- 1Advanced Technologies for Environment and Smart Cities, Department of Chemistry, Faculty of Science of Sfax, University of Sfax, Sfax, Tunisia
- 2Institut Européen des Membranes (UMR5635), University Montpellier, Montpellier, France
The rapid expansion of the textile industry in Tunisia has resulted in ever-increasing discharges of wastewater, making it necessary to set up treatment systems allowing i) its discharge into the environment or ii) to recycle the water in the textile plant. In this study, several unitary operations were tested in order to improve the treated water quality. Thus, the following unit operations were applied: adsorption using Natural Clay (NC) and Powdered Activated Carbon (PAC), ultrafiltration (UF) and nanofiltration (NF) individually or in synergy: UF, nanofiltration, PAC-UF, NC-UF, UF-NF et Adsorption-UF/NF. The experiments were carried out on real textile effluent containing Indigo blue dye. A threshold concentration was observed above which performance no longer increases. NF alone showed better reduction of COD (67%), color (78%), and turbidity (98%) than UF alone (26%, 48%, 95%, respectively). The coupling UF–NF showed a clear improvement in water quality in terms of color (99%) and COD (98%) with an improvement in the NF flux from 67.81 L/h.m2 to 90.62 L/h.m2. No fouling was observed for NF while it is significant during UF. The used of adsorption as pretreatment to filtration leads to an enhancement of the treatment performances with a removal of 99.9%, 99.5% and 79.5% respectively for turbidity, color and COD for NC-UF and a removal of 100%, 99.4% and 79.6% respectively for turbidity, color and COD for PAC-UF. Moreover, the addition of adsorbent controls the fouling and a constant and high flux is quickly attained and remains stable over time. The use of natural clay as adsorbent represents an economically profitable solution since it can be obtained locally and the clay used can be reused in the ceramic industry as an additive for road surfacing without the need for regeneration.
1 Introduction
The greatest threat to the environment and ecosystems is water pollution. Whether accidental or deliberate, this pollution is the result of organic and mineral pollutants from agricultural, urban and industrial sources. The textile industry, in particular, is a major source of pollutant-laden liquid effluents, due to the large volume of water dyeing and substances used in printing, dyeing and finishing operations. Among the industrial sectors, the textile industry which usually uses dyes and a variety of chemical additives (Bedin et al., 2018; Panão et al., 2019). As a result, it is expected that more than 300,000 tons per year will be discharged from the textile industry, excluding other sectors (Kadhom et al., 2020). Textile wastewater is often rich in color and also of extreme pH with various types of chemicals (persistence and toxic). Due to this reason, many countries have now introduced more stringent discharge standards for textile wastewater.
During the past two decades, different treatment technologies have been studied to evaluate the sustainable treatment of textile wastewater. The selection of a suitable type of technology depends on the production process and chemical usage of the textile mill, constituents of effluent, discharge standards and location, capital and operating costs, availability of land area, options of reusing/recycling the treated wastewater and skills and expertise available.
Nowadays, several treatment methods are available for degradation and removal of dyes from wastewater. A few of these methods include, chemical oxidation (Ertugay and Acer, 2017), filtration (Karisma D et al., 2017), biological treatment (Gezer, 2018), biochemical degradations (Hassaan et al., 2017), ion-exchange removal (Ebrahiem et al., 2017), adsorption (EL Alouani et al., 2018), photocatalysis (Pathania et al., 2016). In comparison with chemical and biological methods, membrane based method such as nanofiltration (NF) and ultrafiltration (UF), have emerged a promising physical treatment method. Advantages of membrane based method, in comparison with other methods, include easiness and green operation, high efficiency and low cost treatment (Yanan et al., 2011).
A number of studies exist on the use of hybrid membrane processes for the treatment of wastewater and textile effluents to remove dye content (Sarkar et al., 2014; Nouri Alavijeh et al., 2017; Mzahma et al., 2023 evaluates the quality of waters treated by membrane processes and puts forward a scenario for optimizing the recycling practices of treated textile effluents in agriculture. The results indicate that the NF and RO membranes have a high affinity to remove monovalent and multivalent ions. Indeed, the removal of SO42−, Na+, and Cl− by NF was 83%, 61%, and 55%, respectively. Thus, the RO reduces approximately 96% of these elements; Bousbih et al. (2020) fabricated a ceramic membrane developed from natural Tunisian kaolin clay to evaluate the treatment of textile wastewater. They noted that MF membranes are capable of removing oil from wastewater with a retention rate of 95.3%. They noted the performance of the membrane during the treatment of raw effluents is promising in terms of discoloration (99%), chemical oxygen demand (COD) (80%) and turbidity (98%). Sarioglu Cebeci and Torun (2017) used an NF membrane with a cutoff threshold of 150–300 Da. Experimental data show that the efficiency of this membrane in terms of turbidity, conductivity, COD and color (methylene blue) are 100%, 95%, 98% and 98% respectively.
However, membrane processes are generally limited by the phenomenon of clogging, which leads to a reduction in filtration flux over time. Treatment can follow two approaches in which pretreatment is required; the combination of membrane processes or hybrid treatment. Pre-treatment reduces the clogging effect and improves the performance of the main treatment. Different methods of pre-treatment before membrane filtration have been explored for many years, such as adsorption, coagulation, biological treatment processes and oxidation (Kim et al., 2012; Yu et al., 2017; Tian et al., 2018; Yu et al., 2018). Masmoudi et al. (2015) studied the combination of coagulation-flocculation-nanofiltration and found that this process exhibited a good ability to remove organic pollutants, total discoloration of textile effluents was observed with rejection of 98% of soluble organic matter and 99% of salts. Photocatalysis was applied by Gebrati et al. (2010), their results showed a removal rate of 53.82% for chemical oxygen demand (COD) and 89% for discoloration of effluents. Özgün et al., 2022 presents a comparative study of microfiltration (MF) (0.2 µm and 0.05 µm), ultrafiltration (UF), ozonation (0.1, 0.2 g/L ozone), ultraviolet (UV) irradiation and titanium dioxide (TiO2) (0.05, 0.15, 0.3, 0.5 g/L TiO2) and zeolite adsorption (125, 250, 500 mL/min flow rates) as pre-treatment prior to nanofiltration (NF) and reverse osmosis (RO) for the treatment of real textile washing wastewater. Experiments demonstrated that the applied pre-treatment methods enhanced the flux performance of NF270 and RO membranes except zeolite adsorption. The principal purpose of these alternative by using adsorption is to remove the problematic of contaminants before they reach the membrane surface causing significant fouling effects.
