- 1State Key Laboratory of Marine Geology, Tongji University, Shanghai, China
- 2Department of Producción Vegetaly Microbiología, Universidad Miguel Hernández, Alicante, Spain
Marine Group (MG) I (currently known as Thaumarchaeota) and MG II Archaea were first reported over two decades ago. While significant progress has been made on MG I microbiology and ecology, the progress on MG II has been noticeably slower. The common understanding is that while MG I mainly function as chemolithoautotrophs and occur predominantly in the deep ocean, MG II reside mostly in the photic zone and live heterotrophically. Studies to date have shown that MG II are abundant in the marine aquatic environment and display great seasonal and spatial variation and phylogenetic diversity. They also show unique patterns of organic carbon degradation and their energy requirements may be augmented by light in the photic zone. However, no pure culture of MG II has been obtained and thus their precise ecological role remains elusive.
Introduction
In 1992, DeLong and Fuhrman et al. reported the occurrence of archaea in the cold ocean. Two phylogenetic groups were described based on 16S rRNA sequences: Marine Group I (MG I) within the Crenarchaeota and Marine Group II (MG II) within the Euryarchaeota. Before this discovery, archaea were considered to be obligate extremophiles. Subsequent cultivation-independent microbial surveys revealed them to be abundant and widespread in relatively “common” environments like soil or the most extensive habitat on Earth, the oligotrophic ocean.
Over the past 22 years, tremendous progress has been made on characterizing MG I, which have been reclassified as the archaeal phylum Thaumarchaeota (Brochier-Armanet et al., 2008). However, our understanding of MG II remains fragmented and significantly less than MG I. In contrast to MG I, no pure cultures or even enrichments are available for MG II. Breakthroughs have been made, however, in understanding the potential physiology and biochemistry of MG II by metagenomic approaches (Iverson et al., 2012; Deschamps et al., 2014; Martin-Cuadrado et al., 2015; Orsi et al., 2015). This mini-review summarizes our current understanding of this group and provides an outlook for future research on MG II.
Abundance and Distribution of MG II and other Euryarchaeota in Temperate Latitudes
Early observations on the distribution of MG II were based on the relative abundance of clones in clone libraries generated from 16S rRNA genes amplified using PCR. In general, MG II contributed more to microbial assemblages from surface than deeper waters (Massana et al., 1997) while MG I were found to have more clones in deeper samples, which led to the conclusion that MG II dominate the photic zone and MG I the meso- and bathypelagic waters (Massana et al., 1997, 2000).
The first reliable quantitative analysis of their distribution was provided by fluorescence in situ hybridization (FISH), using either oligo-probes (oligoFISH) or poly-probes (polyFISH). The former tended to give higher estimates of the abundance of all archaea than the sum of MG I and MG II determined by polyFISH (Pernthaler et al., 2002) (Table 1). MG II were estimated to represent around 15% of total archaeal cells in the Atlantic Ocean, with little variation with depth (Teira et al., 2004). In contrast, a time series assessment of planktonic archaea in the Santa Barbara Channel revealed “intermittent” blooms of MG II coinciding with decreases in chlorophyll a (Murray et al., 1999). Another seasonal study of surface water at the German Bight in the North Sea showed a spring bloom of MG II, when they could be >30% of total cell counts and >90% of all archaeal cells (Pernthaler et al., 2002). Catalyzed reporter deposition-FISH (CARD-FISH) using specific oligonucleotide probes improved the quality of FISH quantification (Teira et al., 2004; Herndl et al., 2005) (Table 1). For example, Herndl et al. (2005) reported that the CARD-FISH method yielded twice as many euryarchaeotal cells than the oligoFISH method.
Quantitative PCR (qPCR) was used to estimate the relative abundance of different archaea in surface waters of Blanes Bay in the NW Mediterranean Sea (Galand et al., 2010). This was the first multiple year study showing seasonal variation within MG II, which was divided into two distinct lineages, II.a and II.b, with MG II.b being more abundant during winter mixing when nutrients are more abundant and MG II.a being predominant in summer when nutrients become depleted (Galand et al., 2010) (Table 1). This study led to a better understanding of the distinct ecological roles of MG II in this region and revealed seasonal variation in activity levels or growth rates that are possibly driven by different metabolism and life strategies (Hugoni et al., 2013).
Abundance and Distribution of MG II and other Euryarchaeota in Polar Oceans
Early studies of planktonic archaea in polar oceans were carried out in surface water near Arthur Harbor on the Antarctic Peninsula. These studies revealed that planktonic archaea could count for up to 34% of the prokaryotic cell abundance (DeLong et al., 1994) (Table 1). Later studies (Murray et al., 1998; DeLong et al., 1999) in the same region reported the predominance of MG I over MG II in winter surface water. Sampling in a more dynamic aquatic system in the center of the Gerlache Strait on the Antarctic Peninsula revealed varying contribution of MG II to total planktonic archaea at different depths, with a larger fraction of MG II contributing to the archaeal assemblage at the surface than at depth (Massana et al., 1998).
