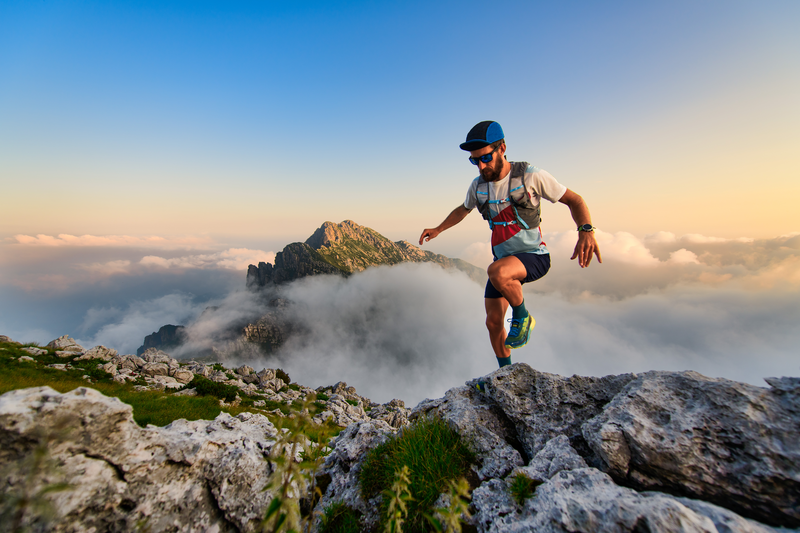
95% of researchers rate our articles as excellent or good
Learn more about the work of our research integrity team to safeguard the quality of each article we publish.
Find out more
ORIGINAL RESEARCH article
Front. Microbiol. , 08 September 2015
Sec. Food Microbiology
Volume 6 - 2015 | https://doi.org/10.3389/fmicb.2015.00907
This article is part of the Research Topic Regulation of gene expression in enteropathogenic bacteria View all 42 articles
The objective of this study was to investigate the effect of Mg2+ ions on biofilm formation by Bacillus species, which are considered as problematic microorganisms in the food industry. We found that magnesium ions are capable to inhibit significantly biofilm formation of Bacillus species at 50 mM concentration and higher. We further report that Mg2+ ions don't inhibit bacterial growth at elevated concentrations; hence, the mode of action of Mg2+ ions is apparently specific to inhibition of biofilm formation. Biofilm formation depends on the synthesis of extracellular matrix, whose production in Bacillus subtilis is specified by two major operons: the epsA-O and tapA operons. We analyzed the effect of Mg2+ ions on matrix gene expression using transcriptional fusions of the promoters for eps and tapA to the gene encoding β galactosidase. The expression of the two matrix operons was reduced drastically in response to Mg2+ ions suggesting about their inhibitory effect on expression of the matrix genes in B. subtilis. Since the matrix gene expression is tightly controlled by Spo0A dependent pathway, we conclude that Mg2+ ions could affect the signal transduction for biofilm formation through this pathway.
The vast majority of bacteria often grow as elaborate multicellular communities, referred to as biofilms (Hall-Stoodley et al., 2004; Kolter and Greenberg, 2006). Biofilm formation represents one of the most successful strategies for survival in natural environments, which protect bacteria and facilitates growth under unfavorable conditions, such as turbulent flow or limited access to nutrients (Stewart and Costerton, 2001; Hall-Stoodley et al., 2004). Biofilm formation is a multistage process in which cells adhere to a surface through production of an extracellular matrix that is typically composed of polysaccharides, proteins, and nucleic acids (Flemming and Wingender, 2010). These exopolymeric substances often surround and protect the bacteria (Shemesh et al., 2010). Thus, biofilm bacteria are more resistant than planktonic cells to various antimicrobials (Costerton, 1999; Mah and O'Toole, 2001).
