- 1School of Materials Science and Engineering, Southeast University, Nanjing, China
- 2Research Institute of Green Construction Materials, Southeast University, Nanjing, China
This research investigated the self-healing potential of early age cracks in cement-based materials incorporating the bacteria which can produce carbonic anhydrase. Cement-based materials specimens were pre-cracked at the age of 7, 14, 28, 60 days to study the repair ability influenced by cracking time, the width of cracks were between 0.1 and 1.0 mm to study the healing rate influenced by width of cracks. The experimental results indicated that the bacteria showed excellent repairing ability to small cracks formed at early age of 7 days, cracks below 0.4 mm was almost completely closed. The repair effect reduced with the increasing of cracking age. Cracks width influenced self-healing effectiveness significantly. The transportation of CO2and Ca2+ controlled the self-healing process. The computer simulation analyses revealed the self-healing process and mechanism of microbiologically precipitation induced by bacteria and the depth of precipitated CaCO3 could be predicted base on valid Ca2+.
Introduction
With low tensile strength, concrete may take cracks easily during its service life (Bang et al., 2010). Cracking is one of the main factors causing the degradation of concrete durability. Repairing timely or self-healing for cracks may extend the service-life of concrete structures (Wang et al., 2014). Thus, inspection and maintenance techniques for concrete structures have therefore become the focus of interesting attention. However, the traditional repair methods such as cement grouting and maintenance are all difficult and expensive. Under such circumstances, the ability of self-healing cracks in concrete raised wide spread attention.
The phenomenon of self-healing in concrete has been known for many years (Jacobsen and Sellevold, 1996; Edvardsen, 1999; Huang et al., 2013). Precipitation of calcium carbonate has been reported to be the most significant factor influencing the self-healing of concrete (Edvardsen, 1999). Many researchers (Gollapudi et al., 1995; Hill and Sleep, 2002; Rodriguez-Navarro et al., 2003) found that some bacteria could induce or improve the precipitation of CaCO3. This phenomenon which was called bio-mineralization existed widespread in nature. Since last decade, Ramakrishnan et al. (1998) published pioneer papers in 1998, bio-mineralization has been developed for the treatment of concrete cracks.
Two main cracks repair mechanisms were proposed in the recent studies. One was proposed by Bang who found one type of bacteria could decompose urea to CO which can react with Ca2+ to form CaCO3 in alkaline environment (Stocks-Fisher et al., 1999; Bang et al., 2001; Dhami et al., 2013). This mechanism was applied mainly for passive repair. However, it is very hard to handle the ammonia decomposed from the urea. The other mechanism mainly applying for self-healing was proposed by Jonkers (Jonkers, 2007; Jonkers and Schlangen, 2008; Jonkers and Thijssen, 2010; Jonkers et al., 2010). One type of bacteria which could decompose specific substrate to CaCO3 was used in his studies. CO2 released in the reaction process which could also trigger CaCO3 precipitated in cracks. But the speed of substrate decomposed is slow and the quantity of CO2 from substrate is limited.
As some microbes in the nature can conduct the inter-conversion between CO2 which in atmosphere and CaCO3, Dreybrodt et al. (1998) thought in the H2O–CO2–CaCO3 system, the slow reaction was considered as one of the rate-limiting steps for the precipitation rate of calcite from supersaturated solutions. Carbonic anhydrase (CA) can catalyze the inter-conversion of CO2 and HCO to improve the absorption of CO2(Heck et al., 1994; Mirjafari et al., 2007). Therefore, CA can aid in the capture of CO2 and the precipitation of CaCO3, following the equations by the research of Li et al. (2011):
In this study, one type of bacteria which can produce carbonic anhydrase was incorporated in cement-based materials to repair cracks. Compared to other self-healing in concrete with bacteria (Bang and Ramakrishnan, 2001; Jonkers, 2007; Wang et al., 2012), the new bacteria can promote the inter-conversion between CO2 which from atmosphere and CaCO3. That means CO2 can be transferred to minerals precipitated in cracks when reacting with soluble Ca2+ rapidly. The bacteria showed excellent repairing ability to small cracks. The depth of CaCO3 precipitated layer was also predicted base on valid Ca2+ and practical experiment verified the accuracy of predicted results.