A comprehensive polyFISH approach was applied to examine the waters off the Antarctic Peninsula for vertical and temporal changes in archaeal cell abundance (Church et al., 2003). The results showed that MG II was low in abundance (<10% of total picoplankton) throughout the water column and did not differ significantly between summer and winter (Church et al., 2003). Based on these results and those of others (DeLong et al., 1998; Massana et al., 1998, 2000; Murray et al., 1999), Church et al. (2003) concluded that MG II were not numerically abundant in the plankton communities of the Southern Ocean. The occurrence of marine MG III and MG IV, two new lineages of Euryarchaeota that are closely related to MG II, has also been observed in deep waters of the Antarctic Polar Front (López-Garcìa et al., 2001a,b).
Bano et al. (2004) were the first to report on the occurrence of MG II, MG III, and MG IV in the Arctic Ocean (Table 1). They also made a comparison of archaeal distribution between Arctic and Antarctic waters and observed that some euryarchaeotal ribotypes were unique to each system. Galand et al. (2006) further observed that MG II were actually the most common archaeal group in 16S rRNA gene clone libraries constructed from the coastal Beaufort Sea. They speculated that the greater abundance of MG II in the Arctic Ocean may be related to the higher availability of labile organic matter from land surrounding the Arctic, as demonstrated by the significant impact of terrestrially derived particles on coastal archaea in the Mackenzie River-influenced Beaufort Sea (Galand et al., 2006, 2008; Wells et al., 2006). Euryarchaeotal abundance was very low throughout the year in surface waters of the Western Arctic (Kirchman et al., 2007; Alonso-Sáez et al., 2008) (Table 1), which lacked direct river inputs (Galand et al., 2008).
Diversity and Phylogeny of MG II and other Euryarchaeota
Early studies using 16S rRNA gene phylogenies showed limited diversity of the MG II group and placed it in close association with Thermoplasma or methanogens (DeLong, 1992; Fuhrman et al., 1992; Massana et al., 1997, 2000). Nevertheless, two major clusters within MG II (which became the MG II.a- and MG II.b lineages later on) and a third branch (MG III) within the Euryarchaeota were also identified in these studies (Fuhrman et al., 1992; Massana et al., 2000).
Our knowledge of MG II diversity was enhanced by the application of high throughput sequencing and metagenomic analysis to studies of bacterial community composition, which revealed expansive diversity of MG II in coastal or estuarine waters. For example, Bano et al. (2004) observed that certain MG II sequences were predominately found in the surface water, the mixed-layer, or the halocline in the Arctic Ocean. Similarly, Galand et al. (2009a) observed a higher diversity of MG II in Arctic surface water in comparison to MG III that were more diverse in deeper Arctic water. Liu et al. (2009) observed that MG II had the greatest diversity in surface water, which decreased with depth in the Gulf of Mexico. Lincoln et al. (2014a) reported that MG II contributed a much higher proportion to total archaeal operational taxonomic units (OTUs) at shallower depths in the Pacific Ocean; however, different individual OTUs dominated the MG II population at different depths. The predominance of MG II in surface water and MG III in deep water was also observed in the South China Sea (Tseng et al., 2015). Overall, MG I share >94% 16S rRNA gene sequence similarity, whereas MG II only share 85% (Massana et al., 2000; Bano et al., 2004; Herfort et al., 2007), hence, MG II appear to be more phylogenetically diverse than MG I.
Genomics and Metagenomics of MG II and their Predicted Physiology
The distributions and abundances of MG II ribotypes (as well as MG III or MG IV) indicate that planktonic Euryarchaeota occupy diverse ecological niches (Murray et al., 1999; Hugoni et al., 2013; Lincoln et al., 2014a). However, little is known about the underlying mechanisms of niche partitioning among these organisms. The differences in distributions of MG II.a and MG II.b during different seasons observed in the Mediterranean Sea were attributed to differential sensitivity to temperature or nutrient and oxygen availability (Hugoni et al., 2013). Overall, our understanding of the ecological and biogeochemical functions of MG II is very fragmentary and incomplete, largely due to the lack of pure cultures and whole genomes that would allow us to better study the physiology and biochemistry of these organisms. However, information from metagenomics and reconstructed or partially assembled genomes is providing insights about these microbes.
Light-harvesting capability of MG II was first deduced from genomic fragments containing proteorhodopsins (Frigaard et al., 2006). So far only MG II from the photic zone are found to contain the proteorhodopsin gene, which occurs in about 10% of the euryarchaeal population (Frigaard et al., 2006). It has been suggested that proteorhodopsins could support a photoheterotrophic lifestyle by generating a light-driven chemiosmotic potential (Frigaard et al., 2006; Iverson et al., 2012). Summer peaks of abundance and activity of MG II.a in surface water of the Mediterranean Sea were attributed to the enhanced phototrophy in response to greater irradiance (Hugoni et al., 2013).
