against influenza B virus infection in ferrets and cynomolgus macaques. Antimicrob. Agents Chemother. 55, 4961–4970. doi: 10.1128/aac.00412-11
Kodama, M., Yoshida, R., Hasegawa, T., Izawa, M., Kitano, M., Baba, K., et al. (2014). The relationship between in vivo antiviral activity and pharmacokinetic parameters of peramivir in influenza virus infection model in mice. Antiviral Res. 109, 110–115. doi: 10.1016/j.antiviral.2014.06.016
Koyama, K., Takahashi, M., Nakai, N., Takakusa, H., Murai, T., Hoshi, M., et al. (2010). Pharmacokinetics and disposition of CS-8958, a long-acting prodrug of the novel neuraminidase inhibitor laninamivir in rats. Xenobiotica 40, 207–216. doi: 10.3109/00498250903447691
Koyama, K., Takahashi, M., Oitate, M., Nakai, N., Takakusa, H., Miura, S., et al. (2009). CS-8958, a prodrug of the novel neuraminidase inhibitor R-125489, demonstrates a favorable long-retention profile in the mouse respiratory tract. Antimicrob. Agents Chemother. 53, 4845–4851. doi: 10.1128/aac.00731-09
Kreijtz, J. H., Kroeze, E. J., Stittelaar, K. J., de Waal, L., van Amerongen, G., van Trierum, S., et al. (2013). Low pathogenic avian influenza A(H7N9) virus causes high mortality in ferrets upon intratracheal challenge: a model to study intervention strategies. Vaccine 31, 4995–4999. doi: 10.1016/j.vaccine.2013.06.071
Kubo, S., Tomozawa, T., Kakuta, M., Tokumitsu, A., and Yamashita, M. (2010). Laninamivir prodrug CS-8958, a long-acting neuraminidase inhibitor, shows superior anti-influenza virus activity after a single administration. Antimicrob. Agents Chemother. 54, 1256–1264. doi: 10.1128/aac.01311-09
Lee, D. H., Kim, J. I., Lee, J. W., Chung, W. H., Park, J. K., Lee, Y. N., et al. (2014). Quantitative measurement of influenza virus replication using consecutive bronchoalveolar lavage in the lower respiratory tract of a ferret model. J. Vet. Sci. 15, 439–442. doi: 10.4142/jvs.2014.15.3.439
Leon, A. J., Banner, D., Xu, L., Ran, L., Peng, Z., Yi, K., et al. (2013). Sequencing, annotation, and characterization of the influenza ferret infectome. J. Virol. 87, 1957–1966. doi: 10.1128/jvi.02476-12
Li, W., Escarpe, P. A., Eisenberg, E. J., Cundy, K. C., Sweet, C., Jakeman, K. J., et al. (1998). Identification of GS 4104 as an orally bioavailable prodrug of the influenza virus neuraminidase inhibitor GS 4071. Antimicrob. Agents Chemother. 42, 647–653.
Lowen, A. C., Bouvier, N. M., and Steel, J. (2014). Transmission in the guinea pig model. Curr. Top. Microbiol. Immunol. 385, 157–183. doi: 10.1007/82_2014_390
Lowen, A. C., Mubareka, S., Tumpey, T. M., Garcia-Sastre, A., and Palese, P. (2006). The guinea pig as a transmission model for human influenza viruses. Proc. Natl. Acad. Sci. U.S.A. 103, 9988–9992. doi: 10.1073/pnas.0604157103
Mabry, J., Rowe, T., Blanchard, E., and Xu, X. (2013). Development of a HEPA-filtered caging system for ferrets: thinking outside the metal box. Lab. Animal Sci. Prof. 1, 41–44.
