- Department of Aquaculture, School of Marine Sciences, Ningbo University, Ningbo, China
Quorum sensing (QS) is a widespread intercellular form of communication to coordinate physiological processes and cooperative activities of bacteria at the population level, and it depends on the production, secretion, and detection of small diffusible autoinducers, such as acyl-homoserine lactones (AHLs), auto-inducing oligo-peptides (AIPs) and autoinducer 2. In this review, the function of QS autoinducers of gram-negative bacteria in different aspects of wastewater treatment systems is examined. Based on research primarily performed over the past 10 years, QS involvement in the formation of biofilm and aerobic granules and changes of the microbial community and degradation/transformation pathways is discussed. In particular, the QS pathway in the role of bacterial infections and disease prevention in aquaculture is addressed. Interference of QS autoinducer-regulated pathways is considered potential treatment for a variety of environmentally related problems. This review is expected to serve as a stepping stone for further study and development strategies based on the mediation of QS-regulated pathways to enhance applications in both wastewater treatment systems and aquaculture.
Introduction
Cell-cell communication is ubiquitously used in microbes and microbial communities to monitor and adapt to their external environment via contact-based chemical exchanges (Lobedanz and Søgaard-Andersen, 2003; Phelan et al., 2012), chemical signaling (Eberhard et al., 1981; Hussain et al., 2008; Schaefer et al., 2008; Galloway et al., 2011) and electric signaling (Nielsen et al., 2010; Shrestha et al., 2013). In one such system, quorum sensing (QS), bacteria can “count” their local population numbers using autoinducers, which are small chemical molecules. QS was first discovered in the marine bacterium Vibrio fischeri (Nealson et al., 1970) and later was coined by Fuqua et al. (1994), referring to the acylated homoserine lactone (AHL)-mediated luxR/luxI regulated system.
In QS bacteria, when the concentration of autoinducer accumulates to a certain threshold, autoinducers bind to transcriptional regulators to alter gene expression profiles (Miller and Bassler, 2001; Di Cagno et al., 2011; Schaefer et al., 2013) or bind to extracellular domains of specific membrane histidine kinase receptors followed by autophosphorylation and a cognate cytoplasmic response regulator (Sturme et al., 2002; Taga et al., 2003; Neiditch et al., 2006; Thoendel et al., 2011; Ke et al., 2015). Autoinducers regulate the expression of QS-dependent genes on a population-wide scale, endowing bacteria with the ability to live in a “society” that controls many important physiological processes and to initiate “co-operative” behaviors, such as biofilm development (Sakuragi and Kolter, 2007; Shepherd and Lindow, 2009; Liu et al., 2011; Anbazhagan et al., 2012; Ren et al., 2013), pathogenesis (Smith et al., 2007; Natrah et al., 2012; Decker et al., 2013; Pande et al., 2013), and pollutant biodegradation (Toyofuku et al., 2007; Yong and Zhong, 2010, 2013b; Wang et al., 2013a,b; Yong et al., 2015; Figure 1). These activities are unlikely to be completed when only one individual bacterium undertakes the task; therefore, QS has a significant impact on the environment (Jayaraman and Wood, 2008; Galloway et al., 2012), healthcare (Biradar and Devi, 2011; Jamuna Bai and Ravishankar Rai, 2011; Galloway et al., 2012; Yong and Zhong, 2013a) and agriculture (Kalia, 2013). Recently, a number of excellent reviews have highlighted the roles of QS systems, particularly the disruption of QS systems, in water treatment systems (Nguyen et al., 2012; Feng et al., 2013; Lade et al., 2014; Siddiqui et al., 2015; Yong et al., 2015), biodegradation (Díaz et al., 2013; Yong et al., 2015), and pathogenesis control (Bhardwaj et al., 2013; LaSarre and Federle, 2013) as well as in the development of novel disease diagnosis strategies and antimicrobial agents (Roy et al., 2011; Nafee et al., 2014; Rampioni et al., 2014). In this review, a selection of recent case studies demonstrating progress in the development of autoinducer-mediated QS systems used by gram-negative bacteria is summarized, and a systematic review of the manipulation of autoinducer-mediated QS systems in wastewater treatment systems and aquaculture is presented.
Basics of QS Signaling Molecules
Three types of signaling molecules are generally produced and secreted by QS bacteria (Di Cagno et al., 2011). Acyl-homoserine lactones (AHLs, Figure 2A) are the best known class of autoinducers and are frequently used by gram-negative bacteria (Galloway et al., 2011), whereas autoinducing peptides (AIPs, Figure 2B) are the major autoinducers produced by gram-positive bacteria (Sturme et al., 2002; Thoendel et al., 2011). One non-species-specific autoinducer used as “universal language,” autoinducer 2 (AI-2) (Figure 2C), is used for intra- and inter-species communication regardless of whether they are gram-negative or gram-positive bacteria (Lowery et al., 2008). Moreover, autoinducer 3 (AI-3) produced by intestinal bacterial species, such as enterohemorrhagic Escherichia coli (EHEC) O157:H7, is involved in pathogen-host interactions (Sperandio et al., 2003; Walters et al., 2006). In addition to these common QS signaling molecules, communication by CAI-1, namely (S)-3-hydroxytridecan-4-one (Figure 2D), is used by V. cholera (Higgins et al., 2007). Relatively simple fatty acid derivatives called diffusible signal factors (DSFs) are used by Burkholderia cepacia and Helicobacter pylori, respectively (Deng et al., 2010; Tanaka et al., 2011). Diffusible extracellular factor (DF) is used by Xanthomonas campestris (He et al., 2011). Pseudomonas quinolone signaling (PQS) is identified in P. aeruginosa (Bala et al., 2013, 2014). Dialkylresorcinol is used by Photorhabdus asymbiotica (Brameyer et al., 2015).
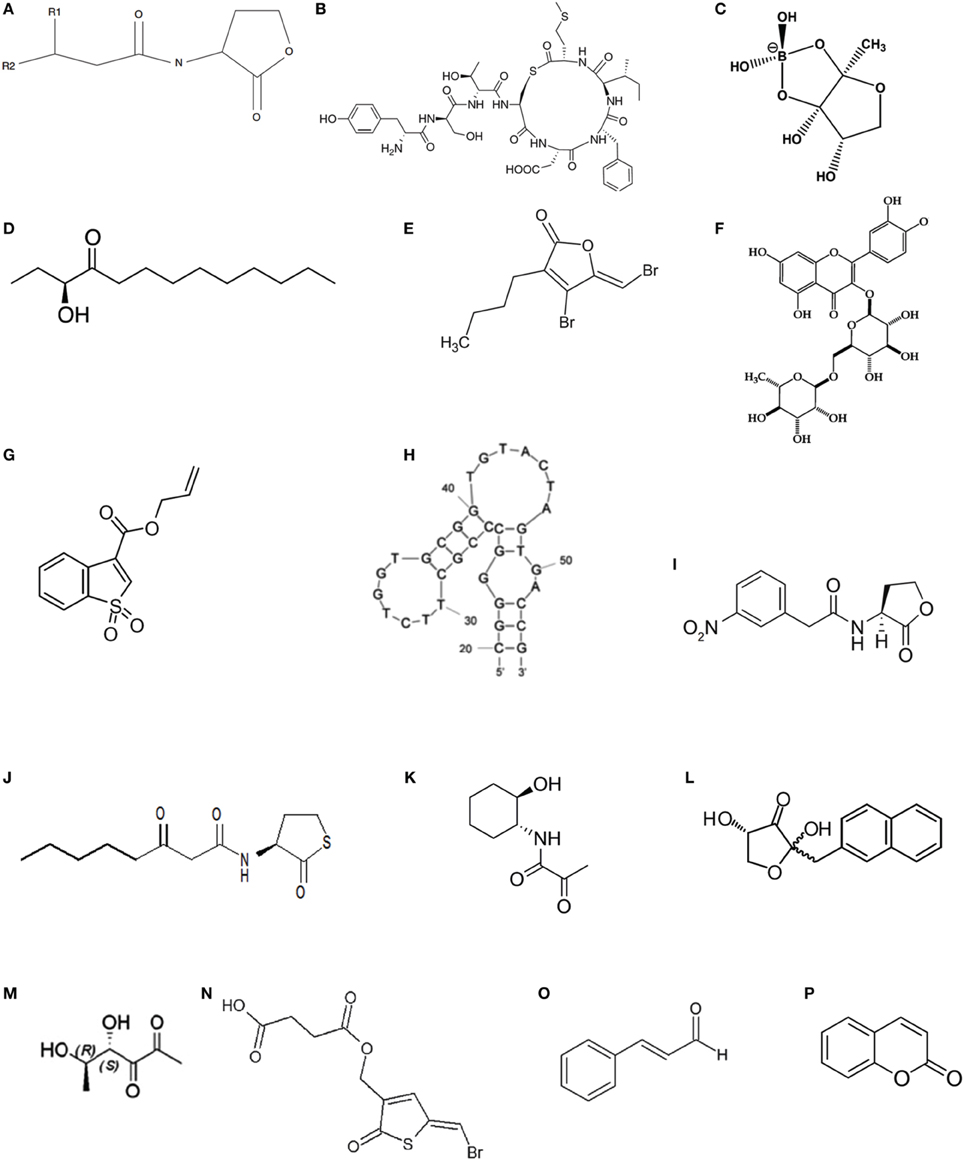
Figure 2. Chemical structures of specific QS signaling molecules, QSIs and agonistic analogs of QS signaling molecules. (A) Basic structure of the AHL signaling molecule where R1 = H, OH, or O and R2 = C1–C18; (B) AIP-1; (C) AI-2; (D) CAI-1 C13-AHK; (E) (5Z)-4-bromo-5-(bromomethykene) -3-butyl-2(5H)-furanone; (F) rutin; (G) allyl benzo[b]thiophene-3-carboxylate 1,1-dioxide; (H) homoserine lactone (HSL) aptamer; (I) N-(3-nitro-phenylacetanoyl)-L-homoserine lactone; (J) N-(3-oxo-acyl)-homocysteine thiolactones; (K) N-(3-oxo-acyl)-trans-2-aminocyclohexanols; (L) naphthyl-DPD; (M) (4S,5R)- -dihydroxyhexanediones; (N) (Z)-4-((5-(bromomethylene)-2-oxo- 2,5-dihydrothiophen-3-yl)-4-oxobutanoic acid); (O) cinnamaldehyde; (P) coumarin.
AHLs are the most common class of autoinducers and are present in approximately 10% of proteobacteria isolated from various ecological niches (Tan et al., 2015). AHL signaling molecules possess a homoserine lactone (HSL) moiety, acyl side chains with 4–18 carbons that determine the structure diversity and a substitution primarily belonging to three types: (a) simple acyl, (b) 3-hydroxyacyl, and (c) 3-oxoacyl groups (Marketon et al., 2002; Schaefer et al., 2008; Thiel et al., 2009; Galloway et al., 2011; Savka et al., 2011). The biological activity of these molecules depends on the stereochemistry (Geske et al., 2008; Galloway et al., 2011). In the LuxI/R system, LuxI proteins are responsible for the synthesis of AHLs, and LuxR proteins combine with AHLs to control target gene expression. This system is the most prevalent QS regulatory system in gram-negative bacteria (Miller and Bassler, 2001). However, LuxR orphans or solos, i.e., LuxR homologs without the cognate autoinducer synthase LuxI, were also reported, such as SdiA in Salmonella enterica and E. coli (Ahmer et al., 1998; Kanamaru et al., 2000), QscR in P. aeruginosa (Fuqua, 2006) and PluR in Photorhabdus luminescens (Brameyer et al., 2015). These LuxR orphans contain an AHL-binding domain at the N-terminus to bind to endogenous AHLs (Lequette et al., 2006), exogenous AHLs (Yao et al., 2006) or other novel signals (Brameyer et al., 2015) as well as a DNA-binding helix-turn-helix (HTH) domain at the C-terminus.
AI-2, which represents a universal “language” to facilitate interspecies communication, is a byproduct of the detoxification of S-adenosylmethionine (SAM), which is catalyzed by S-ribosylhomocysteine lyase (LuxS, EC 4.4.1.21) (Xavier and Bassler, 2005a; Lowery et al., 2008). Studies of the recognition and signal transduction of AI-2 have focused primarily on the LuxP-based system of V. harveyi (Neiditch et al., 2005; Defoirdt et al., 2008) and LasB-based systems of E. coli (Xavier and Bassler, 2005b) and Salmonella sp. (Taga et al., 2003; Zhu et al., 2008). Different bacteria responded to AI-2 in different manners, because differentiation of AI-2 related signaling primarily occurred at the level of transduction. However, recent studies demonstrated that the AI-2 of Campylobacter jejuni strain NCTC 11168e (Holmes et al., 2009) and LuxS in Vibrio ichthyoenteri (Li et al., 2010) may not be involved in cell-cell communication or the autoinducer-regulated phenotypes of cell growth, biofilm formation, or virulence gene expression.