Biofilms are problematic in a broad range of areas, and specifically in the food, environmental, and biomedical fields (Simoes et al., 2010). Within food industry, biofilm formation in dairy processing plants is a most significant problem. The major source of the contamination of dairy products is often associated with biofilms (Flint et al., 1997), particularly biofilms formed by members of the Bacillus genus (Sharma and Anand, 2002; Simoes et al., 2010). As Bacillus species are ubiquitously present in nature, they easily spread through food production systems, and contamination with these species is almost inevitable. The biofilm formed by thermo-resistant Bacillus species in a milk line can rapidly grow to such an extent that the passing milk is contaminated with cells released from the biofilm (Wirtanen et al., 1996). Thus, biofilms formed by Bacillus species is the major type of hygiene problems in dairy industry.
Clearly, preventing biofilm formation would be a much more desirable option than affecting it in the maturation stage, therefore a range of antimicrobial strategies have been proposed to control biofilms. However, conventional cleaning and disinfection regimens or present antimicrobial strategies may contribute to inefficient biofilm control and to the dissemination of resistance (Simoes et al., 2010). Hence, techniques that are able to prevent or control the formation of unwanted biofilms may have adverse side effects. Therefore, it necessitates looking for other methods to prevent and eradicate bacterial biofilms more successfully.
Environmental factors such as electrolyte concentrations and medium composition may have important impacts on biofilm formation (Song and Leff, 2006; Shemesh et al., 2007). Divalent cations, such as Mg2+ and Ca2+, can influence biofilm formation directly through their effect on electro-static interactions and indirectly via physiology-dependent attachment processes by acting as important cellular cations and enzyme cofactors (Fletcher, 1988; Malik and Kakii, 2003; Song and Leff, 2006). In spite of the potentially important role, the effect of Mg2+ ions on bacterial adhesion and biofilm formation has barely been studied. Mg2+ has been shown to influence adherence to surfaces in Pseudomonas spp. (Simoni et al., 2000). Moreover, in Aeromonas hydrophilia, mutations in Mg2+ transport systems result in reduction of swarming and biofilm formation (Merino et al., 2001). Accordingly to Dunne and Burd (1992), 16 mM of Mg2+ significantly enhanced in vitro adhesion of Staphylococcus epidermidis to plastic (Dunne and Burd, 1992). In addition, it was reported that increase in Mg2+ concentrations affected positively on biofilm formation by P. fluorescens (Song and Leff, 2006). Also, it was hypothesized that low Ca2+ or Mg2+ concentrations have the potential to inhibit biofilm formation by some A. flavithermus and Geobacillus spp. strains during the processing of milk formulations (Somerton et al., 2013). Another recent study has showed that biofilm formation decreased with increasing concentration of Mg2+ in Enterobacter cloacae (Zhou et al., 2014). Although recent studies have shown that magnesium might have diverse effects on biofilms, the effect of Mg2+ ions on biofilm formation by sporulation Bacillus species remains largely unknown. Therefore, the purpose of this study was to investigate the effect of Mg2+ ions on biofilm formation by Bacillus species, which are potential importance of biofilm formation in food industrial settings.
The Bacillus subtilis wild strain NCIB3610 (Branda et al., 2001) and Bacillus cereus ATCC 10987 stain, obtained from Michel Gohar's lab collection (INRA, France), were used in this study. For fluorescent microscopy, we used a strain (YC161 with Pspank-gfp) that produced GFP constitutively (Chai et al., 2011) which was obtained from the laboratory collection of Yunrong Chai (Northeastern University, USA). For routine growth, all strains were propagated in Lysogeny broth (LB; 10 g of tryptone, 5 g of yeast extract and 5 g of NaCl per liter) or on solid LB medium supplemented with 1.5% agar. For biofilm generation, bacteria were grown to stationary phase in LB medium at 37°C in shaking culture to around 1 × 108 CFU per ml. Biofilms were generated at 30°C in the biofilm promoting medium LBGM (LB + 1% (v/v) glycerol + 0.1 mM MnSO4) (Shemesh and Chai, 2013). To test the effect of magnesium, sodium or calcium ions on biofilm formation, different concentrations of either MgCl2 (Merck KGaA), NaCl (BIO LAB LTD), or CaCl2 (Merck KGaA) were added directly into the LBGM medium. For colony type biofilm formation, 3 μl of the cells (around 3 × 105 CFU) was spotted onto LBGM medium solidified with 1.5% agar as described previously (Shemesh and Chai, 2013). Plates were incubated at 30°C for 72 h prior to analysis. For pellicle formation, 5 μl of the cells (around 5 × 105 CFU) was mixed within 4 ml of LBGM broth in 12-well plates (Costar). Plates were incubated at 30°C for 24 h. Images were taken using a Zeiss Stemi 2000-C microscope with an axiocam ERc 5s camera.