Materials and Methods
Bacterial Strain
Bacillus mucilaginous L3 (China Center of Industrial Culture Collection, CICC) was used in this study. The growth characteristics of the microorganism is shown in Figure 1.
The results indicated that the best incubation time was about 24 h, and the OD400 was 0.8. After incubated for 24 h in specific liquid medium, the fresh bacterial strain were harvested by high-speed (8000 rpm) centrifugation at 4°C for 10 min. Then the bacterial strains were suspended in sterile deionized water. The final concentration of bacterial strain was about 109 cells/mL.
Preparation of the Specimens
Cement paste specimens were used for all analyses in this study. All mixtures were designed with a water to cement ratio (w/c) of 0.40 by using ordinary Portland cement II 42.5. Four series of specimens were made and the composition of each series is shown in Table 1.
Group G1 are the specimens without any additions. Group G2 are the specimens with nutrient needed for bio-deposition. Group G3 are the specimens only with bacteria. Group G4 are the specimens with nutrient and bacteria at the same times.
Two types of cement specimens, cylinders (φ, 110 mm, H, 45 mm) for testing water permeability and prisms (40 × 40 × 160 mm) for investigating the healing area of cracks, were made. After casting, all molds were put in curing room with a temperature of 20°C and relative humidity of more than 90% for 24 h. The specimens were then de-molded and placed under the standard curing room with a temperature of 20 ± 2°C and relative humidity of 95% until the time of testing.
Cracks Formation and Self-healing Incubation
The specimens from Group G1 to Group G4 were taken out from curing room and early age cracks were created after 7 days. The prisms specimens were wrapped with adhesive tape before bending test. The initial cracks were made by a bending load which was loaded to the prisms specimens until the crack break through the cross section. Then nails with different width were embedded into the initial cracks to create the surface cracks with different width. The cracks formation on prism specimens by embedded method was shown in Figure 2. For prism specimens, the compression test (loading speed 0.5–0.8 Mpa/s) was used to make cracks, whose crack width was below 0.3 mm. For crack-healing quantification under different crack width, the crack width was 0.3, 0.4, 0.5, 0.6, 0.7, 0.8, 0.9, and 1.0 mm, respectively. The crack width measured by crack width measuring instrument (Beijing Koncrete Engineering Testing Technology Co., Ltd) which degree of accuracy is 0.01 mm. For studying the self-healing ability influenced by cracking age, the crack width was controlled in the range of 0.4~0.6 mm. To study the repair ability influenced by cracking time, the cracks were created, respectively, after 7, 14, 28, and 60 days.
The treated cracked specimens were immerged in tap water which was exposed to the atmosphere during the whole repair period. Air was pumped into water to keep the adequate supply of CO2.
Characterization Methods
Three methods were used to characterize the cracks healing efficiency. The crack healing efficiency of specimens was evaluated by water permeation coefficient according to the method reported with a modification (Wang et al., 2012). The cylinder specimen was casted into PVC mold when molding. PVC mold could be connected to the PVC pipe before test and the joint was sealed tightly to avoid leakage. The water in PVC pipe was kept a fixed height to maintain a constant pressure on the surface of cylinder specimen. The volume of passed water could be measured easily during one period of time. The schematic diagram of water permeability test was show in Figure 3. Permeability coefficient of cylinder specimen before and after healing could be calculated according to Darcy's Law shown in Equation (1). Where k (m/s) is permeability coefficient, Q (m3/s) is the amount of water flow, L (m) is the height of specimens, A (m2) is the area of section and Δh (m) is head difference.
