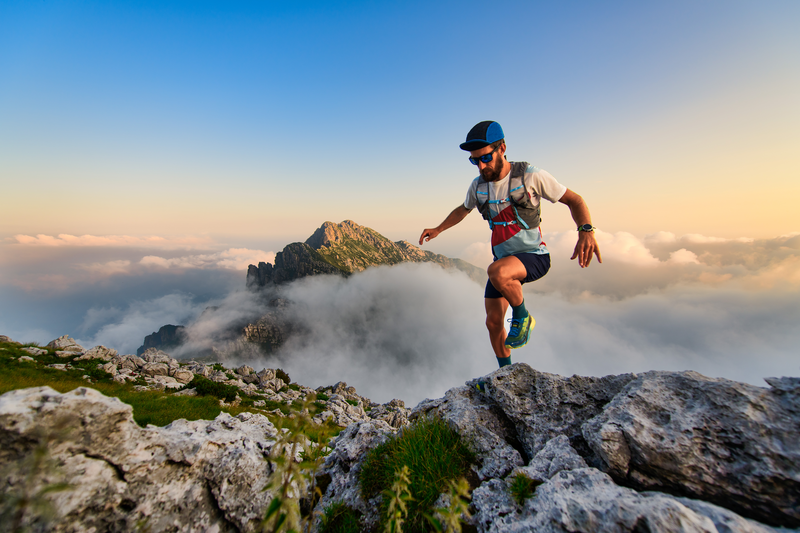
94% of researchers rate our articles as excellent or good
Learn more about the work of our research integrity team to safeguard the quality of each article we publish.
Find out more
ORIGINAL RESEARCH article
Front. Med.
Sec. Intensive Care Medicine and Anesthesiology
Volume 12 - 2025 | doi: 10.3389/fmed.2025.1561017
The final, formatted version of the article will be published soon.
You have multiple emails registered with Frontiers:
Please enter your email address:
If you already have an account, please login
You don't have a Frontiers account ? You can register here
Background: During pressure support ventilation (PSV), the accuracy of non-invasive indicators in diagnosing high or low inspiratory effort has been validated. However, the correlation and agreement of these indicators remain unclear. This study aims to investigate the correlation and agreement among non-invasive inspiratory effort indicators, and to compare characteristics of inspiratory effort in neurocritical and non-neurocritical patients.This was a single-centre prospective observational study. We collected three noninvasive inspiratory effort indicators, pressure muscular index (PMI), the maximal negative swing of airway pressure during expiratory occlusion (ΔPocc), and the airway occlusion pressure during the first 100ms (P0.1). Cutoff values for these indicators derived from esophageal pressure-time product (PTPmus) were chosen for this study. The correlation and agreement of these indicators were analysed using Spearman's rank correlation test and linear weighted Kappa analysis. Characteristics of PSV settings and inspiratory effort in neurocritical and non-neurocritical patients were compared.Results: Ninety-seven patients were enrolled in this study. Correlation analysis showed a moderate correlation between PMI and ΔPocc (rho = -0.524, p < 0.001), ΔPocc and P0.1 (rho = 0.588, p < 0.001), while no correlation between PMI and P0.1 (rho = -0.140, p = 0.172).There was a moderate agreement between ΔPocc and P0.1 (k = 0.459, p < 0.001), a fair agreement between PMI and ΔPocc (k = 0.362, p < 0.001), but no agreement between PMI and P0.1 (k = 0.134, p = 0.072). The correlation of these indicators was similar in neurocritical patients compared with non-neurocritical patients, but agreement was poor.The study showed that PMI and ΔPocc had moderate correlation and fair agreement, ΔPocc and P0.1 had moderate correlation and agreement, while PMI and P0.1 had no correlation and agreement.
Keywords: mechanical ventilation, Pressure support ventilation, Inspiratory effort, PMI, ΔPocc, P0.1
Received: 15 Jan 2025; Accepted: 20 Feb 2025.
Copyright: © 2025 Lv, Shuai, Zhang and Zhou. This is an open-access article distributed under the terms of the Creative Commons Attribution License (CC BY). The use, distribution or reproduction in other forums is permitted, provided the original author(s) or licensor are credited and that the original publication in this journal is cited, in accordance with accepted academic practice. No use, distribution or reproduction is permitted which does not comply with these terms.
* Correspondence:
Linlin Zhang, Beijing Tiantan Hospital, Capital Medical University, Beijing, China
Jian-Xin Zhou, Beijing Shijitan Hospital, Capital Medical University, Beijing, Beijing Municipality, China
Disclaimer: All claims expressed in this article are solely those of the authors and do not necessarily represent those of their affiliated organizations, or those of the publisher, the editors and the reviewers. Any product that may be evaluated in this article or claim that may be made by its manufacturer is not guaranteed or endorsed by the publisher.
Research integrity at Frontiers
Learn more about the work of our research integrity team to safeguard the quality of each article we publish.