For experiments performed with B. cereus, bacteria were grown to stationary phase in LB medium at 37°C in shaking culture to around 5 × 107 CFU per ml. For pellicle formation, 5 μl of the cells (around 2.5 × 105 CFU) was mixed within 4 ml of LBGM broth in glass tubes in the presence or absence of different concentration of MgCl2. The glass tubes were incubated at 30°C for 24 h.
To analyze the effect of magnesium ions on matrix gene expression we used transcriptional fusions of the promoters for eps and tapA to the gene encoding β galactosidase (Chai et al., 2008). Samples of generated pellicles as described above were collected and resuspended in phosphate-buffered saline (PBS) buffer. Typical long bundled chains of cells in the biofilm colony were disrupted using mild sonication as described previously (Branda et al., 2006). Optical density of the cell samples was normalized using OD600. One milliliter of cell suspensions was collected and assayed for β-Galactosidase activity as described previously (Chai et al., 2008).
Initially, the cells were grown in shaking cultures over night at 23°C/150 rpm in LB to around 2 × 109 CFU per ml. On the next morning, the cultures were diluted 1:100 (to around 2 × 107 CFU) into LBGM with or without addition of different concentration of MgCl2 and incubated at 37°C at 150 rpm. The absorbance of the cultures at 600 nm was measured periodically for each culture for 9 h. Each condition had three replicates, and the growth curve experiments were repeated twice. Representative results are shown.
For fluorescent microscopy, we used a strain YC161 that produced GFP constitutively. The strain was first grown in shaking culture for 5 h 37°C/150 rpm in LB to around 1 × 108 CFU per ml. Next, 5 μl (around 5 × 105 CFU) of suspension from the generated culture was introduced into 4 ml of LBGM medium and incubated at 30°C for 24 h statically. Afterwards, one milliliter of suspension from each sample was collected, mildly sonicated (10 s/20% Amp/5) and centrifuged at 5000 rpm for 2 min. Next, the supernatant was removed and the pellet was resuspended by pipetation. For microscopic observation, 3 μl from the samples were transferred onto a glass slide and visualized in a transmitted light microscope using Nomarski differential interference contrast (DIC), at x40 magnification. A confocal laser scanning microscope was used to visualize GFP expression of strain YC161 using an Olympus IX81 confocal laser scanning microscope (CLSM) (Japan) equipped with 488 nm argon-ion and 543 nm helium neon lasers. For experiments performed with B. cereus, the cells were stained with CYTO 9 from the FilmTracer™ LIVE/DEAD Biofilm Viability Kit (Molecular Probes, OR) following instructions of the manufacturer. Fluorescence emission of the stained samples was determined using an Olympus IX81 confocal laser scanning microscope (Japan) equipped with 488 nm argon-ion and 543 nm helium neon lasers.
Statistical analysis was performed using T-test to compare the control and tested samples. Statistical significant was determined at P < 0.05.
The starting point of this investigation was the observation that at the elevated concentrations Mg2+ ions could inhibit biofilm formation by B. subtilis. As seen in Figure 1, Mg2+ ions inhibited notably pellicle formation by B. subtilis in a concentration dependent manner. The inhibitory effect of Mg2+ ions was not restricted to MgCl2 compound since we found that other magnesium salts, such as MgSO4 have also inhibited the pellicle formation (data not shown). This indicates that the inhibitory effect of magnesium salts is attributed to Mg2+ ions. Moreover, colony type biofilm formation was also inhibited significantly in the presence of high concentrations of Mg2+ ions (Figure 1).
Figure 1. Mg2+ ions block biofilm formation of B. subtilis. The effect of addition of different concentrations of MgCl2 to LBGM medium on pellicle and colony biofilm formation by B. subtilis NCIB3610.