Another method to evaluate self-healing was put forward based on image processing. Images for cracks were taken by crack width measuring instrument at the same light source (Figure 4). Image-J was used to achieve the binary photograph and analyze the gray value. Threshold cutting could be achieved easily by setting the gray level threshold of crack at 115 by Image-J. When the image processing of specimen surface cracks at different healing time was carried out, crack areas (pixels) in initial and in different healing time could be counted and the healing efficiency was described by Equation (2). Where α is the area repair rate, A0 is the initial crack area, At is the crack area at healing time. The area repair rate is:
The flexural strength after repaired with different self-healing agent was tested according to the method reported by Li et al. (2013). The three-point flexural loading setup was from MTS Industrial Systems (China) Co., Ltd. The specimens were firstly loaded under three-point flexural configuration and then assembled a whole with tape. Nails were inserted the cracks to control cracks width. After curing certain days in water, the specimens were reloaded as the same testing.
CO2 Diffusion in Cracked Concrete
It was hypothesized that the diffusion of CO2 conformed to Fick's law in this study (Equation 3). Where c (kg/m3) is the concentration of CO2 in concrete, D (m2/s) is the diffusion coefficient, t (s) is time.
The CO2 diffusion in cracked concrete can be derived by the CO2 diffusion in sound concrete. According to the research of Saetta et al. (1995), the diffusion coefficient (Dc) in sound concrete can be simplified as Equation (4). Where Dc, 0 is CO2 diffusion coefficient in sound concrete curing at 28 days when temperature is 20°C and relative humidity is 65%. Dc, 0(m2/s) can be described as Equation (5) according to 50 dates in papers (Chen, 2007) which were the relationship between Dc, 0 and compressive strength of concrete. Where fcuk (Mpa) is compressive strength of concrete. F3(T) is the temperature factor in Equation (6) and F4(H) is the humidity factor in Equation (7). Where K is absolute temperature, RH is relative humidity, RH0 = 65%.
The diffusion coefficient of CO2 in crack relates to crack width according to the study of Song et al. (2006). In this study, cracking specimens were cured in water. When the crack was filled in water, according to the research of Versteeg and van Swaaij (1988). The concentration of CO2 on boundary condition is 6.4216 × 10−4 kg/m3 according to Henry' Law. The model of concrete (16 × 8 cm) is shown in Figure 5. In this model, the size of each mesh is 0.1 × 0.1 mm, the depth of crack is 6 cm and the width of crack is 0.4 mm. The calculation parameters in this model were shown in Table 2, Where Dc is the diffusion coefficient of CO2 in crack, D0 is the diffusion coefficient of CO2 in sound concrete and C0 is the concentration of CO2 on boundary condition.
Ca2+ Dissolving in Cracked Specimens
Group G2 and Group G3 in Section Preparation of the Specimens were used to study the dissolution of Ca2+ in cracked specimens. The prisms specimens were took out after 28 days curing and different crack width (0.2, 0.4, 0.6, 0.8, and 1.0 mm) were created. All surfaces of specimens were sealed with paraffin wax, only cracks were exposed to outside. Then the specimens were immerged in deionized water, the concentration of Ca2+ in water was measured by EDTA titration in 3, 7, and 14 days. The specimen (G2) only with nutrient [Ca(NO3)2] was as a control test.
Results and Discussion
Crack-healing Efficiency of Self-healing Agent
Figure 6 showed the images of the early age cracks during the process of self-healing. Where (Figure 6A) was specimen in Group G1 and (Figure 6B) was specimen in Group G4. It can be seen that crack was almost filled completely after 5 days in Group 4 which cooperated with self-healing agent. But the specimen in Group G1 with no self-healing agent couldn't be healed even curing 20 days.