Because prokaryotes and eukaryotes have coexisted and coevolved for millions of years, the existence of interkingdom communication inevitably evolved (Shiner et al., 2005; Lowery et al., 2008; González and Venturi, 2013). The “languages” of AHLs (Joint et al., 2002; Bortolotti et al., 2015), epinephrine/norepinephrine (Sperandio et al., 2003) and AI-3 systems (Clarke et al., 2006) are used as signaling molecules in interkingdom communication. The first example of QS signaling molecules modulating the host cells was N-(3-oxododecanoyl)-L-homoserine lactone (3-oxo-C12-HSL) secreted by P. aeruginosa (Telford et al., 1998). The immunomodulatory activity of 3-oxo-C12-HSL was further shown to inhibit T-cell differentiation and cytokine production by a mechanism involving an early step in T-cell activation (Ritchie et al., 2005), but this signaling molecule induced the expression of HLA-G, the non-classical class I human leukocyte antigen (HLA), in monocytes and T-cells (Bortolotti et al., 2015). The eukaryotic response to QS is also interesting, and the representative example is the interference of bacterial QS with furanone produced by the red alga Delisea pulchra (Givskov et al., 1996). Since then, the signaling interfering activity of brominated furanones has been extensively explored.
Inhibition and Activation of QS Systems in Gram-Negative Bacteria
Inhibition of QS Systems
Currently, interference with the QS systems is primarily achieved by inhibiting the synthesis of autoinducers, degrading autoinducers, interfering with autoinducer receptors, or inhibiting the autoinducer/receptor complex formation (Figure 3; Lade et al., 2014). The molecules responsible for inhibition of autoinducer-induced QS systems or the autoinducer-regulated phenotype are called quorum-sensing inhibitors (QSIs). QSIs include furanones and their related structural analogs (Figure 2E; Zang et al., 2009; Liu et al., 2010; Steenackers et al., 2010), bismuth porphyrin complexes (Galkin et al., 2015), glycosylation reagents of glycosylated flavonoids (Figure 2F; Brango-Vanegas et al., 2015), and glycomonoterpenols (Mukherji and Prabhune, 2015), heavy metals (Vega et al., 2014) and nanomaterials (Wagh Nee Jagtap et al., 2013; Miller et al., 2015; Singh et al., 2015). The inhibitory effect of furanones is primarily due to their structural similarity to AHLs, but some case studies also showed that furanones may function through degrading the LuxR-type protein (Manefield et al., 2002) or decreasing the DNA-binding activity of the transcriptional regulator protein LuxR (Defoirdt et al., 2007b). In addition to AHL autoinducers, furanones also disrupt the AI-2 biosynthetic pathway by covalently modifying and inactivating LuxS (Zang et al., 2009). In addition to furanones, allyl benzo[b]thiophene-3-carboxylate 1,1-dioxide (Figure 2G) targeted LuxPQ (Zhu et al., 2012), thiazolidinediones and dioxazaborocane targeted LuxR in V. harveyi (Brackman et al., 2013) were also reported to be AI-2 inhibitors. Alkylamine-modified cyclodextrins (Morohoshi et al., 2013) and homoserine lactone (HSL) aptamer (Figure 2H; Zhao et al., 2013) inhibited the QS system through the formation of spatial conformations, whereas silicon dioxide nanoparticles functionalized with β-cyclodextrin reduced the luminous output of V. fischeri through binding of AHLs to the nanoparticles and removal of the AHLs from the immediate bacterial environment (Miller et al., 2015). Recently, ureidothiophene-2-carboxylic acids were described as inhibitors of the PQS-QS enzyme PqsD in P. aeruginosa (Sahner et al., 2015).
In addition to small molecule QSIs, enzymatic degradation is an alternative approach to inhibit bacterial signaling molecules. AHL-lactonases and decarboxylases hydrolyze lactone rings, whereas AHL-acylases and deaminases cleave the acyl side chain (Figure 4; Amara et al., 2011). Acylases that hydrolyze the amide moieties of AHLs have been identified in Streptomyces sp. (Park et al., 2005), P. aeruginosa (Sio et al., 2006), Comamonas (Uroz et al., 2007), Pseudomonas syringae (Shepherd and Lindow, 2009), Streptomyces sp. (Ueda et al., 2010), Bacillus cereus (Sunder et al., 2012), and Kluyvera citrophila (Mukherji et al., 2014). Metallo-beta-lactamases hydrolyze the core lactone ring of AHL signaling molecules and are noted in Acinetobacter sp. (Kang et al., 2004), Rhodococcus sp. (Park et al., 2006; Uroz et al., 2008), Agrobacterium tumefaciens (Liu et al., 2007) and Chryseobacterium sp. (Wang et al., 2012). Oxidoreductases from Rhodococcus erythropolis (Uroz et al., 2005) and Bacillus megaterium (Chowdhary et al., 2007) target the keto group of 3-oxo-HSLs or the acyl side chain itself. Immobilized enzymes on solid materials, such as magnetic enzyme carriers (MECs) (Yeon et al., 2009b; Lee et al., 2014), nanofiltration (NF) (Kim et al., 2011), microporous hollow fiber membranes (Jahangir et al., 2012; Oh et al., 2012), and encapsulation of QQ bacteria Rhodococcus sp. (Oh et al., 2012; Kim et al., 2013), have been used to inhibit QS signaling systems.
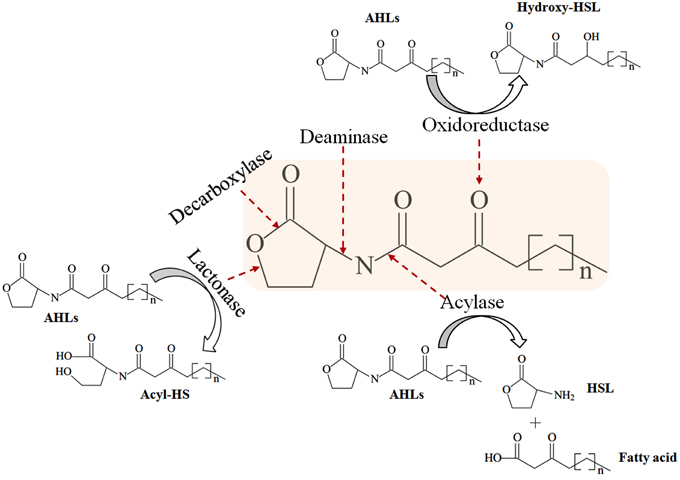
Figure 4. Modes of AHL-degrading enzymes and their catalytic sites. Revised from Chen et al. (2013).
Activation of QS Systems
Compared with the well-studied QSIs, research on activation of QS is in its infancy. Agonistic analogs of QS signaling molecules were believed to trigger the activation of the host defense system and thus allow resistance to develop via activation of virulence factors at low population densities (Defoirdt et al., 2004). N-(3-nitro-phenylacetanoyl)-L-homoserine lactone (Figure 2I) was one of the first reported super-agonists of AHLs to trigger bioluminescence in V. fischeri, increasing activity relative to native AHLs (Geske et al., 2007a,b). In the non-AHL-producing Salmonella enterica serovar Typhimurium, the LuxR homolog SdiA was activated by N-(3-oxo-acyl)-homocysteine thiolactones (3O-AHTLs, Figure 2J) and N-(3-oxo-acyl)-trans-2-aminocyclohexanols (Figure 2K) at concentrations lower than those of most active AHLs (Janssens et al., 2007). Naphthyl-DPD (Figure 2L), the agonistic analog of AI-2 precursor DPD, is an efficient synergistic agonist in V. harveyi even at low nanomolar concentrations. The synergistic effect is based on binding of one DPD unit to one LuxPQ domain and binding of naphthyl-DPD to the second LuxPQ domain, allowing a favorable conformational change that leads to early induction of QS activity (Mandabi et al., 2015). Chemicals such as (4S,5R)-dihydroxyhexanediones (DHDs, Figure 2M) is not only a synergistic agonist for the LsrB receptor in E. coli but also an agonist in V. harveyi with a LuxP receptor, displaying an EC50 = 0.65 μM. This activity is the strongest agonistic activity reported thus far in V. harveyi (Rui et al., 2012).
Regulation of QS Systems by Sub-lethal Antibiotics
Interestingly, sub-lethal antibiotics also function as QS system mediators. Azithromycin, ceftazidime, and ciprofloxacin (CPR) reduce the production of the AHLs, most likely due to the changes in membrane permeability in the presence of antibiotics that decreased flux of AHLs and expression of QS-regulated genes (Skindersoe et al., 2008). Similarly, streptomycin at sub-lethal concentrations reduced 3-OH-C12-HSL levels through the down-regulation of abaI and A1S_0112 from Acinetobacter baumannii (Niu et al., 2008; Clemmer et al., 2011; Saroj and Rather, 2013). Aspirin at sub-lethal concentrations is an efficient inhibitor of QS, virulence, and toxins in P. aeruginosa via the interaction between the aspirin aryl group and Tyr-88 of the LasR receptor by strong π–π stacking interactions (El-Mowafy et al., 2014). However, some antibiotics, such as CPR, metronidazole, and tinidazole, exhibited a dose-dependent augmentation in response to QS systems, exhibiting an AHL-like effect. Alternatively, at the concentrations tested, these antibiotics may themselves act as QS signaling molecules (Struss et al., 2012). The contradictory effect of CPR on QS systems may be due to the different reporter cells used, e.g., P. aeruginosa PAO1 (Skindersoe et al., 2008) and E. coli expressing P. aeruginosa QS systems (Struss et al., 2012).
Quorum Sensing and Environmentally Related Processes
Quorum Sensing and Wastewater Treatment Systems
In wastewater treatment systems, one critical step involves removal of organic pollutants by biological treatments based on the microbial decomposition of pollutants into small molecules with less or even no toxicity, which simultaneously generates energy for microbial metabolism and the building blocks for cell synthesis. This method is now considered an essential step in wastewater treatment system and has been classified into two primary groups: fixed-film systems and activated sludge systems (Feng et al., 2013). QS systems are involved in the following aspects of these processes.
Involvement in Biofilm Formation
Biofilms consist of bacterial cells surrounded by an extracellular matrix consisting of secreted proteins, polysaccharides, nucleic acids, and dead cells; biofilm development progresses through the stages of initial attachment, irreversible attachment, maturation I, and maturation II (Figure 3; Parsek and Greenberg, 2005). AHLs freely diffuse in the microenvironment and are involved in biofilm formation in P. aeruginosa (Davies et al., 1998), Pantoea stewartii (Koutsoudis et al., 2006), Acinetobacter sp. (Kang and Park, 2010), and Serratia plymuthica (Liu et al., 2011). In P. aeruginosa, the las QS system regulates biofilm formation through control of the pel operon that encodes the glucose-rich matrix exopolysaccharide, a component of the biofilm matrix (Davies et al., 1998; Sakuragi and Kolter, 2007). However, exogenous 3-oxo-octanoyl-L-homoserine lactone (C8-oxo-HSL) increased the growth rate of P. aeruginosa cells on an ultra-filtration membrane biofilm without influencing the production of extracellular polymeric substances (EPS, Xia et al., 2012). The correlation between QS and EPS production in a growing biofilm under various conditions was modeled, illustrating the benefits of QS regulation by developing a thick, protective layer of EPS or by clogging the environment with biomass to secure a nutrient supply and outcompete other colonies (Frederick et al., 2011).
In the natural environment, biofilm formation by microorganisms can be beneficial or detrimental from the human perspective. Biofilms formed by degrading microorganisms are generally used to remove hydrocarbons, heavy metals, and nutrients to improve the mineralization process, as they offer a sessile, protective environment with optimal pH, solute concentrations and redox potential (Singh et al., 2006). Aerobic granules cultivated in sequencing batch reactors (SBRs) for biological wastewater treatment are considered a special type of biofilm and share many features of biofilm systems (Liu and Tay, 2002; Yang et al., 2004). Aerobic granules possess the merits of high stability and flexibility, low energy requirements, reduced footprint, aerobic, and anoxic zones inside the granules, and reduced investment and operational costs in practical applications compared with conventional activated sludge (Aqeel et al., 2015; Long et al., 2015; Pronk et al., 2015; Wagner et al., 2015). In aerobic granules, a gradient microbial population of aerobic bacteria, anaerobic bacteria, and dead microbial cells under the granule surface co-exists due to the presence of oxygen and nutrient gradients (Figure 5; Liu et al., 2002). AHLs produced by some of these bacteria, e.g., Aeromonas sp., Pseudomonas sp. and Acinetobacter sp., were detected (Valle et al., 2004; Morgan-Sagastume et al., 2005; Chong et al., 2012; Yong and Zhong, 2013b). Both the autoinducers of AHL and AI-2 were observed in the biomass during the period of aerobic granulation, and this phenomenon was frequently ATP-dependent (Xiong and Liu, 2010; Zhang et al., 2011; Jiang and Liu, 2013; Wang et al., 2014). AHLs at picomolar to nanomolar concentrations are strongly and mostly positively correlated with the initiation of granulation, formation of highly structured granules, and maintenance of granular structure in the granulation ecosystem.