Despite the identification of proteorhodopsins in MG II genomes, Deschamps et al. (2014) did not find any genes encoding proteorhodopsin homologs in a MG II/III-Euryarchaeota dataset of nine metagenomes from deep-Mediterranean waters. Similarly, Baker et al. (2013) failed to detect any proteorhodopsin genes in the deep Guaymas metatranscriptome. These results support an earlier conclusion that deep-sea dwelling MG II Euryarchaeota are different from proteorhodopsin-containing MG II living in the ocean photic zone (Frigaard et al., 2006). Deschamps et al. (2014) also noted that the deep sea MG II Euryarchaeota have abundant genes targeting amino acid, carbohydrate and lipid transport and metabolism, which are typical of heterotrophic prokaryotes. Orsi et al. (2015) found that the rhodopsins of MG II in metagenomes from large cell size fractions (>0.8 μm) are phylogenetically distinct from rhodopsin genes found in metagenomes obtained with smaller size fractions, suggesting a possible difference in photoheterotrophy between the free-living and the particle-associated MG II groups.
Iverson et al. (2012) obtained the first nearly complete genome of a MG II, belonging to subgroup II.a, from metagenomic assemblies obtained from surface seawater in the Puget Sound. The genome suggested that MG II.a was a particle-associated microbe and the cells were predicted to be motile, photo-heterotrophic and capable of degrading polymers such as proteins and lipids. This validated the prediction of Béjà et al. (2000) that surface MG II were capable of proteolysis. The deep water genomic fragments (Moreira et al., 2004; Martin-Cuadrado et al., 2008; Deschamps et al., 2014) do not seem to have this metabolic feature. More recently, Orsi et al. (2015) quantified the abundance and distribution of MG II 16S rRNA genes in size-fractionated seawater samples from the euphotic zone of the central California Current System and showed that MG II abundance was highest in the particulate fraction, indicating that some MG II euryarchaeotes were physically associated with particles. These authors also found that the genome content of particle-attached MG II suggested an increased capacity for surface adhesion, transcriptional regulation and catabolism of high molecular weight substrates.
Another genome of MG II was assembled from the Mediterranean deep chlorophyll maximum (DCM), which belonged to subgroup II.b (Martin-Cuadrado et al., 2015). The authors used FISH to detect these cells in DCM samples and proposed the Class Thalassoarchaea (archaea from the sea) to name members of the MG II.b. They also confirmed by recruitment of genomic fragments from the Mediterranean Sea and Puget Sound that Thalassoarchaea are inhabitants of the oligotrophic photic zone while MG II.a are adapted to more coastal or even estuarine (brackish) habitats, which indicate that subgroups of MG II may represent different ecotypes. Two recent metatranscriptomic studies (Baker et al., 2013; Ottesen et al., 2013) found that MG II transcripts were present at levels similar to numerically dominant groups like Pelagibacter or SAR86. Actually, metatranscriptomic data show in general much greater contribution of MG II than their expected numbers from metagenomics would predict, suggesting that MG II populations may be very dynamic and capable of responding rapidly to changing conditions.
A diagram summarizing the key metabolic functions of the MG II as derived from metagenomics is displayed in Figure 1. In general, MG II metabolic genes include those encoding functions associated with glycolysis, the tricarboxylic acid cycle, and phosphorylation complexes indicative of aerobic respiration. However, the identification of some of the genes of the assimilatory sulfate reduction pathway in the Thalassoarchaea genomic fragments (Martin-Cuadrado et al., 2008, 2015) suggests capacity for anaerobic respiration within low-oxygen microenvironments such as organic particles, which is supported by Orsi et al. (2015). In addition, a complete non-oxidative pentose phosphate pathway was identified in both available genomes of MG II, but some of the enzymes of the irreversible oxidative branch were not found in MG II.b, probably due to the incompleteness of this genome. Several transporters were identified, but the nature of the substrates could not be identified in most of the cases. An agarase-like gene is present in the MG II.b (Figure 1); however, it is unknown whether this group of archaea can use agar as carbon source. Transporters for branched-chain amino acids and di/oligopeptides were very abundant, supporting the model that protein degradation may be important to the metabolism of these microbes. Also, sequences encoding several drug-efflux pumps were abundant in both clades, which was suggested to indicate a defensive life style, typical of organisms exposed to natural toxin, i.e., from blooms of cyanobacteria producing marine biotoxins (Martin-Cuadrado et al., 2015).