Maines, T. R., Belser, J. A., Gustin, K. M., van Hoeven, N., Zeng, H., Svitek, N., et al. (2012). Local innate immune responses and influenza virus transmission and virulence in ferrets. J. Infect. Dis. 205, 474–485. doi: 10.1093/infdis/jir768
Maines, T. R., Jayaraman, A., Belser, J. A., Wadford, D. A., Pappas, C., Zeng, H., et al. (2009). Transmission and pathogenesis of swine-origin 2009 A(H1N1) influenza viruses in ferrets and mice. Science 325, 484–487. doi: 10.1126/science.1177238
Makela, M. J., Pauksens, K., Rostila, T., Fleming, D. M., Man, C. Y., Keene, O. N., et al. (2000). Clinical efficacy and safety of the orally inhaled neuraminidase inhibitor zanamivir in the treatment of influenza: a randomized, double-blind, placebo-controlled European study. J. Infect. 40, 42–48. doi: 10.1053/jinf.1999.0602
Margine, I., and Krammer, F. (2014). Animal models for influenza viruses: implications for universal vaccine development. Pathogens 3, 845–874. doi: 10.3390/pathogens3040845
Marjuki, H., Mishin, V. P., Chesnokov, A. P., De La Cruz, J. A., Davis, C. T., Villanueva, J. M., et al. (2015). Neuraminidase mutations conferring resistance to oseltamivir in influenza A(H7N9) viruses. J. Virol. 89, 5419–5426. doi: 10.1128/jvi.03513-14
Marjuki, H., Mishin, V. P., Chesnokov, A. P., De La Cruz, J. A., Fry, A. M., Villanueva, J., et al. (2014). An investigational antiviral drug, DAS181, effectively inhibits replication of zoonotic influenza A virus subtype H7N9 and protects mice from lethality. J. Infect. Dis. 210, 435–440. doi: 10.1093/infdis/jiu105
Marois, I., Cloutier, A., Garneau, E., Lesur, O., and Richter, M. V. (2015). The administration of oseltamivir results in reduced effector and memory CD8+ T cell responses to influenza and affects protective immunity. FASEB J. 29, 973–987. doi: 10.1096/fj.14-260687
Marriott, A. C., Dove, B. K., Whittaker, C. J., Bruce, C., Ryan, K. A., Bean, T. J., et al. (2014). Low dose influenza virus challenge in the ferret leads to increased virus shedding and greater sensitivity to oseltamivir. PLoS ONE 9:e94090. doi: 10.1371/journal.pone.0094090
Martel, C. J., and Aasted, B. (2009). Characterization of antibodies against ferret immunoglobulins, cytokines and CD markers. Vet. Immunol. Immunopathol. 132, 109–115. doi: 10.1016/j.vetimm.2009.05.011
McBrayer, A., Camp, J. V., Tapp, R., Yamshchikov, V., Grimes, S., Noah, D. L., et al. (2010). Course of seasonal influenza A/Brisbane/59/07 H1N1 infection in the ferret. Virol. J. 7, 149. doi: 10.1186/1743-422x-7-149
McCullers, J. A. (2011). Preventing and treating secondary bacterial infections with antiviral agents. Antivir. Ther. 16, 123–135. doi: 10.3851/imp1730
Mendel, D. B., Tai, C. Y., Escarpe, P. A., Li, W., Sidwell, R. W., Huffman, J. H., et al. (1998). Oral administration of a prodrug of the influenza virus neuraminidase inhibitor GS 4071 protects mice and ferrets against influenza infection. Antimicrob. Agents Chemother. 42, 640–646.
Moore, I. N., Lamirande, E. W., Paskel, M., Donahue, D., Qin, J., and Subbarao, K. (2014). Severity of clinical disease and pathology in ferrets experimentally infected with influenza viruses is influenced by inoculum volume. J. Virol. 88, 13879–13891. doi: 10.1128/jvi.02341-14
Munster, V. J., de Wit, E., van den Brand, J. M., Herfst, S., Schrauwen, E. J., Bestebroer, T. M., et al. (2009). Pathogenesis and transmission of swine-origin 2009 A(H1N1) influenza virus in ferrets. Science 325, 481–483. doi: 10.1126/science.1177127
Music, N., Reber, A. J., Lipatov, A. S., Kamal, R. P., Blanchfield, K., Wilson, J. R., et al. (2014). Influenza vaccination accelerates recovery of ferrets from lymphopenia. PLoS ONE 9:e100926. doi: 10.1371/journal.pone.0100926
Nahar, K., Gupta, N., Gauvin, R., Absar, S., Patel, B., Gupta, V., et al. (2013). In vitro, in vivo and ex vivo models for studying particle deposition and drug absorption of inhaled pharmaceuticals. Eur. J. Pharm. Sci. 49, 805–818. doi: 10.1016/j.ejps.2013.06.004
Ng, P. S., Bohm, R., Hartley-Tassell, L. E., Steen, J. A., Wang, H., Lukowski, S. W., et al. (2014). Ferrets exclusively synthesize Neu5Ac and express naturally humanized influenza A virus receptors. Nat. Commun. 5:5750. doi: 10.1038/ncomms6750
Nguyen, H. T., Nguyen, T., Mishin, V. P., Sleeman, K., Balish, A., Jones, J., et al. (2013). Antiviral susceptibility of highly pathogenic avian influenza A(H5N1) viruses isolated from poultry, Vietnam, 2009-2011. Emerg. Infect. Dis. 19, 1963–1971. doi: 10.3201/eid1912.130705
Nicholson, K. G., Aoki, F. Y., Osterhaus, A. D., Trottier, S., Carewicz, O., Mercier, C. H., et al. (2000). Efficacy and safety of oseltamivir in treatment of acute influenza: a randomised controlled trial. Neuraminidase Inhibitor Flu Treatment Investigator Group. Lancet 355, 1845–1850.