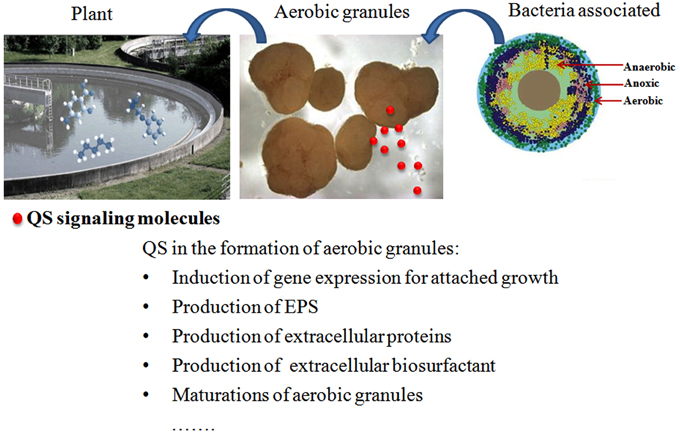
Figure 5. Schematic diagrams of aerobic granulation, attached bacteria, and function of QS signaling molecules.
Mature granules or their extracts induced the bacterial attached-growth state with a high adhesion capability, contributing to the initial cell attachment and subsequent biofilm growth on a Flow-Cell cover slide, thus accelerating the formation of aerobic granules from conventional activated sludge (Ren et al., 2010, 2013). This effect may be attributed to the increased activity of AHL signaling molecules contained in the mature granules or their extracts (Li et al., 2014). The correlation between QS signaling molecules and the production of EPS, a significant factor promoting the maintenance of aerobic granular structures during the development of aerobic granules and biofilm growth, was confirmed (Jiang and Liu, 2012; Li et al., 2014; Tan et al., 2014). AHLs also regulated the production of extracellular biosurfactants (or act as biosurfactants) (Daniels et al., 2006) and extracellular proteins (Lv et al., 2014a). The involvement of QS in the formation of aerobic granules was also confirmed using quorum quenching (QQ). A high rate of QQ activity was presented in the floccular sludge. However, when the floccular biomass was transformed into granular sludge, the QQ activity of the community was reduced by 30%, whereas the levels of C4–C8 AHLs associated with the initiation of granulation increased up to 10- to 100-fold. However, when the granule disintegrates, C6-HSL, and C8-HSL levels decreased with the reduced sludge particle size (Tan et al., 2014, 2015).
Biofouling is an adverse effect of the biofilm that forms by the attachment of microorganisms on the surface of various solid matrixes. This condition is the primary hindrance during the development and application of membrane bioreactors (MBR) and consumes 60% of MBR operating fees (Drews, 2010). AHL autoinducers from C4-HSL to C14-HSL (Kim et al., 2009, 2013; Yeon et al., 2009a; Cuadrado-Silva et al., 2013) as well as AI-2 (Davies et al., 1998; Xu and Liu, 2010) have frequently been detected during biofouling in the MBR environment. Furthermore, a positive correlation between AHLs and membrane biofouling was confirmed because the strong AHL activity corresponded to an abrupt increase in the transmembrane pressure (TMP) in a fouled MBR (Yeon et al., 2009a). The presence of autoinducers in the biofilm and the relationship between autoinducers and biofilm formation phenotype, TMP and EPS measurements suggest alternative strategies for controlling membrane biofouling via interference of the autoinducer-mediated QS systems. In fact, the activation and inhibition of QS systems are two antagonistic coexisting processes among various bacterial communities in different wastewater treatment systems (Song et al., 2014). Chemicals, such as 5-hydroxy- 3[(1R)-1-hydroxypropyl]-4-methylfuran-2(5H)-one, (5R)-3,4-dihydroxy-5-[(1S)- 1,2-dihydroxyethyl]furan-2(5H)-one, kojic acid (Dobretsov et al., 2007, 2011), and non-halogenated 2(5H)-furanone (Ponnusamy et al., 2010), are QSIs that control the formation of biofilms. Given the toxicity of furanones, non-toxic agents were preferred alternative strategies to control biofouling, which may mitigate membrane biofouling without disturbing bacterial growth. Vanillin was reported to reduce the production of AHL autoinducers and decrease the biofilm formation of Aeromonas hydrophila on a membrane at very low concentrations (Ponnusamy et al., 2009, 2013). Further application of vanillin to a reverse osmosis membrane in a CDC reactor exhibited 97% biofilm reduction (Kappachery et al., 2010). D-tyrosine significantly reduced the microbial attachment on a solid matrix through its inhibitory effect on the synthesis of AI-2, eDNA and extracellular polysaccharides and proteins (Xu and Liu, 2011). In addition to small chemicals, acylases are also used to reduce the biofouling from fouled reverse osmosis membranes (Paul et al., 2009; Yeon et al., 2009a; Kim et al., 2012). To overcome the limitations of a short half-life and low efficiency using free enzymes, immobilized acylase bound to magnetic particles was used to inactivate the AHL autoinducers to reduce biofilm formation and enhance membrane permeability (Yeon et al., 2009b). Alternatively, more feasible and economic methods based on the encapsulation of Rhodococcus sp. BH4 and recombinant E. coli-producing AHL-lactonase in microporous membranes were developed. The biofilm formed on the membrane in the microbial vessel reactor was approximately half of the total attached biomass and thinner and sparser compared with that in the control reactor (Oh et al., 2012). Lactonase from Rhodococcus sp. BH4 is responsible for the degradation of a wide range of AHLs and thus inhibition of biofouling (Oh et al., 2013). To overcome the limitation of transference of mass and AHLs from the mixed liquor to the microbial vessel encapsulated Rhodococcus sp. BH4, cell entrapping beads (CEB), i.e., moving beads of alginate containing Rhodococcus sp. BH4, were developed with a better capacity to mitigate biofouling. In MBRs, the time to achieve a TMP of 70 kPa in the presence of CEBs was 10-fold longer than that in the absence of CEBs (Kim et al., 2013). Another macrocapsule consisting of the membrane coating layer and alginate core with Rhodococcus sp. BH4 was developed, and it decreased to half the total amount of biocakes (Kim et al., 2015). Pseudomonas sp. 1A1 probably producing AHL-acylase to degrade AHLs were also encapsulated in the ceramic microbial vessel, leading to significantly reduced polysaccharides and proteins (Cheong et al., 2013, 2014). However, although disruption of QS systems has been employed to mitigate biofouling, the above findings have yet to be used in practice.
Optimization of the Composition of the Bacterial Community
It is generally accepted that removal of different pollutants by microorganisms is dominated by the percentage of and interaction between different microbes. In activated sludge communities from industrial wastewater treatment systems, a dominant functional member of the Thauera genus was transiently supplanted by a member of the Comamonas genus in response to the exogenous AHL. In addition, phenol degradation rates were restored to the original higher levels (Valle et al., 2004). In another long-term operated bioreactor, greater than 50% of the top 50 most abundant community members exhibited a strong positive correlation with at least one AHL and granulation (Tan et al., 2014). Xanthomonadaceae (Tan et al., 2014) and Flavobacterium (Lv et al., 2014b) in aerobic granules correlated with increased AHLs content. In contrast, an AHL signal degrader from the Comamonadaceae family exhibited a negative correlation with both the AHL concentration and granulation (Tan et al., 2014).
Strengthen the Biodegradation/Biotransformation Pathways in Gram-Negative Bacteria
QS bacteria can degrade or transform phenol (Valle et al., 2004; Yong and Zhong, 2010), hexadecane (Kang and Park, 2010), phenanthrene, or pyrene (Huang et al., 2013), nitrogen (Toyofuku et al., 2007, 2008; De Clippeleir et al., 2011) and total organic carbon (Zhang et al., 2015). The first evidence demonstrating AHL involvement in biodegradation was observed in phenol degradation by activated sludge. The addition of 2 μM AHLs could maintain the phenol biodegradation ability for a longer period. However, this work did not present any evidence for the QS effect on bacterial biodegradation or pollutant metabolism (Valle et al., 2004). A more detailed study examined the RhlI/R QS system in a P. aeruginosa organic pollutant degrader from industrial and municipal wastewater and its involvement in biodegradation and denitrification. Mutants of △rhlI and △rhlR exhibited increased denitrification activity (Toyofuku et al., 2007) and reduced phenol degradation (Yong and Zhong, 2010, 2013b), and the exogenous addition of the cognate AHL molecules or AHL extracts induced the QS mutant strains to exhibit denitrification activity similar to the wild-type strain. The repression of mutant ΔrhlI but not mutant ΔrhlIR could also be relieved by supplementation with AHL extracts or synthetic C4-HSL. These data supplied concrete evidence to demonstrate the involvement of the QS system in biodegradation (Yong and Zhong, 2010, 2013b). abaI encodes the autoinducer synthases of the LuxI family in another important aromatic compound degrader, Acinetobacter sp. (Smith et al., 2007; Niu et al., 2008; Anbazhagan et al., 2012), which is also involved in biofilm formation and hexadecane biodegradation. Its activity is enhanced by adding exogenous 3-OH-C12-HSL (Kang and Park, 2010). However, in another case study in activated sludge, short chain AHLs were responsible for nicotine degradation and had an increased effect on Acinetobacter sp. TW colonization, whereas long chain AHLs are secreted and contribute to resistance to the toxin nicotine (Montgomery et al., 2013; Wang et al., 2013a,b).
The expression and activity of enzymes involved in biodegradation are regulated by autoinducers and QS systems. During the degradation of anthranilate and phenol by P. aeruginosa, expression of catechol-1,2-dioxygenase (C12O) and catechol 2,3-dioxygenase (C23O) was significantly enhanced by the addition of N-decanoyl-L-homoserine lactone (C10-HSL), N-octanoyl-L-homoserine lactone (C8-HSL) or C4-HSL (Chugani and Greenberg, 2010) through the rhlI/R QS system (Yong and Zhong, 2013b), respectively. In denitrification, the rhlI/R QS system repressed the promoter activities of nark1, nirS, norC, and nosR and thus the corresponding activities of reductase, reductase, and NO reductase were higher in the mutant of △rhlR (Toyofuku et al., 2007). Moreover, the reduction of NO reductase and reductase and induction of reductase at the post-transcriptional level are also regulated by the PQS-based QS system in P. aeruginosa (Toyofuku et al., 2008).
Quorum Sensing and Aquaculture
QS systems were extensively confirmed to be involved in regulation of virulence of pathogens in aquaculture. Virulence factors of the opportunistic pathogen V. harveyi, including extracellular toxin (Manefield et al., 2000), siderophore (Lilley and Bassler, 2000), metalloprotease (Mok et al., 2003), type III secretion system (Henke and Bassler, 2004), phospholipase, caseinase, and gelatinase (Natrah et al., 2011b), are regulated by QS systems. In a number of other Vibrio species, such as V. cholerae, V. vulnificus, V. anguillarum, V. splendidus, V. aestuarianus, and V. vulnificus, the expression of metalloproteases is also modulated by QS (Decker et al., 2013; Ha et al., 2014). More direct evidence revealed that attenuation of QS in pathogenic Aeromonas spp. and V. campbellii resulted in significantly reduced mortality toward their respective hosts burbot (Natrah et al., 2012), larvae of brine shrimp and giant freshwater prawn (Pande et al., 2013).
Due to the close association between the QS system and virulence of aquatic pathogens, ecological strategies are the preferred option to overcome the problems of acquisition of antibiotic resistance and the spread of resistant genes when antibiotics or disinfectants are used to treat bacterial diseases (Homem and Santos, 2011; Singh, 2015). QSIs, such as the natural furanones of (5Z)-4-bromo-5-(bromomethykene)-3-butyl-2(5H)-furanone (Figure 2E; Defoirdt et al., 2006, 2007a,b), halogenated furanones (Zang et al., 2009), furanones F2 (Liu et al., 2010), brominated 3-alkyl-5-methylene-2(5H)-furanones, and alkylmaleic anhydrides (Steenackers et al., 2010), and other chemicals of thiophenone (Z)-4-((5-(bromomethylene)-2-oxo-2,5-dihydrothiophen-3-yl)-4-oxobutanoic acid) (Figure 2N; Defoirdt et al., 2012; Pande et al., 2013), are effective in attenuating bacterial virulence. Because the therapeutic index of furanones is likely too low to be used in the aquaculture given their relatively high toxicity to higher organisms (Defoirdt et al., 2006; Pande et al., 2013), non-toxic natural compounds were explored. Cinnamaldehyde (Figure 2O), a QS-disrupting compound, protects burbot (Lota lota L.) larvae from A. hydrophila and A. salmonicida (Natrah et al., 2012), the giant freshwater prawn Macrobrachium rosenbergii from V. harveyi (Pande et al., 2013) and brine shrimp larvae from V. harveyi (Brackman et al., 2013). Coumarin (Figure 2P) was effective in reducing the biofilm formation of E. tarda, V. anguillarum, E. coli, and Staphylococcus aureus. The above four strains use three types of autoinducers, e.g., AHLs, AI-2, and agr, suggesting that coumarin could be used as a universal QS inhibitor to attenuate bacterial disease in aquaculture (Gutiérrez-Barranquero et al., 2015). The trend of using probiotic microorganisms to control disease in aquaculture is encouraging, as they can disrupt the QS systems of pathogens. The positive effect of AHL-degrading Bacillus sp. may result from the degradation of autoinducers in addition to the production of growth-inhibiting substances (Defoirdt et al., 2004). The microalgae Chlorella saccharophila CCAP211/48, which is commonly used in aquaculture, exhibited stable inhibitory activity on V. harveyi with the production of QS antagonistic metabolites that have not been reported previously in microalgae (Natrah et al., 2011a).