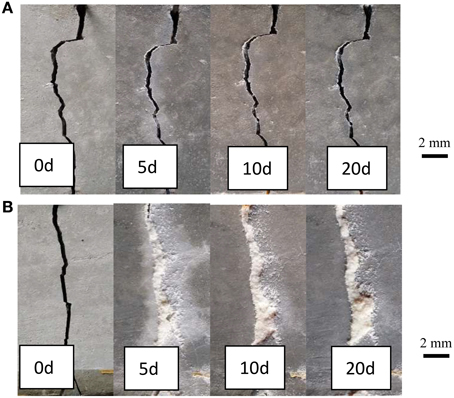
Figure 6. Surface images of specimens after different healing time. (A) G1, Specimens without self-healing agent; (B) G4, specimens with self-healing agent.
Figure 7 showed the permeability coefficient changed over healing time in each group. The initial permeability coefficient of all specimens was about 2.0 × 10−5 m/s. The water permeability of specimens in each group decreased after 30 days of immersion in water. The permeability coefficient of specimen in Group G4 declined to 1.28 × 10−8 m/s after 30 days, and the phenomenon of water permeability had disappeared. The permeability coefficient of specimen in Group G1 only declined to 2.53 × 10−7 m/s after 30 days and the permeability-resistant ability couldn't be improved efficiently during the healing process. The permeability coefficient of specimen in Group G3 only adding bacteria was lower than Group G2 only adding nutrient. It can be concluded that the bacteria could improve CaCO3 precipitate to heal the cracks.
Moreover, the flexural strength of specimens with different self-healing agent after repaired was tested. The results of the flexural strength for the repaired specimens were shown in Figure 8. Compared to the normalized strength of specimens G1, G2, and G3, the normalized strength of specimens G4 with nutrient and bacteria at the same times reached to 2.1. The results indicated that the flexural strength of specimens repaired with nutrient and bacteria could be increased 40, 90.9, and 110% than other self-healing agent (G3, G2, and G1), respectively.
Cracking-healing Quantification under Different Crack Width
Figure 9 showed the binary images of different width crack which processed by Image-J after different healing stages. The area repair rate of different width cracks was different. The crack with a width of 0.3 mm was nearly closed after 5 days of immersion in tap water. While the crack with a width of 0.5 mm could be healed fully after 10 days. When crack width increased to 1.0 mm, it couldn't be healed well even after 20 days.
The area repair rate of specimens with different crack width after different healing time was calculated based on Equation (2) as shown in Figure 10. It can be seen that the crack was more difficult to repair with the increase of average crack width and the self-healing efficiency of microbial agent declined. Excellent healing effect could be got for the cracks whose average width was below 0.4 mm, the average area repair rate reached over 90% after 30 days. For the cracks between 0.5 and 0.8 mm, the average area repair rate ranged from 60 to 80%, and the larger standard deviation showed obvious difference in self-healing efficiency for different cracks. However, the repair ability of microbial self-healing agent was limited for crack width up to 0.9 mm, and the corresponding average area repair rate was lower than 30% after curing 30 days.
When crack occurred in cement paste specimen, CO2 in atmosphere went into crack from crack surface and Ca2+ immigrated from cement to crack. CO2 would be transferred to HCO induced by microbes in crack and finally precipitated CaCO3 when combined with Ca2+ in the alkaline environment.
It can be explained as follows: Firstly, The CaCO3 precipitation appeared in crack surface, then increased gradually, and fully healed the crack below 0.3 mm. More mineral are needed for larger width cracks for completely healed. Secondly, Ca2+ provided by self-healing agent escaped outside to water more easily, resulting in the loss of self-healing agent.
Cracking-healing Efficiency under Different Cracking Age
Concrete cracking may occur in any time of service life. As shown in Figure 11, the self-healing effect was excellent for cracking at early age of 7 days. The self-healing efficiency of cracks dropped when cracking age increasing. The area repair rate declined significantly when cracking after 28 days. When the cracking age was more than 60 days, self-healing agent had lost the repair effect. The survival numbers of bacteria in matrix reduced with the increasing of cracking age because of the high alkaline environment in cement-based materials. When the amount of active bacteria reduced to quite small, CaCO3 precipitation couldn't be induced. Jonkers et al. (2010) studied the hydration of cement in 28 days would decrease the pores which ranged from 2 to 10 μm greatly in matrix. The living space for bacteria decreased, resulting in death of bacteria. On the other hand, the decrease of pores also made transportation of Ca2+ more difficult.