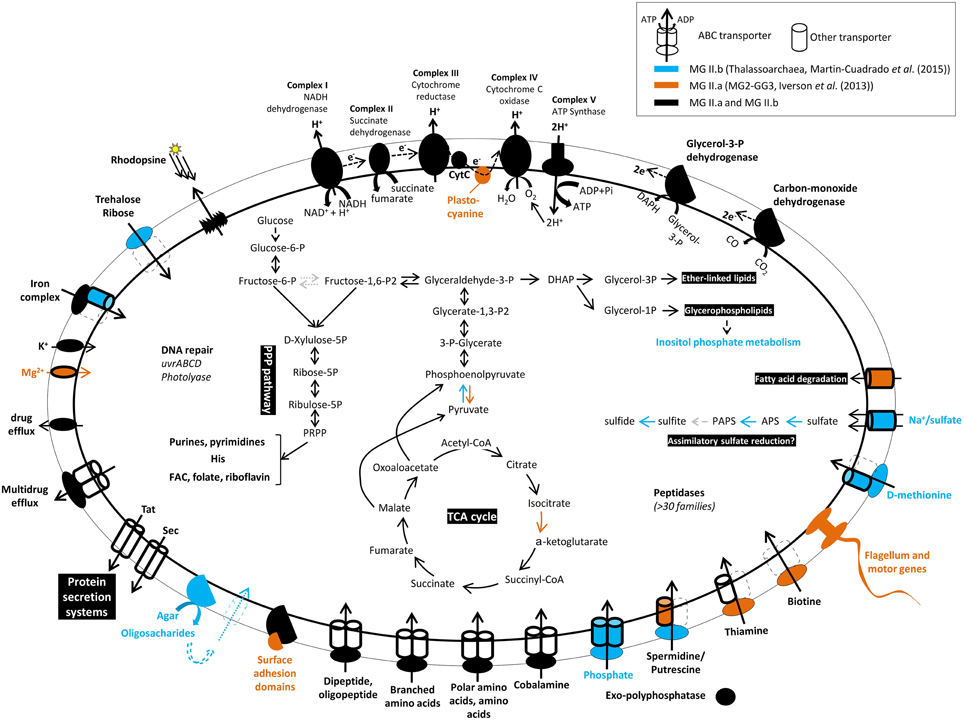
Figure 1. Predicted metabolism of Marine Group II Archaea (groups II.a and II.b) based on genomes reconstructed from metagenomes available in the literature.
Last but not least, the biogeochemical function of MG II is receiving increasing attention. The TEX86 paleo sea surface temperature proxy was originally based on the premise that archaeal lipids used in formulating the proxy are solely produced by Thaumarchaeota living in the surface ocean (Schouten et al., 2002). However, evidence increasingly demonstrates that temperature is not the only variable affecting the tetraether lipid distribution in the ocean (see reviews by Pearson and Ingalls, 2013; Schouten et al., 2013). In particular, the biological source of tetraether lipids in the ocean requires further consideration. Recently, Lincoln et al. (2014a) and Wang et al. (2015) provide evidence supporting an earlier speculation (Turich et al., 2007) that MG II may be significant contributors to tetraether lipids in coastal and open oceans. This is, however, still a highly debated topic (Lincoln et al., 2014b; Schouten et al., 2014) and a critical step and potentially growing area of research is verifying tetraether production by MG II under controlled laboratory conditions, using either enrichment or, hopefully, pure cultures.
Summary and Future Research Directions in Marine Archaea
Archaea are now recognized as equally important as bacteria in the global ocean carbon cycle. Advances in MG I (Thaumarchaeota) research over the past two decades have been tremendous, particularly in our understanding of the ecological and biogeochemical functions of these organisms. In comparison, our understanding of MG II, despite their widespread occurrence in the ocean, is still rudimentary. Recently, the concept of the microbial carbon pump (Jiao et al., 2010) further highlighted the importance of microorganisms (including both archaea and bacteria) in long term storage of dissolved organic carbon, which is the largest pool of organic carbon in the ocean. While a number of issues hinder advancing our understanding of the microbial processes mediating ocean biogeochemistry, the lack of information on archaea is particularly severe (Kujawinski, 2011). Archaea have several unique features that distinguish them from bacteria, including membrane lipids that are more resistant to degradation than bacterial lipids and capability in surviving harsh environments in which carbon metabolism by other organisms may be inhibited. Future research on marine archaea, including both MG I and MG II, may focus on the following topics in order of importance and ease of accomplishment for the fields of microbial ecology and biogeochemistry:
1. Increasing genomic coverage of MG II lineages using metagenomics and single-cell genomics.
2. Enriching and isolating MG II followed by physiological and biochemical studies.
3. Validating the lipid composition of MG II and re-evaluating the sources of GDGTs in the open ocean.
4. Distinguishing relative contributions of archaea and bacteria in the production and transformation of recalcitrant dissolved organic carbon in the ocean.