Opportunities and Challenges
QS systems dynamically control gene expression in a cell density-dependent manner, based on the production, secretion, and detection of autoinducers. The concentration of autoinducers increases with the cell density. Once a threshold level is achieved, autoinducers induce the expression of QS-dependent target genes to facilitate environmental adaptation. As a global gene regulatory network, autoinducer-induced QS systems are involved in the regulation of bacterial virulence, conjugative plasmid transfer, sporulation, biofilm formation, antimicrobial peptide synthesis, and symbiosis. Future studies and applications of autoinducer-induced QS systems may focus on the following aspects.
• Although increasing attention has been paid to the ecological applications of QS regulation of biofilms, the mechanism by which bacterial autoinducer-induced QS systems operate in the “city” of biofilms remains rudimentary. A clear understanding is required to explore how the biofilm influences the initial QS system and subsequently the QS-regulated gene expression profiles and the development of multi-species biofilms.
• The QS system plays an important role in biodegradation. Thus, QS manipulation may become increasingly important in biodegradation and environmental bioremediation at the population/community and molecular level. However, QS systems have not gained the attention that they deserve in biodegradation compared with their function in many other biological processes. Further, study of the QS systems in biodegradation may accelerate the speed and rate of pollutant degradation. Additionally, the improvement of biodegradation efficiency by engineering the QS system is in progress and is a strategy that deserves more attention in the future. Moreover, the aromatic degradation pathway is a source of new signaling molecules and/or QQ signaling molecules. Thus, QS regulation of aromatic degradation processes and the latter as sources of signaling molecules are also notable topics.
• The design of novel antimicrobial agents based on QS regulation to control bacterial infections is recommended, despite that it will likely be many years before clinically safe and effective QSIs are available for real-life applications. Considering the fact that microbial consortia are composed of different species and a wide range of QS-regulated gene expression, it should be noted that the entire QS-regulated network would not be affected by the QSI of a particular QS pathway.
Developments in the field of QS and the QS-regulated behaviors in the natural environment and host will clearly address these questions. The answers to these open questions will undoubtedly bring new insights and surprises.
Funding
This work was financially supported by the National Natural Science Foundation of China (NSFC) grant 31200041, the Zhejiang Provincial Natural Science Foundation of China (LR14C190001), the Program of Science and Technology of Ningbo city of China (2015C50057), the Young Academic Leaders in Colleges and Universities in Zhejiang Province (pd2013099), the Zhejiang Open Foundation of the Most Important Subjects (xkzsc1408), and the K. C. Wong Magna Fund in Ningbo University.
Conflict of Interest Statement
The authors declare that the research was conducted in the absence of any commercial or financial relationships that could be construed as a potential conflict of interest.
References
Ahmer, B. M., van Reeuwijk, J., Timmers, C. D., Valentine, P. J., and Heffron, F. (1998). Salmonella typhimurium encodes an SdiA homolog, a putative quorum sensor of the LuxR family, that regulates genes on the virulence plasmid. J. Bacteriol. 180, 1185–1193.
Amara, N., Krom, B. P., Kaufmann, G. F., and Meijler, M. M. (2011). Macromolecular inhibition of quorum sensing: enzymes, antibodies, and beyond. Chem. Rev. 111, 195–208. doi: 10.1021/cr100101c
Anbazhagan, D., Mansor, M., Yan, G. O., Md Yusof, M. Y., Hassan, H., and Sekaran, S. D. (2012). Detection of quorum sensing signal molecules and identification of an autoinducer synthase gene among biofilm forming clinical isolates of Acinetobacter spp. PLoS ONE 7:e36696. doi: 10.1371/journal.pone.0036696
Aqeel, H., Basuvaraj, M., Hall, M., Neufeld, J. D., and Liss, S. N. (2015). Microbial dynamics and properties of aerobic granules developed in a laboratory-scale sequencing batch reactor with an intermediate filamentous bulking stage. Appl. Microbiol. Biotechnol. doi: 10.1007/s00253-015-6981-7. [Epub ahead of print].
Bala, A., Chhibber, S., and Harjai, K. (2014). Pseudomonas quinolone signalling system: a component of quorum sensing cascade is a crucial player in the acute urinary tract infection caused by Pseudomonas aeruginosa. Int. J. Med. Microbiol. 304, 1199–1208. doi: 10.1016/j.ijmm.2014.08.013
Bala, A., Gupta, R. K., Chhibber, S., and Harjai, K. (2013). Detection and quantification of quinolone signalling molecule: a third quorum sensing molecule of Pseudomonas aeruginosa by high performance-thin layer chromatography. J. Chromatogr. B. 930, 30–35. doi: 10.1016/j.jchromb.2013.04.027
Bhardwaj, A. K., Vinothkumar, K., and Rajpara, N. (2013). Bacterial quorum sensing inhibitors: attractive alternatives for control of infectious pathogens showing multiple drug resistance. Recent. Pat. Antiinfect. Drug. Discov. 8, 68–83. doi: 10.2174/1574891X11308010012
Biradar, B., and Devi, P. (2011). Quorum sensing in plaque biofilms: challenges and future prospects. J. Contemp. Dent. Pract. 12, 479–485. doi: 10.5005/jp-journals-10024-1080
Bortolotti, D., LeMaoult, J., Trapella, C., Di Luca, D., Carosella, E. D., and Rizzo, R. (2015). Pseudomonas aeruginosa quorum sensing molecule N-(3-Oxododecanoyl)-l-Homoserine-Lactone induces HLA-G expression in human immune cells. Infect. Immun. 83, 3918–3925. doi: 10.1128/IAI.00803-15
Brackman, G., Al Quntar, A. A., Enk, C. D., Karalic, I., Nelis, H. J., van Calenbergh, S., et al. (2013). Synthesis and evaluation of thiazolidinedione and dioxazaborocane analogues as inhibitors of AI-2 quorum sensing in Vibrio harveyi. Bioorg. Med. Chem. 21, 660–667. doi: 10.1016/j.bmc.2012.11.055
Brameyer, S., Kresovic, D., Bode, H. B., and Heermann, R. (2015). Dialkylresorcinols as bacterial signaling molecules. Proc. Natl. Acad. Sci. U.S.A. 112, 572–577. doi: 10.1073/pnas.1417685112
Brango-Vanegas, J., Costa, G. M., Ortmann, C. F., Schenkel, E. P., Reginatto, F. H., Ramos, F. A., et al. (2015). Glycosylflavonoids from Cecropia pachystachya Trécul are quorum sensing inhibitors. Phytomedicine 21, 670–675. doi: 10.1016/j.phymed.2014.01.001
Chen, F., Gao, Y., Chen, X., Yu, Z., and Li, X. (2013). Quorum quenching enzymes and their application in degrading signal molecules to block quorum sensing-dependent infection. Int. J. Mol. Sci. 14, 17477–17500. doi: 10.3390/ijms140917477
Cheong, W. S., Lee, C. H., Moon, Y. H., Oh, H. S., Kim, S. R., Lee, S. H., et al. (2013). Isolation and identification of indigenous quorum quenching bacteria, Pseudomonas sp. 1A1, for biofouling control in MBR. Ind. Eng. Chem. Res. 52, 10554–10560. doi: 10.1021/ie303146f
Cheong, W. S., Kim, S. R., Oh, H. S., Lee, S. H., Yeon, K. M., Lee, C. H., et al. (2014). Design of quorum quenching microbial vessel to enhance cell viability forbiofouling control in membrane bioreactor. J. Microbiol. Biotechnol. 24, 97–105. doi: 10.4014/jmb.1311.11008
Chong, G., Kimyon, O., Rice, S. A., Kjelleberg, S., and Manefield, M. (2012). The presence and role of bacterial quorum sensing in activated sludge. Microb. Biotechnol. 5, 621–633. doi: 10.1111/j.1751-7915.2012.00348.x
Chowdhary, P. K., Keshavan, N., Nguyen, H. Q., Peterson, J. A., González, J. E., and Haines, D. C. (2007). Bacillus megaterium CYP102A1 oxidation of acyl homoserine lactones and acyl homoserines. Biochemistry 46, 14429–14437. doi: 10.1021/bi701945j
Chugani, S., and Greenberg, E. P. (2010). LuxR homolog-independent gene regulation by acyl-homoserine lactones in Pseudomonas aeruginosa. Proc. Natl. Acad. Sci. U.S.A. 107, 10673–10678. doi: 10.1073/pnas.1005909107
Clarke, M. B., Hughes, D. T., Zhu, C., Boedeker, E. C., and Sperandio, V. (2006). The QseC sensor kinase: a bacterial adrenergic receptor. Proc. Natl. Acad. Sci. U.S.A. 103, 10420–10425. doi: 10.1073/pnas.0604343103
Clemmer, K. M., Bonomo, R. A., and Rather, P. N. (2011). Genetic analysis of surface motility in Acinetobacter baumannii. Microbiology 157, 2534–2544. doi: 10.1099/mic.0.049791-0
Cuadrado-Silva, C. T., Castellanos, L., Arévalo-Ferro, C., and Osorno, O. E. (2013). Detection of quorum sensing systems of bacteria isolated from fouled marine organisms. Biochem. Syst. Ecol. 46, 101–107. doi: 10.1016/j.bse.2012.09.010
Daniels, R., Reynaert, S., Hoekstra, H., Verreth, C., Janssens, J., Braeken, K., et al. (2006). Quorum signal molecules as biosurfactants affecting swarming in Rhizobiumetli. Proc. Natl. Acad. Sci. U.S.A. 103, 14965–14970. doi: 10.1073/pnas.0511037103
Davies, D. G., Parsek, M. R., Pearson, J. P., Iglewski, B. H., Costerton, J. W., and Greenberg, E. P. (1998). The involvement of cell-to-cell signals in the development of a bacterial biofilm. Science 280, 295–298. doi: 10.1126/science.280.5361.295
De Clippeleir, H., Defoirdt, T., Vanhaecke, L., Vlaeminck, S. E., Carballa, M., Verstraete, W., et al. (2011). Long-chain acyl homoserine lactones increase the anoxic ammonium oxidation rate in an OLAND biofilm. Appl. Microbiol. Biotechnol. 90, 1511–1519. doi: 10.1007/s00253-011-3177-7
Decker, S. D., Reynaud, Y., and Saulnier, D. (2013). First molecular evidence of cross-species induction of metalloprotease gene expression in Vibrio strains pathogenic for Pacific oyster Crassostrea gigas involving a quorum sensing system. Aquaculture 392, 1–7. doi: 10.1016/j.aquaculture.2013.01.033
Defoirdt, T., Benneche, T., Brackman, G., Coenye, T., Sorgeloos, P., and Scheie, A. A. (2012). A quorum sensing-disrupting brominated thiophenone with a promising therapeutic potential to treat luminescent vibriosis. PLoS ONE 7:e41788. doi: 10.1371/journal.pone.0041788
Defoirdt, T., Boon, N., Bossier, P., and Verstraete, W. (2004). Disruption of bacterial quorum sensing: an unexplored strategy to fight infections in aquaculture. Aquaculture 240, 69–88. doi: 10.1016/j.aquaculture.2004.06.031
Defoirdt, T., Boon, N., Sorgeloos, P., Verstraete, W., and Bossier, P. (2007a). Alternatives to antibiotics to control bacterial infections: luminescent vibriosis in aquaculture as an example. Trends Biotechnol. 25, 472–479. doi: 10.1016/j.tibtech.2007.08.001
Defoirdt, T., Boon, N., Sorgeloos, P., Verstraete, W., and Bossier, P. (2008). Quorum sensing and quorum quenching in Vibrio harveyi: lessons learned from in vivo work. ISME J. 2, 19–26. doi: 10.1038/ismej.2007.92
Defoirdt, T., Crab, R., Wood, T. K., Sorgeloos, P., Verstraete, W., and Bossier, P. (2006). Quorum sensing-disrupting brominated furanones protect the gnotobiotic brine shrimp Artemia franciscana from pathogenic Vibrio harveyi, Vibrio campbellii, and Vibrio parahaemolyticus isolates. Appl. Environ. Microbiol. 72, 6419–6423. doi: 10.1128/AEM.00753-06
Defoirdt, T., Miyamoto, C. M., Wood, T. K., Meighen, E. A., Sorgeloos, P., Verstraete, W., et al. (2007b). The natural furanone (5Z)-4-bromo-5-(bromomethylene)-3-butyl-2(5H)-furanone disrupts quorum sensing-regulated gene expression in Vibrio harveyi by decreasing the DNA-binding activity of the transcriptional regulator protein luxR. Environ. Microbiol. 9, 2486–2495. doi: 10.1111/j.1462-2920.2007.01367.x
Deng, Y., Wu, J., Eberl, L., and Zhang, L. H. (2010). Structural and functional characterization of diffusible signal factor family quorum-sensing signals produced by members of the Burkholderia cepacia complex. Appl. Environ. Microbiol. 76, 4675–4683. doi: 10.1128/AEM.00480-10
Di Cagno, R., De Angelis, M., Calasso, M., and Gobbetti, M. (2011). Proteomics of the bacterial cross-talk by quorum sensing. J. Proteomics 74, 19–34. doi: 10.1016/j.jprot.2010.09.003
Díaz, E., Jiménez, J. I., and Nogales, J. (2013). Aerobic degradation of aromatic compounds. Curr. Opin. Biotech. 24, 431–442. doi: 10.1016/j.copbio.2012.10.010
Dobretsov, S., Dahms, H. U., Huang, Y., Wahl, M., and Qian, P. Y. (2007). The effect of quorum-sensing blockers on the formation of marine microbial communities and larval attachment. FEMS Microbiol. Ecol. 60, 177–188. doi: 10.1111/j.1574-6941.2007.00285.x
Dobretsov, S., Teplitski, M., Bayer, M., Gunasekera, S., Proksch, P., and Paul, V. J. (2011). Inhibition of marine biofouling by bacterial quorum sensing inhibitors. Biofouling 27, 893–905. doi: 10.1080/08927014.2011.609616
Drews, A. (2010). Membrane fouling in membrane bioreactors-Characterisation, contradictions, cause and cures. J. Membr. Sci. 363, 1–28. doi: 10.1016/j.memsci.2010.06.046
El-Mowafy, S. A., Abd El Galil, K. H., El-Messery, S. M., and Shaaban, M. I. (2014). Aspirin is an efficient inhibitor of quorum sensing, virulence and toxins in Pseudomonas aeruginosa. Microb. Pathog. 74, 25–32. doi: 10.1016/j.micpath.2014.07.008
Eberhard, A., Burlingame, A. L., Eberhard, C., Kenyon, G. L., Nealson, K. H., and Oppenheimer, N. J. (1981). Structural identification of autoinducer of Photobacterium fischer luciferase. Biochemistry 20, 2444–2449. doi: 10.1021/bi00512a013
Feng, L., Wu, Z. Y., and Yu, X. (2013). Quorum sensing in water and wastewater treatment biofilms. J. Environ. Biol. 43, 437–444.
