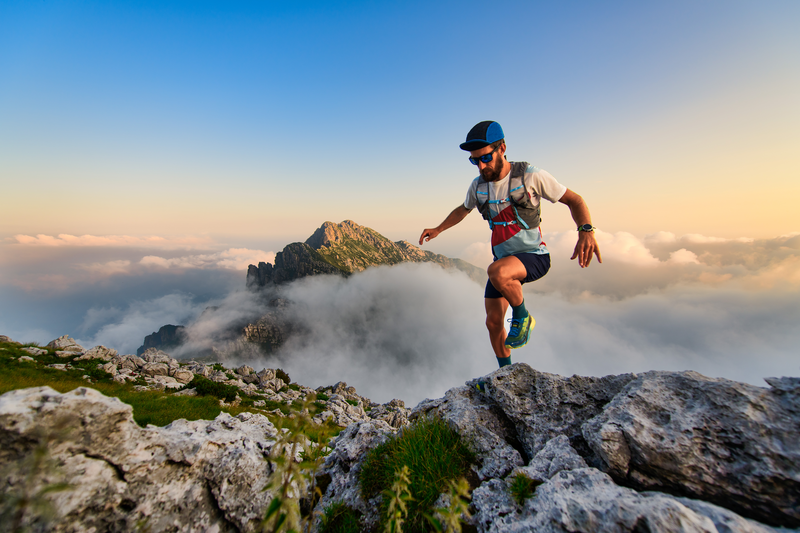
94% of researchers rate our articles as excellent or good
Learn more about the work of our research integrity team to safeguard the quality of each article we publish.
Find out more
ORIGINAL RESEARCH article
Front. Med.
Sec. Ophthalmology
Volume 12 - 2025 | doi: 10.3389/fmed.2025.1545099
This article is part of the Research Topic Myopia in Childhood and Adolescence View all 8 articles
The final, formatted version of the article will be published soon.
You have multiple emails registered with Frontiers:
Please enter your email address:
If you already have an account, please login
You don't have a Frontiers account ? You can register here
PURPOSE Several researches have revealed that choroidal thickness (ChT) and sclera/choroidal hypoxia have close relationship with myopia happen and development.Far infrared ray (FIR) is a traditional method to enhance microcirculation. In this study, we estimated the effect of FIR for myopia control and explored underlying mechanisms. Furthermore, we compared the efficacy of far-infrared ray from two different sources in myopia control. METHODS Guinea pigs were divided into three groups and all of them underwent minus lens induction for four weeks. Two groups received simultaneous far-infrared ray intervention, from FIR radiator (FIRR) lamp and innovative FIR material (FIRM).Refraction, axial length (AL), ChT, and hypoxia-labeled pimonidazole in the choroid and sclera were measured.Both FIRR and FIRM inhibited the increase of refraction and AL, and the decrease of ChT. They also attenuated the hypoxia of choroid and sclera. Compared with FIRR, FIRM had better effect on myopia control and hypoxia attenuation.However, the difference in AL didn't show significance.CONCLUSION FIR has satisfactory effect for myopia control and the innovative FIR material maybe start a revolution for myopia control in the near future.
Keywords: Myopia, Far-infrared radiation (FIR), hypoxia, Choroid, Sclera, Blood perfusion
Received: 14 Dec 2024; Accepted: 10 Mar 2025.
Copyright: © 2025 Xu, Liu, GuoJi, Zhou and Huang. This is an open-access article distributed under the terms of the Creative Commons Attribution License (CC BY). The use, distribution or reproduction in other forums is permitted, provided the original author(s) or licensor are credited and that the original publication in this journal is cited, in accordance with accepted academic practice. No use, distribution or reproduction is permitted which does not comply with these terms.
* Correspondence:
Yifei Huang, Senior Department of Ophthalmology, Chinese PLA General Hospital, Beijing, China
Disclaimer: All claims expressed in this article are solely those of the authors and do not necessarily represent those of their affiliated organizations, or those of the publisher, the editors and the reviewers. Any product that may be evaluated in this article or claim that may be made by its manufacturer is not guaranteed or endorsed by the publisher.
Research integrity at Frontiers
Learn more about the work of our research integrity team to safeguard the quality of each article we publish.