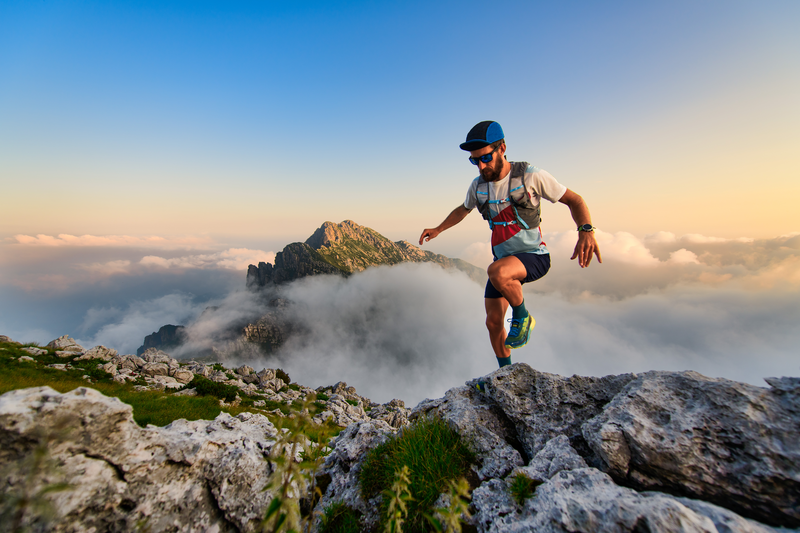
94% of researchers rate our articles as excellent or good
Learn more about the work of our research integrity team to safeguard the quality of each article we publish.
Find out more
FOCUSED REVIEW article
Front. Microbiol. , 20 August 2014
Volume 5 - 2014 | https://doi.org/10.3389/fmicb.2014.00434
This article mentions parts of:
Green tea as an effective antimicrobial for urinary tract infections caused by Escherichia coli
Green tea is a popular drink, especially in Asian countries, although its popularity continues to spread across the globe. The health benefits of green tea, derived from the leaves of the Camellia sinensis plant, have been studied for many years. Fairly recently, researchers have begun to look at the possibility of using green tea in antimicrobial therapy, and the potential prevention of infections. The particular properties of catechins found in the tea have shown promise for having antimicrobial effects. There are four main catechins (polyphenols) found in green tea: (-)-epicatechin (EC), (-)-epicatechin-3-gallate (ECG), (-)-epigallocatechin (EGC), and (-)-epigallocatechin-3-gallate (EGCG). Three of these, ECG, EGC, and EGCG have been shown to have antimicrobial effects against a variety of organisms. These catechins have exhibited a variety of antimicrobial mechanisms. The results of studies on the antimicrobial effects of green tea have shown that the potential for preventive and therapeutic purposes is present. Further data collection on studies performed with human consumption during the course of infections, and studies on the occurrence of infections in populations that consume regular amounts of green tea will be necessary to complete the picture of its antimicrobial possibilities.
Tea is a very popular drink world-wide. It is produced from the plant Camellia sinensis, which is grown in at least 30 countries, and grows best in certain tropical and subtropical regions (Gupta et al., 2014). Tea is mainly produced as four varieties; white, green, Oolong, and black. White tea is made from very young tea leaves or buds; green tea is made from mature unfermented leaves; Oolong tea from partially fermented leaves; and black tea from fully fermented leaves (Jigisha et al., 2012; Gupta et al., 2014). Most of the black tea produced is consumed in the United States, Oolong tea is most popular in China and Taiwan, and green tea is most popular in China, Japan, and Korea (Cabrera et al., 2006). Among the health benefits that have been studied using green tea are: as an antioxidant, anti-inflammatory, anticarcinogenic, in cardiovascular health, oral health, and as an antimicrobial. Antioxidant effects come from the ability of green tea to limit the amount of free radicals by binding to reactive oxygen species (ROS) (Serafini et al., 2011; Jigisha et al., 2012; Gupta et al., 2014). Anti-inflammatory effects may be a result of increased production of IL-10, an anti-inflammatory cytokine. Inflammation is involved in, among other conditions, arthritis, cardiovascular disease, aging, and cancer (Serafini et al., 2011; Jigisha et al., 2012). The anticarcinogenic effects of green tea have been seen in many types of cancer, and the mechanisms may include inhibiting angiogenesis and cell growth, and inducing apoptosis in cancer cells (Serafini et al., 2011; Jigisha et al., 2012; Subramani and Natesh, 2013). Cardiovascular effects include the antioxidant and anti-inflammatory effects, and consumption of green tea has been shown to inhibit atherosclerosis, reduce lipid levels overall, and improve the ratio of LDL to HDL (Serafini et al., 2011; Jigisha et al., 2012). The effects for oral health are related to both teeth and gums. The main cause of dental caries is the bacteria Streptococcus mutans. Green tea has a direct antimicrobial effect on this bacteria, plus it seems to inhibit the attachment of the bacteria to oral surfaces. In addition, green tea is a natural source of fluoride (Jigisha et al., 2012; Gupta et al., 2014). Green tea has been shown to have antimicrobial effects against a variety of gram positive and gram negative bacteria (e.g., Escherichia coli, Salmonella spp., Staphylococcus aureus, Enterococcus spp.), some fungi (e.g., Candida albicans), and a variety of viruses (e.g., HIV, herpes simplex, influenza) (Jigisha et al., 2012; Steinmann et al., 2013). These antimicrobial effects will be discussed in more detail later in this paper.
KEY CONCEPT 1. Antimicrobial
Antibacterial agents attack microorganisms through four main mechanisms: inhibiting cell wall synthesis (β-lactams drugs, such as the penicillins and cephalosporins), inhibiting protein synthesis (aminoglycosides, tetracyclines, etc.), inhibiting nucleic acid synthesis (fluoroquinolones), or inhibiting metabolic pathways (trimethoprim, sulfonamides). Antiviral agents may target host cell entry, interfere with various stages of viral replication or assembly, or interfere with virus release from the host cell. Since fungi are eukaryotes, antifungal agents have to key in on differences between human and fungal cell processes. Because the parasites are a widely diverse group of organisms, antiparasitic agents are quite often specific for a certain parasite or related parasite group (e.g., nematodes).