Frederick, M. R., Kuttler, C., Hense, B. A., and Eberl, H. J. (2011). A mathematical model of quorum sensing regulated EPS production in biofilm communities. Theor. Biol. Med. Model. 8, 8–36. doi: 10.1186/1742-4682-8-8
Fuqua, W. C., Winans, S. C., and Greenberg, E. P. (1994). Quorum sensing in bacteria: the LuxR-LuxI family of cell density-responsive transcriptional regulators. J. Bacteriol. 176, 269–275.
Fuqua, C. (2006). The QscR quorum-sensing regulon of Pseudomonas aeruginosa: an orphan claims its identity. J. Bacteriol. 188, 3169–3171. doi: 10.1128/JB.188.9.3169-3171.2006
Galloway, W. R., Hodgkinson, J. T., Bowden, S. D., Welch, M., and Spring, D. R. (2011). Quorum sensing in Gram-negative bacteria: small-molecule modulation of AHL and Al-2 quorum sensing pathways. Chem. Rev. 111, 28–67. doi: 10.1021/cr100109t
Galloway, W. R., Hodgkinson, J. T., Bowden, S., Welch, M., and Spring, D. R. (2012). Applications of small molecule activators and inhibitors of quorum sensing in Gram-negative bacteria. Trends Microbiol. 20, 449–458. doi: 10.1016/j.tim.2012.06.003
Galkin, M., Ivanitsia, V., Ishkov, Y., Galkin, B., and Filipova, T. (2015). Characteristics of the Pseudomonas aeruginosa PA01 intercellular signaling pathway (quorum sensing) functioning in presence of porphyrins bismuth complexes. Pol. J. Microbiol. 64, 101–106.
Geske, G. D., O'Neill, J. C., and Blackwell, H. E. (2007a). N-phenylacetanoyl-L-homoserine lactones can strongly antagonize or superagonize quorum sensing in Vibrio fischeri. ACS Chem. Biol. 2, 315–319. doi: 10.1021/cb700036x
Geske, G. D., O'Neill, J. C., Miller, D. M., Mattmann, M. E., and Blackwell, H. E. (2007b). Modulation of bacterial quorum sensing with synthetic ligands: systematic evaluation of N-acylated homoserine lactones in multiple species and new insights into their mechanisms of action. J. Am. Chem. Soc. 129, 13613–13625. doi: 10.1021/ja074135h
Geske, G. D., O'Neill, J. C., and Blackwell, H. E. (2008). Expanding dialogues: from natural autoinducers to non-natural analogues that modulate quorum sensing in Gram-negative bacteria. Chem. Soc. Rev. 37, 1432–1447. doi: 10.1039/b703021p
Givskov, M., de Nys, R., Manefield, M., Gram, L., Maximilien, R., Eberl, L., et al. (1996). Eukaryotic interference with homoserine lactone-mediated prokaryotic signalling. J. Bacteriol. 178, 6618–6622.
González, J. F., and Venturi, V. (2013). A novel widespread interkingdom signaling circuit. Trends Plant Sci. 18, 167–174. doi: 10.1016/j.tplants.2012.09.007
Gutiérrez-Barranquero, J. A., Reen, F. J., McCarthy, R. R., and O'Gara, F. (2015). Deciphering the role of coumarin as a novel quorum sensing inhibitor suppressing virulence phenotypes in bacterial pathogens. Appl. Microbiol. Biotechnol. 99, 3303–3316. doi: 10.1007/s00253-015-6436-1
Ha, C., Kim, S. K., Lee, M. N., and Lee, J. H. (2014). Quorum sensing-dependent metalloprotease VvpE is important in the virulence of Vibrio vulnificus to invertebrates. Microb. Pathog. 71–72, 8–14. doi: 10.1016/j.micpath.2014.04.001
Henke, J. M., and Bassler, B. L. (2004). Quorum sensing regulates type III secretion in Vibrio harveyi and Vibrio parahaemolyticus. J. Bacteriol. 186, 3794–3805. doi: 10.1128/JB.186.12.3794-3805.2004
He, Y. W., Wu, J., Zhou, L., Yang, F., He, Y. Q., Jiang, B. L., et al. (2011). Xanthomonas campestris diffusible factor is 3-hydroxybenzoic acid and is associated with xanthomonadin biosynthesis, cell viability, antioxidant activity, and systemic invasion. Mol. Plant Microbe Interact. 24, 948–957. doi: 10.1094/MPMI-02-11-0031
Higgins, D. A., Pomianek, M. E., Kraml, C. M., Taylor, R. K., Semmelhack, M. F., and Bassler, B. L. (2007). The major Vibrio cholerae autoinducer and its role in virulence factor production. Nature 450, 883–886. doi: 10.1038/nature06284
Hussain, M. B., Zhang, H. B., Xu, J. L., Liu, Q., Jiang, Z., and Zhang, L. H. (2008). The acyl-homoserine lactone-type quorum-sensing system modulates cell motility and virulence of Erwinia chrysanthemipv zeae. J. Bacteriol. 190, 1045–1053. doi: 10.1128/JB.01472-07
Holmes, K., Tavender, T. J., Winzer, K., Wells, J. M., and Hardie, K. R. (2009). AI-2 does not function as a quorum sensing molecule in Campylobacter jejuni during exponential growth in vitro. BMC Microbiol. 9:214. doi: 10.1186/1471-2180-9-214
Homem, V., and Santos, L. (2011). Degradation and removal methods of antibiotics from aqueous matrices-A review. J. Environ. Manage 92, 2304–2347. doi: 10.1016/j.jenvman.2011.05.023
Huang, Y., Zeng, Y., Yu, Z., Zhang, J., Feng, H., and Lin, X. (2013). In silico and experimental methods revealed highly diverse bacteria with quorum sensing and aromatics biodegradation systems–a potential broad application on bioremediation. Bioresour. Technol. 148, 311–316. doi: 10.1016/j.biortech.2013.08.155
Jahangir, D., Oh, H. S., Kim, S. R., Park, P. K., Lee, C. H., and Lee, J. K. (2012). Specific location of encapsulated quorum quenching bacteria for biofouling control in an external submerged membrane bioreactor. J. Membrane Sci. 411, 130–136. doi: 10.1016/j.memsci.2012.04.022
Jamuna Bai, A., and Ravishankar Rai, V. (2011). Bacterial quorum sensing and food industry. Compr. Rev. Food Sci. F. 10, 183–193. doi: 10.1111/j.1541-4337.2011.00150.x
Janssens, J. C. A., Metzger, K., Daniels, R., Ptacek, D., Verhoeven, T., Habel, L. W., et al. (2007). Synthesis of N-acyl homoserine lactone analogues reveals strong activators of SdiA, the Salmonella enterica serovar Typhimurium LuxR homologue. Appl. Environ. Microbiol. 73, 535–544. doi: 10.1128/AEM.01451-06
Jayaraman, A., and Wood, T. K. (2008). Bacterial quorum sensing: signals, circuits, and implications for biofilms and disease. Annu. Rev. Biomed. Eng. 10, 145–167. doi: 10.1146/annurev.bioeng.10.061807.160536
Jiang, B., and Liu, Y. (2012). Roles of ATP-dependent N-acylhomoserine lactones (AHLs) and extracellular polymeric substances (EPSs) in aerobic granulation. Chemosphere 88, 1058–1064. doi: 10.1016/j.chemosphere.2012.04.059
Jiang, B., and Liu, Y. (2013). Dependence of structure stability and integrity of aerobic granules on ATP and cell communication. Appl. Microbiol. Biotechnol. 97, 5105–5012. doi: 10.1007/s00253-012-4315-6
Joint, I., Tait, K., Callow, M. E., Callow, J. A., Milton, D., Williams, P., et al. (2002). Cell-to-cell communication across the prokaryote–eucaryote boundary. Science 298:1207. doi: 10.1126/science.1077075
Kalia, V. C. (2013). Quorum sensing inhibitors: an overview. Biotechnol. Adv. 31, 224–245. doi: 10.1016/j.biotechadv.2012.10.004
Kanamaru, K., Kanamaru, K., Tatsuno, I., Tobe, T., and Sasakawa, C. (2000). SdiA, an Escherichia coli homologue of quorum-sensing regulators, controls the expression of virulence factors in enterohaemorrhagic Escherichia coli O157:H7. Mol. Microbiol. 38, 805–816. doi: 10.1046/j.1365-2958.2000.02171.x
Kang, B. R., Lee, J. H., Ko, S. J., Lee, Y. H., Cha, J. S., Cho, B. H., et al. (2004). Degradation of acyl-homoserine lactone molecules by Acinetobacter sp. strain C1010. Can. J. Microbiol. 50, 935–941. doi: 10.1139/W04-083
Kang, Y. S., and Park, W. (2010). Contribution of quorum-sensing system to hexadecane degradation and biofilm formation in Acinetobacter sp. strain DR1. J. Appl. Microbiol. 109, 1650–1659. doi: 10.1111/j.1365-2672.2010.04793.x
Kappachery, S., Paul, D., Yoon, J., and Kweon, J. H. (2010). Vanillin, a potential agent toprevent biofouling of reverse osmosis membrane. Biofouling 26, 667–672. doi: 10.1080/08927014.2010.506573
Ke, X., Miller, L. C., and Bassler, B. L. (2015). Determinants governing ligand specificity of the Vibrio harveyi LuxN quoeum-sensing receptor. Mol. Microbiol. 95, 127–142. doi: 10.1111/mmi.12852
Koutsoudis, M. D., Tsaltas, D., Minogue, T. D., and von Bodman, S. B. (2006). Quorum-sensing regulation governs bacterial adhesion, biofilm development, and host colonization in Pantoea stewartii subspecies stewartii. Proc. Natl. Acad. Sci. U.S.A. 103, 5983–5988. doi: 10.1073/pnas.0509860103
Kim, J. H., Choi, D. C., Yeon, K. M., Kim, S. R., and Lee, C. H. (2011). Enzyme-immobilized nanofiltration membrane to mitigate biofouling based on quorum quenching. Environ. Sci. Technol. 45, 1601–1607. doi: 10.1021/es103483j
Kim, S. J., Lee, S. Y., Hong, S. K., Oh, Y. S., Seoul, M. J., Kweon, J. H., et al. (2009). Biofouling of reverse osmosis membranes: microbial quorum sensing and fouling propensity. Desalination 247, 303–315. doi: 10.1016/j.desal.2008.12.033