To confirm that the significant inhibition in biofilm formation by Mg2+ ions is not a result of toxicity to bacterial cells, we tested the effect of different concentrations of Mg2+ ions on bacterial growth. As shown in Supplementary Figure 1, the growth curve analysis suggests about very little effect of the Mg2+ ions on bacterial growth at the tested concentrations; hence, the mode of action of Mg2+ ions is apparently specific to inhibition of biofilm formation.
Biofilm formation depends on the synthesis of extracellular matrix, whose production in B. subtilis is specified by two major operons: the epsA-O and tapA operons (Kearns et al., 2005; Branda et al., 2006; Chu et al., 2006). The epsA-O operon is responsible for the production of the exopolysaccharides whereas the tapA operon is responsible for the production of amyloid-like fibers (Chai et al., 2008; Romero et al., 2010). We hypothesized that the inhibitory effect of Mg2+ ions on biofilm formation could be due to down-regulation of the genes involved in matrix synthesis. To test this hypothesis, we analyzed the effect of Mg2+ ions on matrix gene expression using transcriptional fusions of the promoters for epsA-O and tapA to the gene encoding β galactosidase. The expression of the matrix operons was notably reduced in response to the addition of Mg2+ ions (Figure 2A). The reduction in eps expression was relatively small (around four-fold) but significant, while tapA expression was decreased almost 14.5-fold at elevated concentrations of Mg2+ ions (Figure 2A). This result suggests that addition of Mg2+ ions down regulates expression of the extracellular matrix genes in B. subtilis.
Figure 2. The effect of Mg2+, Ca2+, and Na+ ions on transcription of the operons responsible for the matrix production. Transcription of the operons responsible for the matrix production is differentially regulated in response to (A) Mg2+, (B) Na+, and (C) Ca2+ ions. The left panel shows results from RL4548 cells that bear the Peps-lacZ transcriptional fusion and the right panel demonstrates results from RL4582 cells that bear the PtapA-lacZ transcriptional fusion;. *P-value < 0.05 compared to control.
Next, we visualized microscopically the effect of magnesium ions by testing bundling phenotype of fluorescently tagged B. subtilis cells (YC161 with Pspank-gfp), which produce GFP constitutively (Chai et al., 2011). As seen in Figure 3, there is significant reduction in bundling ability of B. subtilis cells in the presence of 25 mM MgCl2 and higher. This result further confirms the potential of Mg2+ ions to inhibit biofilm formation by B. subtilis.
Figure 3. Mg2+ ions block the biofilm bundles formation of B. subtilis. CLSM images of fluorescently tagged B. subtilis cells (YC161 with Pspank-gfp) following 24 h incubation in biofilm promoting medium.
Subsequently, we wondered whether other common salts may also affect the biofilm formation at the same concentration as it does MgCl2. Therefore, we tested the effect of NaCl and CaCl2 on biofilm formation by B. subtilis. Notably, none of those compounds could inhibit the biofilm formation in the same manner as MgCl2. Although, there was not a significant difference in pellicle formation or bundling ability of B. subtilis cells in the presence of NaCl at tested concentrations (Figure 4), nonetheless, NaCl could slightly affect the expression of eps operon at 100 mM concentration (Figure 2B). Interestingly, we detected a very slight inhibition in the pellicle formation in the presence of 50 mM or higher concentrations of CaCl2 (Figure 5), while expression of the eps and tapA operons was found to be somewhat downregulated (Figure 2C). It should be noted that neither NaCl nor CaCl2 affected notably bacterial growth (Supplementary Figures 2 and 3).
Figure 4. The effect of Na+ ions on biofilm formation by B. subtilis. The effect of addition of different concentrations of NaCl to LBGM medium on pellicle formation by B. subtilis NCIB3610.
Figure 5. The effect of Ca2+ ions on biofilm formation by B. subtilis. The effect of addition of different concentrations of CaCl2 to LBGM medium on pellicle formation by B. subtilis NCIB3610.