The hybrid adsorption-membrane filtration process can constitute an effective solution providing totally discolored water (Al-Bastaki and Banat, 2004; Zheng et al., 2009). The color removal efficiency can be controlled by varying adsorbent dosage, the type of the adsorbent and the addition of the membrane separation process (Guo et al., 2005; Kim et al., 2008). Many adsorbent materials are available for color removal from dyeing waste water treatment. Activated carbon (Mourid et al., 2017), waste wood (Tripti and Dilip, 2018, coconut husks (Aljeboree et al. (2017), orange peel (Ahmed et al., 2020), agricultural waste such as Terminalia arjuna sawdust (Shakoor et al., 2018), sawdust (Mashkoor and Nasar, 2018), citrus limetta peel (Shakoor and Nasar, 2016), eggshell (de Oliveira Zonato et al., 2022), cucumis sativus peel (Shakoor and Nasar, 2017), corncob (Song et al., 2019), Punica granatum peel (Shakoor and Nasar, 2018), Artocarpus camansi peel (Chieng et al., 2017) etc. Monnot et al. (2016), studied the adsorption of indigo dye using TiO2 modified clay and zeolite ultrafiltration membrane hybrid system and they find that the presence of Sm-TiO2 NCs reduced UF membrane fouling (Permeate flow increased from 71 L/h.m2 (UF only) to 182 L/h.m2 (Sm-TiO2/UF)). Same performances were observed by Acero et al. (2012) who noted that the pre-treatment of effluents with powdered activated carbon before UF makes it possible to improve the filtration flow and reduce the clogging phenomenon. In another side, Lakdioui and EL Harfi (2015) studied the performance of a UF membrane (PSU/titanium dioxide TiO2/DMF) and the adsorption of indigo bleu on powdered activated carbon. They noticed that the retention of indigo by adsorption (80%) is slightly higher than that by UF (76%). To study the behavior of an NF membrane with respect to eliminating indigo dye.
This work focuses on the application of adsorption alone and combined with the membrane process in a hybrid system (Adsorption-Ultrafiltration in continuous mode) for the treatment of colored effluents from the textile industry. In order to highlight the interest of combining adsorption with membrane separation in qualitative and quantitative terms. Activated carbon (PAC) and natural clay (NC) are the adsorbents used. Ultrafiltration (UF) and nanofiltration (NF) have also been used in different configurations: alone and hybrids (adsorption (A)/UF, A/UF/NF and UF/NF).
2 Materials and methods
2.1 Wastewater influent
The studied effluents were taken from the outlet of SITEX wastewater treatment plant (Ksar Hellal, Tunisia) which corresponds to an activated sludge treatment (Chamam et al., 2007). These effluents are known to be highly colored confirming the presence of dye mainly based on indigo bleu with a small amount of reactive dyes Their discharge into the natural environment involves the release of numerous surfactants, biocidal compounds, solid suspensions, dispersing and wetting agents, dyes and trace metals, which, of course are toxic to most living organisms (Table 1). Moreover, the heterogeneity of their composition makes it difficult or almost impossible to obtain pollution thresholds imposed by environmental standards, after treatment by usual techniques.
2.2 Adsorbents
In this study, two types of adsorbents were studied in order to highlight the influence of their properties on the adsorption performance.
- Powdered activated carbon (PAC): This is the most popular commercial adsorbent due to its wide adsorption capacity. PAC is used as control/reference adsorbent.
- Natural clay (NC): is a clay collected from the region of Fouchana (North–East, Tunisia). It comes from local clay sediments. This clay is brown and have spots of iron oxide in places. This Natural clay was selected for its physical properties (fine particles) and its nearby availability, which is an economic advantage. Their characteristics are listed in Table 2.
2.3 Membranes and filtration units
Multitubular ceramic ultrafiltration membrane (KERASEP) based on titanium dioxide and alumina (TiO2- Al2O3) was used. The membrane has a weight cut-off of 150 KDa and a total filtration surface area of 0.155 m2. The feed unit is equipped with a feed tank of 30 L, a flowmeter to determine the feed flow and two pressure gauges placed upstream and downstream of the membrane whereas the tangential flow is ensured by means of a circulation pump (Figure 1).
For the NF tests, a laboratory-scale test bench (Ioncep50) provided by the company PTE (water purification and treatment) was used (Figure 1). The membrane used is spiral-wound module. The feed tank has a capacity of 30 L. The tangential circulation is ensured by means of a circulation pump (VEMAT type VMB 71 B, Italy) which also helps to ensure the transmembrane pressure. The operating pressure (TMP) was fixed to 7 bars for NF and filtration tests were performed at ambient temperature 25°C ± 3°C thanks to a cooling system.
The UF and NF experimental set-up operates in recirculation mode, i.e., the retentate returns to the feed tank and the permeate is recovered. Filtration flux measurement and permeate sampling are carried out every 10 min during filtration time.
The characteristics of both membranes are illustrated in Table 3.
2.4 Experimental protocol
2.4.1 Membrane permeability measurement
The membrane permeability is determined by representing the variation of water permeate flux with the transmembrane pressure (TMP). The water permeability is given by Eq. 1:
Where ΔP is the pressure difference on either side of the membrane (bar). Lp is the water permeability (L h−1 m−2 bar−1) and JW the water permeate flux (L/h m2).
The water permeability found is of 230 L h−1 m−2 bar−1 for ultrafiltration against 12.56 L h−1 m−2 bar−1 for nanofiltration (Figure 2).
2.4.2 Filtration protocol
The UF and NF experimental device operates in recirculation mode, i.e., the retentate returns to the feed tank and the permeate is recovered. The measurement of the filtration flow and the sampling of the permeate are carried out during the filtration time (every 10 min).
2.4.3 Protocol for the combination of membrane processes
In the case of a UF/NF membrane process combination, the UF is considered as pretreatment for the NF. A volume of 30 L of solution (real effluent) was poured into the UF supply bench at the start of each test, the permeate obtained is collected then feeds the NF. The operating pressure must be determined subsequently for the two membranes. The temperature of the effluent during the tests is set at ambient 25 ± 3 (cooling system). The filtration flow is measured continuously over time for both UF and NF membranes.
2.4.4 Hybrid adsorption/UF process protocol
The protocol for the hybrid adsorption/membrane filtration process for wastewater treatment is described below (Figure 3).
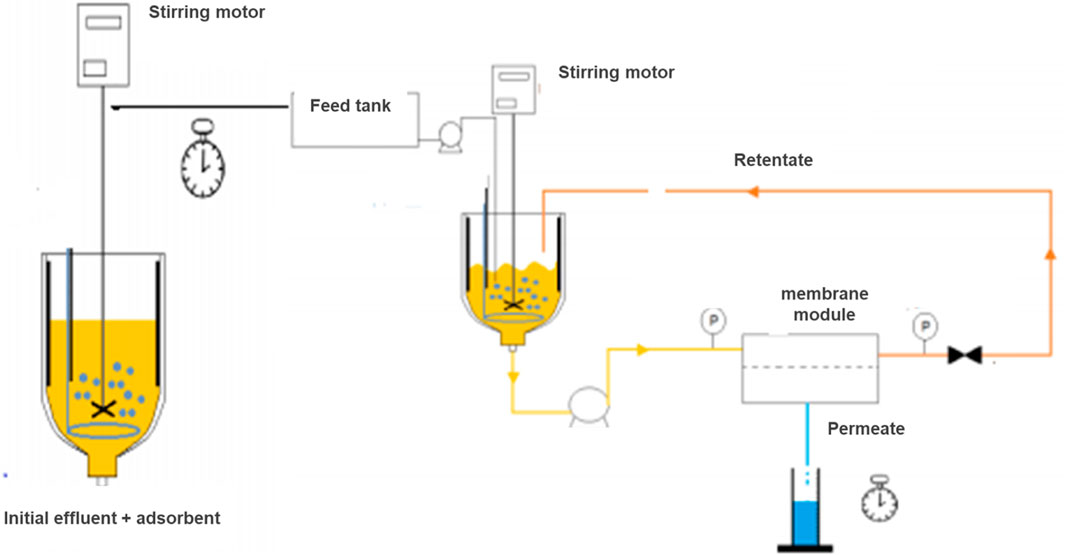
Figure 3. Schematic diagram of the hybrid system combining the adsorption and membrane processes: Adsorption–Ultrafiltration.
