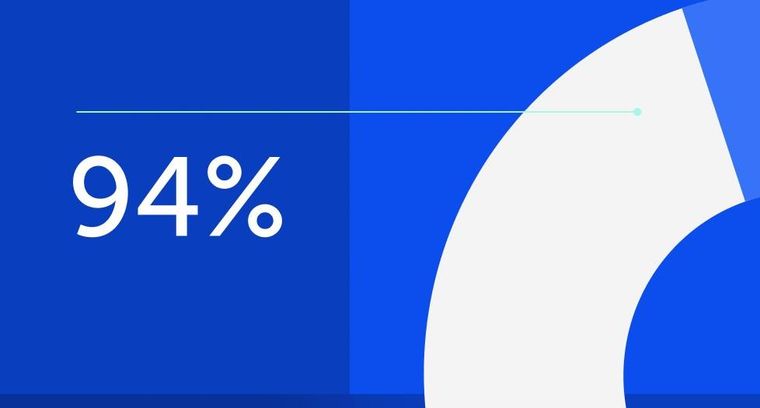
94% of researchers rate our articles as excellent or good
Learn more about the work of our research integrity team to safeguard the quality of each article we publish.
Find out more
REVIEW article
Front. Med., 24 February 2025
Sec. Pulmonary Medicine
Volume 12 - 2025 | https://doi.org/10.3389/fmed.2025.1542400
This article is part of the Research TopicPulmonary Involvement in Systemic Autoimmune Rheumatic Diseases (SARDs): from Diagnostic Tools to Therapeutic StrategiesView all 7 articles
Rheumatoid arthritis (RA) is a systemic autoimmune disease that affects millions of people worldwide and is characterized by persistent inflammation, pain, and joint destruction. In RA, the dysregulation of the immune system is well documented. However, the genetic basis of the disease is not fully understood, especially when extra-articular organs are involved. Interstitial lung disease (ILD) is a major cause of morbidity and mortality in patients with RA. Notably, RA-ILD shares several risk factors with idiopathic pulmonary fibrosis (IPF), namely male gender, smoking history, usual interstitial pneumonia (UIP) pattern of fibrosis, and association with the MUC5B rs35705950 polymorphism. In addition, other genetic susceptibilities are reported in RA-ILD for some HLA alleles and other less studied polymorphisms. However, the pathobiology of RA-ILD, particularly whether and to what extent genetic and environmental factors interact to determine the disease, remains elusive. In this review, we summarize and critically discuss the most recent literature on the genetics and pathogenesis of RA-ILD. The main clinical aspects of RA-ILD are also discussed.
Rheumatoid arthritis (RA) is a systemic autoimmune disorder of unknown etiology that primarily causes erosive symmetric polyarthritis (1–3). RA represents a significant global healthcare burden, with an estimated prevalence of about 0.5–1% worldwide (1–3); the disease is more common in females than in males (4). Intriguingly, the prevalence and incidence of RA have risen during the last century, and currently, the disease affects more than 17·6 million people worldwide (5). Of note, the prevalence of the disease appears to decrease from North to South and from urban to rural areas (6, 7). The mechanism of RA is highly complex, involving inflammatory and autoimmune processes, and the primary trigger is unknown (8).
Broadly, RA is characterized by an insidious clinical onset with nonspecific symptoms, such as fatigue and sometimes fever, associated with pain, stiffness, and swelling in multiple joints. In most cases, the disease is progressive and gradually worsens, leading to uncontrolled inflammation that damages joint structures, including cartilage and bone (9, 10). The diagnosis can be made based on clinical features; in addition, serological biomarkers can be useful, including rheumatoid factor (RF) (11), which can test positive in 80% of people with RA, and antibodies to cyclic citrullinated peptides (ACPA) which can be found in 60 to 70% of patients (12).
Extra-articular manifestations (EAMs) of the disease occur in nearly 50% of all RA patients, and many organs, such as the spleen, lungs, and nervous system, can be affected (13). Lung involvement is one of the most frequent EAMs (60–80% of cases) (14). RA can virtually affect any part of the respiratory tract, from the pleura to blood vessels, with interstitial lung disease (ILD) representing the most frequent manifestation and a major determinant of prognosis (15, 16). However, who will develop ILD and what are the underlying mechanisms of this association remain largely unknown. Furthermore, it has been speculated that several genetics and environmental factors concur for developing ILD (17). With this background, this review aims to discuss the impact of ILD in patients with RA, highlighting the most recent evidence regarding genetic risk factors.