It becomes increasingly clear that most of the bacteria in their natural state exist as surface associated matrix enclosed biofilms. Bacteria are much protected from environmental insults as well as various antimicrobial treatments in the biofilm mode of growth. Our results show that Mg2+ inhibits biofilm formation by B. subtilis at 25 mM and higher concentration, although at low concentrations (5 and 10 mM) Mg2+ enhanced biofilm formation of B. subtilis. Apparently, the inhibitory effect of ions is conserved in other Bacillus species too. Using CLSM method, we observed a notable inhibition in biofilm formation by B. cereus (Supplementary Figure 5), while bacterial growth was not affected in the presence of Mg2+ ions (Supplementary Figure 4). Interestingly, the results of our study are in consistence with some of the previous findings regarding the effect of magnesium ions on bacterial adhesion and biofilm formation by different species. Previous studies have shown that Mg2+ has varying effects on bacterial adhesion (Marcus et al., 1989; Dunne and Burd, 1992; Tamura et al., 1994), which could be explained due to the difference in bacterial species and Mg2+ concentrations used in the various studies. For instance, Tamura et al. (1994) showed that 2 mM magnesium had no significant effect on adherence of Streptococci, while higher concentrations enhanced adherence to a small degree (Tamura et al., 1994). Another study showed that at 16 mM magnesium significantly enhanced the in vitro adhesion of S. epidermidis to plastic surface (Dunne and Burd, 1992). Additional study has showed that Mg2+ enhanced adherence of mucoid in one P. aeruginosa strain tested and showed no effect on the other (Marcus et al., 1989). It was further found that increase in Mg2+ concentrations positively influenced bacterial attachment but the effect changed over time during biofilm formation (Song and Leff, 2006).
It was proposed previously that high Mg2+ concentration might contribute to an increase in exopolysaccharide (EPS) production and biofilm stabilization (Costerton et al., 1995). However, it was also found that biofilm formation decreased with increasing concentration of Mg2+ in E. cloacae (Zhou et al., 2014). In our study, we showed that the expression of the two major operons responsible for biofilm matrix production were reduced notably in response to Mg2+ ions, suggesting about an inhibitory effect on expression of the matrix genes in B. subtilis. We demonstrated that Mg2+ ions are capable to profoundly inhibit biofilm formation of B. subtilis at 25 mM concentration and higher. Since the matrix gene expression is tightly controlled by Spo0A~P dependent pathway (Shemesh and Chai, 2013), it is conceivable that Mg2+ ions could affect the signal transduction for biofilm formation through this pathway. Although, it is also possible that matrix gene expression is alternatively turned on by a Spo0A~P independent pathway such as YwcC-SlrA pathway (Chai et al., 2009). It will be interesting to further investigate in future studies how the inhibitory effect of Mg2+ ions affect a certain signaling pathway involved in biofilm formation.
In our study we decided to determine whether other divalent metal ions such as Ca2+ (CaCl2) or monovalent metal ions such as Na+ (NaCl) can inhibit biofilm formation. Our results showed that Ca2+ and Na+ ions did not significantly decrease biofilm formation by B. subtilis. It is known that the Ca2+ ions have beneficial effect of on the mechanical stability of various biofilms (Rose, 2000). Moreover, it is also established that Ca2+ ions is important for bacterial biofilm formation (Geesey et al., 2000). Calcium is thought to promote thicker bacterial biofilms, primarily through ionic bridging of the extracellular matrix material (Rose and Turner, 1998; Körstgens et al., 2001). Previous studies have shown that the addition of Ca2+ caused a significant increase in S. paucimobilis biofilm formation at different concentration levels (Guvensen et al., 2012). Another study has shown that Pseudoalteromonas spp. produces larger amounts of biofilm-associated polysaccharide with increased Ca2+ (Patrauchan et al., 2005). Additional study showed that the amount of extracellular polysaccharide material of an alginate-producing of Pseudomonas aeruginosa is induced as much as eight-fold in response to Ca2+ ions (Sarkisova et al., 2005). It was also recently shown that Na+ ions could also affect auto-aggregation and biofilm formation in some foodborne pathogens (Xu et al., 2010).
In overall, results of the present study show the inhibitory effect of Mg2+ ions on B. subtilis biofilm formation as well as reduction in expression of main genes involved in biofilm formation. Findings of this study can open opportunities for development of novel strategies to control biofilm formation in various settings by using small natural molecules. Hence, different magnesium salts can be used to prevent or inhibit bacterial colonization and biofilm formation of Bacillus species in industrial and clinical settings.