In this study, powdered activated carbon (PAC) and natural clay (NC) were chosen. Natural clay was selected as a natural adsorbent for its physical properties (fine particles) and its availability in nature, which constitutes an economic advantage.
All tests are carried out at constant volume (the volume of permeate is equal to the volume added) and therefore at a constant concentration. From a 5 L beaker, the adsorbent–textile effluent mixture to be treated is sent using a membrane pump (Quattra Flow 150S, Pall, France) into the feed tank with a volume of 30 L.
To study the adsorption/UF coupling, the influence of the adsorbent dose on the performance of the UF membrane is evaluated from 50 mg/L up to 300 mg/L for the two adsorbents. The different doses of adsorbents are added directly to the UF feed tank (zero contact time). The permeate is recovered to essentially measure COD, dye content, and turbidity.
2.5 Analytical methods
The purification efficiency was monitored by measuring the following parameters, COD, dye content and turbidity. Chemical oxygen demand (COD) analysis was measured with a LCK 214 Mercury-free method Test. Turbidity was determined by a HACH 2100AN IS turbidimeter. Dye concentration was determined calorimetrically at maximum absorbance at 590 nm using UV visible spectrophotometer (UV 7205 JENWAY spectrophotometer). The rejection rate was determined using Eq. 2:
Where Cp and Cf are concentrations in feed and permeate, respectively.
3 Results and discussion
3.1 Treatment by membrane processes
3.1.1 Direct UF treatment
From Figure 4A, it is worth noting that the permeate flux decreases drastically from 445.12 to 257.29 L/h m2 after 190 min of filtration when only UF was applied. This decline represents 43% of the initial permeate flux value. This decrease is due in part to pore clogging, essentially linked to the accumulation of organic matter in the vicinity of the membrane (Dhakal et al., 2018; Yin et al., 2019). The formation of fouling layer increases the membrane retention, which seems to stabilize after almost 1 h of filtration.
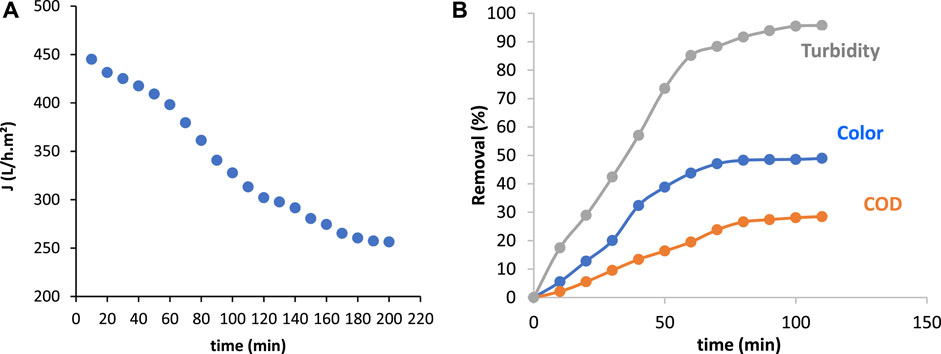
Figure 4. (A) Variation of permeate flux with time after direct UF (TMP = 3 bar et T = 25°C); (B) Evolution of the retention of turbidity, color and COD with time after direct UF textile effluent treatment (TMP = 3 bars).
Indeed, Figure 4B shows a very high turbidity retention (>95%) followed by a medium color retention (50%) and a low COD retention (28%) after 1 h of filtration. These results confirm that ultrafiltration removes only a small proportion of organic matter. According to the results found by Chollom et al. (2015), COD removal did not exceed 35%. This finding confirms that UF membrane retains only a small fraction of organic molecules due to the difference between the membrane pore size and the retained molecules size very smaller than the pore size (Shon et al., 2009). Then, in order to increase the COD retention, there are two possible solutions, decreasing the membrane weight cut-off by adding Nanofiltration or/and adding adsorbent (PAC or AN).
3.1.2 Direct NF treatment
Tests on the treatment of textile industry effluent by NF were carried out at different transmembrane pressure (TMP) (4–8 bar). The results show that filtration flux increases with increasing TMP (Figure 5A), ranging from 20.65 L/h.m2 (4 bar) to 70.17 L/h.m2 (7 bar). The permeability is closed to that determined with pure water. Nevertheless, the curve does not pass through zero confirming the negative impact of the osmotic pressure, around 2 bar (Figure 5B). Moreover, above 7–8 bar, the function Jw = f(TMP) would no longer be linear.
Concerning the water quality, the NF membrane results in total turbidity removal of 98% and color retention of around 78% under a transmembrane pressure of 7 bars. However, NF does not totally remove the organic matter in terms of COD (68%) (Figure 6) even if COD performance is at least twice as good as UF. As with NF, performance increases over time, confirming the positive impact of the membrane deposit on retention performance.
3.1.3 Coupling of UF/NF
The UF is used as NF pretreatment: the UF permeate is indeed the NF feed. The tests were carried out at constant pressure and the change in permeate flow over time reflects the fouling propensity (Figure 7). First, an increase in permeate flow was observed from 67.44 L/h.m2 (NF alone) to 90.61 L/h.m2 (UF + NF). This improvement indicates that the use of UF as a pretreatment makes it possible to eliminate the molecules responsible for membrane fouling. Moreover, additional tests perform to measure the fouling reversibility (measurement of used rinsed membranes water flux) showed that the clogging of the NF membrane was reversible for the UF/NF operation, whereas a 35% loss of flux was measured for the NF experiment. From an economic point of view, this hybrid treatment made possible to increase the lifetime of the membrane and also reduce the membrane cleaning frequency. In addition, Figures 8A, B shows a clear improvement in water quality: the color retention rate was 77.81% for single NF against 99.97% for UF/NF and the COD retention was 68.38% for single NF against 98.68% for UF/NF.