Kim, S. R., Lee, K. B., Kim, J. E., Won, Y. J., Yeon, K. M., Lee, C. H., et al. (2015). Macroencapsulation of quorum quenching bacteria by polymeric membrane layer and its application to MBR for biofouling control. J. Membrane Sci. 473, 109–117. doi: 10.1016/j.memsci.2014.09.009
Kim, M., Lee, S., Park, H. D., Choi, S. I., and Hong, S. (2012). Biofouling control by quorum sensing inhibition and its dependence on membrane surface. Water Sci. Technol. 66, 1424–1430. doi: 10.2166/wst.2012.307
Kim, S. R., Oh, H. S., Jo, S. J., Yeon, K. M., Lee, C. H., Lim, D. J., et al. (2013). Biofouling control with bead-entrapped quorum quenching bacteria in membrane bioreactors: physical and biological effects. Environ. Sci. Technol. 47, 836–842. doi: 10.1021/es303995s
Lade, H., Paul, D., and Kweon, J. H. (2014). Quorum quenching mediated approaches for control of membrane biofouling. Int. J. Biol. Sci. 10, 550–565. doi: 10.7150/ijbs.9028
LaSarre, B., and Federle, M. J. (2013). Exploiting quorum sensing to confuse bacterial pathogens. Microbiol. Mol. Biol. R. 77, 73–111. doi: 10.1128/MMBR.00046-12
Lee, B., Yeon, K. M., Shim, J., Kim, S. R., Lee, C. H., Lee, J., et al. (2014). Effective antifouling using quorum-quenching acylase stabilized in magnetically-separable mesoporous silica. Biomacromolecules 15, 1153–1159. doi: 10.1021/bm401595q
Lequette, Y., Lee, J. H., Ledgham, F., Lazdunski, A., and Greenberg, E. P. (2006). A distinct QscR regulon in the Pseudomonas aeruginosa quorum-sensing circuit. J. Bacteriol. 188, 3365–3370. doi: 10.1128/JB.188.9.3365-3370.2006
Li, X., Han, Y., Yang, Q., and Zhang, X. H. (2010). Detection of quorum sensing signal molecules and mutation of luxS gene in Vibrio ichthyoenteri. Res. Microbiol. 161, 51–57. doi: 10.1016/j.resmic.2009.10.004
Li, Y., Lv, J., Zhong, C., Hao, W., Wang, Y., and Zhu, J. (2014). Performance and role of N-acyl-homoserine lactone (AHL)-based quorum sensing (QS) in aerobic granules. J. Environ. Sci. 26, 1615–1621. doi: 10.1016/j.jes.2014.05.028
Lilley, B. N., and Bassler, B. L. (2000). Regulation of quorum sensing in Vibrio harveyi by LuxO and sigma-54. Mol. Microbiol. 36, 940–954. doi: 10.1046/j.1365-2958.2000.01913.x
Liu, W. T., Chan, O. C., and Fang, H. H. P. (2002). Characterization of microbial community in granular sludge treating brewery wastewater. Water Res. 36, 1767–1775. doi: 10.1016/S0043-1354(01)00377-3
Liu, H. B., Lee, J. H., Kim, J. S., and Park, S. (2010). Inhibitors of the Pseudomonas aeruginosa quorum-sensing regulator, QscR. Biotechnol Bioeng. 106, 119–126. doi: 10.1002/bit.22672
Liu, D., Thomas, P. W., Momb, J., Hoang, Q. Q., Petsko, G. A., Ringe, D., et al. (2007). Structure and specificity of a quorum-quenching lactonase (AiiB) from Agrobacterium tumefaciens. Biochemistry 46, 11789–11799. doi: 10.1021/bi7012849
Liu, X. G., Jia, J. L., Popat, R., Ortori, C. A., Li, J., Diggle, S. P., et al. (2011). Characterisation of two quorum sensing systems in the endophytic Serratia plymuthica strain G3: differential control of motility and biofilm formation according to life-style. BMC Microbiol. 11:26. doi: 10.1186/1471-2180-11-26
Liu, Y., and Tay, J. H. (2002). The essential role of hydrodynamic shear force in the formation of biofilm and granular sludge. Water Res. 36, 1653–1665. doi: 10.1016/S0043-1354(01)00379-7
Lobedanz, S., and Søgaard-Andersen, L. (2003). Identification of the C-signal, a contact-dependent morphogen coordinating multiple developmental responses in Myxococcus xanthus. Genes Dev. 17, 2151–2161. doi: 10.1101/gad.274203
Long, B., Yang, C. Z., Pu, W. H., Yang, J. K., Jiang, G. S., Dan, J. F., et al. (2015). The treatment of solvent recovery raffinate by aerobic granular sludge in a pilot-scale sequencing batch reactor. J. Water Health. 13, 746–757. doi: 10.2166/wh.2015.247
Lowery, C. A., Dickerson, T. J., and Janda, K. D. (2008). Interspecies and interkingdom communication mediated by bacterial quorum sensing. Chem. Soc. Rev. 37, 1337–1346. doi: 10.1039/b702781h
Lv, J., Wang, Y., Zhong, C., Li, Y., Hao, W., and Zhu, J. (2014a). The effect of quorum sensing and extracellular proteins on the microbial attachment of aerobic granular activated sludge. Bioresour. Technol. 152, 53–58. doi: 10.1016/j.biortech.2013.10.097
Lv, J., Wang, Y., Zhong, C., Li, Y., Hao, W., and Zhu, J. (2014b). The microbial attachment potential and quorum sensing measurement of aerobic granular activated sludge and flocculent activated sludge. Bioresour Technol. 151, 291–296. doi: 10.1016/j.biortech.2013.10.013
Mandabi, A., Ganin, H., and Meijler, M, M., (2015). Synergistic activation of quorum sensing in Vibrio harveyi. Bioorg. Med. Chem. Lett. 25, 3966–3969. doi: 10.1016/j.bmcl.2015.07.028
Manefield, M., Harris, L., Rice, S. A., de Nys, R., and Kjelleberg, S. (2000). Inhibition of luminescence and virulence in the black tiger prawn (Penaeus monodon) pathogen Vibrio harveyi by intercellular signal antagonists. Appl. Environ. Microbiol. 66, 2079–2084. doi: 10.1128/AEM.66.5.2079-2084.2000
Manefield, M., Rasmussen, T. B., Henzter, M., Andersen, J. B., Steinberg, P., Kjelleberg, S., et al. (2002). Halogenated furanones inhibit quorum sensing through accelerated LuxR turnover. Microbiology 148, 1119–1127. doi: 10.1099/00221287-148-4-1119
Marketon, M. M., Gronquist, M. R., Eberhard, A., and González, J. E. (2002). Characterization of the Sinorhizobium meliloti sinR/sinI locus and the production of novel N-acyl homoserine lactones. J. Bacteriol. 184, 205686–205695. doi: 10.1128/JB.184.20.5686-5695.2002
Miller, M. B., and Bassler, B. L. (2001). Quorum sensing in bacteria. Annu. Rev. Microbiol. 55, 165–199. doi: 10.1146/annurev.micro.55.1.165
Miller, K. P., Wang, L., Chen, Y. P., Pellechia, P. J., Benicewicz, B. C., and Decho, A. W. (2015). Engineering nanoparticles to silence bacterial communication. Front. Microbiol. 6:189. doi: 10.3389/fmicb.2015.00189
Mok, K. C., Wingreen, N. S., and Bassler, B. L. (2003). Vibrio harveyi quorum sensing: a coincidence detector for two autoinducers controls gene expression. EMBO J. 22, 870–881. doi: 10.1093/emboj/cdg085
Montgomery, K., Charlesworth, J. C., LeBard, R., Visscher, P. T., and Burn, B. P. (2013). Quorum sensing in extreme environments. Life 3, 131–148. doi: 10.3390/life3010131
Morgan-Sagastume, F., Boon, N., Dobbelaere, S., Defoirdt, T., and Verstraete, W. (2005). Production of acylated homoserine lactones by Aeromonas and Pseudomonas strains isolated from municipal activated sludge. Can. J. Microbiol. 51, 924–933. doi: 10.1139/w05-077
Morohoshi, T., Tokita, K., Ito, S., Saito, Y., Maeda, S., Kato, N., et al. (2013). Inhibition of quorum sensing in gram-negative bacteria by alkylamine-modified cyclodextrins. J. Biosci. Bioeng. 116, 175–179. doi: 10.1016/j.jbiosc.2013.01.022
Mukherji, R., and Prabhune, A. (2015). A new class of bacterial quorum sensing antagonists: glycomonoterpenols synthesized using linalool and alpha terpineol. World J. Microbiol. Biotechnol. 31, 841–849. doi: 10.1007/s11274-015-1822-5
Mukherji, R., Varshney, N. K., Panigrahi, P., Suresh, C. G., and Prabhune, A. (2014). A new role for penicillin acylases: degradation of acyl homoserine lactone quorum sensing signals by Kluyvera citrophila penicillin G acylase. Enzyme Microb. Technol. 56, 1–7. doi: 10.1016/j.enzmictec.2013.12.010
Nafee, N., Husari, A., Maurer, C. K., Lu, C., de Rossi, C., Steinbach, A., et al. (2014). Antibiotic-free nanotherapeutics: ultra-small, mucus-penetrating solid lipid nanoparticles enhance the pulmonary delivery and anti-virulence efficacy of novel quorum sensing inhibitors. J. Control. Release 192, 131–140. doi: 10.1016/j.jconrel.2014.06.055
Natrah, F. M., Alam, M. I., Pawar, S., Harzevili, A. S., Nevejan, N., Boon, N., et al. (2012). The impact of quorum sensing on the virulence of Aeromonas hydrophila and Aeromonas salmonicida towards burbot (Lota lota L.) larvae. Vet. Microbiol. 159, 77–82. doi: 10.1016/j.vetmic.2012.03.014
Natrah, F. M. I., Kenmegne, M. M., Wiyoto, W., Sorgeloos, P., Bossier, P., and Defoirdt, T. (2011a). Effects of micro-algae commonly used in aquaculture on acyl-homoserine lactone quorum sensing. Aquaculture 317, 53–57. doi: 10.1016/j.aquaculture.2011.04.038
Natrah, F. M., Ruwandeepika, H. A., Pawar, S., Karunasagar, I., Sorgeloos, P., Bossier, P., et al. (2011b). Regulation of virulence factors by quorum sensing in Vibrio harveyi. Vet. Microbiol. 154, 124–129. doi: 10.1016/j.vetmic.2011.06.024
Nealson, K. H., Platt, T., and Hastings, J. W. (1970). Cellular control of the synthesis and activity of the bacterial luminescent system. J. Bacteriol. 104, 313–322.