5. Elucidating the evolutionary and/or horizontal gene transfer pathways of MG II in contrast to or in concert with MG I.
Conflict of Interest Statement
The authors declare that the research was conducted in the absence of any commercial or financial relationships that could be construed as a potential conflict of interest.
Acknowledgments
We thank Tommy Phelps for inspiring discussion on MG II. Constructive comments from Meng Li, James Hollibaugh and Jeremy Dodsworth improved the quality of an earlier version of the paper. Constructive comments from the two reviewers are greatly appreciated. The MG II research at Tongji University is supported by the Ministry of Science and Technology (Award No. 2013CB955703 to CLZ), the National Science Foundation of China (Award Nos. 41530105, 91028005 and 91428308 to CLZ), and the “National Thousand Talents” program through the State Key Laboratory of Marine Geology at Tongji University (CLZ). FR-V laboratory is supported by projects MEDIMAX BFPU2013-48007-P from the Spanish Ministerio de Economía y Competitividad, MaCuMBA Project 311975 of the European Commission FP7 and PROMETEO II/2014/012 project AQUAMET from the Generalitat Valenciana.
References
Alonso-Sáez, L., Sánchez, O., Gasol, J. M., Balagué, V., and Pedrós-Alio, C. (2008). Winter-to-summer changes in the composition and single-cell activity of near-surface Arctic prokaryotes. Environ. Microbiol. 10, 2444–2454. doi: 10.1111/j.1462-2920.2008.01674.x
Baker, B. J., Sheik, C. S., Taylor, C. A., Jain, S., Bhasi, A., Cavalcoli, J. D., et al. (2013). Community transcriptomic assembly reveals microbes that contribute to deep-sea carbon and nitrogen cycling. ISME J. 7, 1962–1973. doi: 10.1038/ismej.2013.85
Bano, N., Ruffin, S., Ransom, B., and Hollibaugh, J. T. (2004). Phylogenetic composition of Arctic Ocean Archaeal assemblages and comparison with Antarctic assemblages. Appl. Environ. Microbiol. 70, 781–789. doi: 10.1128/AEM.70.2.781-789.2004
Béjà, O., Suzuki, M. T., Koonin, E. V., Aravind, L., Hadd, A., Nguyen, L. P., et al. (2000). Construction and analysis of bacterial artificial chromosome libraries from a marine microbial assemblage. Environ. Microbiol. 2, 516–529. doi: 10.1046/j.1462-2920.2000.00133.x
Brochier-Armanet, C., Boussau, B., Gribaldo, S., and Forterre, P. (2008). Mesophilic Crenarchaeota: proposal for a third archaeal phylum, the Thaumarchaeota. Nat. Rev. Microbiol. 6, 245–252. doi: 10.1038/nrmicro1852
Church, M., DeLong, E., Ducklow, H., Karner, M., Preston, C., and Karl, D. (2003). Abundance and distribution of planktonic Archaea and Bacteria in the waters west of the Antarctic Peninsula. Limnol. Oceanogr. 48, 1893–1902. doi: 10.4319/lo.2003.48.5.1893
DeLong, E. F. (1992). Archaea in coastal marine environments. Proc. Natl. Acad. Sci. U.S.A. 89, 5685–5689. doi: 10.1073/pnas.89.12.5685
DeLong, E. F., King, L. L., Massana, R., Cittone, H., Murray, A., Schleper, C., et al. (1998). Dibiphytanyl ether lipids in nonthermophilic crenarchaeotes. Appl. Environ. Microbiol. 64, 1133–1138.
DeLong, E. F., Taylor, L. T., Marsh, T. L., and Preston, C. M. (1999). Visualization and enumeration of marine planktonic archaea and bacteria by using polyribonucleotide probes and fluorescent in situ hybridization. Appl. Environ. Microbiol. 65, 5554–5563.
DeLong, E. F., Wu, K. Y., Prézelin, B. B., and Jovine, R. V. (1994). High abundance of Archaea in Antarctic marine picoplankton. Nature 371, 695–697. doi: 10.1038/371695a0
Deschamps, P., Zivanovic, Y., Moreira, D., Rodriguez-Valera, F., and López-García, P. (2014). Pangenome evidence for extensive interdomain horizontal transfer affecting lineage core and shell genes in uncultured planktonic thaumarchaeota and euryarchaeota. Genome Biol. Evol. 6, 1549–1563. doi: 10.1093/gbe/evu127
Frigaard, N. U., Martinez, A., Mincer, T. J., and DeLong, E. F. (2006). Proteorhodopsin lateral gene transfer between marine planktonic Bacteria and Archaea. Nature 439, 847–850. doi: 10.1038/nature04435
Fuhrman, J. A., McCallum, K., and Davis, A. A. (1992). Novel major archaebacterial group from marine plankton. Nature 356, 148–149.