The medically important components of green tea are the polyphenols, most importantly the flavonoids. The main flavonoids in tea are the catechins, making up 30–40% of the water-soluble solids in green tea (Wang and Ho, 2009; Roowi et al., 2010). The different types of tea vary in the amount of catechins that they contain, with green tea containing the most, then Oolong tea, then black tea. The initial steaming process in the production of green tea destroys the enzyme polyphenol oxidase, thus protecting the polyphenol content. There are four main catechins in tea. (-)-epicatechin (EC), (-)-epicatechin-3-gallate (ECG), (-)-epigallocatechin (EGC), and (-)-epigallocatechin-3-gallate (EGCG). In green tea, EGCG is the most abundant, representing approximately 59% of the total catechins. EGC is next, making up approximately 19%. Then ECG, at 13.6%; and EC, at 6.4% (Cabrera et al., 2006; Jigisha et al., 2012). In addition to the type of tea, the amount of catechins can be affected by: where on the plant the leaves are harvested, leaf processing, geographical location, growing conditions, and tea preparation (Fernandez et al., 2002; Lin et al., 2003; Cabrera et al., 2006).
In order for any of the components of green tea to be a health benefit they have to have bioavailability This is most commonly assessed by measuring plasma, urine, and perhaps (in directed studies) tissue levels of the components after ingestion; usually by taking samples at regularly timed intervals. Various studies conducted using consumption of green tea beverage (Henning et al., 2004; Stalmach et al., 2009; Clifford et al., 2013), green tea extract (Lee et al., 1995; Yang et al., 1998; Henning et al., 2004), or specific catechins (Chow et al., 2001; Van Amelsvoort et al., 2001), have shown that ECG, EGCG, and metabolites of EC and EGC can be detected in blood plasma; and metabolites of EC and EGC only can be detected in urine. The peak concentration in blood plasma occurs at approximately 2 h after ingestion; and the peak concentration in urine occurs approximately 4–6 h after consumption. Some studies were performed using various dosages of the catechins, and show that the bioavailability of the catechins is directly proportional to the amount consumed (Nakagawa et al., 1997; Yang et al., 1998; Chow et al., 2001, 2003; Ullmann et al., 2003). Table 1 shows a summary of the results of these various bioavailability studies, giving data specifically for EGCG and EGC. The most abundant catechins in green tea, EGCG and EGC, also occur in the greatest concentration in the body after consumption (EGCG in plasma, and EGC in both plasma and urine).
KEY CONCEPT 2. Bioavailability
Bioavailability is a measure of how much of a substance can be found in blood, tissues, urine, etc., after being introduced into the body. Enough of the substance must be present at the site of an infection in order to be useful in fighting the microorganism(s) involved.
In a previous study (Reygaert and Jusufi, 2013), it was projected that using a brewed preparation of Japanese green tea (containing approximately 20 mg of EGC per gram of tea) would result in approximately 150 mg of ECG per cup of tea. The amount of EGC excreted in the urine would be approximately 3.5 mg per cup of tea.
In addition to the direct antimicrobial effects of catechins discussed below (damage to the bacterial cell membrane, inhibition of fatty acid synthesis, inhibition of enzyme activity, etc.), there are also some effects that may contribute to the total antimicrobial effect in infected individuals. These effects include inhibition of inflammation (particularly inflammation caused by oxidative stress, such as vascular), more specifically, by increasing the synthesis of nitric oxide (Yamakuchi et al., 2008), inhibiting angiotensin II and IL-6-induced C-reactive protein expression (Li et al., 2012), suppression of IL-6 and RANKL production in infected osteoblast-like cells (Ishida et al., 2007), inhibiting IL-8 production (Hirao et al., 2010), and inhibiting hyaluronidase activity (activated by chronic inflammation) by inhibition of IL-12 (Adcocks et al., 2002).
Many of the direct effects of tea catechins are a result of the catechins binding to the bacterial lipid bilayer cell membrane which then causes damage to the membrane (Sirk et al., 2008, 2009). This damage can then lead to a variety of related antimicrobial effects. Researchers studying Escherichia coli found that when exposed to green tea polyphenols, the bacterial response was changed regulation of 17 individual genes. Nine genes were upregulated and eight were downregulated. One of the major outcomes of this change in regulation was damage to the bacterial cell membrane (Cho et al., 2007). Tea catechins have less effect on gram negative bacterial cell membranes due to the fact that the LPS outer membrane of gram negative bacteria is negatively charged (Ikigai et al., 1993). Bacterial cell membrane damage inhibits the ability of the bacteria to bind to host cells (Sharma et al., 2012), and inhibits the ability of the bacteria to bind to each other to form biofilms, which are significant in pathogenesis (Blanco et al., 2005). Bacterial membrane damage also results in an inability of the bacteria to be able to secrete toxins (Sugita-Konishi et al., 1999; Shah et al., 2008). Directly related to the homeostasis of the bacterial cell membrane is fatty acid synthesis.
Fatty acids in bacteria have important functions; as a component of phospholipid cell membranes (and mycolic acid in cell walls of mycobacteria), and as an excellent energy source. Researchers have recently begun to look at the potential of targeting fatty acid biosynthesis for antimicrobial drug development (Wang and Ma, 2013). Researchers have found that green tea components (especially EGCG) inhibit specific reductases (FabG, FabI) in bacterial type II fatty acid synthesis (Zhang and Rock, 2004; Li et al., 2006). Inhibition of fatty acid synthesis by green tea has also been found to inhibit bacterial production of toxic metabolites (Sakanaka and Okada, 2004).