The authors declare that the research was conducted in the absence of any commercial or financial relationships that could be construed as a potential conflict of interest.
Contribution from the Agricultural Research Organization, the Volcani Center, Beit Dagan, Israel, No. 720/15-E Series is acknowledged. We would like to thank Dr. Y. Chai from the Northeastern University for B. subtilis strains and helpful discussions. We thank Dr. Michel Gohar for the B. cereus strain. We also acknowledge members of the Shemesh and Steinberg laboratories for helpful discussions and some technical assistance, especially Danielle duanis-assaf and Ievgeniia Ostrov. We are also grateful to Eduard Belausov from ARO for excellent technical assistance with confocal microscopy. This work was partially supported by the COST ACTION FA1202 BacFoodNet. The authors of the paper declare no conflict of interest.
The Supplementary Material for this article can be found online at: http://journal.frontiersin.org/article/10.3389/fmicb.2015.00907
Branda, S. S., Chu, F., Kearns, D. B., Losick, R., and Kolter, R. (2006). A major protein component of the Bacillus subtilis biofilm matrix. Mol. Microbiol. 59, 1229–1238. doi: 10.1111/j.1365-2958.2005.05020.x
Branda, S. S., González-Pastor, J. E., Ben-Yehuda, S., Losick, R., and Kolter, R. (2001). Fruiting body formation by Bacillus subtilis. Proc. Natl. Acad. Sci. U.S.A. 98, 11621–11626. doi: 10.1073/pnas.191384198
Chai, Y., Chu, F., Kolter, R., and Losick, R. (2008). Bistability and biofilm formation in Bacillus subtilis. Mol. Microbiol. 67, 254–263. doi: 10.1111/j.1365-2958.2007.06040.x
Chai, Y., Kolter, R., and Losick, R. (2009). Paralogous antirepressors acting on the master regulator for biofilm formation in Bacillus subtilis. Mol. Microbiol. 74, 876–887. doi: 10.1111/j.1365-2958.2009.06900.x
Chai, Y., Norman, T., Kolter, R., and Losick, R. (2011). Evidence that metabolism and chromosome copy number control mutually exclusive cell fates in Bacillus subtilis. EMBO J. 30, 1402–1413. doi: 10.1038/emboj.2011.36
Chu, F., Kearns, D. B., Branda, S. S., Kolter, R., and Losick, R. (2006). Targets of the master regulator of biofilm formation in Bacillus subtilis. Mol. Microbiol. 59, 1216–1228. doi: 10.1111/j.1365-2958.2005.05019.x
Costerton, J. W. (1999). Introduction to biofilm. Int. J. Antimicrob. Agents 11, 217–221. discussion: 237–239. doi: 10.1016/S0924-8579(99)00018-7
Costerton, J. W., Lewandowski, Z., Caldwell, D. E., Korber, D. R., and Lappin-Scott, H. M. (1995). Microbial biofilms. Annu. Rev. Microbiol. 49, 711–745. doi: 10.1146/annurev.mi.49.100195.003431
Dunne, W. M. Jr, Burd, E. M. (1992). The effects of magnesium, calcium, EDTA, and pH on the in vitro adhesion of Staphylococcus epidermidis to plastic. Microbiol. Immunol. 36, 1019–1027. doi: 10.1111/j.1348-0421.1992.tb02106.x
Flemming, H. C., and Wingender, J. (2010). The biofilm matrix. Nat. Rev. Microbiol. 8, 623–633. doi: 10.1038/nrmicro2415
Fletcher, M. (1988). Attachment of Pseudomonas fluorescens to glass and influence of electrolytes on bacterium-substratum separation distance. J. Bacteriol. 170, 2027–2030.