Fuhrman, J. A., and Davis, A. A. (1997). Widespread Archaea and novel Bacteria from the deep sea as shown by 16S rRNA gene sequences. Mar. Ecol. Prog. Ser. 150, 275–285. doi: 10.3354/meps150275
Galand, P. E., Casamayor, E. O., Kirchman, D. L., Potvin, M., and Lovejoy, C. (2009a). Unique archaeal assemblages in the Arctic Ocean unveiled by massively parallel tag sequencing. ISME J. 3, 860–869. doi: 10.1038/ismej.2009.23
Galand, P. E., Gutiérrez-Provecho, C., Massana, R., Gasol, J. M., and Casamayor, E. O. (2010). Inter-annual recurrence of archaeal assemblages in the coastal NW Mediterranean Sea (Blanes Bay Microbial Observatory). Limnol. Oceanogr. 55, 2117–2125. doi: 10.4319/lo.2010.55.5.2117
Galand, P. E., Lovejoy, C., Hamilton, A. K., Ingram, R. G., Pedneault, E., and Carmack, E. C. (2009b). Archaeal diversity and a gene for ammonia oxidation are coupled to oceanic circulation. Environ. Microbiol. 11, 971–980. doi: 10.1111/j.1462-2920.2008.01822.x
Galand, P. E., Lovejoy, C., Pouliot, J., and Vincent, W. F. (2008). Heterogeneous archaeal communities in the particle-rich environment of an arctic shelf ecosystem. J. Mar. Syst. 74, 774–782. doi: 10.1016/j.jmarsys.2007.12.001
Galand, P. E., Lovejoy, C., and Vincent, W. F. (2006). Remarkably diverse and contrasting archaeal communities in a large arctic river and the coastal Arctic Ocean. Aquat. Microb. Ecol. 44, 115–126. doi: 10.3354/ame044115
Herfort, L., Schouten, S., Abbas, B., Veldhuis, M. J., Coolen, M. J., Wuchter, C., et al. (2007). Variations in spatial and temporal distribution of Archaea in the North Sea in relation to environmental variables. FEMS Microb. Ecol. 62, 242–257. doi: 10.1111/j.1574-6941.2007.00397.x
Herndl, G. J., Reinthaler, T., Teira, E., van Aken, H., Veth, C., Pernthaler, A., et al. (2005). Contribution of Archaea to total prokaryotic production in the deep Atlantic Ocean. Appl. Environ. Microbiol. 71, 2303–2309. doi: 10.1128/AEM.71.5.2303-2309.2005
Hugoni, M., Taib, N., Debroas, D., Domaizon, I., Dufournel, I. J., Bronner, G., et al. (2013). Structure of the rare archaeal biosphere and seasonal dynamics of active ecotypes in surface coastal waters. Proc. Natl. Acad. Sci. U.S.A. 110, 6004–6009. doi: 10.1073/pnas.1216863110
Iverson, V., Morris, R. M., Frazar, C. D., Berthiaume, C. T., Morales, R. L., and Armbrust, E. V. (2012). Untangling genomes from metagenomes: revealing an uncultured class of marine Euryarchaeota. Science 335, 587–590. doi: 10.1126/science.1212665
Jiao, N., Herndl, G. J., Hansell, D. A., Benner, R., Kattner, G., Wilhelm, S. W., et al. (2010). Microbial production of recalcitrant dissolved organic matter: long-term carbon storage in the global ocean. Nat. Rev. Microbiol. 8, 593–599. doi: 10.1038/nrmicro2386
Karner, M. B., DeLong, E. F., and Karl, D. M. (2001). Archaeal dominance in the mesopelagic zone of the Pacific Ocean. Nature 409, 507–510. doi: 10.1038/35054051
Kirchman, D. L., Elifantz, H., Dittel, A. I., Malmstrom, R. R., and Cottrell, M. T. (2007). Standing stocks and activity of Archaea and Bacteria in the western Arctic Ocean. Limnol. Oceanogr. 52, 495–507. doi: 10.4319/lo.2007.52.2.0495
Kujawinski, E. B. (2011). The impact of microbial metabolism on marine dissolved organic matter. Ann. Rev. Mar. Sci. 3, 567–599. doi: 10.1146/annurev-marine-120308-081003