In addition to the enzymes involved in fatty acid synthesis, research with green tea has been shown to have effects on other essential bacterial enzymes. Researchers found that green tea catechins have an inhibitory effect on protein tyrosine phosphatase and cysteine proteinases in certain anaerobic oral bacteria (Okamoto et al., 2003, 2004). Researchers have also found that green tea catechins have the ability to interfere with DNA replication by interacting with, and thereby inhibiting the function of DNA gyrase (Grandišar et al., 2007). Studies on antifolate activity in microorganisms have shown that green tea polyphenols can inhibit the enzyme dihydrofolate reductase in bacteria and yeast, effectively blocking the ability of the microorganisms to synthesize folate (Navarro-Martinez et al., 2005, 2006). More recently is has been discovered that bioflavonoids (including those from green tea) could inhibit the activity of bacterial ATP synthase, reducing the ability of the microorganisms to produce enough energy (Chinnam et al., 2010).
Researchers have found that green tea catechins have inhibitory effects on many other bacterial functions. One such effect is inhibiting synthesis of PBP2′ in methicillin-resistant Staphylococcus aureus (MRSA) This inhibition leads to reversal of resistance to β-lactam drugs (Yam et al., 1998). Another effect on bacteria is the inhibition by green tea catechins on the conjugative transfer of the R plasmid in Escherichia coli (Zhao et al., 2001a). This could lead to decreased sharing of antimicrobial genes between bacteria. Research on staphylococci has shown the ability of green tea catechins to inhibit the activity of efflux pumps. In this particular study the efflux pump was Tet(K) which is involved in resistance to tetracycline (Sudano Roccaro et al., 2004). This same type of activity could very well-occur with other efflux pumps, which could enhance the ability of certain drugs to have an antimicrobial effect. A study performed with Helicobacter pylori found that green tea catechins blocked the ability of the bacteria to bind to the toll-like receptor-4 (TLR-4) on gastric epithelial cells (Lee et al., 2004). This would lead to a drastic reduction in that organism's ability to cause gastric disease. There are also inhibitory effects found with viruses. Research with HIV-1 has shown that green tea EGCG binds to the CD4 T-cell receptor, blocking the binding of the virus. This ability to block viral cell binding has been proposed for use in therapy for HIV-1 infection (Williamson et al., 2006).
KEY CONCEPT 3. Resistant
Microorganisms have a variety of methods that they use to overcome antimicrobial agents (antimicrobial-resistance). There are four main mechanisms that are used: inhibiting uptake of a drug, increased excretion of a drug (e.g., efflux pumps), structural or functional modification of the microorganism drug target, or inactivating the drug directly. This antimicrobial-resistance may be acquired from another similar organism, or a result of a mutation in one or more of the microorganism's own genes.
KEY CONCEPT 4. MRSA
Methicillin-resistant Staphylococcus aureus (MRSA) have acquired a gene that allows production of an altered penicillin-binding protein (PBP), PBP2′. PBPs are transpeptidases that are used in the synthesis of the peptidoglycan layer of the bacterial cell wall. PBP2′ has a greatly reduced affinity for most of the β-lactam drugs, making these drugs ineffective against MRSA. This makes the treatment of infections with MRSA much more difficult and costly.
Researchers have taken the concept of green tea catechins as having antimicrobial effects and expanded on this by performing studies that would determine if the green tea catechins might work synergistically with known antimicrobial agents. Many of these studies have focused on Staphylococcus aureus (particularly MRSA), Staphylococcus epidermidis, and Escherichia coli, among others. Examples of those studies have found that green tea catechins work synergistically with tetracycline against S. aureus and S. epidermidis (Sudano Roccaro et al., 2004; Fanaki et al., 2008); with penicillin against S. epidermidis (Haghjoo et al., 2013); with penicillin, oxacillin, ampicillin/sulbactam, and imipenem against MRSA (Hu et al., 2001, 2002; Zhao et al., 2001b; Stapleton et al., 2006; Aboulmagd et al., 2011); and with chloramphenicol, ciprofloxacin, and cefotaxime against isolates of E. coli having various levels of resistance, including extended-spectrum beta-lactamase (ESBL) producers (Fanaki et al., 2008; Cui et al., 2012a; Passat, 2012).
KEY CONCEPT 5. ESBL
Extended-spectrum β-lactamase (ESBL) producing organisms are most often members of the gram negative Enterobacteriaceae family. Beta-lactamases are enzymes produced by the organism that are able to hydrolyze β-lactam drugs, rendering these drugs inactive. Like MRSA, the ESBLs have an increased resistance to penicillins and cephalosporins, but are sensitive to carbapenems and β-lactamase inhibitors (drugs that inhibit the activity of β-lactamases and are used together with a β-lactam drug, such as ampicillin/sulbactam). Many ESBL producers are also multidrug-resistant organisms (MRSOs), making treatment of these infections very difficult.
A large variety of research has been performed assessing whether or not green tea has antimicrobial activities. A summary of examples of this research is included in Table 2. The research results shown do suggest that green tea may be effective against many organisms. The research is pretty well-balanced between gram positive and gram negative organisms, with a few viruses, fungi and a parasite also represented. The main gram positive organism represented is S. aureus. That is not surprising considering the ongoing menace of MRSA. The most commonly studied gram negative organism is E. coli. These results represent potentially useful information for battling the emerging threat of ESBL and Carbapenem-resistant Enterobacteriaceae (CRE) organisms. In a previous study, green tea extract was shown to be effective against E. coli strains isolated from UTIs. Many of these isolates (59/80) were multi-drug resistant (MDROs), and six of the strains were ESBLs (Reygaert and Jusufi, 2013). The results shown in Table 2, along with the possible synergistic activities mentioned earlier, suggest that green tea could be extremely useful as an antimicrobial agent. There are certain things to keep in mind when viewing the results in Table 2. First, is the type of green tea and the preparation used. The research may have used green tea from various locations around the globe. For the experiments, the preparation may have included freshly brewed green tea, green tea extracts (water, alcohol), or purified extracts of certain of the green tea catechins. There are also various other factors that may affect the composition of the tea (refer to the section on green tea composition). The second thing to pay attention to is the type of result reported. There are four basic types of results shown: growth in colony-forming units (CFUs), or plaque-forming units (PFUs) for viruses; minimal inhibitory concentration (MIC); the size of zones of inhibition; and the optical density (O.D.) of growth in liquid cultures. The percentage of increase or decrease of any of these parameters may also be shown. In addition, for viruses, other growth or detection measures made be shown. Overall, the results indicate that green tea has great potential as an antimicrobial agent.