Numerical Simulation of CO2 in Crack
Figure 12 showed the concentration of CO2 in concrete when immerging in water after 5 days. It can be seen that the concentration of CO2 decreased in depth direction. When the depth was 10 mm, the concentration of CO2 declined about 50% compared to crack surface. The concentration on crack surface was highest, leaded to CaCO3 precipitated quickly around the crack surface and finally closed the crack. And in depth of crack, the concentration of CO2 inside was lower and CO2 was insufficient to precipitate CaCO3. In other hand, the close of crack surface obstructed the CO2 diffusing into crack, further hindered the CaCO3 precipitating in the depth direction of crack.
Predicting CaCO3 Precipitated Depth Base on Valid Ca2+
Table 3 showed the dissolution of Ca2+ in Group G2 and Group G4. The amount of dissolute Ca2+ in G2 was higher than G4. Ca2+in paste immigrated from crack to outside because of the concentration gradient. The bacteria in G4 may impact the amount of dissolute Ca2+. Ca2+ will cling to bacteria because of its negative cells and the bacteria induce CO2 transferring to HCO quickly on crack surface, further combining with Ca2+. The difference of the amount of dissolute Ca2+ between G2 and G4 was called valid Ca2+ which reflected the effect of CaCO3 precipitated induced by bacteria. The valid Ca2+ meant the part of Ca2+ which reacted with HCO then transferred to CaCO3.
The valid Ca2+ in different crack width was shown in Table 4. It can be concluded that larger crack was benefit to the dissolution of Ca2+, but the amount of valid Ca2+ was lower in larger crack. Ca2+ escaped outside more easily in large crack, but the capture effect of Ca2+ by bacteria was limited. The loss of Ca2+ which escaped outside to water decreased the amount of valid Ca2+. It also can be seen that the amount of valid Ca2+ dissolute in 3 days contributed more than 70% to total valid Ca2+ dissolute in 14 days except crack width was 0.2 mm. The results indicated that the crack healing occurred mainly at early age. At this period of time, bacteria had high activity to transfer CO2 and much HCO could participate in reaction. Crack surface became narrow gradually and finally closed with the increase of healing time. At the late age, the closing of crack surface made the dissolution of Ca2+ inside more difficult.
The depth of precipitated CaCO3 could be predicted base on valid Ca2+ shown in Figure 13. When crack width was 0.2 mm, the healing depth in crack was, respectively, 0.5, 0.64, and 1.09 mm after 3, 7, and 14 days. The depth of precipitated CaCO3 decreased with the increasing of crack width. When crack width was 1.0 mm, the maximum depth of precipitated CaCO3 was only 0.10 mm after 14 days.
Verification of the Depth of Precipitated CaCO3
In practical experiment, prisms specimens with self-healing agent were used. The crack (0.2, 0.4, 0.6, 0.8, and 1.0 mm) was made after 7 days curing. Then specimens with crack were immerged into tape water which exposed to atmosphere to 14 days. Figure 14 is the illustration image of cracking specimen.
Images of fracture section could be got by digital camera shown in Figure 15. It can be seen that a layer of white sediments precipitated on fracture section. The depth of precipitated CaCO3 could be measured by ruler tool. White lines were the measurement position of precipitated depth. Finally the average precipitated depth was calculated to represent the CaCO3 precipitated depth.
Figure 16 showed the CaCO3 precipitated in depth direction of fracture section under different crack width. The amount of precipitated CaCO3 decreased with the increase of depth and the deposited layer had irregular boundary when crack width was 0.2 mm. The maximum depth of deposited layer was 1.11 mm. When crack width was 0.6 mm, CaCO3 mainly precipitated on crack surface. There were no obvious CaCO3 found when depth exceeded 0.3 mm. When crack width was 1.0 mm, the depth of precipitated CaCO3 layer was only about 0.1 mm.
