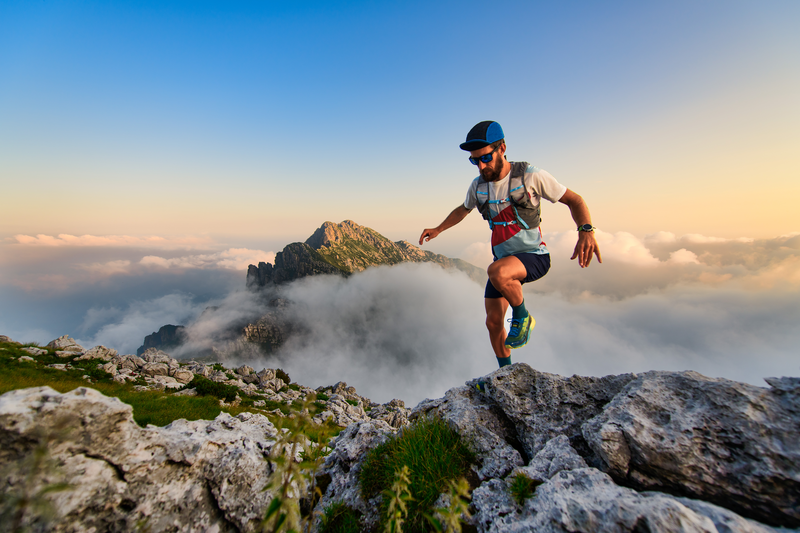
94% of researchers rate our articles as excellent or good
Learn more about the work of our research integrity team to safeguard the quality of each article we publish.
Find out more
ORIGINAL RESEARCH article
Front. Med.
Sec. Infectious Diseases: Pathogenesis and Therapy
Volume 12 - 2025 | doi: 10.3389/fmed.2025.1556623
This article is part of the Research Topic SARS-CoV-2 Vaccines Beyond the Pandemic Era View all 24 articles
The final, formatted version of the article will be published soon.
You have multiple emails registered with Frontiers:
Please enter your email address:
If you already have an account, please login
You don't have a Frontiers account ? You can register here
Post-COVID syndrome, also known as long COVID, has emerged as a major public health concern, affecting a substantial proportion of individuals recovering from SARS-CoV-2 infection. This condition is characterized by persistent symptoms lasting at least two months after acute infection, significantly impacting quality of life and increasing healthcare burdens. In Kazakhstan, the recognition of post-COVID syndrome in national clinical protocols highlights the need for effective prevention and management strategies. Vaccination has been suggested as a key intervention to reduce the severity and prevalence of long COVID symptoms, yet data on its effectiveness, particularly for the domestic QazVac vaccine, remain limited. The aim of this study is to investigate the impact of vaccination with the domestic QazVac vaccine on the features of humoral and cellular immunity in patients with post-COVID conditions and to identify the leading clinical variants of the course. We analyzed data from 90 vaccinated and 217 non-vaccinated patients, examining sex, age, smoking status, BMI, comorbidities, and clinical manifestations. There were no significant differences between the groups with regard to sex, age, and smoking status. However, the characteristics of the subjects indicated that vaccination was correlated with a lower prevalence of diabetes mellitus (2.2% vs. 11.1%, P = 0.011) and cardiovascular diseases (0.0% vs. 10.1%, P = 0.047), and a higher prevalence of hypertension among non-vaccinated subjects. With regard to clinical symptoms, vaccinated individuals presented a significantly decreased frequency of neurological (51.1% vs. 74.2%, P = 0.001), gastrointestinal (4.4% vs. 15.2%, P = 0.008), respiratory (21.1% vs. 36.4%, P = 0.009), rheumatological symptoms (26.7% vs. 38.7%, P = 0.044), and kidney symptoms (2.2% vs. 9.7%, P = 0.024). In contrast, unvaccinated participants had more memory loss (49.8% vs. 22.2%, P < 0.001), depression (31.3% vs. 6.7%, P < 0.001), joint pain (33.2% vs. 14.4%, P = 0.001), and other psychopathological symptoms. A sharp decrease in the frequency of neurological, gastrointestinal, respiratory, and rheumatological symptoms was recorded in vaccinated patients, advocating for the protective role of vaccination against long COVID-19 sequelae. These findings highlight the potential for vaccination to mitigate the burden of post-COVID complications across various organ systems.
Keywords: Post-COVID-19 Condition, Immunoglobulin g (IgG), Flow Cytometry, QazVac vaccine, Cellular Immune Response
Received: 07 Jan 2025; Accepted: 12 Mar 2025.
Copyright: © 2025 Madenbayeva, Kurmangaliyeva, Urazayeva, Kurmangaliyev, Bazargaliyev and Kudabayeva. This is an open-access article distributed under the terms of the Creative Commons Attribution License (CC BY). The use, distribution or reproduction in other forums is permitted, provided the original author(s) or licensor are credited and that the original publication in this journal is cited, in accordance with accepted academic practice. No use, distribution or reproduction is permitted which does not comply with these terms.
* Correspondence:
Akzhan M. Madenbayeva, West Kazakhstan Marat Ospanov State Medical University, Aktobe, Kazakhstan
Saulesh S Kurmangaliyeva, West Kazakhstan Marat Ospanov State Medical University, Aktobe, Kazakhstan
Disclaimer: All claims expressed in this article are solely those of the authors and do not necessarily represent those of their affiliated organizations, or those of the publisher, the editors and the reviewers. Any product that may be evaluated in this article or claim that may be made by its manufacturer is not guaranteed or endorsed by the publisher.
Research integrity at Frontiers
Learn more about the work of our research integrity team to safeguard the quality of each article we publish.