3.2 Hybrid adsorption/UF treatment
3.2.1 Effect of dose of adsorbent
From Figure 9, it should be noted that the permeate flux decreases drastically and continuously when UF was applied without adsorbent whereas the use of adsorbent, allows a rapid permeate flux stabilization (after only 15 min of filtration) whatever the dose of adsorbent used (50 mg/L–300 mg/L). For PAC, the stabilized permeate flux increases with the adsorbent dose from 259.2 L/h.m2 for 50 mg PAC/L to 385.67 L/h.m2 for 150 mg PAC /L. As the permeate flux no longer increases above 150 mgPAC/L (Figure 8A), increasing the adsorbent above 150 mgPAC/L is not useful as it does not bring any additional gains. The value is nevertheless twice lower that the threshold concentration obtained during adsorption (300 mgPAC/L). Concerning the natural clay NC, the same trends (slightly higher) are observed: the stabilized flow was 314 L/h.m2 for a dose of 50 mgNC/L; 351 L/h.m2 for a dose of 100 mgNC/L and increased to 400 L/h.m2 for NC doses above 150 mgNC/L (Figure 8B). Consequently, the optimal dose of 150 mg/L for PAC and 200 mg/L for NC were retained for the following tests. The results confirm that coupling of adsorption with the UF membrane limits the clogging phenomenon (Tansakul et al., 2011; Zielińska and Galik, 2017).
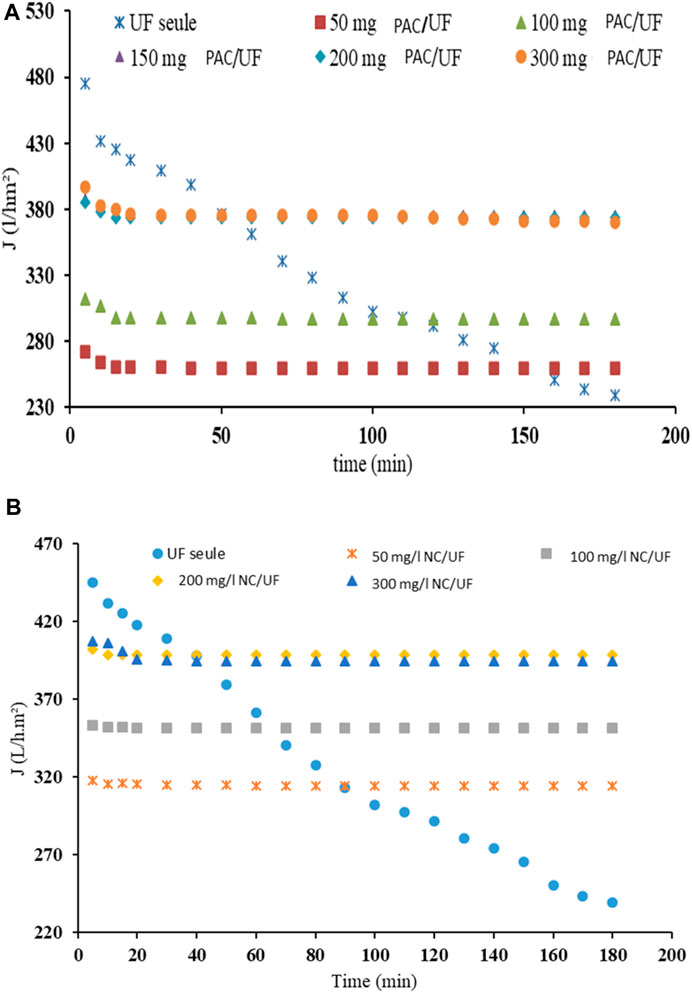
Figure 9. Variation of permeate flux with time (TMP = 3 bar) at different doses of (A) Powdered Activated Carbon PAC; (B) Natural Clay NC.
The performance of the hybrid adsorbent/UF process in terms of turbidity, color and COD removal was investigated. Table 4 shows that adsorption plays a dominant role in the hybrid process, with COD retention ranging from less than 30% in the case of single UF and 53%–59%, to 47%–52% when 50 mg/L and 100 mg/L of PAC and NC respectively are added to the solution. At low doses (50–100 mg/L), the Adsorption/UF system in the presence of both adsorbents (PAC and NC) has no effect on COD retention. This may be due to the fact that the particles present in the feed solution were less than 150 kDa, resulting in high rejection by the UF. On the other hand, the hybrid system with the high adsorbent dosage achieved a higher COD removal efficiency than direct UF. Since the size of the aggregates formed during adsorption depends on the type of adsorbent (Stoller, 2009), in our case, the size or quantity of the flocs generated during adsorption appears to be high, which enabled more efficient size exclusion (Zielińska and Galik, 2017). We observed an increase in the COD retention during filtration with increasing adsorbent concentration, PAC (50–150 mg/L) and NC (50–200 mg/L). This increase can be explained by the accumulation of organic matter in the vicinity of the membrane, and also by the adsorption of organic substances on the adsorbents. An increase in the dose of PAC or NC applied lead to an increase in the number of free sites capable of adsorbing organic molecules. As the PAC and NC particles are totally retained by the UF membrane, the retention of organic matter is therefore increased compared to the application of a single UF.
Considering Table 4, the 150 mg/L CAP dose shows higher retention than that in the presence of 50 mg/L and 100 mg/L PAC, reaching 79.4%. Indeed, Lee and Kim (2014), Kim et al. (2008) and Lin et al. (1999) have shown that PAC preferentially adsorbs the organic matter. Whereas in the case of NC, the maximum COD retention was of 79% for a dose of 200 mg/L of NC. Increasing the dose beyond 150 mg/L for PAC and 200 mg/L for NC did not improve the retention. This confirms that the optimum dose of PAC and NC in the hybrid system is 150 mg/L and 200 mg/L respectively. The results recorded in this study are similar to those reported in the work of Seyhi et al. (2012). The retention of organic matter is very important to avoid the risk of damage to the UF membrane. The hybrid Adsorption/UF system is capable of removing COD, in this context, Lafi et al. (2018) used a hybrid UF/electrodialysis process to treat textile wastewater, they noted that the COD retention rate passes 54% and 77% for UF alone and UF/ED respectively. These results are comparable with our case study (Adsorption/UF).
In addition, increasing the concentration of PAC and NC adsorbent in the hybrid system led to an increase in the turbidity retention. Turbidity removal were 96.52%, 98.49% and 100% for PAC doses of 50 mg/L, 100 mg/L and 150 mg/L respectively. In the case of NC, the turbidity retention was over 99.90% for any clay doses added. In the case of a single UF, the retention reached 95%. These results are comparable to those obtained with single UF (95.72%). According to the literature, ultrafiltration is capable of removing turbidity, suspended matter and colloids (Naddeo et al., 2015; Arhin et al., 2018).
Color removal performance was also monitored. The color retention increased simultaneously with increasing adsorbent dose added to the hybrid system (Adsorption/UF). This behavior can be explained by the adsorbent’s efficiency in adsorbing dye molecules. Color retention did not exceed 50% for a single UF, 64%–99% for PAC doses 50 mg/L - 150 mg/L and 65%–83% for NC doses 50 mg/L - 200 mg/L. Al-Bastaki and Banat (2004) found that the addition of bentonite to the UF process significantly increased the retention of the blue methylene dye, representative of textile dye.