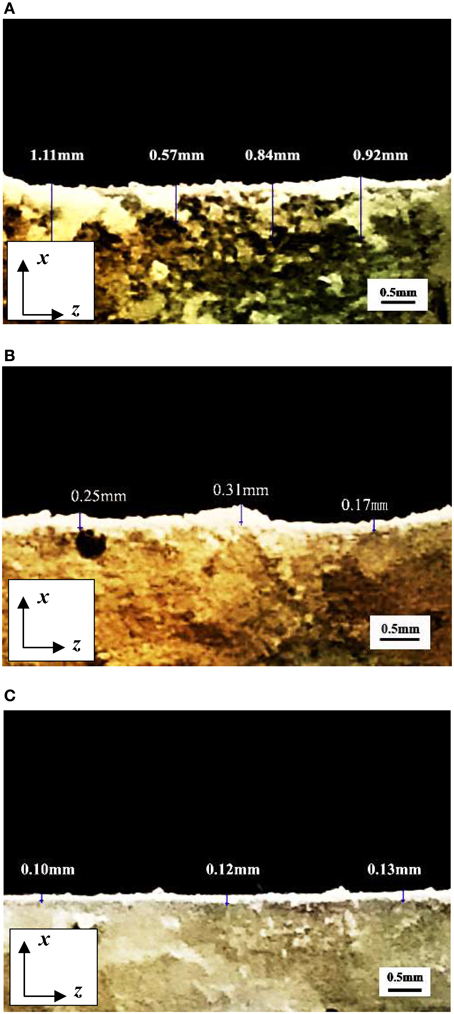
Figure 16. The CaCO3 precipitated in depth direction of fracture section under different crack width. (A) Crack width was 0.2 mm; (B) Crack width was 0.6 mm; (C) Crack width was 1.0 mm.
The comparison between predicted result and experimental result of CaCO3 precipitated depth was shown in Table 5. It can be seen that the predicted depth of precipitated CaCO3 fit well with the practical experimental results when crack width was larger than 0.2 mm. This result indicted that predicted depth of precipitated CaCO3 base on valid Ca2+ was accurate.
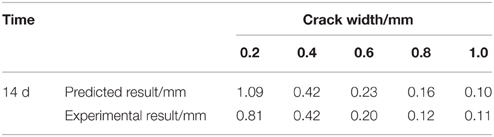
Table 5. The predicted and experimental result of CaCO3 precipitated depth under different crack width.
Conclusion
The results showed that the bacteria showed excellent repairing ability to small cracks and the self-healing agent can be applied for self-healing of early age cracks in cement-based material. However, the self-healing capacity depended on many factors. The cracks of early age with small width (below 0.3 mm) could be almost fully filled after cured in water.
The diffusion coefficient of CO2 in crack relates to crack width was also studied. CO2 only focused on the surface of crack whose depth was below 1.0 mm. The highest concentration of CO2 on surface leaded to CaCO3 precipitated quickly and finally closed the crack, further hindered CO2 diffused into crack. And in depth of crack, the concentration of CO2 inside was lower and CO2 was insufficient to precipitate CaCO3.
The depth of CaCO3 precipitated layer was predicted base on valid Ca2+ in this study and practical experiment verified the accuracy of predicted results. The depth of precipitated CaCO3 declined with the increasing of crack width, which coincided with the self-healing efficiency under different cracks width. A certain amount of CaCO3 precipitated on the surface of cracks, finally closed the crack surface, and obstructed the self-healing in depth of crack.
Conflict of Interest Statement
The authors declare that the research was conducted in the absence of any commercial or financial relationships that could be construed as a potential conflict of interest.
Acknowledgments
Authors are much appreciated the financial support from National Nature Science Foundation of China (Grant No. 51178104), Ph.D. Program's Foundation from Ministry of Education of China (Grant No. 20110092110033) and the National Program on Key Basic Research Project (973 Program, Grant No. 2015CB6551002).