About 10% of patients with RA exhibit respiratory symptoms related to ILD (18–20). Notably, interstitial features on computed tomography (CT) scans have been found in up to 67% of cases, and lung involvement can sometimes occur before any articular manifestations (18, 21). Notwithstanding, the development of ILD is most prevalent in the initial years following the diagnosis of RA, although it can arise at any time during the course of the disease (22). Rheumatologists play a pivotal role in identifying ILD in RA: early suspicion is crucial, and the initial evaluation should involve a thorough chest examination and spirometry (23). It is essential also to remember that patients with RA without specific risk factors or respiratory symptoms can still be at risk of developing ILD (24). A high-resolution CT scan (HRCT) is necessary to detect lung involvement, characterize the pattern, and monitor disease evolution. More invasive diagnostic procedures, such as bronchoscopy or lung biopsy, are generally unnecessary and are only performed if diagnostic uncertainty persists. The usual interstitial pneumonia (UIP) pattern is the most frequently observed finding in RA-ILD and is characterized by reticulation, traction bronchiectasis, and honeycombing. The nonspecific interstitial pneumonia (NSIP) pattern is less common, while other patterns, such as organizing pneumonia (OP), are also seen but less frequently (25–27) (Figure 1).
Figure 1. Axial CT scans illustrating the radiological presentation of patients with RA-ILD. (A,B) Usual interstitial pneumonia pattern in two male patients with rheumatoid arthritis. Honeycombing and traction bronchiectasis are visible in both patients. (C) Mild and migrant organizing pneumonia in female patients with rheumatoid arthritis. (D) Mild reticulation in an asymptomatic male patient with RA.
Although RA-ILD can manifest with dry cough and dyspnea, some patients remain asymptomatic for a long time. Chest imaging is mandatory in all patients with abnormal findings on physical examination, such as “velcro”-like crackles or digital clubbing (28).
In the last two decades, large-scale Genome-Wide Association Studies (GWAS) have revealed many common disease-associated variants in the context of different ILDs, especially in idiopathic pulmonary fibrosis (IPF), where the interest in genetic predisposition has steadily increased (29). Given the strong similarities with IPF, such as the presence of the UIP pattern, the same variants and polymorphisms have started to be investigated also in patients with RA-ILD (Table 1).
MUC5B encodes a pivotal protein called Mucin-5B. It is one of the five gel-forming mucins in the saliva, cervical mucus, and lung (30). The MUC5B gain-of-function single nucleotide polymorphism in rs35705950 was deeply studied and is the strongest and the most known genetic risk factor for developing IPF (31, 32). Shortly, the rs35705950 polymorphism is associated with a 1.6 increased expression of Mucin-5B in the normal lung, which may impede a correct alveolar repair. Despite being associated with a higher risk of pulmonary fibrosis and some initial methodological discussion, recent studies demonstrated that in IPF patients, the rs35705950 T allele is associated with more prolonged survival (33, 34). In the last few years, the role of rs35705950 MUC5B has been investigated in patients with RA-ILD. Notably, Juge and coworkers identified that the MUC5B promoter variant was associated with an increased risk of ILD among patients with RA, particularly those with evidence of usual interstitial pneumonia pattern (UIP pattern) on high-resolution computer tomography (35). In the following years, the same authors advanced a new risk score that can help physicians identify RA patients at risk of subclinical ILD (36). The authors found a prevalence of subclinical RA-ILD of 19.0 and 16.9% in the two study cohorts. They also confirmed the role of the MUC5B rs35705950 T allele as independent risk factors for RA-ILD among the male sex, older age at RA onset, and increased disease activity in the joints (36). However, in a study conducted on 683 Korean patients, Joo and coworkers found no association between rs35705950 and risk of RA-ILD (odd ratio (OR) 2.49, 95% confidence interval (CI) 0.64 to 9.69, p = 0.187), probably because of the low prevalence of the mutant T allele in Asia. Nonetheless, they showed a significant association between the MUC5B rs35705950 T allele and UIP pattern (OR 4.90, 95% CI 1.23 to 19.59, p = 0.024) but not in patients with RA without ILD (37). All these studies shed light on the fact that there is a strict connection between this particular polymorphism and the specific radiologic pattern (i.e., UIP). Beyond this speculation, many authors also wondered if MUC5B polymorphism and the UIP pattern could represent a common denominator between RA-ILD and IPF. More in detail, the main question is: are we speaking about RA-UIP or IPF with RA? In a recent study conducted by Leavy et al., the authors demonstrated a considerable causal effect of IPF on seropositive RA, likely indicating that some mechanism associated with the development of UIP can promote RA (38). However, the connection between RA-ILD and IPF is currently under investigation, and future large multicenter studies are needed to better understand the intricate and complex connection between these two diseases (39, 40).