3.2.2 Effect of transmembrane pressure
Transmembrane pressure (TMP) is one of the parameters influencing ultrafiltration membrane performance in terms of filtration flux and pollutant retention (COD, turbidity, color, etc.). Experiments were carried out using the hybrid CAP/UF and NC/UF processes.
The results illustrated in Table 5 show that the best filtration fluxes are achieved at TMPs of 3 and 4 bar. However, a TMP of 3 bar achieves very good efficiencies in terms of COD, color and turbidity removal. For this reason, we have chosen 3 bar as the operating pressure. This result shows that permeate flux increases with increasing TMP. Above 3 bar, however, the flux no longer changes. It increases from 92.6 L/h.m2 to 385.2 L/h.m2 for TMPs between 1 and 3 bar for PAC/UF and from 105.5 L/h.m2 to 375.9 L/h.m2 for TMPs between 1 and 3 bar for NC/UF. Furthermore, filtration flux evolves linearly at low pressures (1 and 2 bar), then becomes pressure-independent at higher values (3 and 4 bar). The linear relationship between filtration flux and TMP indicates that concentration polarization has not yet taken place (Banat and AI-Bastaki, 2004). At 3 bar and above, concentration polarization occurs regardless of the membrane treatment configuration.
Table 5 illustrates pollutants removal in terms of turbidity, color and COD at different TMPs. The results exhibit that the flux-TMP relationship is linear until 3 bars. A TMP of 3 bar achieves very good efficiencies in terms of COD, color and turbidity removal. Therefore, 3 bar was chosen as the optimal operating pressure. Above 3 bar, the permeate flux no longer increases confirming that fouling layer conceals the pressure driving force. Otherwise, the COD retention peak at 3 bar (80%) (NC/UF and PAC/UF) does not increase any further confirming the UF working pressure of 3 bars.
3.2.3 Fouling determination
Fouling was defined as the loss of permeability (L.h−1.m−2. bar−1) over filtration time. Membrane clogging can be represented in a number of ways. Most simply, it can be represented graphically by showing the evolution of the permeability of one of its components: either the transmembrane pressure in the case of a constant flux test, or the flux in the case of a constant pressure test. This evolution can be a function of time or of the volume of water filtered, often normalized according to the filtering surface area (m2) for purposes of comparison between different membranes. The linearity of this drop in permeability is typically associated with the pore clogging or of a “cake” model.
Numerous studies show that organic matter is a major clogger of UF membranes for water potabilization (Cai et al., 2013; Said et al., 2015; Amosa, 2016). Indeed, although adsorbent particles can form a fairly porous layer themselves, the empty spaces between these particles can be filled by colloids and other organic matter.
From Figure 10, we can see that the coupling adsorption/membrane has a better benefit, especially on the performance of the UF. In our study, water permeability after filtration and before acid-base cleaning (227.24 L h−1 m−2 bar−1) was comparable to that of pure water in the presence of the new membrane (232.78 L h−1 m−2 bar−1). Membrane clogging can be eliminated by rinsing with water, and membrane permeability recovered completely without chemical cleaning. On the other hand, when single UF was applied, membrane permeability (131.51 L h−1 m−2 bar−1) falls sharply compared with that of the new membrane. This decrease is essentially due to fouling establishment.
3.3 Hybrid adsorption/UF/NF treatment
3.3.1 Adsorption/UF/NF performance
This section is focused on the study of hybrid processes: adsorption coupled with ultrafiltration, with the aim of improving the performance of the UF and subsequently of the NF. As far as coupling Adsorption/UF is concerned, only the effect of coupling NC/UF was studied under optimal adsorbent dose of 200 mg/L and TMP of 3 bar.
The permeate flux from the hybrid NC/UF process serves as feed for the NF. The variation of NF filtration flux with time is shown in Figure 11. We can see that the permeate flux in the NC/UF/NF configuration remains stable (97 L/h.m2). This implies that the hybrid process is capable of limiting the phenomenon of membrane clogging (Amosa, 2016). On the other hand, for NF and UF/NF, we observe a slight decrease in filtration flux over time. This decrease is essentially due to possible interactions between the solution and the membrane surface. If we compare the UF/NF and NC/UF/NF systems, the permeate flux obtained with NC/UF/NF appears to be higher than that with UF/NF.
Figure 12A shows the variation in retention rate in color as a function of time for real effluent treated with NF, UF/NF and NC/UF/NF. It can be seen that the retention was improved when NC/UF/NF was applied. The color removal was around 75.36%, 96.27% and 99.35% after 70 min filtration for single NF, UF/NF and NC/UF/NF respectively.
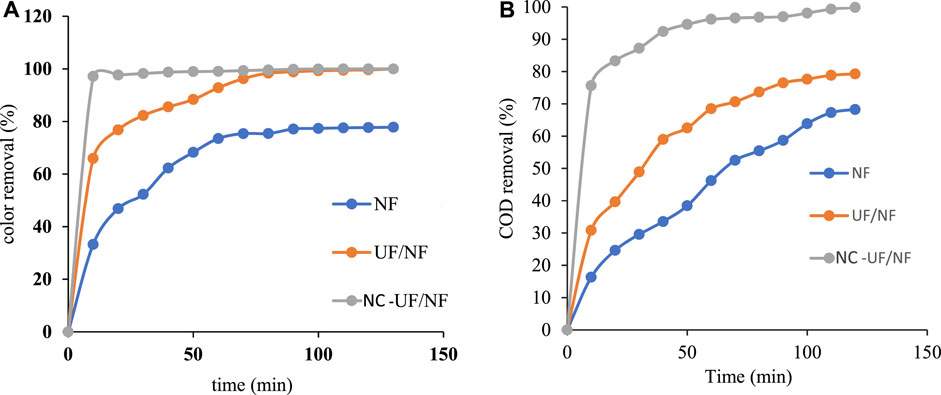
Figure 12. (A) Color evolution as a function of filtration time for the different processes (TMPNF = 7bars); (B) COD retention rate over time for the different processes (TMPNF = 7 bars, TMPUF = 3bars).
Figure 12B shows the COD retention by NF membrane using the permeates from the hybrid NC/UF process as feed solution. The retention still increases with the filtration time. It varies from 16.32% to 68.15%, 30.85%–79.26% and 75.62%–99.33% between 10 min and 120 min for NF, UF/NF and NC/UF/NF respectively. However, the combined UF/NF process shows low COD retention (79%) compared to the NC/UF/NF system (>99%). The addition of adsorption therefore further improves NF membrane performance, which is linked to the adsorption of part of the organic matter by NC. The results confirm that improvements in NF performance in terms of retention and permeate flux can be achieved by coupling adsorption and UF at the same time.
Table 6 summarizes the COD in the permeate for different configurations under the optimal conditions for each configuration.