KEY CONCEPT 6. CRE
Carbapenem-resistant Enterobacteriaceae (CREs) are similar to ESBLs. These organisms are resistant to all β-lactam drugs, and are not inactivated by β-lactamase inhibitors. The most common ESBL-producing organisms are Escherichia coli and Klebsiella pneumoniae. Treatment options are extremely limited for infections involving these organisms.
The data discussed shows that green tea catechins EGCG and EGC are bioavailable in plasma (both) and urine (EGC only) at potentially therapeutic levels and in a timely manner (see Table 1). Antimicrobial studies using various green tea extracts have shown that there is a huge potential for the use of green tea in antimicrobial therapy (see Table 2). In a previous study, the amount of EGC from brewed Japanese green tea excreted in the urine was calculated to be approximately 3.5 mg per cup of tea. Since the results of the study showed that most of the bacterial strains tested were susceptible to EGC levels of ≤0.72 mg, one cup of tea should be able to control growth of the bacteria for up to 6 h or even longer (Reygaert and Jusufi, 2013).
While the research results that have been discussed suggest that green tea may be effective against many organisms, there are certain issues that need to be addressed concerning these results. The first issue is how the results are displayed. The difficulty of assessing these types of results, and how these results relate to each other among the different research groups, can be confusing. This brings us to the second major issue. Various agencies, such as the Clinical and Laboratories Standards Institute (CLSI) in the United States, have strict protocols for the determination of antimicrobial susceptibility. Most of the research results that have been performed using green tea have not necessarily followed any of these types of guidelines. Therefore, the results of these experiments may differ from what the results would have been if strict protocols had been used. In the future, it will be most helpful if researchers would take the time to use these protocols. That will make comparing the results, and assessing if the results are meaningful so much easier. In addition (and most importantly), in order for the results to be able to be translated into clinical use, the protocols will need to be followed. That having been addressed, it is still very encouraging to look at the research performed and see that green tea could very possibly be an effective antimicrobial agent, especially against multidrug-resistant strains, and in particular, MRSA and ESBL producing organisms. Hopefully, in the future, researchers will be able to study the effects of green tea on infections in humans. This type of research is a critical part of determining the antimicrobial capabilities of green tea. It might possibly be incorporated into research with other antimicrobial compounds. Perhaps naturopathic practitioners could begin to collect data on patients using green tea. With emerging multidrug-resistant organisms and the lack of effective new antimicrobial drugs being produced, we cannot afford to ignore the potential of green tea.
The author declares that the research was conducted in the absence of any commercial or financial relationships that could be construed as a potential conflict of interest.
Aboulmagd, E., Al-Mohammed, H. I., and Al-Badry, S. (2011). Synergism and postantibiotic effect of green tea extract and imipenem against methicillin-resistant Staphylococcus aureus. Microbiol. J. 1, 89–96. doi: 10.3923/mj.2011.89.96
Adcocks, C., Collin, P., and Buttle, D. J. (2002). Catechins from green tea (Camellia sinensis) inhibitbovine and human cartilage proteoglycan and type II collagen degradation in vitro. J. Nutr. 132, 341–346.
Aman, S., Naim, A., Siddiqi, R., and Naz, S. (2013). Antimicrobial polyphenols from small tropicalfruits, tea and spice oilseeds. Food Sci. Technol. Int. 20, 241–251. doi: 10.1177/1082013213482476
Araghizadeh, A., Kohanteb, J., and Fani, M. M. (2013). Inhibitory activity of green tea (Camellia sinensis) extract on some clinically isolated cariogenic and periodontopathic bacteria. Med. Princ. Pract. 22, 368–372. doi: 10.1159/000348299
Archana, S., and Abraham, J. (2011). Comparative analysis of antimicrobial activity of leaf extracts from fresh green tea, commercial green tea and black tea on pathogens. J. Appl. Pharm. Sci. 1, 149–152.
Bandyopadhyay, D., Chatterjee, T. K., Dasgupta, A., Lourduraja, J., and Dastidar, S. G. (2005). In vitro and in vivo antimicrobial action of tea: the commonest beverage of Asia. Biol. Pharm. Bull. 28, 2125–2127. doi: 10.1248/bpb.28.2125
Blanco, A. R., Sudano-Roccaro, A., Spoto, G. C., Nostro, A., and Rusciano, D. (2005). Epigallocatechin gallate inhibits biofilm formation by ocular staphylococcal isolates. Antimicrob. Agents Chemother. 49, 4339–4343. doi: 10.1128/AAC.49.10.4339-4343.2005
Cabrera, C., Artacho, R., and Gimenez, R. (2006). Beneficial effects of green tea – a review. J. Am. Coll. Nutr. 25, 79–99. doi: 10.1080/07315724.2006.10719518
Chinnam, N., Dadi, P. K., Sabri, S. A., Ahmad, M., Kabir, M. A., and Ahmad, Z. (2010). Dietary bioflavonoids inhibit Escherichia coli ATP synthesis in a differential manner. Int. J. Biol. Macromol. 46, 478–486. doi: 10.1016/j.ijbiomac.2010.03.009
Cho, Y. S., Schiller, N. L., Kahng, H. Y., and Oh, K. H. (2007). Cellular responses and proteomic analysis of Escherichia coli exposed to green tea polyphenols. Curr. Microbiol. 55, 501–506. doi: 10.1007/s00284-007-9021-8
Cho, Y. S., Schiller, N. L., and Oh, K. H. (2008). Antibacterial effects of green tea polyphenols on clinical isolates of methicillin-resistant Staphylococcus aureus. Curr. Microbiol. 57, 542–546. doi: 10.1007/s00284-008-9239-0
Chow, H. H., Cai, Y., Alberts, D. S., Hakim, I., Dorr, R., Shahi, F., et al. (2001). Phase I pharmacokinetic study of tea polyphenols following single-dose administration of epigallocatechin gallate and polyphenon E. Cancer Epidemiol. Biomarkers Prev. 10, 53–58.