References
Bang, S. S., Galinat, J. K., and Ramakrishnan, V. (2001). Calcite precipitation induced by polyurethane-immobilized Bacillus pasteurii. Enzyme Microb. Technol. 28, 404–409. doi: 10.1016/S0141-0229(00)00348-3
Bang, S. S., Lippert, J. J., Yerra, U., Mulukutla, S., and Ramakrishnan, V. (2010). Microbial calcite, a bio-based smart nanomaterial in concrete remediation. Int. J. Smart Nano Mater. 1, 28–39. doi: 10.1080/19475411003593451
Bang, S. S., and Ramakrishnan, V. (2001). “Microbiologically-enhanced crack remediation,” in Proceedings of the International Symposium on Industrial Application of Microbial Genomes (Daegu), 3–13.
Chen, L. T. (2007). Research on Concrete Carbonation Model and its Parameters. Xi'an: Xi'an University of Architecture and Technology.
Dhami, N. K., Reddy, M. S., and Mukherjee, A. (2013). Biomineralization of calcium carbonates and their engineered applications: a review. Front Microbiol. 4:314. doi: 10.3389/fmicb.2013.00314
Dreybrodt, W., Eisenlohr, L., Madry, B., and Ringer, S. (1998). Precipitation kinetics of calcite in the system CaCO3-H2O-CO2: the conversion to CO2 by the slow process H++HCO—CO2+H2O as a rate limiting step. Geochim. Cosmochim. Acta 61, 3897–3904. doi: 10.1016/S0016-7037(97)00201-9
Edvardsen, C. (1999). Water permeability and autogenous healing of cracks in concrete. ACI Mater. J. 96, 448–454.
Gollapudi, U. K., Knutson, C. L., Bang, S. S., and Islam, M. R. (1995). A new method for controlling leaching through permeable channels. Chemosphere 30, 695–705. doi: 10.1016/0045-6535(94)00435-W
Heck, R. W., Tanhauser, S. M., Manda, R., Tu, C., Laipis, P. J., and Slivermann, D. N. (1994). Catalytic properties of mouse carbonic anhydrase V. J. Biol. Chem. 269, 24742–24746.
Hill, D. D., and Sleep, B. E. (2002). Effects of biofilm growth on flow and transport through a glass parallel plate fracture. J. Contam. Hydro. 56, 227–246. doi: 10.1016/S0169-7722(01)00210-8
Huang, H. L., Ye, G., and Damidot, D. (2013). Characterization and quantification of self-healing behaviors of microcracks due to further hydration in cement paste. Cem. Concr. Res. 52, 71–81. doi: 10.1016/j.cemconres.2013.05.003
Jacobsen, S., and Sellevold, E. J. (1996). Self healing of high strength concrete after deterioration by freeze/thaw. Cem. Concr. Res. 26, 55–62. doi: 10.1016/0008-8846(95)00179-4
Jonkers, H. M. (2007). “Self healing concrete: a biological approach,” in Self Healing Materials: An alternative Approach to 20 Centuries of Materials Science, ed S. van der Zwaag (Delft: Springer), 195–204.