3.3.2 Determination of fouling
In order to compare the fouling establishment during the different treatment stages, the relative permeate fluxes evolution (J(t)/Jo) was considered according to the specific permeate volume (V/membrane area: L/m2). The evolution of this normalized fouling (mass of deposit retain by the membrane per unit surface) was given in Figure 13. It can be show that the fouling during direct effluent filtration is significant. The dynamic fouling response is the same whatever the membrane pore size (for UF or NF membrane). The addition of the adsorbent greatly limits the fouling, with a slight decrease in flux of less than 4% for UF and 1% for NF was observed.
4 Conclusion
Improving effluent treatment efficiency in the textile industry can be achieved by combining several unit operations based on hybrid adsorption and membrane processes treatment system.
• During single filtration, water quality changes over time, confirming the influence of the deposit on pore size and therefore final retention. The Optimal operating pressures was 3 bars for UF and seven for NF. The fouling was still significant whereas the water quality remains constant after 80–90 min of filtration, achieving a COD removal efficiency of 26% and 68% for respectively UF and NF.
• The NF applied in post-treatment to UF allows also to improve the water quality (COD removal of 98%), but does not solve the problem of UF fouling.
• The addition of adsorption step before filtration (even under low concentration) makes possible to control fouling while improving water quality. Indeed, a constant flux was quickly attained and remained stable over time for both hybrid systems. It was observed that there is adsorbent dose (PAC 150 mg/L, NC 200 mg/L) above which efficacy remains constant.
• The COD retention achieved a maximum of 79% for both adsorbents (PAC/UF and NC/UF).
• The combination of adsorption/UF/NF gives very good results in terms of pollutants removal. The removal for turbidity, color and COD were of 100%, 99.3% and 99.1% respectively.
• The addition of the adsorbent greatly limits the fouling, with a slight decrease in flux of less than 4% for UF and 1% for NF was observed.
The choice of unit operations depends on what happens to the water destination. If the water is to be returned to the process, the target is to be colorless, whereas its discharge into the environment must comply with COD standard. In this specific case, only the combination of the three operations (Adsorption/UF/NF) will enable the standard to be met.
Data availability statement
The original contributions presented in the study are included in the article/Supplementary Material, further inquiries can be directed to the corresponding author.
Author contributions
SB: Writing–original draft. MM: Writing–review and editing. AA: Writing–review and editing. GL: Writing–review and editing. MH: Writing–review and editing. RB: Writing–review and editing.
Funding
The author(s) declare that no financial support was received for the research, authorship, and/or publication of this article.
Conflict of interest
The authors declare that the research was conducted in the absence of any commercial or financial relationships that could be construed as a potential conflict of interest.
Publisher’s note
All claims expressed in this article are solely those of the authors and do not necessarily represent those of their affiliated organizations, or those of the publisher, the editors and the reviewers. Any product that may be evaluated in this article, or claim that may be made by its manufacturer, is not guaranteed or endorsed by the publisher.
References
Al-Bastaki, N., and Banat, F. (2004). Combining ultrafiltration and adsorption on bentonite in a one-step process for the treatment of colored waters. Resour. Conserv. Recycl 41, 103–113. doi:10.1016/j.resconrec.2003.07.001
Acero, J. L., Javier, B., Francisco, J., and Fernando, R. T. (2012). Coupling of adsorption, coagulation, and ultrafiltration processes for the removal of emerging contaminants in a secondary effluent. Chem. Eng. J. 210, 1–8. doi:10.1016/j.cej.2012.08.043
Ahmed, M., Mashkoor, F., and Abu, N. (2020). Development, characterization, and utilization of magnetized orange peel waste as a novel adsorbent for the confiscation of crystal violet dye from aqueous solution. Groundw. Sustain. Dev. 10, 100322. doi:10.1016/j.gsd.2019.100322
Aljeboree, A. M., Alshirifi, A. N., and Alkaim, A. F. (2017). Kinetics and equilibrium study for the adsorption of textile dyes on coconut shell activated carbon. Arabian J. Chem. 10, S3381–S3393. doi:10.1016/j.arabjc.2014.01.020
Amosa, M. K. (2016). Towards sustainable membrane filtration of palm oil mill effluent: analysis of fouling phenomena from a hybrid PAC-UF process. Appl. Water Sci. 7, 3365–3375. doi:10.1007/s13201-016-0483-3
Arhin, S. G., Banadda, N., Komakech, A. J., Pronk, W., and Marks, S. J. (2018). Optimization of hybrid coagulation-ultrafiltration process for potable water treatment using response surface methodology. Water Sci. Technol. Water Supply 18, 862–874. doi:10.2166/ws.2017.159
Banat, F., and Ai-Bastaki, N. (2004). Treating dye wastewater by an integrated process of adsorption using activated carbon and ultrafiltration. Desalination 170, 69–75. doi:10.1016/j.desal.2004.02.093
Bedin, K., Souza, I., Cazetta, A. L., SpessatoRonix, L. A., and Almeida, V. D. C. (2018). CO2-spherical activated carbon as a new adsorbent for methylene blue removal: kinetic, equilibrium and thermodynamic studies. J. Mol. Liq. 269, 132–139. doi:10.1016/j.molliq.2018.08.020
Bousbih, S., Emna Errais, R. B. A., Darragi, F., Duplay, J., Trabelsi, M., Daramola, M. O., et al. (2020). Treatment of textile wastewater using monolayered ultrafiltation ceramic membrane fabricated from natural kaolin clay. Environ. Technol. 42 (3), 3348–3359. doi:10.1080/09593330.2020.1729242
Cai, M., Wang, S., and Liang, H. (2013). Modeling and fouling mechanisms for ultrafiltration of Huanggi (Radix astragalus) extracts. Food Sci. Biotechnol. 22, 407–412. doi:10.1007/s10068-013-0094-9
Chamam, B., Heran, M., and Grasmick, A. (2007). Raja Ben Amar, comparison of textile dye treatment by biosorption and membrane bioreactor. Environ. Technol. 28, 19. doi:10.1080/09593332808618892
Chieng, H. I., Lim, L. B. L., and Priyantha, N. (2017). Enhancement of crystal violet dye adsorption on Artocarpus camansi peel through sodium hydroxide treatment. Desalin. Water Treat. 58, 320–331. doi:10.5004/dwt.2017.0326
Chollom, M. N., Rathilal, S., Pillay, V. L., and Alfa, D. (2015). The applicability of nanofiltration for the treatment and reuse of textile reactive dye effluent. Water sa. 41, 398–405. doi:10.4314/wsa.v41i3.12
de Oliveira Zonato, R., Ramos Estevam, B., Dias Perez, I., dos Santos Ribeiro, V. A., and Freire Boina, R. (2022). Eggshell as an adsorbent for removing dyes and metallic ions in aqueous solutions. Clean. Chem. Eng. 2, 100023. doi:10.1016/j.clce.2022.100023
Dhakal, N., Salinas-Rodriguez, S. G., Ouda, A., Schippers, J. C., and Kennedy, M. D. (2018). Fouling of ultrafiltration membranes by organic matter generated by marine algal species. J. Membr.Sci. 555, 418–428. doi:10.1016/j.memsci.2018.03.057
Ebrahiem, E., Al-Maghrabi, M. N., and Mobarki, A. R. (2017). Removal of organic pollutants from industrial wastewater by applying photo–fenton oxidation technology. Arab. J. Chem. 10, S1674–S1679. doi:10.1016/j.arabjc.2013.06.012
El Alouani, M., Alehyen, S., El Achouri, M., and Taibi, M. (2018). Removal of cationic dye–methylene blue from aqueous solution by adsorption on fly ash-based geopolymer. J. Mater. Environ. Sci. 9, 32–46. doi:10.26872/jmes.2018.9.1.5
Ertugay, N., and Acer, F. N. (2017). Removal of COD and color from direct blue 71 azo dye wastewater by fenton’s oxidation: kinetic study. Arab. J. Chem. 10, S1158–S1163. doi:10.1016/j.arabjc.2013.02.009
Gebrati, L., Idrissi, L. L., Mountassir, Y., and Nejmeddine, A. (2010). Detoxification of textile industry effluents by photocatalytic treatment. Environ. Technol. 31, 625–632. doi:10.1080/09593331003592253
Gezer, B. (2018). Adsorption capacity for the removal of organic dye pollutants from wastewater using carbon powder. Int. J. Agric. For. 2, 1–14.