Nishiura, H., Yen, H. L., and Cowling, B. J. (2013). Sample size considerations for one-to-one animal transmission studies of the influenza A viruses. PLoS ONE 8:e55358. doi: 10.1371/journal.pone.0055358
Oh, D. Y., Barr, I. G., and Hurt, A. C. (2015). A novel video tracking method to evaluate the effect of influenza infection and antiviral treatment on ferret activity. PLoS ONE 10:e0118780. doi: 10.1371/journal.pone.0118780
Oh, D. Y., Lowther, S., McCaw, J. M., Sullivan, S. G., Leang, S. K., Haining, J., et al. (2014). Evaluation of oseltamivir prophylaxis regimens for reducing influenza virus infection, transmission and disease severity in a ferret model of household contact. J. Antimicrob Chemother. 69, 2458–2469. doi: 10.1093/jac/dku146
Panozzo, J., Oh, D. Y., Margo, K., Morton, D. A., Piedrafita, D., Mosse, J., et al. (2015). Evaluation of a dry powder delivery system for laninamivir in a ferret model of influenza infection. Antiviral Res. 120, 66–71. doi: 10.1016/j.antiviral.2015.05.007
Paquette, S. G., Banner, D., Huang, S. S., Almansa, R., Leon, A., Xu, L., et al. (2015). Influenza transmission in the mother-infant dyad leads to severe disease, mammary gland infection, and pathogenesis by regulating host responses. PLoS Pathog. 11:e1005173. doi: 10.1371/journal.ppat.1005173
Paquette, S. G., Huang, S. S., Banner, D., Xu, L., Leomicronn, A., Kelvin, A. A., et al. (2014). Impaired heterologous immunity in aged ferrets during sequential influenza A H1N1 infection. Virology 46, 177–183. doi: 10.1016/j.virol.2014.07.013
Peng, X., Alfoldi, J., Gori, K., Eisfeld, A. J., Tyler, S. R., Tisoncik-Go, J., et al. (2014). The draft genome sequence of the ferret (Mustela putorius furo) facilitates study of human respiratory disease. Nat. Biotechnol. 32, 1250–1255. doi: 10.1038/nbt.3079
Reddy, M. B., Yang, K. H., Rao, G., Rayner, C. R., Nie, J., Pamulapati, C., et al. (2015). Oseltamivir population pharmacokinetics in the ferret: model application for pharmacokinetic/pharmacodynamic study design. PLoS ONE 10:e0138069. doi: 10.1371/journal.pone.0138069
Reuman, P. D., Keely, S., and Schiff, G. M. (1989). Assessment of signs of influenza illness in the ferret model. J. Virol. Methods 24, 27–34. doi: 10.1016/0166-0934(89)90004-9
Richard, M., Schrauwen, E. J., de Graaf, M., Bestebroer, T. M., Spronken, M. I., van Boheemen, S., et al. (2013). Limited airborne transmission of H7N9 influenza A virus between ferrets. Nature 501, 560–563. doi: 10.1038/nature12476
Roberts, K. L., Shelton, H., Stilwell, P., and Barclay, W. S. (2012). Transmission of a 2009 H1N1 pandemic influenza virus occurs before fever is detected, in the ferret model. PLoS ONE 7:e43303. doi: 10.1371/journal.pone.0043303
Rossignol, J. F. (2014). Nitazoxanide: a first-in-class broad-spectrum antiviral agent. Antiviral Res. 110, 94–103. doi: 10.1016/j.antiviral.2014.07.014
Rowe, T., Leon, A. J., Crevar, C. J., Carter, D. M., Xu, L., Ran, L., et al. (2010). Modeling host responses in ferrets during A/California/07/2009 influenza infection. Virology 401, 257–265. doi: 10.1016/j.virol.2010.02.020