Flint, S. H., Bremer, P. J., and Brooks, J. D. (1997). Biofilms in dairy manufacturing plant - description, current concerns and methods of control. Biofouling 11, 81–97. doi: 10.1080/08927019709378321
Geesey, G. G., Wigglesworth-Cooksey, B., and Cooksey, K. E. (2000). Influence of calcium and other cations on surface adhesion of bacteria and diatoms: A review. Biofouling 15, 195–205. doi: 10.1080/08927010009386310
Guvensen, N. C., Demir, S., and Ozdemir, G. (2012). Effects of magnesium and calcium cations on biofilm formation by Sphingomonas Paucimobilis from an industrial environment. Fresenius Environ. Bull. 21, 3685–3692. doi: 10.1016/j.copbio.2013.05.185
Hall-Stoodley, L., Costerton, J. W., and Stoodley, P. (2004). Bacterial biofilms: from the natural environment to infectious diseases. Nat. Rev. Microbiol. 2, 95–108. doi: 10.1038/nrmicro821
Kearns, D. B., Chu, F., Branda, S. S., Kolter, R., and Losick, R. (2005). A master regulator for biofilm formation by Bacillus subtilis. Mol. Microbiol. 55, 739–749. doi: 10.1111/j.1365-2958.2004.04440.x
Kolter, R., and Greenberg, E. P. (2006). Microbial sciences: the superficial life of microbes. Nature 441, 300–302. doi: 10.1038/441300a
Körstgens, V., Flemming, H. C., Wingender, J., and Borchard, W. (2001). Influence of calcium ions on the mechanical properties of a model biofilm of mucoid Pseudomonas aeruginosa. Water Sci. Technol. 43, 49–57.
Mah, T. F., and O'Toole, G. A. (2001). Mechanisms of biofilm resistance to antimicrobial agents. Trends Microbiol. 9, 34–39. doi: 10.1016/S0966-842X(00)01913-2
Malik, A., and Kakii, K. (2003). Intergeneric coaggregations among Oligotropha carboxidovorans and Acinetobacter species present in activated sludge. FEMS Microbiol. Lett. 224, 23–28. doi: 10.1016/S0378-1097(03)00391-4
Marcus, H., Austria, A., and Baker, N. R. (1989). Adherence of Pseudomonas aeruginosa to tracheal epithelium. Infect. Immun. 57, 1050–1053.
Merino, S., Gavín, R., Altarriba, M., Izquierdo, L., Maguire, M. E., and Tomás, J. M. (2001). The MgtE Mg2+ transport protein is involved in Aeromonas hydrophila adherence. FEMS Microbiol. Lett. 198, 189–195. doi: 10.1111/j.1574-6968.2001.tb10641.x
Patrauchan, M. A., Sarkisova, S., Sauer, K., and Franklin, M. J. (2005). Calcium influences cellular and extracellular product formation during biofilm-associated growth of a marine Pseudoalteromonas sp. Microbiology 151(Pt 9), 2885–2897. doi: 10.1099/mic.0.28041-0
Romero, D., Aguilar, C., Losick, R., and Kolter, R. (2010). Amyloid fibers provide structural integrity to Bacillus subtilis biofilms. Proc. Natl. Acad. Sci. U.S.A. 107, 2230–2234. doi: 10.1073/pnas.0910560107
Rose, R. K. (2000). The role of calcium in oral streptococcal aggregation and the implications for biofilm formation and retention. Biochim. Biophys. Acta 1475, 76–82. doi: 10.1016/S0304-4165(00)00048-9
Rose, R. K., and Turner, S. J. (1998). Extracellular volume in streptococcal model biofilms: effects of pH, calcium and fluoride. Biochim. Biophys. Acta 1379, 185–190. doi: 10.1016/S0304-4165(97)00098-6
Sarkisova, S., Patrauchan, M. A., Berglund, D., Nivens, D. E., and Franklin, M. J. (2005). Calcium-induced virulence factors associated with the extracellular matrix of mucoid Pseudomonas aeruginosa biofilms. J. Bacteriol. 187, 4327–4337. doi: 10.1128/JB.187.13.4327-4337.2005
Sharma, M., and Anand, S. K. (2002). Biofilms evaluation as an essential component of HACCP for food/dairy industry - a case. Food Control 13, 469–477. doi: 10.1016/S0956-7135(01)00068-8
Shemesh, M., and Chai, Y. (2013). A combination of glycerol and manganese promotes biofilm formation in Bacillus subtilis via histidine kinase KinD signaling. J. Bacteriol. 195, 2747–2754. doi: 10.1128/JB.00028-13