Guo, W. S., Shim, W. G., Vigneswaran, S., and Ngo, H. H. (2005). Effect of operating parameters in a submerged membrane adsorption hybrid system: experiments and mathematical modeling. J. Membr. Sci. 247, 65–74. doi:10.1016/j.memsci.2004.09.009
Hassaan, M. A., El Nemr, A., and Madkour, F. F. (2017). Testing the advanced oxidation processes on the degradation of Direct Blue 86 dye in wastewater. J. Aquat. Res. 43, 11–19. doi:10.1016/j.ejar.2016.09.006
Kadhom, M., Albayati, N., Alalwan, H., and Al-furaiji, M. (2020). Removal of dyes by agricultural waste. Sustain. Chem. Pharm. 16 (1), 100259. doi:10.1016/j.scp.2020.100259
Karisma, D., Febrianto, G., and Mangindaan, D. (2017). Removal of dye from textile wastewater by using nanofiltration polyetherimide membrane. Environ. Earth. Sci. 109, 1–7. doi:10.1088/1755-1315/109/1/012012
Kim, E. S., Liu, Y., and El-Din, M. G. (2012). Evaluation of membrane fouling for in-line filtration of oil sands process-affected water: the effects of pretreatment conditions. Environ. Sci. Technol. 46, 2877–2884. doi:10.1021/es203813s
Kim, J., Cai, Z., and Benjamin, M. M. (2008). Effects of adsorbents on membrane fouling by natural organic matter. J. Membr. Sci. 310, 356–364. doi:10.1016/j.memsci.2007.11.007
Lafi, R., Gzara, L., Lajimi, R. H., and Hafiane, A. (2018). Treatment of textile wastewater by a hybrid ultrafiltration/electrodialysis process. Chem. Eng. PROCESS Process Intensif. 132, 105–113. doi:10.1016/j.cep.2018.08.010
Lakdioui, T., and El Harfi, A. (2015). Elimination of indigo dyes existing in liquid discharges by ultrafiltration processes and adsorption. Moroc. J. Chem. 3, 441–448. doi:10.48317/IMIST.PRSM/morjchem-v3i3.2711
Lee, S. J., and Kim, J. H. (2014). Differential natural organic matter fouling of ceramic versus polymeric ultrafiltration membranes. Water Res. 48, 43–51. doi:10.1016/j.watres.2013.08.038
Lin, C. F., Huang, Y. J., and Hao, O. J. (1999). Ultrafiltration processes for removing humic substances: effect of molecular weight fractions and PAC treatment. Water Res. 33, 1252–1264. doi:10.1016/s0043-1354(98)00322-4
Mashkoor, S., and Nasar, A. (2018). Adsorptive decontamination of synthetic wastewater containing crystal violet dye by employing Terminalia arjuna sawdust waste. Groundw. Sustain. Dev. 7, 30–38. doi:10.1016/j.gsd.2018.03.004
Masmoudi, G., Ellouz, E., and Amar, R. B. (2015). Hybrid coagulation/membrane process treatment applied to the treatment of industrial dyeing effluent. Desalination water Treat. 57, 6781–6791. doi:10.1080/19443994.2015.1012747
Monnot, M., Laborie, S., and Cabassud, C. (2016). Granular activated carbon filtration plus ultrafiltration as a pretreatment to seawater desalination lines: impact on water quality and UF fouling. Desalination 383, 1–11. doi:10.1016/j.desal.2015.12.010
Mourid, E., Lakraimi, M., El Khattabi, E., Benaziz, L., and Berraho, M. (2017). Removal of textile dye acid green 1 from wastewater by activated carbon. J. Mater. Environ. Sci. 8, 3121–3130.
Mzahma, S., Duplay, J., Souguir, D., Amar, R. B., Ghazi, M., and Mohamed, H. (2023). Membrane processes treatment and possibility of agriculture reuse of textile effluents: study case in Tunisia. Water 15 (7), 1430. doi:10.3390/w15071430
Naddeo, V., Belgiorno, V., Borea, L., Secondes, M. F. N., and Ballesteros, F. J. (2015). Control of fouling formation in membrane ultrafiltration by ultrasound irradiation. Environ. Technol. 36, 1299–1307. doi:10.1080/09593330.2014.985731
Nouri Alavijeh, H., Sadeghi, M., Rajaeieh, M., Moheb, A., Sadani, M., and Ismail, A. F. (2017). Integrated ultrafiltration membranes and chemical coagulation for treatment of baker’s yeast wastewater. J. Membr. Sci. Technol. 7, 1–9. doi:10.4172/2155-9589.1000173
Özgün, H., Sakar, H., Agtaş, M., and Koyuncu, I. (2022). Investigation of pre-treatment techniques to improve membrane performance in real textile wastewater treatment. Int. J. Environ. Sci. Technol. 20, 1539–1550. doi:10.1007/s13762-022-04034-w
Panão, E. L. S., Campos, H. H. C., LimaRinaldi, A. W. M. K., Lima-Tenório, E. T., Tenório-Neto, M. R., Guilherme, T., et al. (2019). Ultra-absorbent hybrid hydrogel based on alginate and SiO2 microspheres: a high-water-content system for removal of methylene blue. J. Mol. Liq. 276, 204–213. doi:10.1016/j.molliq.2018.11.157
Pathania, D., Gupta, D., Al-Muhtaseb, H., Sharma, G., Kumar, A., Naushad, M., et al. (2016). Photocatalytic degradation of highly toxic dyes using chitosan–g–poly(acrylamide)/ZnS in presence of solar irradiation. J. Photochem. Photobiol. A. Chem. 329, 61–68. doi:10.1016/j.jphotochem.2016.06.019
Said, M., Ahmad, A., Mohammad, A. W., Mohd Nor, M. T., and Sheikh Abdullah, S. R. (2015). Blocking mechanism of PES membrane during ultrafiltration of POME. J. Ind. Eng. Chem. 21, 182–188. doi:10.1016/j.jiec.2014.02.023
Sarioglu Cebeci, M., and Torun, T. B. (2017). Treatment of textile wastewater using nanofiltration. Eur. Sci. J., 169–175.