Neiditch, M. B., Federle, M. J., Miller, S. T., Bassler, B. L., and Hughson, F. M. (2005). Regulation of LuxPQ receptor activity by the quorum-sensing signal autoinducer-2. Mol. Cell 18, 507–518. doi: 10.1016/j.molcel.2005.04.020
Neiditch, M. B., Federle, M. J., Pompeani, A. J., Kelly, R. C., Swem, D. L., Jeffrey, P. D., et al. (2006). Ligand-induced asymmetry in histidine sensor kinase complex regulates quorum sensing. Cell 126, 1095–1108. doi: 10.1016/j.cell.2006.07.032
Nguyen, T., Roddick, F. A., and Fan, L. (2012). Biofouling of water treatment membranes: a review of the underlying causes, monitoring techniques and control measures. Membranes 2, 804–840. doi: 10.3390/membranes2040804
Niu, C., Clemmer, K. M., Bonomo, R. A., and Rather, P. N. (2008). Isolation and characterization of an autoinducer synthase from Acinetobacter baumannii. J. Bacteriol. 190, 3386–3392. doi: 10.1128/JB.01929-07
Nielsen, L. P., Risgaard-Petersen, N., Fossing, H., Christensen, P. B., and Sayama, M. (2010). Electric currents couple spatially separated biogeochemical processes in marine sediment. Nature 463, 1071–1074. doi: 10.1038/nature08790
Niu, C., Clemmer, K. M., Bonomo, R. A., and Rather, P. N. (2008). Isolation and characterization of an autoinducer synthase from Acinetobacter baumannii. J. Bacteriol. 190, 3386–3392. doi: 10.1128/JB.01929-07
Oh, H. S., Kim, S. R., Cheong, W. S., Lee, C. H., and Lee, J. K. (2013). Biofouling inhibition in MBR by Rhodococcus sp. BH4 isolated from real MBR plant. Appl. Microbiol. Biotechnol. 97, 10223–10231. doi: 10.1007/s00253-013-4933-7
Oh, H. S., Yeon, K. M., Yang, C. S., Kim, S. R., Lee, C. H., Park, S. Y., et al. (2012). Control of membrane biofouling in MBR for wastewater treatment by quorum quenching bacteria encapsulated in microporous membrane. Environ. Sci. Technol. 46, 4877–4884. doi: 10.1021/es204312u
Pande, G. S. J., Scheie, A. A., Benneche, T., Wille, M., Sorgeloos, P., Bossier, P., et al. (2013). Quorum sensing-disrupting compounds protect larvae of the giant freshwater prawn Macrobrachium rosenbergii from Vibrio harveyi infection. Aquaculture 406–407, 121–124. doi: 10.1016/j.aquaculture.2013.05.015
Park, S. Y., Kang, H. O., Jang, H. S., Lee, J. K., Koo, B. T., and Yum, D. Y. (2005). Identification of extracellular N-acylhomoserine lactone acylase from a Streptomyces sp. and its application to quorum quenching. Appl. Environ. Microbiol. 71, 2632–2641. doi: 10.1128/AEM.71.5.2632-2641.2005
Park, S. Y., Hwang, B. J., Shin, M. H., Kim, J., Kim, H. K., and Lee, J. K. (2006). N-acyl homoserine lactonase producing Rhodococcus spp. with different AHL−degrading activities. FEMS Microbiol. Lett. 261, 102–108. doi: 10.1111/j.1574-6968.2006.00336.x
Parsek, M. R., and Greenberg, E. P. (2005). Sociomicrobiology: the connections between quorum sensing and biofilms. Trends Microbiol. 13, 27–33. doi: 10.1016/j.tim.2004.11.007
Paul, D., Kim, Y. S., Ponnusamy, K., and Kweon, J. H. (2009). Application of quorum quenching to inhibit biofilm formation. Environ. Eng. Sci. 26, 1319–1324. doi: 10.1089/ees.2008.0392
Phelan, V. V., Liu, W. T., Pogliano, K., and Dorrestein, P. C. (2012). Microbial metabolic exchange–the chemotype-to-phenotype link. Nat. Chem. Biol. 8, 26–35. doi: 10.1038/nchembio.739
Ponnusamy, K., Paul, D., Kim, Y. s., and Kweon, J. H. (2010). 2(5H)-Furanone: a prospective strategy for biofouling-control in membrane biofilm bacteria by quorum sensing inhibition. Braz. J. Microbiol. 41, 227–234. doi: 10.1590/S1517-83822010000100032
Ponnusamy, K., Paul, D., and Kweon, J. H. (2009). Inhibition of quorum sensing mechanism and Aeromonas hydrophila biofilm formation by vanillin. Environ. Eng. Sci. 26, 1359–1363. doi: 10.1089/ees.2008.0415
Ponnusamy, K., Kappachery, S., Thekeettle, M., Song, J., and Kweon, J. (2013). Anti-biofouling property of vanillin on Aeromonas hydrophila initial biofilmon various membrane surfaces. World J. Microbiol. Biotechnol. 29, 1695–1703. doi: 10.1007/s11274-013-1332-2
Pronk, M., de Kreuk, M. K., de Bruin, B., Kamminga, P., Kleerebezem, R., and van Loosdrecht, M. C. (2015). Full scale performance of the aerobic granular sludge process for sewage treatment. Water Res. 84, 207–217. doi: 10.1016/j.watres.2015.07.011
Rampioni, G., Leoni, L., and Williams, P. (2014). The art of antibacterial warfare: deception through interference with quorum sensing–mediated communication. Bioorg. Chem. 55, 60–68. doi: 10.1016/j.bioorg.2014.04.005
Ren, T. T., Li, X. Y., and Yu, H. Q. (2013). Effect of N-acy-l-homoserine lactones-like molecules from aerobic granules on biofilm formation by Escherichia coli K12. Bioresour. Technol. 129, 655–658. doi: 10.1016/j.biortech.2012.12.043
Ren, T. T., Yu, H. Q., and Li, X. Y. (2010). The quorum-sensing effect of aerobic granules on bacterial adhesion, biofilm formation, and sludge granulation. Appl. Microbiol. Biotechnol. 88, 789–797. doi: 10.1007/s00253-010-2796-8
Ritchie, A. J., Jansson, A., Stallberg, J., Nilsson, P., Lysaght, P., and Cooley, M. A. (2005). The Pseudomonas aeruginosa quorum-sensing molecule N-3-(oxododecanoyl)-L-homoserine lactone inhibits T-cell differentiation and cytokine production by a mechanism involving an early step in T-cell activation. Infect Immun. 73, 1648–1655. doi: 10.1128/IAI.73.3.1648-1655.2005
Roy, V., Adams, B. L., and Bentley, W. E. (2011). Developing next generation antimicrobials by intercepting AI-2 mediated quorum sensing. Enzyme Microb. Tech. 49, 113–123. doi: 10.1016/j.enzmictec.2011.06.001
Rui, F., Marques, J. C., Miller, S. T., Maycock, C. D., Xavier, K. B., and Ventura, M. R. (2012). Stereochemical diversity of AI-2 analogs modulates quorum sensing in Vibrio harveyi and Escherichia coli. Bioorg. Med. Chem. 20, 249–256. doi: 10.1016/j.bmc.2011.11.007
Sahner, J. H., Empting, M., Kamal, A., Weidel, E., Groh, M., Börger, C., et al. (2015). Exploring the chemical space of ureidothiophene-2-carboxylic acids as inhibitors of the quorum sensing enzyme PqsD from Pseudomonas aeruginosa. Eur. J. Med. Chem. 96, 14–21. doi: 10.1016/j.ejmech.2015.04.007
Sakuragi, Y., and Kolter, R. (2007). Quorum-sensing regulation of the biofilm matrix genes(pel) of Pseudomonas aeruginosa. J. Bacteriol. 189, 5383–5386. doi: 10.1128/JB.00137-07
Saroj, S. D., and Rather, P. N. (2013). Streptomycin Inhibits Quorum Sensing in Acinetobacter baumannii. Antimicrob. Agents Chemother. 57, 1926–1929. doi: 10.1128/AAC.02161-12
Savka, M. A., Le, P. T., and Burr, T. J. (2011). LasR receptor for detection of long-chain quorum-sensing signals: identification of N-acyl-homoserine lactones encoded by the avsI locus of Agrobacterium vitis. Curr. Microbiol. 62, 101–110. doi: 10.1007/s00284-010-9679-1
Schaefer, A. L., Greenberg, E. P., Oliver, C. M., Oda, Y., Huang, J. J., Bittan-Banin, G., et al. (2008). A new class of homoserine lactone quorum-sensing signals. Nature 454, 595–599. doi: 10.1038/nature07088
Schaefer, A. L., Lappala, C. R., Morlen, R. P., Pelletier, D. A., Lu, T. Y., Lankford, P. K., et al. (2013). LuxR-and luxI-type quorum-sensing circuits are prevalent in members of the Populus deltoides microbiome. Appl. Environ. Microbiol. 79, 5745–5752. doi: 10.1128/AEM.01417-13
Shepherd, R. W., and Lindow, S. E. (2009). Two dissimilar N-acyl-homoserine lactone acylases of Pseudomonas syringae influence colony and biofilm morphology. Appl. Environ. Microbiol. 75, 45–53. doi: 10.1128/AEM.01723-08
Shiner, E. K., Rumbaugh, K. P., and Williams, S. C. (2005). Inter-kingdom signaling: deciphering the language of acyl homoserine lactones. FEMS Microbiol. Rev. 29, 935–947. doi: 10.1016/j.femsre.2005.03.001
Shrestha, P. M., Rotaru, A. E., Summers, Z. M., Shrestha, M., Liu, F. H., and Lovley, D. R. (2013). Transcriptomic and genetic analysis of direct interspecies electron transfer. Appl. Environ. Microbiol. 79, 2397–2404. doi: 10.1128/AEM.03837-12
Siddiqui, M. F., Rzechowicz, M., Winters, H., Zularisam, A. W., and Fane, A. G. (2015). Quorum sensing based membrane biofouling control for watertreatment: a review. J. Water Process Eng. 7, 112–122. doi: 10.1016/j.jwpe.2015.06.003
Singh, R. P. (2015). Attenuation of quorum sensing-mediated virulence in Gram-negative pathogenic bacteria: implications for the post-antibiotic era. Med. Chem. Commun. 6, 259–272. doi: 10.1039/C4MD00363B
Singh, R., Paul, D., and Jain, R. K. (2006). Biofilms: implications in bioremediation. Trends Microbiol. 14, 389–397. doi: 10.1016/j.tim.2006.07.001
Singh, B. R., Singh, B. N., Singh, A., Khan, W., Naqvi, A. H., and Singh, H. B. (2015). Mycofabricated biosilver nanoparticles interrupt Pseudomonas aeruginosa quorum sensing systems. Sci. Rep. 5:13719. doi: 10.1038/srep13719
Sio, C. F., Otten, L. G., Cool, R. H., Diggle, S. P., Braun, P. G., Bos, R., et al. (2006). Quorum quenching by an N-acyl-homoserine lactone acylase from Pseudomonas aeruginosa PAO1. Infect. Immun. 74, 1673–1682. doi: 10.1128/IAI.74.3.1673-1682.2006
Skindersoe, M. E., Alhede, M., Phipps, R., Yang, L., Jensen, P. O., Rasmussen, T. B., et al. (2008). Effects of antibiotics on quorum sensing in Pseudomonas aeruginosa. Antimicrob. Agents Chemother. 52, 3648–3663. doi: 10.1128/AAC.01230-07
Smith, M. G., Gianoulis, T. A., Pukatzki, S., Mekalanos, J. J., Ornston, L. N., Gerstein, M., et al. (2007). New insights into Acinetobacter baumannii pathogenesis revealed by high-density pyrosequencing and transposon mutagenesis. Genes Dev. 21, 601–614. doi: 10.1101/gad.1510307
Song, X. N., Cheng, Y. Y., Li, W. W., Li, B. B., Sheng, G. P., Fang, C. Y., et al. (2014). Quorum quenching is responsible for the underestimated quorum sensing effects in biological wastewater treatment reactors. Bioresour. Technol. 171, 472–476. doi: 10.1016/j.biortech.2014.08.027
Sperandio, V., Torres, A. G., Jarvis, B., Nataro, J. P., and Kaper, J. B. (2003). Bacteria–host communication: the language of hormones. Proc. Natl. Acad. Sci. U.S.A. 100, 8951–8956. doi: 10.1073/pnas.1537100100
Steenackers, H. P., Levin, J., Janssens, J. C., De Weerdt, A., Balzarini, J., Vanderleyden, J., et al. (2010). Structure-activity relationship of brominated 3-alkyl-5-methylene-2(5H)-furanones and alkylmaleic anhydrides as inhibitors of Salmonella biofilm formation and quorum sensing regulated bioluminescence in Vibrio harveyi. Bioorg. Med. Chem. 18, 5224–5233. doi: 10.1016/j.bmc.2010.05.055
Struss, A. K., Pasini, P., Flomenhoft, D., Shashidhar, H., and Daunert, S. (2012). Investigating the effect of antibiotics on quorum sensing with whole-cell biosensing systems. Anal. Bioanal. Chem. 402, 3227–3236. doi: 10.1007/s00216-012-5710-7
Sturme, M. H., Kleerebezem, M., Nakayama, J., Akkermans, A. D., Vaugha, E. E., and de Vos, W. M. (2002). Cell to cell communication by autoinducing peptides in gram-positive bacteria. Antonie Van Leeuwenhoek 81, 233–243. doi: 10.1023/A:1020522919555
Sunder, A. V., Kumar, A., Naik, N., and Pundle, A. V. (2012). Characterization of a new Bacillus cereus ATUAVP1846 strain producing penicillin V acylase, and optimization of fermentation parameters. Ann. Microbiol. 62, 1287–1293. doi: 10.1007/s13213-011-0374-7
Taga, M. E., Miller, S. T., and Bassler, B. L. (2003). Lsr-mediated transport and processing of AI-2 in Salmonella typhimurium. Mol. Microbiol. 50, 1411–1427. doi: 10.1046/j.1365-2958.2003.03781.x
Tan, C. H., Koh, K. S., Xie, C., Tay, M., Zhou, Y., Williams, R., et al. (2014). The role of quorum sensing signalling in EPS production and the assembly of a sludge community into aerobic granules. ISME J. 8, 1186–1197. doi: 10.1038/ismej.2013.240
Tan, C. H., Koh, K. S., Xie, C., Zhang, J., Tan, X. H., Lee, G. P., et al. (2015). Community quorum sensing signalling and quenching: microbial granular biofilm assembly. npj Biofilms Microbiomes 1:15006. doi: 10.1038/npjbiofilms.2015.6
Tanaka, R., Kunisada, T., Kushida, N., Yamada, K., Ikeda, S., Noike, M., et al. (2011). Branched fatty acids inhibit the biosynthesis of menaquinone in Helicobacter pylori. J. Antibiot. 64, 151–153. doi: 10.1038/ja.2010.133
Telford, G., Wheeler, D., Williams, P., Tomkins, P. T., Appleby, P., Sewell, H., et al. (1998). The Pseudomonas aeruginosa quorum-sensing signal molecule N-(3-oxododecanoyl)-L-homoserine lactone has immunomodulatory activity. Infect. Immun. 66, 36–42.
