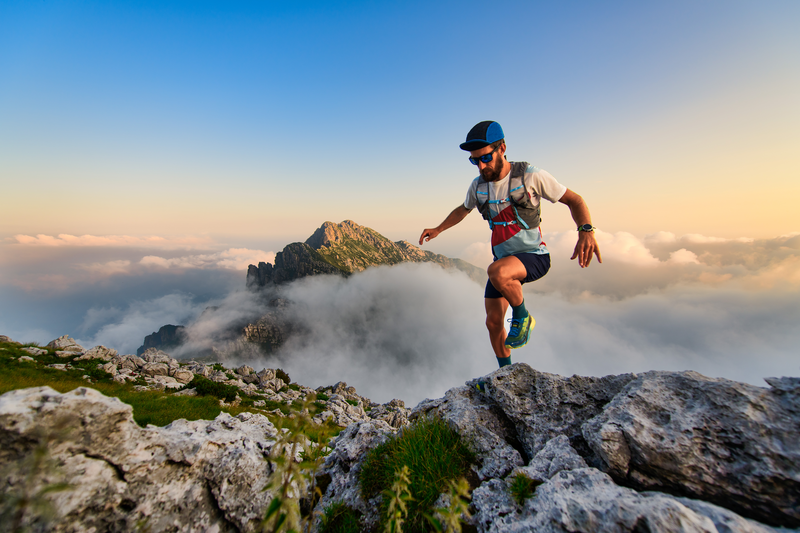
94% of researchers rate our articles as excellent or good
Learn more about the work of our research integrity team to safeguard the quality of each article we publish.
Find out more
MINI REVIEW article
Front. Med.
Sec. Pulmonary Medicine
Volume 12 - 2025 | doi: 10.3389/fmed.2025.1543033
The final, formatted version of the article will be published soon.
You have multiple emails registered with Frontiers:
Please enter your email address:
If you already have an account, please login
You don't have a Frontiers account ? You can register here
Lung cancer (LC) remains one of the leading causes of cancer-related mortality worldwide, emphasizing the urgent need for innovative diagnostic tools to improve early detection and patient outcomes. Contrast-enhanced ultrasound (CEUS) has emerged as a promising complement to conventional imaging modalities, offering distinct advantages such as real-time dynamic imaging, cost-effectiveness, and the absence of ionizing radiation. By enhancing the visualization of tumor vascularization, CEUS enables differentiation between benign and malignant pulmonary nodules while providing valuable insights into tumor angiogenesis, a hallmark of malignancy, and therapeutic response. Additionally, CEUS demonstrates utility in assessing regional lymph nodes, detecting distant metastases, and analyzing blood flow dynamics through quantitative methods such as time-intensity curve analysis. Despite these benefits, certain limitations persist, including reduced efficacy in imaging deep-seated lesions, variability due to patient-specific physiological factors, and dependency on operator expertise. However, advancements in targeted contrast agents, integration with multimodal imaging techniques, and the application of artificial intelligence hold significant potential to address these challenges. This review systematically evaluates the clinical applications, advantages, and limitations of CEUS in LC diagnosis, providing a comprehensive understanding of its role in modern precision oncology. Furthermore, it highlights future research directions aimed at enhancing diagnostic accuracy, improving clinical workflows, and expanding the adoption of CEUS in routine practice.
Keywords: lung cancer, contrast-enhanced ultrasound, diagnosis, Multimodal Imaging, Advance
Received: 10 Dec 2024; Accepted: 03 Mar 2025.
Copyright: © 2025 Huang, Zeng, Zhang, Liu and Feng. This is an open-access article distributed under the terms of the Creative Commons Attribution License (CC BY). The use, distribution or reproduction in other forums is permitted, provided the original author(s) or licensor are credited and that the original publication in this journal is cited, in accordance with accepted academic practice. No use, distribution or reproduction is permitted which does not comply with these terms.
* Correspondence:
Chong Feng, The Affiliated Hongqi Hospital of Mudanjiang Medical University, Mudanjiang, China
Disclaimer: All claims expressed in this article are solely those of the authors and do not necessarily represent those of their affiliated organizations, or those of the publisher, the editors and the reviewers. Any product that may be evaluated in this article or claim that may be made by its manufacturer is not guaranteed or endorsed by the publisher.
Research integrity at Frontiers
Learn more about the work of our research integrity team to safeguard the quality of each article we publish.