Jonkers, H. M., and Schlangen, E. (2008). Development of a bacteria-based self-healing concrete. Proc. Int. FIB. Symp. 1, 425–430. doi: 10.1201/9781439828410.ch72
Jonkers, H. M., and Thijssen, A. (2010). “Bacteria mediated remediation of concrete structures,” in Proceedings of the Second International Symposium on Service Life Design for Infrastructures, eds K. van Breugel, G. Ye, and Y. D. Yuan, 833–840. Available online at: http://hdl.handle.net/1854/LU-1265990
Jonkers, H. M., Thijssen, A., Muyzer, G., Copuroglu, O., and Schlangen, E. (2010). Application of bacteria as self-healing agent for the development of sustainable concrete. Ecol. Eng. 36, 230–235. doi: 10.1016/j.ecoleng.2008.12.036
Li, W., Liu, L. P., Zhou, P. P., Cao, L., Yu, L. J., and Jiang, S. Y. (2011). Calcite precipitation induced by bacteria and bacterially produced carbonic anhydrase. Curr. Sci. 100, 502–508. Available online at: http://www.currentscience.ac.in/Volumes/100/04/0502.pdf
Li, W., Jiang, Z., Yang, Z., Zhao, N., and Yuan, W. (2013). Self-healing efficiency of cementitious materials containing Microcapsules filled with healing adhesive: mechanical restoration and healing process monitored by water absorption. PLoS ONE 8:e81616. doi: 10.1371/journal.pone.0081616
Mirjafari, P., Asghari, K., and Mahinpey, N. (2007). Investigating the application of enzyme carbonic anhydrase for CO2 sequestration purposes. Ind. Eng. Chem. Res. 46, 921–926. doi: 10.1021/ie060287u
Ramakrishnan, V., Bang, S. S., and Deo, K. S. (1998). “A novel technique for repairing cracks in high performance concrete using bacteria,” in Proceeding of International Conference on High Performance High Strength Concrete (Perth, WA), 597–618.
Rodriguez-Navarro, C., Rodriguez-Gallego, M., Ben Chekroun, K., and Gonzalez-Muñoz, M. T. (2003). Conservation of ornamental stone by myxococcusxanthus-induced carbonate biomineralization. Appl. Environ. Microbiol. 69, 2182–2193. doi: 10.1128/AEM.69.4.2182-2193.2003
Saetta, A. V., Schrefler, B. A., and Vitaliani, R. V. (1995). 2-D Model for carbonation and moisture/heat flow in porous materials. Cem. Concr. Res. 25, 1703–1712. doi: 10.1016/0008-8846(95)00166-2
Song, H. W., Kwon, S. J., Byun, K. J., and Park, C. K. (2006). Predicting carbonation in early-aged cracked concrete. Cem. Concr. Res. 36, 979–989. doi: 10.1016/j.cemconres.2005.12.019
Stocks-Fisher, S., Galinat, J. K., and Bang, S. S. (1999). Microbiological precipitation of CaCO3. Soil Biol. Biochem. 31, 1563–1571. doi: 10.1016/S0038-0717(99)00082-6
Versteeg, G. F., and van Swaaij, W. P. M. (1988). Solubility and diffusivity of acid gases (CO2, N2O) in aqueous alkanolamine solutions. J. Chem. Eng. Data 33, 29–34. doi: 10.1021/je00051a011
Wang, J. Y., Sones, H., Verstraete, W., and De Belie, N. (2014). Self-healing concrete by use of microencapsulated bacterial spores. Cem. Concr. Res. 56, 139–152. doi: 10.1016/j.cemconres.2013.11.009
Keywords: cement-based materials, bacteria, early age, self-healing, mechanism
Citation: Qian C, Chen H, Ren L and Luo M (2015) Self-healing of early age cracks in cement-based materials by mineralization of carbonic anhydrase microorganism. Front. Microbiol. 6:1225. doi: 10.3389/fmicb.2015.01225
Received: 13 July 2015; Accepted: 19 October 2015;
Published: 04 November 2015.
Edited by:
Varenyam Achal, East China Normal University, ChinaReviewed by:
Sandra Simaria Lucas, University of Greenwich, UKJian-Guo Dai, The Hong Kong Polytechnic University, Hong Kong
Shaopeng Wu, Wuhan University of Technology, China
Copyright © 2015 Qian, Chen, Ren and Luo. This is an open-access article distributed under the terms of the Creative Commons Attribution License (CC BY). The use, distribution or reproduction in other forums is permitted, provided the original author(s) or licensor are credited and that the original publication in this journal is cited, in accordance with accepted academic practice. No use, distribution or reproduction is permitted which does not comply with these terms.
*Correspondence: Huaicheng Chen, Y2hlbmhjaDg2QDE2My5jb20=