Rutigliano, J. A., Doherty, P. C., Franks, J., Morris, M. Y., Reynolds, C., and Thomas, P. G. (2008). Screening monoclonal antibodies for cross-reactivity in the ferret model of influenza infection. J. Immunol. Methods 336, 71–77. doi: 10.1016/j.jim.2008.04.003
Ryan, D. M., Ticehurst, J., Dempsey, M. H., and Penn, C. R. (1994). Inhibition of influenza virus replication in mice by GG167 (4-guanidino-2,4-dideoxy-2,3-dehydro-N-acetylneuraminic acid) is consistent with extracellular activity of viral neuraminidase (sialidase). Antimicrob. Agents Chemother. 38, 2270–2275. doi: 10.1128/AAC.38.10.2270
Smee, D. F., Julander, J. G., Tarbet, E. B., Gross, M., and Nguyen, J. (2012a). Treatment of oseltamivir-resistant influenza A (H1N1) virus infections in mice with antiviral agents. Antiviral Res. 96, 13–20. doi: 10.1016/j.antiviral.2012.07.002
Smee, D. F., von Itzstein, M., Bhatt, B., and Tarbet, E. B. (2012b). Exacerbation of influenza virus infections in mice by intranasal treatments and implications for evaluation of antiviral drugs. Antimicrob. Agents Chemother. 56, 6328–6333. doi: 10.1128/aac.01664-12
Smith, J. H., Nagy, T., Driskell, E., Brooks, P., Tompkins, S. M., and Tripp, R. A. (2011). Comparative pathology in ferrets infected with H1N1 influenza A viruses isolated from different hosts. J. Virol. 85, 7572–7581. doi: 10.1128/jvi.00512-11
Smith, W., Andrewes, C. H., and Laidlaw, P. P. (1933). A virus obtained from influenza patients. Lancet 222, 66–68. doi: 10.1016/S0140-6736(00)78541-2
Stark, G. V., Long, J. P., Ortiz, D. I., Gainey, M., Carper, B. A., Feng, J., et al. (2013). Clinical profiles associated with influenza disease in the ferret model. PLoS ONE 8:e58337. doi: 10.1371/journal.pone.0058337
Takashita, E., Meijer, A., Lackenby, A., Gubareva, L., Rebelo-de-Andrade, H., Besselaar, T., et al. (2015). Global update on the susceptibility of human influenza viruses to neuraminidase inhibitors, 2013-2014. Antiviral Res. 117, 27–38. doi: 10.1016/j.antiviral.2015.02.003
Thangavel, R. R., and Bouvier, N. M. (2014). Animal models for influenza virus pathogenesis, transmission, and immunology. J. Immunol. Methods 410, 60–79. doi: 10.1016/j.jim.2014.03.023
The MIST (1998). Randomised trial of efficacy and safety of inhaled zanamivir in treatment of influenza A and B virus infections. The MIST (Management of Influenza in the Southern Hemisphere Trialists) Study Group. Lancet 352, 1877–1881. doi: 10.1016/S0140-6736(98)10190-3
Treanor, J. J., Hayden, F. G., Vrooman, P. S., Barbarash, R., Bettis, R., Riff, D., et al. (2000). Efficacy and safety of the oral neuraminidase inhibitor oseltamivir in treating acute influenza: a randomized controlled trial. US Oral Neuraminidase Study Group. JAMA 283, 1016–1024. doi: 10.1001/jama.283.8.1016
Triana-Baltzer, G. B., Gubareva, L. V., Nicholls, J. M., Pearce, M. B., Mishin, V. P., Belser, J. A., et al. (2009). Novel pandemic influenza A(H1N1) viruses are potently inhibited by DAS181, a sialidase fusion protein. PLoS ONE 4:e7788. doi: 10.1371/journal.pone.0007788
Turner, P. V., Brabb, T., Pekow, C., and Vasbinder, M. A. (2011). Administration of substances to laboratory animals: routes of administration and factors to consider. J. Am. Assoc. Lab. Anim. Sci. 50, 600–613.