Thiel, V., Kunze, B., Verma, P., Wagner-Döbler, I., and Schulz, S. (2009). New structural variants of homoserine lactones in bacteria. Chem. Biochem. 10, 1861–1868. doi: 10.1002/cbic.200900126
Thoendel, M., Kavanaugh, J. S., Flack, C. E., and Horswill, A. R. (2011). Peptide signaling in the staphylococci. Chem. Rev. 111, 117–151. doi: 10.1021/cr100370n
Toyofuku, M., Nomura, N., Fujii, T., Takaya, N., Maseda, H., Sawada, I., et al. (2007). Quorum sensing regulates denitrification in Pseudomonas aeruginosa PAO1. J. Bacteriol. 189, 4969–4972. doi: 10.1128/JB.00289-07
Toyofuku, M., Nomura, N., Kuno, E., Tashiro, Y., Nakajima, T., and Uchiyama, I. (2008). Influence of the Pseudomonas quinolone signal on denitrification in Pseudomonas aeruginosa. J. Bacteriol. 190, 7947–7956. doi: 10.1128/JB.00968-08
Ueda, S., Sakamoto, K., Oohata, N., Tsuboi, M., Yamashita, M., Hino, M., et al. (2010). Screening and characterization of microorganisms with FR901379 acylase activity. J. Antibiot. 63, 65–70. doi: 10.1038/ja.2009.119
Uroz, S., Chhabra, S. R., Camara, M., Williams, P., Oger, P., and Dessaux, Y. (2005). N-Acylhomoserine lactone quorum-sensing molecules are modified and degraded by Rhodococcus erythropolis W2 by both amidolytic and novel oxido-reductase activities. Microbiology 151, 3313–3322. doi: 10.1099/mic.0.27961-0
Uroz, S., Oger, P. M., Chapelle, E., Adeline, M. T., Faure, D., and Dessaux, Y. (2008). A Rhodococcus qsdA-encoded enzyme defines a novel class of large-spectrum quorum-quenching lactonases. Appl. Environ. Microbiol. 74, 1357–1366. doi: 10.1128/AEM.02014-07
Uroz, S., Oger, P., Chhabra, S. R., Cámara, M., Williams, P., and Dessaux, Y. (2007). N-acyl homoserine lactones are degraded via an amidolytic activity in Comamonas sp. strain D1. Arch. Microbiol. 187, 249–256. doi: 10.1007/s00203-006-0186-5
Valle, A., Bailey, M. J., Whiteley, A. S., and Manefield, M. (2004). N-Acyl-L-homoserine lactones (AHLs) affect microbial community composition and function in activated sludge. Environ. Microbiol. 6, 424–433. doi: 10.1111/j.1462-2920.2004.00581.x
Vega, L. M., Mathieu, J., Yang, Y., Pyle, B. H., McLean, R. J. C., and Alvarez, P. J. J. (2014). Nickel and cadmium ions inhibit quorum sensing and biofilm formation without affecting viability in Burkholderia multivorans. Int. Biodeter. Biodegr. 91, 82–87. doi: 10.1016/j.ibiod.2014.03.013
Wagh Nee Jagtap, M. S., Patil, R. H., Thombre, D. K., Kulkarni, M. V., Gade, W. N., and Kale, B. B. (2013). Evaluation of anti-quorum sensing activity of silver nanowires. Appl. Microbiol. Biotechnol. 97, 3593–3601. doi: 10.1007/s00253-012-4603-1
Wagner, J., Guimarães, L. B., Akaboci, T. R., and Costa, R. H. (2015). Aerobic granular sludge technology and nitrogen removal for domestic wastewater treatment. Water Sci. Technol. 71, 1040–1046. doi: 10.2166/wst.2015.064
Walters, M., Sircili, M. P., and Sperandio, V. (2006). AI-3 synthesis is not dependent on luxS in Escherichia coli. J. Bacteriol. 188, 5668–5681. doi: 10.1128/JB.00648-06
Wang, J. H., He, H. Z., Wang, M. Z., Wang, S., Zhang, J., Wei, W., et al. (2013a). Bioaugmentation of activated sludge with Acinetobacter sp. TW enhances nicotine degradation in a synthetic tobacco wastewater treatment system. Bioresour. Technol. 142, 445–453. doi: 10.1016/j.biortech.2013.05.067
Wang, M. Z., He, H. Z., Zheng, X., Feng, H. J., Lv, Z. M., and Shen, D. S. (2014). Effect of Pseudomonas sp. HF-1 inoculum on construction of a bioaugmented system for tobacco wastewater treatment: analysis from quorum sensing. Environ. Sci. Pollut. Res. 21, 7945–7955. doi: 10.1007/s11356-014-2760-8
Wang, W. Z., Morohoshi, T., Someya, N., and Ikeda, T. (2012). AidC, a novel Nacylhomoserine lactonase from the potato root-associated Cytophaga–Flavobacteria–Bacteroides (CFB) group bacterium Chryseobacterium sp. strain StRB126. Appl. Environ. Microbiol. 78, 7985–7992. doi: 10.1128/AEM.02188-12
Wang, M., Xu, J., Wang, J., Wang, S., Feng, H., Shentu, J., et al. (2013b). Differences between 4-fluoroaniline degradation and antoinducer release by Acinetobacter sp. TW: implications for operating conditions in bacterial bioaugmentation. Environ. Sci. Pollut. Res. Int. 20, 6201–6209. doi: 10.1007/s11356-013-1660-7
Xavier, K. B., and Bassler, B. L. (2005a). Interference with AI-2-mediated bacterial cell-cell communication. Nature 437, 750–753. doi: 10.1038/nature03960
Xavier, K. B., and Bassler, B. L. (2005b). Regulation of uptake and processing of the quorum sensing autoinducer AI-2 in Escherichia coli. J. Bacteriol. 187, 238–248. doi: 10.1128/JB.187.1.238-248.2005
Xia, S., Zhou, L., Zhang, Z., and Li, J. (2012). Influence and mechanism of N-(3-oxooxtanoyl)-L-homoserine lactone (C8-oxo-HSL) on biofilm behaviors at the early stage. J. Environ. Sci. 24, 2035–2040. doi: 10.1016/S1001-0742(11)61060-7
Xiong, Y. H., and Liu, Y. (2010). Involvement of ATP andAutoinducer-2 in aerobic granulation. Biotechnol. Bioeng. 105, 51–58. doi: 10.1002/bit.22526
Xu, H. J., and Liu, Y. (2010). Control of microbial attachment by inhibition of ATP and ATP-mediated autoinducer-2. Biotechnol. Bioeng. 107, 31–36. doi: 10.1002/bit.22796
Xu, H., and Liu, Y. (2011). Reduced microbial attachment by D-amino acid-inhibited AI-2 and EPS production. Water. Res. 45, 5796–5804. doi: 10.1016/j.watres.2011.08.061
Yang, S. F., Liu, Q. S., Tay, J. H., and Liu, Y. (2004). Growth kinetics of aerobic granules developed in sequencing batch reactors. Lett. Appl. Microbiol. 38, 106–112. doi: 10.1111/j.1472-765X.2003.01452.x
Yao, Y., Martinez-Yamout, M. A., Dickerson, T. J., Brogan, A. P., Wright, P. E., and Dyson, H. J. (2006). Structure of the Escherichia coli quorum sensing protein SdiA: activation of the folding switch by acyl homoserine lactones. J. Mol. Biol. 355, 262–273. doi: 10.1016/j.jmb.2005.10.041
Yeon, K. M., Cheong, W. S., Oh, H. S., Lee, W. N., Hwang, B. K., Lee, C. H., et al. (2009a). Quorum sensing: a new biofouling control paradigm in a membrane bioreactor for advanced wastewater treatment. Environ. Sci. Technol. 43, 380–385. doi: 10.1021/es8019275
Yeon, K. M., Lee, C. H., and Kim, J. (2009b). Magnetic enzyme carrier for effective biofouling control in the membrane bioreactor based on enzymatic quorum quenching. Environ. Sci. Technol. 43, 7403–7409. doi: 10.1021/es901323k
Yong, Y. C., Wu, X. Y., Sun, J. Z., Cao, Y. X., and Song, H. (2015). Engineering quorum sensing signaling of Pseudomonas for enhanced wastewater treatment and electricity harvest: a review. Chemosphere 140, 18–25. doi: 10.1016/j.chemosphere.2014.10.020
Yong, Y. C., and Zhong, J. J. (2010). N-acylated homoserine lactone production and involvement in the biodegradation of aromatics by an environmental isolate of Pseudomonas aeruginosa. Process Biochem. 45, 1944–1948. doi: 10.1016/j.procbio.2010.05.006
Yong, Y., and Zhong, J. (2013a). Impacts of quorum sensing on microbial metabolism and human health. Adv. Biochem. Eng. Biotechnol. 131, 25–61. doi: 10.1007/10_2012_138
Yong, Y. C., and Zhong, J. J. (2013b). Regulation of aromatics biodegradation by rhl quorum sensing system through induction of catechol meta-cleavage pathway. Bioresour. Technol. 136, 761–765. doi: 10.1016/j.biortech.2013.03.134
Zang, T., Lee, B. W., Cannon, L. M., Ritter, K. A., Dai, S., Ren, D., et al. (2009). A naturally occurring brominated furanone covalently modifies and inactivates LuxS. Bioorg. Med. Chem. Lett. 19, 6200–6204. doi: 10.1016/j.bmcl.2009.08.095
Zhang, S. H., Yu, X., Guo, F., and Wu, Z. Y. (2011). Effect of interspecies quorum sensing on the formation of aerobic granular sludge. Water Sci. Technol. 64, 1284–1290. doi: 10.2166/wst.2011.723
Zhang, K., Zheng, X., Shen, D. S., Wang, M. Z., Feng, H. J., He, H. Z., et al. (2015). Evidence for existence of quorum sensing in a bioaugmented system by acylated homoserine lactone-dependent quorum quenching. Environ. Sci. Pollut. Res. Int. 22, 6050–6056. doi: 10.1007/s11356-014-3795-6
Zhao, Z. G., Yu, Y. M., Xu, B. Y., Yan, S. S., Xu, J. F., Liu, F., et al. (2013). Screening and anti-virulent study of N-acyl homoserine lactones DNA aptamers against Pseudomonas aeruginosa quorum sensing. Biotechnol. Bioproc. Eng. 18, 406–412. doi: 10.1007/s12257-012-0556-6
Zhu, P., Peng, H., Ni, N., Wang, B., and Li, M. (2012). Novel AI-2 quorum sensing inhibitors in Vibrio harveyi identified through structure-based virtual screening. Bioorg. Med. Chem. Lett. 22, 6413–6417. doi: 10.1016/j.bmcl.2012.08.062
Keywords: quorum sensing, autoinducer, QS regulation, wastewater treatment system, aquaculture
Citation: Zhang W and Li C (2016) Exploiting Quorum Sensing Interfering Strategies in Gram-Negative Bacteria for the Enhancement of Environmental Applications. Front. Microbiol. 6:1535. doi: 10.3389/fmicb.2015.01535
Received: 19 June 2015; Accepted: 21 December 2015;
Published: 08 January 2016.
Edited by:
Varenyam Achal, East China Normal University, ChinaReviewed by:
Steven Ripp, University of Tennessee, USAChristopher L. Hemme, University of Rhode Island, USA
Copyright © 2016 Zhang and Li. This is an open-access article distributed under the terms of the Creative Commons Attribution License (CC BY). The use, distribution or reproduction in other forums is permitted, provided the original author(s) or licensor are credited and that the original publication in this journal is cited, in accordance with accepted academic practice. No use, distribution or reproduction is permitted which does not comply with these terms.
*Correspondence: Chenghua Li, bGljaGVuZ2h1YUBuYnUuZWR1LmNu