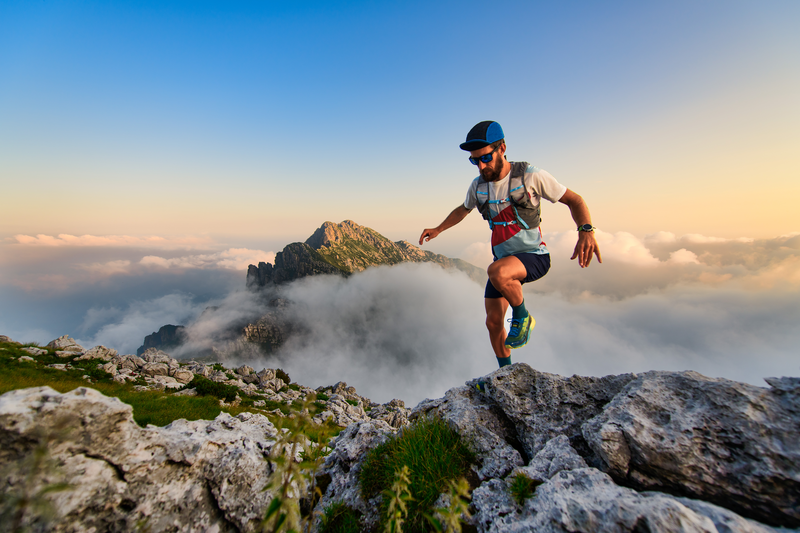
94% of researchers rate our articles as excellent or good
Learn more about the work of our research integrity team to safeguard the quality of each article we publish.
Find out more
ORIGINAL RESEARCH article
Front. Med.
Sec. Ophthalmology
Volume 12 - 2025 | doi: 10.3389/fmed.2025.1568787
This article is part of the Research Topic Imaging in the Diagnosis and Treatment of Eye Diseases View all 17 articles
The final, formatted version of the article will be published soon.
You have multiple emails registered with Frontiers:
Please enter your email address:
If you already have an account, please login
You don't have a Frontiers account ? You can register here
Purpose: This study aims to investigate the influence of dendritic cells (DCs) on corneal nerve morphology and the clinical significance in chronic Femtosecond Laser-Assisted Laser in Situ Keratomileusis (FS-LASIK) related dry eye disease (DED).: The cross-sectional study was conducted involving healthy control, DED without FS-LASIK group, and DED after FS-LASIK group. Clinical parameters such as ocular surface disease index (OSDI), fluorescein tear breakup time (FBUT), corneal fluorescein staining (CFS) scores, Schirmer I test (SⅠt), Cochet-Bonnet esthesiometer (C-BE) were recorded. DCs of in vivo confocal microscopy images were included or excluded during corneal nerve segmentation. Key morphological parameters, including corneal nerve fiber density (CNFD), corneal nerve branch density (CNBD), tortuosity, and box-count fractal dimension (Boxdim), were measured. The impact of DCs on nerve metrics and clinical parameters and the correlations between each other were assessed. Results: The significant reduce in key morphological parameters was observed after eliminating DCs. Significant differences of morphological parameters were observed in DED after FS-LASIK group compared with other two groups. With the increased presence of DCs density in DED especially in DED after FS-LASIK group, the presence of DCs introduced false positives in the correlation analysis of DCs density with corneal morphology in DED after FS-LASIK and in the correlation analysis of corneal morphology with clinical characteristics in DED without FS-LASIK. Conclusions: The presence of DCs introduces significant biases in the assessments of corneal nerve morphology, primarily false-positive results in DED especially chronic FS-LASIK related DED. Their exclusion improves the precision of nerve measurements, which may enhance the clinical evaluation of corneal nerve morphology. These findings highlight the importance of precise segmentation techniques to minimize DCs related interference in clinical practice.
Keywords: Dendritic Cells, Corneal nerve, morphology, FS-LASIK, Dry eye disease
Received: 30 Jan 2025; Accepted: 03 Mar 2025.
Copyright: © 2025 Liu, Ma, Zhao, Li, Li, Sun, Duan, Zhao and Qi. This is an open-access article distributed under the terms of the Creative Commons Attribution License (CC BY). The use, distribution or reproduction in other forums is permitted, provided the original author(s) or licensor are credited and that the original publication in this journal is cited, in accordance with accepted academic practice. No use, distribution or reproduction is permitted which does not comply with these terms.
* Correspondence:
Yitian Zhao, Cixi Institute of BioMedical Engineering, Ningbo Institute of Materials Technology and Engineering, Chinese Academy of Sciences, Cixi, China
Hong Qi, Department of Ophthalmology, Peking University Third Hospital, Beijing, China
Disclaimer: All claims expressed in this article are solely those of the authors and do not necessarily represent those of their affiliated organizations, or those of the publisher, the editors and the reviewers. Any product that may be evaluated in this article or claim that may be made by its manufacturer is not guaranteed or endorsed by the publisher.
Research integrity at Frontiers
Learn more about the work of our research integrity team to safeguard the quality of each article we publish.