Urso, R., Blardi, P., and Giorgi, G. (2002). A short introduction to pharmacokinetics. Eur. Rev. Med. Pharmacol. Sci. 6, 33–44.
van der Vries, E., Stittelaar, K. J., van Amerongen, G., Veldhuis Kroeze, E. J., de Waal, L., Fraaij, P. L., et al. (2013). Prolonged influenza virus shedding and emergence of antiviral resistance in immunocompromised patients and ferrets. PLoS Pathog. 9:e1003343. doi: 10.1371/journal.ppat.1003343
van der Vries, E., Veldhuis Kroeze, E. J., Stittelaar, K. J., Linster, M., Van der Linden, A., Schrauwen, E. J., et al. (2011). Multidrug resistant 2009 A/H1N1 influenza clinical isolate with a neuraminidase I223R mutation retains its virulence and transmissibility in ferrets. PLoS Pathog. 7:e1002276. doi: 10.1371/journal.ppat.1002276
Vidana, B., Martinez, J., Martinez-Orellana, P., Garcia Migura, L., Montoya, M., Martorell, J., et al. (2014). Heterogeneous pathological outcomes after experimental pH1N1 influenza infection in ferrets correlate with viral replication and host immune responses in the lung. Vet. Res. 45, 85. doi: 10.1186/s13567-014-0085-8
Wong, Z. X., Jones, J. E., Anderson, G. P., and Gualano, R. C. (2011). Oseltamivir treatment of mice before or after mild influenza infection reduced cellular and cytokine inflammation in the lung. Influenza Other Respir. Viruses 5, 343–350. doi: 10.1111/j.1750-2659.2011.00235.x
Yen, H. L., Lipatov, A. S., Ilyushina, N. A., Govorkova, E. A., Franks, J., Yilmaz, N., et al. (2007). Inefficient transmission of H5N1 influenza viruses in a ferret contact model. J. Virol. 81, 6890–6898. doi: 10.1128/jvi.00170-07
Yun, N. E., Linde, N. S., Zacks, M. A., Barr, I. G., Hurt, A. C., Smith, J. N., et al. (2008). Injectable peramivir mitigates disease and promotes survival in ferrets and mice infected with the highly virulent influenza virus, A/Vietnam/1203/04 (H5N1). Virology 374, 198–209. doi: 10.1016/j.virol.2007.12.029
Zarogiannis, S. G., Noah, J. W., Jurkuvenaite, A., Steele, C., Matalon, S., and Noah, D. L. (2012). Comparison of ribavirin and oseltamivir in reducing mortality and lung injury in mice infected with mouse adapted A/California/04/2009 (H1N1). Life Sci. 90, 440–445. doi: 10.1016/j.lfs.2011.12.014
Keywords: ferret, antiviral, animal model, influenza, effectiveness
Citation: Oh DY and Hurt AC (2016) Using the Ferret as an Animal Model for Investigating Influenza Antiviral Effectiveness. Front. Microbiol. 7:80. doi: 10.3389/fmicb.2016.00080
Received: 02 December 2015; Accepted: 18 January 2016;
Published: 04 February 2016.
Edited by:
Slobodan Paessler, University of Texas Medical Branch, USAReviewed by:
Paul Horwood, Institut Pasteur in Cambodia, CambodiaBarry Rockx, Rijksinstituut voor Volksgezondheid en Milieu, Netherlands
Copyright © 2016 Oh and Hurt. This is an open-access article distributed under the terms of the Creative Commons Attribution License (CC BY). The use, distribution or reproduction in other forums is permitted, provided the original author(s) or licensor are credited and that the original publication in this journal is cited, in accordance with accepted academic practice. No use, distribution or reproduction is permitted which does not comply with these terms.
*Correspondence: Ding Y. Oh, dingthomas.oh@influenzacentre.org; Aeron C. Hurt, aeron.hurt@influenzacentre.org