Shemesh, M., Kolter, R., and Losick, R. (2010). The biocide chlorine dioxide stimulates biofilm formation in Bacillus subtilis by activation of the histidine kinase KinC. J. Bacteriol. 192, 6352–6356. doi: 10.1128/JB.01025-10
Shemesh, M., Tam, A., and Steinberg, D. (2007). Expression of biofilm-associated genes of Streptococcus mutans in response to glucose and sucrose. J Med Microbiol. 56(Pt 11), 1528–1535. doi: 10.1099/jmm.0.47146-0
Simoes, M., Simoes, L. C., and Vieira, M. J. (2010). A review of current and emerging control strategies. LWT Food Sci. Technol. 43, 573–583. doi: 10.1016/j.lwt.2009.12.008
Simoni, S. F., Bosma, T. N. P., Harms, H., and Zehnder, A. J. B. (2000). Bivalent cations increase both the subpopulation of adhering bacteria and their adhesion efficiency in sand columns. Environ. Sci. Technol. 34, 1011–1017. doi: 10.1021/es990476m
Somerton, B., Flint, S., Palmer, J., Brooks, J., and Lindsay, D. (2013). Preconditioning with cations increases the attachment of Anoxybacillus flavithermus and Geobacillus species to stainless steel. Appl. Environ. Microbiol. 79, 4186–4190. doi: 10.1128/AEM.00462-13
Song, B., and Leff, L. G. (2006). Influence of magnesium ions on biofilm formation by Pseudomonas fluorescens. Microbiol. Res. 161, 355–361. doi: 10.1016/j.micres.2006.01.004
Stewart, P. S., and Costerton, J. W. (2001). Antibiotic resistance of bacteria in biofilms. Lancet 358, 135–138. doi: 10.1016/S0140-6736(01)05321-1
Tamura, G. S., Kuypers, J. M., Smith, S., Raff, H., and Rubens, C. E. (1994). Adherence of group B streptococci to cultured epithelial cells: roles of environmental factors and bacterial surface components. Infect. Immun. 62, 2450–2458.
Wirtanen, G., Husmark, U., and Mattila-Sandholm, T. (1996). Microbial evaluation of the biotransfer potential from surfaces with Bacillus biofilms after rinsing and cleaning procedures in closed food-processing systems. J. Food Prot. 59, 727–733.
Xu, H., Zou, Y., Lee, H. Y., and Ahn, J. (2010). Effect of NaCl on the biofilm formation by foodborne pathogens. J. Food Sci. 75, M580–M585. doi: 10.1111/j.1750-3841.2010.01865.x
Keywords: biofilm formation, magnesium ions, food industry, Bacillus species, microbial development
Citation: Oknin H, Steinberg D and Shemesh M (2015) Magnesium ions mitigate biofilm formation of Bacillus species via downregulation of matrix genes expression. Front. Microbiol. 6:907. doi: 10.3389/fmicb.2015.00907
Received: 21 May 2015; Accepted: 19 August 2015;
Published: 08 September 2015.
Edited by:
Beiyan Nan, Taxas A&M University, USAReviewed by:
Anushree Malik, Indian Institute of Technology Delhi, IndiaCopyright © 2015 Oknin, Steinberg and Shemesh. This is an open-access article distributed under the terms of the Creative Commons Attribution License (CC BY). The use, distribution or reproduction in other forums is permitted, provided the original author(s) or licensor are credited and that the original publication in this journal is cited, in accordance with accepted academic practice. No use, distribution or reproduction is permitted which does not comply with these terms.
*Correspondence: Moshe Shemesh, Department of Food Quality and Safety, Institute for Postharvest Technology and Food Sciences, Agricultural Research Organization, The Volcani Center, 50250 Bet-Dagan, Israel,bW9zaGVzaEBhZ3JpLmdvdi5pbA==
Disclaimer: All claims expressed in this article are solely those of the authors and do not necessarily represent those of their affiliated organizations, or those of the publisher, the editors and the reviewers. Any product that may be evaluated in this article or claim that may be made by its manufacturer is not guaranteed or endorsed by the publisher.
Research integrity at Frontiers
Learn more about the work of our research integrity team to safeguard the quality of each article we publish.