Sarkar, S., Ghish, S., Banejee, P., Larbot, A., Cerneaux, S., Bandyopadhyay, S., et al. (2014). Preparation and characterization of single layer ultra filtration alumina membrane directly over porous clay-alumina tubular and capillary support for textile effluent treatment. Trans. Indian Ceram. Soc. 73, 197–204. doi:10.1080/0371750x.2014.882246
Seyhi, B., Droguia, P., Buelna, G., and Blais, J. F. (2012). Removal of bisphenol-A from spiked synthetic effluents using an immersed membrane activated sludge process. J. Sep. Purif. Technol. 87, 1 0 1–109. doi:10.1016/j.seppur.2011.11.029
Shakoor, F., Nasar, A., Inamuddin, , and Asiri, A. M. (2018). Exploring the reusability of synthetically contaminated wastewater containing crystal violet dye using tectona grandis sawdust as a very low-cost adsorbent. Sci. Rep. 8, 8314. doi:10.1038/s41598-018-26655-3
Shakoor, S., and Nasar, A. (2016). Removal of methylene blue dye from artificially contaminated water using citrus limetta peel waste as a very low cost adsorbent. J. Taiwan Inst. Chem. Eng. 66, 154–163. doi:10.1016/j.jtice.2016.06.009
Shakoor, S., and Nasar, A. (2017). Adsorptive treatment of hazardous methylene blue dye from artificially contaminated water using cucumis sativus peel waste as a low-cost adsorbent. Groundw. Sustain. Dev. 5, 152–159. doi:10.1016/j.gsd.2017.06.005
Shakoor, S., and Nasar, A. (2018). Utilization of Punica granatum peel as an eco-friendly biosorbent for the removal of methylene blue dye from aqueous solution. J. Appl. Biotechnol. Bioeng. 5, 242–249. doi:10.15406/jabb.2018.05.00145
Shon, H. K., Kim, S. H., Vigneswaran, S., Ben Aim, R., Lee, S., and Cho, J. (2009). Physicochemical pretreatment of seawater fouling reduction and membrane characterization. Desalination 238, 10–21. doi:10.1016/j.desal.2008.01.030
Song, Y., Peng, R., Chen, S., and Xiong, Y. (2019). Adsorption of crystal violet onto epichlorohydrin modified corncob. Desalin. WATER Treat. 154, 376–384. doi:10.5004/dwt.2019.24067
Stoller, M. (2009). On the effect of flocculation as pretreatment process and particle size distribution for membrane fouling reduction. Desalination 240, 209–217. doi:10.1016/j.desal.2007.12.042
Tansakul, C., Laborie, S., and Cabassud, C. (2011). Adsorption combined with ultrafiltration to remove organic matter from seawater. Wat Res. 45, 6362–6370. doi:10.1016/j.watres.2011.09.024
Tian, J. Y., Wu, C., Yu, H., Gao, S., Li, G., Cui, F., et al. (2018). Applying ultraviolet/persulfate (UV/PS) pre-oxidation for controlling ultrafiltration membrane fouling by natural organic matter (NOM) in surface water. Water Res. 132, 190–199. doi:10.1016/j.watres.2018.01.005
Tripti, B. G., and Dilip, H. L. (2018). Adsorption of indigo carmine and methylene blue dye: taguchi’s design of experiment to optimize removal efficiency. Indian Acad. Sci. 43, 170–182. doi:10.1007/s12046-018-0931-x
Uyanık, I., Özkan, O., and Koyuncu, I. (2017). NF-RO membrane performance for treating the effiuent of an organized industrial zone wastewater treatment plant: effect of different UF types. Water 9, 1–10. doi:10.3390/w9070506
Yanan, D., Yanlei, S., Wenjuan, C., Jinming, P., Yan, Z., and Zhongyi, J. (2011). Ultrafiltration enhanced with activated carbon adsorption for efficient dye removal from aqueous solution. Chin. J. Chem. Eng. 19, 863–869. doi:10.1016/s1004-9541(11)60066-9
Yin, H., Qiu, P., Qian, Y., kong, Z., Zheng, X., tang, Z., et al. (2019). Textile wastewater treatment for water reuse: a case study. Processes 7, 34–21. doi:10.3390/pr7010034
Yu, W. Z., Liu, T., Crawshaw, J., Liu, T., and Graham, N. (2018). Ultrafiltration and nanofiltration membrane fouling by natural organic matter: mechanisms and mitigation by pre-ozonation and pH. Water Res. 139, 353–362. doi:10.1016/j.watres.2018.04.025
Yu, W. Z., Zhang, D. Z., and Graham, N. J. D. (2017). Membrane fouling by extracellular polymeric substances after ozone pre-treatment: variation of nano-particles size. Water Res. 120, 146–155. doi:10.1016/j.watres.2017.04.080
Zheng, L., Su, Y., Wang, J., and Jiang, Z. (2009). Adsorption and recovery of methylene blue from aqueous solution through ultrafiltration technique. Sep. Purif. Technol. 68, 244–249. doi:10.1016/j.seppur.2009.05.010
Keywords: indigo blue, adsorption, natural clay, ultrafiltration, nanofiltration, hybrid process
Citation: Ben Salah S, Missaoui M, Attia A, Lesage G, Heran M and Ben Amar R (2024) Treatment of real textile effluent containing indigo blue dye by hybrid system combining adsorption and membrane processes. Front. Membr. Sci. Technol. 3:1348992. doi: 10.3389/frmst.2024.1348992
Received: 03 December 2023; Accepted: 29 February 2024;
Published: 18 March 2024.
Edited by:
Maria Giovanna Buonomenna, Ordine dei Chimici e Fisici Della Campania and MIUR, ItalyReviewed by:
Atikah Mohd Nasir, Universiti Kebangsaan Malaysia, MalaysiaNurasyikin Misdan, Universiti Tun Hussein Onn Malaysia, Malaysia
Copyright © 2024 Ben Salah, Missaoui, Attia, Lesage, Heran and Ben Amar. This is an open-access article distributed under the terms of the Creative Commons Attribution License (CC BY). The use, distribution or reproduction in other forums is permitted, provided the original author(s) and the copyright owner(s) are credited and that the original publication in this journal is cited, in accordance with accepted academic practice. No use, distribution or reproduction is permitted which does not comply with these terms.
*Correspondence: Raja Ben Amar, YmVuYW1hci5yYWphQHlhaG9vLmNvbQ==