Lincoln, S. A., Wai, B., Eppley, J. M., Church, M. J., Summons, R. E., and DeLong, E. F. (2014a). Planktonic Euryarchaeota are a significant source of archaeal tetraether lipids in the ocean. Proc. Natl. Acad. Sci. U.S.A. 111, 9858–9863. doi: 10.1073/pnas.1409439111
Lincoln, S. A., Wai, B., Eppley, J. M., Church, M. J., Summons, R. E., and DeLong, E. F. (2014b). Reply to Schouten et al.: Marine Group II planktonic Euryarchaeota are significant contributors to tetraether lipids in the ocean. Proc. Natl. Acad. Sci. U.S.A. 111, E4286. doi: 10.1073/pnas.1416736111
Liu, B., Ye, G., Wang, F., Bell, R., Noakes, J., Short, T., et al. (2009). Community structure of archaea in the water column above gas hydrates in the Gulf of Mexico. Geomicrobiol. J. 26, 363–369. doi: 10.1080/01490450902929290
López-Garcìa, P., López-López, A., Moreira, D., and Rodrìguez-Valera, F. (2001a). Diversity of free-living prokaryotes from a deep-sea site at the Antarctic Polar Front. FEMS Microb. Ecol. 36, 193–202. doi: 10.1111/j.1574-6941.2001.tb00840.x
López-Garcìa, P., Moreira, D., López-López, A., and Rodrìguez-Valera, F. (2001b). A novel haloarchaeal-related lineage is widely distributed in deep oceanic regions. Environ. Microbiol. 3, 72–78. doi: 10.1046/j.1462-2920.2001.00162.x
Martin-Cuadrado, A.-B., Garcia-Heredia, I., Moltó, A. G., López-Úbeda, R., Kimes, N., López-García, P., et al. (2015). A new class of marine Euryarchaeota group II from the mediterranean deep chlorophyll maximum. ISME J. 9, 1619–1634. doi: 10.1038/ismej.2014.249
Martin-Cuadrado, A. B., Rodriguez-Valera, F., Moreira, D., Alba, J. C., Ivars-Martinez, E., Henn, M. R., et al. (2008). Hindsight in the relative abundance, metabolic potential and genome dynamics of uncultivated marine archaea from comparative metagenomic analyses of bathypelagic plankton of different oceanic regions. ISME J. 2, 865–886. doi: 10.1038/ismej.2008.40
Massana, R., DeLong, E. F., and Pedrós-Alió, C. (2000). A few cosmopolitan phylotypes dominate planktonic archaeal assemblages in widely different oceanic provinces. Appl. Environ. Microbiol. 66, 1777–1787. doi: 10.1128/AEM.66.5.1777-1787.2000
Massana, R., Murray, A. E., Preston, C. M., and DeLong, E. F. (1997). Vertical distribution and phylogenetic characterization of marine planktonic Archaea in the Santa Barbara Channel. Appl. Environ. Microbiol. 63, 50–56.
Massana, R., Taylor, L. T., Murray, A. E., Wu, K. Y., Jeffrey, W. H., and DeLong, E. F. (1998). Vertical distribution and temporal variation of marine planktonic archaea in the Gerlache Strait, Antarctica, during early spring. Limnol. Oceanogr. 43, 607–617. doi: 10.4319/lo.1998.43.4.0607
Moreira, D., Rodríguez-Valera, F., and López-García, P. (2004). Analysis of a genome fragment of a deep-sea uncultivated Group II euryarchaeote containing 16S rDNA, a spectinomycin-like operon and several energy metabolism genes. Environ. Microbiol. 6, 959–969. doi: 10.1111/j.1462-2920.2004.00644.x
Murray, A., Blakis, A., Massana, R., Strawzewiski, S., Passow, U., Alldredge, A., et al. (1999). A timeseries assessment of planktonic archaeal variability in the Santa Barbara Channel. Aquat. Microb. Ecol. 20, 129–145. doi: 10.3354/ame020129
Murray, A., Preston, C., Massana, R., Taylor, L., Blakis, A., Wu, K., et al. (1998). Seasonal and spatial variability of bacterial and archaeal assemblages in the coastal waters near Anvers Island, Antarctica. Appl. Environ. Microbiol. 64, 2585–2595.
