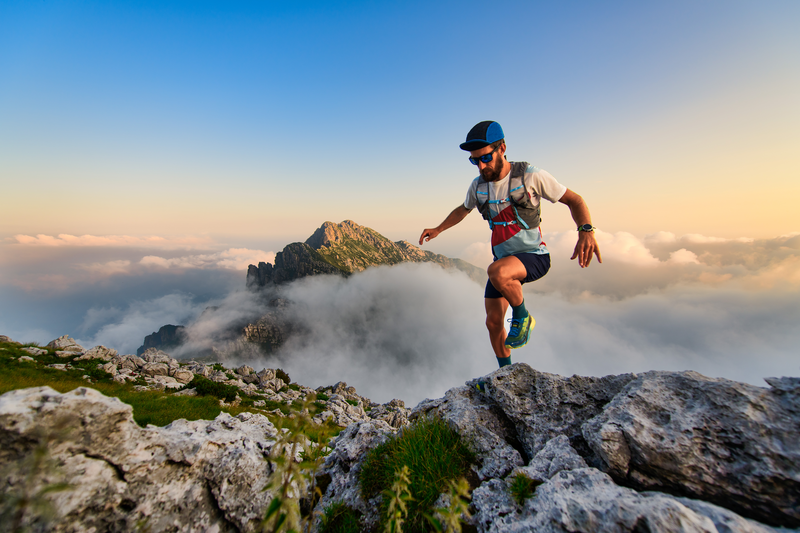
95% of researchers rate our articles as excellent or good
Learn more about the work of our research integrity team to safeguard the quality of each article we publish.
Find out more
REVIEW article
Front. Med.
Sec. Dermatology
Volume 12 - 2025 | doi: 10.3389/fmed.2025.1554669
This article is part of the Research Topic Advancing Atopic Dermatitis Management: Translational Research and Emerging Therapies View all 4 articles
The final, formatted version of the article will be published soon.
You have multiple emails registered with Frontiers:
Please enter your email address:
If you already have an account, please login
You don't have a Frontiers account ? You can register here
Atopic dermatitis is a chronic inflammatory skin condition with a high prevalence worldwide and multifaceted pathogenesis. In general, conventional treatments for moderate to severe AD often led to relapses. Therefore, to understand the possible factors of chronic relapse of AD and to look for biological markers that predict the relapse or poor prognosis of AD will be helpful for clinical treatment. New insights into the intractability of genetic factors (mutations in FLG, SPINK5, STAT, KIF3A, claudin-1, Ovol1, and HLA-DRB1) for AD patients. Routine factors can guide the improvement of patients' lifestyle, the importance of environmental factors (including psychological environment), and clinicians' grasp of anti-infection treatment. The inflammatory axis (CD30-CD30L axis, IL-9-IL-18 axis) provides new insights into the inflammatory pathways of AD and may be a target for future therapies. Low NKG2D expression may have adverse effects on prognosis. Prognostic biomarkers can play an important role in treatment monitoring, disease progression and recurrence, and provide the possibility for more personalized treatment.
Keywords: atopic dermatitis, STAT (signal transducer and activator of transcription), Axis, NKG2D, ceramide profiles
Received: 02 Jan 2025; Accepted: 31 Mar 2025.
Copyright: © 2025 Liu, Guo, Li and Xu. This is an open-access article distributed under the terms of the Creative Commons Attribution License (CC BY). The use, distribution or reproduction in other forums is permitted, provided the original author(s) or licensor are credited and that the original publication in this journal is cited, in accordance with accepted academic practice. No use, distribution or reproduction is permitted which does not comply with these terms.
* Correspondence:
Hui Xu, Department of Dermatology, Affiliated Hospital of Jiangsu University, Zhenjiang, China
Disclaimer: All claims expressed in this article are solely those of the authors and do not necessarily represent those of their affiliated organizations, or those of the publisher, the editors and the reviewers. Any product that may be evaluated in this article or claim that may be made by its manufacturer is not guaranteed or endorsed by the publisher.
Research integrity at Frontiers
Learn more about the work of our research integrity team to safeguard the quality of each article we publish.