Chow, H. H., Cai, Y., Hakim, I. A., Crowell, J. A., Shahi, F., Brooks, C. A., et al. (2003). Pharmacokinetics and safety of green tea polyphenols after multiple- dose administration of epigallocatechin gallate and polyphenon E in healthy individuals. Clin. Cancer Res. 9, 3312–3319.
Clifford, M. N., van der Hooft, J. J., and Crozier, A. (2013). Human studies on the absorption, distribution, metabolism, and excretion of tea polyphenols. Am. J. Clin. Nutr. 98, 1619S–1630S. doi: 10.3945/ajcn.113.058958
Cui, Y., Kim, S. H., Kim, H., Yeom, J., Ko, K., Park, W., et al. (2012a). AFM probing the mechanism of synergistic effects of the green tea polyphenol (-)-epigallocatechin-3-gallate (EGCG) with cefotaxime against extended-spectrum beta-lactamase (ESBL)-producing Escherichia coli. PLoS ONE 7:e48880. doi: 10.1371/journal.pone.0048880
Cui, Y., Oh, Y. J., Youn, M., Lee, I., Pak, H. K., Park, W., et al. (2012b). AFM study of the differential inhibitory effects of the green tea polyphenol (-)-epigallocatechin-3-gallate (EGCG) against gram-positive and gram-negative bacteria. Food Microbiol. 29, 80–87. doi: 10.1016/j.fm.2011.08.019
de Oliveira, A., Adams, A. D., Lee, L. H., Murray, S. R., Hsu, S. D., Hammond, J. R., et al. (2013). Inhibition of herpes simplex virus type 1 with the modified green tea polyphenol palmitoyl-epigallocatechin gallate. Food Chem. Toxicol. 52, 207–215. doi: 10.1016/j.fct.2012.11.006
Fanaki, N. H., Kassem, M. A., Fawzi, M. A., and Dabbous, F. (2008). Influence of aqueous green tea extract on the antimicrobial activity of some antibiotics against multiresistant clinical isolates. Egypt. J. Med. Microbiol. 17, 449–460.
Fassina, G., Buffa, A., Benelli, R., Varnier, O. E., Noonan, D. M., and Albini, A. (2002). Polyphenolic antioxidant (-)-epigallocatechin-3-gallate from green tea as a candidate anti-HIV agent. AIDS 16, 939–941. doi: 10.1097/00002030-200204120-00020
Fernandez, P. L., Pablos, F., Martin, M. J., and Gonzalez, A. G. (2002). Study of catechin and xanthine tea profiles as geographical tracers. J. Agric. Food Chem. 59, 1833–1839. doi: 10.1021/jf0114435
Fukazawa, H., Suzuki, T., Wakita, T., and Murakami, Y. (2012). A cell-based microplate colorimetric screen identifies 7,8-benzoflavone and green tea gallate catechins a inhibitors of the hepatitis C virus. Biol. Pharm. Bull. 35, 1320–1327. doi: 10.1248/bpb.b12-00251
Gordon, N. C., and Wareham, D. W. (2010). Antimicrobial activity of the green tea polyphenol (-)-epigallocatechin-3-gallate (EGCG) against clinical isolates of Stenotrophomonas maltophilia. Int. J. Antimicrob. Agents 36, 129–131. doi: 10.1016/j.ijantimicag.2010.03.025
Grandišar, H., Pristovšek, P., Plaper, A., and Jerala, R. (2007). Green tea inhibit bacterial DNA gyrase by interaction with its ATP binding site. J. Med. Chem. 50, 264–271. doi: 10.1021/jm060817o
Gupta, D. A., Bhaskar, D. J., Gupta, R. K., Karim, B., Jain, A., and Dalai, D. R. (2014). Green tea: a review on its natural anti-oxidant therapy and cariostatic benefits. Biol. Sci. Pharm. Res. 2, 8–12. Available online at: http://journalissues.org/abstract/gupta-et-al/
Haghjoo, B., Lee, L. H., Habiba, U., Tahir, H., Olabi, M., and Chu, T. C. (2013). The synergistic effects of green tea polyphenols and antibiotics against potential pathogens. Adv. Biosci. Biotechnol. 4, 959–967. doi: 10.4236/abb.2013.411127
Henning, S. M., Niu, Y., Lee, N. H., Thames, G. D., Minutti, R. R., Wang, H., et al. (2004). Bioavailability and antioxidant activity of tea flavanols after consumption of green tea, black tea, or a green tea extract supplement. Am. J. Clin. Nutr. 80, 1558–1564.