Orsi, W. D., Smith, J. M., Wilcox, H. M., Swalwell, J. E., Carini, P., Worden, A. Z., et al. (2015). Ecophysiology of uncultivated marine euryarchaea is linked to particulate organic matter. ISME J. 9, 1747–1763. doi: 10.1038/ismej.2014.260
Ottesen, E. A., Young, C. R., Eppley, J. M., Ryan, J. P., Chavez, F. P., Scholin, C. A., et al. (2013). Pattern and synchrony of gene expression among sympatric marine microbial populations. Proc. Natl. Acad. Sci. U.S.A. 110, E488–E497. doi: 10.1073/pnas.1222099110
Pearson, A., and Ingalls, A. E. (2013). Assessing the use of archaeal lipids as marine environmental proxies. Annu. Rev. Earth Planet. Sci. 41, 359–384. doi: 10.1146/annurev-earth-050212-123947
Pernthaler, A., Preston, C. M., Pernthaler, J., DeLong, E. F., and Amann, R. (2002). Comparison of fluorescently labeled oligonucleotide and polynucleotide probes for the detection of pelagic marine bacteria and archaea. Appl. Environ. Microbiol. 68, 661–667. doi: 10.1128/AEM.68.2.661-667.2002
Schouten, S., Hopmans, E. C., Schefuß, E., and Sinninghe Damsté, J. S. (2002). Distributional variations in marine crenarchaeotal membrane lipids: a new tool for reconstructing ancient sea water temperatures? Earth Planet. Sci. Letts. 204, 265–274. doi: 10.1016/s0012-821x(02)00979-2
Schouten, S., Hopmans, E. C., and Sinninghe Damsté, J. S. (2013). The organic geochemistry of glycerol dialkyl glycerol tetraether lipids: a review. Org. Geochem. 54, 19–61. doi: 10.1016/j.orggeochem.2012.09.006
Schouten, S., Villanueva, L., Hopmans, E. C., van der Meer, M. T., and Sinninghe Damsté, J. S. (2014). Are marine group II Euryarchaeota significant contributors to tetraether lipids in the ocean? Proc. Natl. Acad. Sci. U.S.A. 111, E4285. doi: 10.1073/pnas.1416176111
Teira, E., Reinthaler, T., Pernthaler, A., Pernthaler, J., and Herndl, G. J. (2004). Combining catalyzed reporter deposition-fluorescence in situ hybridization and microautoradiography to detect substrate utilization by bacteria and Archaea in the deep ocean. Appl. Environ. Microbiol. 70, 4411–4414. doi: 10.1128/AEM.70.7.4411-4414.2004
Teira, E., Van Aken, H., Veth, C., and Herndl, G. J. (2006). Archaeal uptake of enantiomeric amino acids in the meso-and bathypelagic waters of the North Atlantic. Limnol. Oceanogr. 51, 60–69. doi: 10.4319/lo.2006.51.1.0060
Tseng, C.-H., Chiang, P.-W., Lai, H.-C., Shiah, F.-K., Hsu, T.-C., Chen, Y.-L., et al. (2015). Prokaryotic assemblages and metagenomes in pelagic zones of the South China Sea. BMC Genomics 16:219. doi: 10.1186/s12864-015-1434-3
Turich, C., Freeman, K. H., Bruns, M. A., Conte, M., Jones, A. D., and Wakeham, S. G. (2007). Lipids of marine Archaea: patterns and provenance in the water-column and sediments. Geochim. Cosmochim. Acta 71, 3272–3291. doi: 10.1016/j.gca.2007.04.013
Wang, J.-X., Wei, Y., Wang, P., Hong, Y., and Zhang, C. L. (2015). Unusually low TEX 86 values in the transitional zone between Pearl River estuary and coastal South China Sea: impact of changing archaeal community composition. Chem. Geol. 402, 18–29. doi: 10.1016/j.chemgeo.2015.03.002
Wells, L. E., Cordray, M., Bowerman, S., Miller, L. A., Vincent, W. F., and Deming, J. W. (2006). Archaea in particle-rich waters of the Beaufort Shelf and Franklin Bay, Canadian Arctic: clues to an allochthonous origin? Limnol. Oceanogr. 51, 47–59. doi: 10.4319/lo.2006.51.1.0047
Wells, L. E., and Deming, J. W. (2003). Abundance of Bacteria, the Cytophaga-Flavobacterium cluster and Archaea in cold oligotrophic waters and nepheloid layers of the Northwest Passage, Canadian Archipelago. Aquat. Microb. Ecol. 31, 19–31. doi: 10.3354/ame031019
Keywords: archaea, Marine Group II, heterotrophic metabolism, carbon cycle
Citation: Zhang CL, Xie W, Martin-Cuadrado A-B and Rodriguez-Valera F (2015) Marine Group II Archaea, potentially important players in the global ocean carbon cycle. Front. Microbiol. 6:1108. doi: 10.3389/fmicb.2015.01108
Received: 09 July 2015; Accepted: 25 September 2015;
Published: 13 October 2015.
Edited by:
Dennis A. Bazylinski, University of Nevada at Las Vegas, USAReviewed by:
Zhe-Xue Quan, Fudan University, ChinaGerhard J. Josef Herndl, University of Vienna, Austria
Copyright © 2015 Zhang, Xie, Martin-Cuadrado and Rodriguez-Valera. This is an open-access article distributed under the terms of the Creative Commons Attribution License (CC BY). The use, distribution or reproduction in other forums is permitted, provided the original author(s) or licensor are credited and that the original publication in this journal is cited, in accordance with accepted academic practice. No use, distribution or reproduction is permitted which does not comply with these terms.
*Correspondence: Chuanlun L. Zhang, YXJjaGFlYXpoYW5nXzFAdG9uZ2ppLmVkdS5jbg==;
Francisco Rodriguez-Valera, ZnJ2YWxlcmFAdW1oLmVz