Hirao, K., Yumoto, H., Nakanishi, T., Mukai, K., Takahashi, K., Takegawa, D., et al. (2010). Tea catechins reduce inflammatory reactions via mitogen-activated protein kinase pathways in toll-like receptor 2 ligand-stimulated dental pulp cells. Life Sci. 86, 654–660. doi: 10.1016/j.lfs.2010.02.017
Hirasawa, M., and Takada, K. (2004). Multiple effects of green tea catechin on the antifungal activity of antimycotics against Candida albicans. J. Antimicrob. Chemother. 53, 225–229. doi: 10.1093/jac/dkh046
Hirasawa, M., Takada, K., Makimura, M., and Otake, S. (2002). Improvement of periodontal status by green tea catechin using a local delivery system: a clinical pilot study. J. Periodontal Res. 37, 433–438. doi: 10.1034/j.1600-0765.2002.01640.x
Hu, Z. Q., Zhao, W. H., Asano, N., Yoda, Y., Hara, Y., and Shimamura, T. (2002). Epigallocatechin gallate synergistically enhances the activity of carbapenems against methicillin-resistant Staphylococcus aureus. Antimicrob. Agents Chemother. 46, 558–560. doi: 10.1128/AAC.46.2.558-560.2002
Hu, Z. Q., Zhao, W. H., Hara, Y., and Shimamura, T. (2001). Epigallocatechin gallate synergy with ampicillin/sulbactam against 28 clinical isolates of methicillin-resistant Staphylococcus aureus. J. Antimicrob. Chemother. 48, 361–364. doi: 10.1093/jac/48.3.361
Ikigai, H., Nakae, T., Hara, Y., and Shimamura, T. (1993). Bactericidal catechins damage the lipid bilayer. Biochim. Biophys. Acta 1147, 132–136. doi: 10.1016/0005-2736(93)90323-R
Ishida, I., Kohda, C., Yanagawa, Y., Miyaoka, H., and Shimamura, T. (2007). Epigallocatechin gallate suppresses expression of receptor activator of NF-kappaB ligand (RANKL) in Staphylococcus aureus infection in osteoblast-like NRG cells. J. Med. Microbiol. 56, 1042–1046. doi: 10.1099/jmm.0.47029-0
Jigisha, A., Nishant, R., Navin, K., and Pankaj, G. (2012). Green tea: a magical herb with miraculous outcomes. Int. Res. J. Pharm. 3, 139–148. Available online at: http://www.irjponline.com/admin/php/uploads/1083_pdf.pdf
Kim, S. H., Lee, L. S., Bae, S. M., Han, S. J., Lee, B. R., and Ahn, W. S. (2008). Antimicrobial and antifungal effects of a green tea extract against vaginal pathogens. J. Womens Med. 1, 27–36. Available online at: http://210.101.116.28/W_files/kiss8/40323392_pv.pdf
Kohda, C., Yanagawa, Y., and Shimamura, T. (2008). Epigallocatechin gallate inhibits intracellular survival of Listeria monocytogenes in macrophages. Biochem. Biophys. Res. Commun. 365, 310–315. doi: 10.1016/j.bbrc.2007.10.190
Kristanti, R. A., and Punbusayakul, N. (2009). Inhibitory effect of commercial Assam green tea infusion in watermelon juice. Asian J. Food Agroind. 2, S249–S255.
Kumar, A., Kumar, A., Thakur, P., Patil, S., Payal, C., Kumar, A., et al. (2012). Antibacterial activity of green tea (Camellia sinensis) extracts against various bacteria isolated from environmental sources. Recent Res. Sci. Technol. 4, 19–23. Available online at: http://recent-science.com/index.php/rrst/article/view/10583/5370
Lee, K. M., Yeo, M., Choue, J. S., Jin, J. H., Park, S. J., Cheong, J. Y., et al. (2004). Protective mechanism of epigallocatechin-3-gallate against Helicobacter pylori-induced gastric epithelial cytotoxicity via the blockage of TLR-4 signaling. Helicobacter 9, 632–642. doi: 10.1111/j.1083-4389.2004.00281.x
Lee, M. J., Wang, Z. Y., Li, H., Chen, L., Sun, Y., Gobbo, S., et al. (1995). Analysis of plasma and urinary tea polyphenols in human subjects. Cancer Epidemiol. Biomarkers Prev. 4, 393–399.
Lee, S. Y., Gwon, S. Y., Kim, S. J., and Moon, B. K. (2009). Inhibitory effect of commercial green tea and rosemary leaf powders on the growth of foodborne pathogens in laboratory media and oriental-style rice cakes. J. Food Prot. 72, 1107–1111.
Li, B. H., Zhang, R., Du, Y. T., Sun, Y. H., and Tian, W. X. (2006). Inactivation mechanism of the beta-ketoacyl-[acyl carrier protein] reductase of bacterial type-II fatty acid synthesis by epigallocatechin gallate. Biochem. Cell Biol. 84, 755–762. doi: 10.1139/o06-047
Li, M., Liu, J. T., Pang, X. M., Han, C. J., and Mao, J. J. (2012). Epigallocatechin-3-gallate inhibits angiotensin II and interleukin-6-induced C-reactive protein production in macrophages. Pharmacol. Rep. 64, 912–918. doi: 10.1016/S1734-1140(12)70886-1
Lin, Y. S., Tsai, Y. J., Tsay, J. S., and Lin, J. K. (2003). Factors affecting the levels of tea polyphenols and caffeine in tea leaves. J Agric. Food Chem. 51, 1864–1873. doi: 10.1021/jf021066b
Mbata, T. I., Debiao, L. U., and Saikia, A. (2008). Antibacterial activity of the crude extract of Chinese green tea (Camellia sinensis) on Listeria monocytogenes. Afr. J. Biotechnol. 7, 1571–1573. doi: 10.5897/AJB06.316
Naderi, N. J., Naikan, M., Kharazi Fard, M. J., and Zardi, S. (2011). Antibacterial activity of Iranian green and black tea on Streptococcus mutans: an in vitro study. J. Dent. (Tehran) 8, 55–59.
Nakagawa, K., Okuda, S., and Miyazawa, T. (1997). Dose-dependent incorporation of tea catechins, (-)-epigallocatechin-3-gallate and (-)-epigallocatechin, into human plasma. Biosci. Biotechnol. Biochem. 61, 1981–1985. doi: 10.1271/bbb.61.1981
Navarro-Martinez, M. D., Garcia-Canovas, F., and Rodriguez-Lopez, J. N. (2006). Tea polyphenol epigallocatechin-3-gallate inhibits ergosterol synthesis by disturbing folic acid metabolism in Candida albicans. J. Antimicrob. Chemother. 57, 1083–1092. doi: 10.1093/jac/dkl124
Navarro-Martinez, M. D., Navarro-Peran, E., Cabezas-Herrera, J., Ruiz-Gomez, J., Garcia-Canovas, F., and Rodriguez-Lopez, J. N. (2005). Antifolate activity of epigallocatechin gallate against Stenotrophomonas maltophilia. Antimicrob. Agents Chemother. 49, 2914–2920. doi: 10.1128/AAC.49.7.2914-2920.2005
Okamoto, M., Leumg, K. P., Ansai, T., Sugimoto, A., and Maeda, N. (2003). Inhibitory effects of green tea catechins on protein tyrosine phosphatase in Prevotella intermedia. Oral Microbiol. Immunol. 18, 192–195. doi: 10.1034/j.1399-302X.2003.00056.x
Okamoto, M., Sugimoto, A., Leung, K. P., Nakayama, K., Kamaguchi, A., and Maeda, N. (2004). Inhibitory effect of green tea catechins on cysteine proteinases in Porphyromonas gingivalis. Oral Microbiol. Immunol. 19, 118–120. doi: 10.1046/j.0902-0055.2003.00112.x
Osterburg, A., Gardner, J., Hyon, S. H., Neely, A., and Babcock, G. (2009). Highly antibiotic-resistant Acinetobacter baumannii clinical isolates are killed by the green tea polyphenol (-)-epigallocatechin-3-gallate (EGCG). Clin. Microbiol. Infect. 15, 341–346. doi: 10.1111/j.1469-0691.2009.02710.x
Passat, D. N. (2012). Interactions of black and green tea water extracts with antibiotics activity in local urinary isolated Escherichia coli. J. AlNahrain Univ. 15, 134–142. Available online at: http://www.jnus.org/pdf/1/2012/1/636.pdf
Paveto, C., Guida, M. C., Esteva, M. I., Martino, V., Coussio, J., Flawia, M. M., et al. (2004). Anti-Trypanosoma cruzi activity of green tea (Camellia sinensis) catechins. Antimcrob. Agents Chemother. 48, 69–74. doi: 10.1128/AAC.48.1.69-74.2004
Radji, M., Agustama, R. A., Elya, B., and Tjampkasari, C. R. (2013). Antimicrobial activity of green tea extract against isolates of methicillin-resistant Staphylococcus aureus and multi-drug resistant Pseudomonas aeruginosa. Asian Pac. J. Trop. Biomed. 3, 663–667. doi: 10.1016/S2221-1691(13)60133-1
Rao, S., Timsina, B., and Nadumane, V. K. (2014). Antimicrobial effects of medicinal plants and their comparative cytotoxic effects on HEPG2 cell line. Int. J. Pharm. Pharm. Sci. 6, 101–105. Available online at: http://www.ijppsjournal.com/Vol6Issue1/7601.pdf
Reygaert, W., and Jusufi, I. (2013). Green tea as an effective antimicrobial for urinary tract infections caused by Escherichia coli. Front. Microbiol. 4:162. doi: 10.3389/fmicb.2013.00162
Roowi, S., Stalmach, A., Mullen, W., Lean, M. E., Edwards, C. A., and Crozier, A. (2010). Green tea flavan-3-ols: colonic degradation and urinary excretion of catabolites by humans. J. Agric. Food Chem. 58, 1296–1304. doi: 10.1021/jf9032975
Sakanaka, S., and Okada, Y. (2004). Inhibitory effects of green tea polyphenols on the production of a virulence factor of the periodontal-disease-causing anaerobic bacterium Porphyromonas gingivalis. J. Agric. Food Chem. 52, 1688–1692. doi: 10.1021/jf0302815
Serafini, M., Del Rio, D., Yao, D. N., Bettuzzi, S., and Peluso, I. (2011). “Health benefits of tea”, in Herbal Medicine: Biomolecular and Clinical Aspects, 2nd Edn, Chapter 12, eds I. F. F. Benzie and S. Wachtel-Galor (Boca Rotan, FL: CRC Press), 239–262. doi: 10.1201/b10787-13
Shah, S., Stapleton, P. D., and Taylor, P. W. (2008). The polyphenol (-)-epicatechin gallate disrupts the secretion of virulence-related proteins by Staphylococcus aureus. Lett. Appl. Microbiol. 46, 181–185. doi: 10.1111/j.1472-765X.2007.02296.x
Sharma, A., Gupta, S., Sarethy, I. P., Dang, S., and Gabrani, R. (2012). Green tea extract: possible mechanism and antibacterial activity on skin pathogens. Food Chem. 135, 672–675. doi: 10.1016/j.foodchem.2012.04.143
Shin, W. J., Kim, Y. K., Lee, K. H., and Seong, B. L. (2012). Evaluation of the antiviral activity of a green tea solution as a hand-wash disinfectant. Biosci. Biotechnol. Biochem. 76, 581–584. doi: 10.1271/bbb.110764
Sirk, T. W., Brown, E. F., Friedman, M., and Sum, A. K. (2009). Molecular binding of catechins to biomembranes: relationship to biological activity. J. Agric. Food Chem. 57, 6720–6728. doi: 10.1021/jf900951w
Sirk, T. W., Brown, E. F., Sum, A. K., and Friedman, M. (2008). Molecular dynamics study on the biophysical interactions of seven green tea catechins with lipid bilayers of cell membranes. J. Agric. Food Chem. 56, 7750–7758. doi: 10.1021/jf8013298
Song, J. M., Lee, K. H., and Seong, B. L. (2005). Antiviral effect of catechins in green tea on influenza virus. Antiviral Res. 68, 66–74. doi: 10.1016/j.antiviral.2005.06.010
Stalmach, A., Troufflard, S., Serafini, M., and Crozier, A. (2009). Absorption, metabolism and excretion of Choladi green tea flavan-3-ols by humans. Mol. Nutr. Food Res. 53, S44–S53. doi: 10.1002/mnfr.200800169
Stapleton, P. D., Shah, S., Hara, Y., and Taylor, P. W. (2006). Potentiation of catechin gallate-mediated sensitization of Staphylococcus aureus to oxacillin by nongalloylated catechins. Antimicrob. Agents Chemother. 50, 752–755. doi: 10.1128/AAC.50.2.752-755.2006
Steinmann, J., Buer, J., Pietschmann, T., and Steinmann, E. (2013). Anti-infective properties of epigallocatechin-3-gallate (ECGC), a component of green tea. Br. J. Pharmacol. 168, 1059–1073. doi: 10.1111/bph.12009
Stoicov, C., Saffari, R., and Houghton, J. M. (2009). Green tea inhibits Helicobacter pylori growth in vivo and in vitro. Int. J. Antimicrob. Agents 33, 473–478. doi: 10.1016/j.ijantimicag.2008.10.032
Subramani, C., and Natesh, R. K. (2013). Molecular mechanisms and biological implications of green tea polyphenol, (-)-epigallocatechin -3- gallate. Int. J. Pharm. Biosci. Tech. 1, 54–63.
Sudano-Roccaro, A., Blanco, A. R., Giuliano, F., Rusciano, D., and Enea, V. (2004). Epigallocatechin-gallate enhances the activity of tetracycline in staphylococci by inhibiting its efflux from bacterial cells. Antimicrob. Agents Chemother. 48, 1968–1973. doi: 10.1128/AAC.48.6.1968-1973.2004
Sugita-Konishi, Y., Hara-Kudo, Y., Amano, F., Okubo, T., Aoi, N., Iwaki, M., et al. (1999). Epigallocatechin gallate and gallocatechin gallate in green tea catechins inhibit extracellular release of vero toxin from enterohemorrhagic Escherichia coli O157:H7. Biochim. Biophys. Acta 1472, 42–50. doi: 10.1016/S0304-4165(99)00102-6
Ullmann, U., Haller, J., Decourt, J. P., Girault, N., Girault, J., Richard-Caudron, A. S., et al. (2003). A single ascending dose study of epigallocatechin gallate in healthy volunteers. J. Int. Med. Res. 31, 88–101. doi: 10.1177/147323000303100205
Van Amelsvoort, J. M., Van Hof, K. H., Mathot, J. N., Mulder, T. P., Wiersma, A., and Tijburg, L. B. (2001). Plasma concentrations of individual tea catechins after a single oral dose in humans. Xenobiotica 31, 891–901. doi: 10.1080/00498250110079149
Wang, Y., and Ho, C. T. (2009). Polyphenolic chemistry of tea and coffee: a century of progress. J. Agric. Food Chem. 57, 8109–8114. doi: 10.1021/jf804025c
Wang, Y., and Ma, S. (2013). Recent advances in inhibitors of bacterial fatty acid synthesis type II (FASII) system enzymes as potential antibacterial agents. ChemMedChem 8, 1589–1608. doi: 10.1002/cmdc.201300209
Williamson, M. P., McCormick, T. G., Nance, C. L., and Shearer, W. T. (2006). Epigallocatechin gallate, the main polyphenol in green tea, binds to the T-cell receptor, CD4: potential for HIV- 1 therapy. J. Allergy Clin. Immunol. 118, 1369–1374. doi: 10.1016/j.jaci.2006.08.016
Xu, J., Wang, J., Deng, F., Hu, Z., and Wang, H. (2008). Green tea extract and its major component epigallocatechin gallate inhibits hepatitis B virus in vitro. Antiviral Res. 78, 242–249. doi: 10.1016/j.antiviral.2007.11.011
Yam, T. S., Hamilton-Miller, J. M., and Shah, S. (1998). The effect of a component of tea (Camellia sinensis) on methicillin resistance, PBP2' synthesis, and beta-lactamase production in Staphylococcus aureus. J. Antimicrob. Chemother. 42, 211–216. doi: 10.1093/jac/42.2.211
Yam, T. S., Shah, S., and Hamilton-Miller, J. M. (1997). Microbiological activity of whole and fractionated crude extracts of tea (Camellia sinensis), and of tea components. FEMS Microbiol. Lett. 152, 169–174. doi: 10.1111/j.1574-6968.1997.tb10424.x
Yamakuchi, M., Bao, C., Ferlito, M., and Lowenstein, C. J. (2008). Epigallocatechin gallate inhibits endothelial exocytosis. Biol. Chem. 389, 935–941. doi: 10.1515/BC.2008.095
Yang, C. S., Chen, L., Lee, M. J., Balentine, D., Kuo, M. C., and Schantz, S. P. (1998). Blood and urine levels of tea catechins after ingestion of different amounts of green tea by human volunteers. Cancer Epidemiol. Biomarkers Prev. 7, 351–354.
Zhang, Y. M., and Rock, C. O. (2004). Evaluation of epigallocatechin gallate and related plant polyphenols as inhibitors of the FabG and FabI reductases of bacterial type II fatty-acid synthesis. J. Biol. Chem. 279, 30994–31001. doi: 10.1074/jbc.M403697200
Zhao, W. H., Hu, Z. Q., Hara, Y., and Shimamura, T. (2001a). Inhibition by epigallocatechin gallate (EGCg) of conjugative R plasmid transfer in Escherichia coli. J. Infect. Chemother. 7, 195–197. doi: 10.1007/s101560100035
Keywords: antimicrobial, green tea, synergism, catechins, EGCG
Citation: Reygaert WC (2014) The antimicrobial possibilities of green tea. Front. Microbiol. 5:434. doi: 10.3389/fmicb.2014.00434
Received: 30 April 2014; Accepted: 31 July 2014;
Published online: 20 August 2014.
Edited by:
Giovanna Suzzi, Università degli Studi di Teramo, ItalyReviewed by:
Nicoletta Belletti, IRTA, SpainCopyright © 2014 Reygaert. This is an open-access article distributed under the terms of the Creative Commons Attribution License (CC BY). The use, distribution or reproduction in other forums is permitted, provided the original author(s) or licensor are credited and that the original publication in this journal is cited, in accordance with accepted academic practice. No use, distribution or reproduction is permitted which does not comply with these terms.
*Correspondence:cmV5Z2FlcnRAb2FrbGFuZC5lZHU=
Disclaimer: All claims expressed in this article are solely those of the authors and do not necessarily represent those of their affiliated organizations, or those of the publisher, the editors and the reviewers. Any product that may be evaluated in this article or claim that may be made by its manufacturer is not guaranteed or endorsed by the publisher.
Research integrity at Frontiers
Learn more about the work of our research integrity team to safeguard the quality of each article we publish.