Telomeres are described as the end part of chromosomes, and their role is to protect against DNA damage. In vertebrates, they consist of TTAGGG repeats (41). Consequently, the shortening of telomere length can lead to cell senescence and apoptosis in various ways, resulting in several immunological disorders and organ aging (42, 43). Telomere shortening has been deeply studied in IPF, and the presence of pathogenic variants in telomere-related genes, such as TERT, TERC, PARN, and RTEL1 genes, are strongly associated with the risk of developing pulmonary fibrosis (44–46). However, the interest in telomere disorders in other diseases, such as COVID-19 (47, 48), has increased worldwide, with no exception on RA (49) and RA-ILD (50). In a landmark study, Juge et al. reported increased TERT, RTEL1, PARN, and SFPTC rare variants in patients with RA-ILD when compared with 1,010 control individuals of European ancestry, with an odds ratio (OR) of 3.17 and a 95% confidence interval (CI) of 1.53–6.12 (p < 0.001) (50). More recently, the association between telomere length and RA-ILD among a group of Veterans (54 RA-ILD patients and 92 RA-non-ILD patients) in the United States was investigated (51). The prevalence of ILD was significantly higher in patients with short vs. normal-length telomeres (73.3% vs. 32.8%, p = 0.002), and short telomeres were independently associated with increased odds of prevalent ILD compared to normal-length telomeres. Similar results were reported 1 year later in a study showing that shortened telomere length also correlated with baseline disease severity among RA-ILD patients (52). Telomere lengths were also compared between different ILDs and matched healthy controls (53). Seven patients with the RA-UIP ILD pattern appeared to have lower values compared with stable IPF, CPFE (combined pulmonary fibrosis and emphysema), and healthy controls [T/S (telomere index) mean 0.74 (±0.15) vs. 0.87 (±0.07) vs. 2.26 (±0.36)] (53). Very importantly, beyond the risk of ILD, telomere length has also been proposed as a potential new biomarker for the development of RA without ILD. It has also been suggested that short telomere length could enhance disease progression, and studies examining telomere length in RA have found that telomere erosion seems to occur more rapidly in subjects with RA than in healthy controls and that telomere lengths are shorter in those with the RA-risk Human Leukocyte Antigen (HLA)-shared epitope genes (54, 55). All these studies suggest that telomere shortening might contribute to the pathogenesis both for RA and RA-ILD (56).
The Human Leukocyte Antigen (HLA) system is a complex of genes located in chromosome 6 (6p21.3). HLA is highly polymorphic, containing about 220 genes, many of which are pivotal for immune system coordination and the response against bacterial and viral infections (57). Class I contains the classic genes HLA-A, -B, and -C, while HLA class II comprises the HLA-DRB1, -DPB1, and -DQB1 genes. Some HLA-DR alleles are known to be linked with RA in different ethnic groups, while the DRB1 gene is known as the major genetic susceptibility locus for RA (58, 59). This was confirmed, above all, in patients who are positive for RF or ACPA (60, 61). In addition, some studies have stressed the association of extra-articular manifestations of RA with HLA-DR, including ILD. Of note, specific types of HLA (such as HLA-DRB1*16, DQB1*06, and DR2 serological group) were associated with a higher risk of ILD in RA. On the other hand, HLA-DRB1*04 (corrected P [Pc] = 0.0054, odds ratio [OR] 0.57), a shared epitope (SE) (p = 0.0055, OR 0.66), and DQB1*04 (Pc = 0.0036, OR 0.57) seems to be protective against interstitial lung involvement (62, 63).
As previously mentioned, with the advent of next-generation sequencing (NGS), new opportunities were opened for genetics. In the specific context of RA-ILD, an interesting study was conducted by Jonsson et al. in 2022. In this project, 1,118 early RA patients were consecutively evaluated and followed prospectively; 60 (5.6%) had pulmonary fibrosis at the time of diagnosis or developed it during the disease course. 571,151 genome-wide single-nucleotide polymorphisms (SNPs) were ultimately analyzed. Four SNPs were associated with pulmonary fibrosis in RA: beyond the well-known MUC5B polymorphism rs35705950 and the TERT polymorphisms rs2736100, TOLLIP (rs111521887) and FAM13A (rs2609255) were also associated with a higher risk of ILD. In addition, older age and RF positivity were associated with an increased risk of ILD (64). The TOLLIP contributes to the toll-like receptors (TLR) pathways and is important for the endo-lysosome/autophagosome pathway in response to infections. The TOLLIP polymorphism has been deeply investigated in the pathogenesis and prognosis of idiopathic pulmonary fibrosis (IPF) and has been reported in small cohorts with conflicting results to be associated with treatment response (65–68). In the same way, the FAM13A variants have been associated with susceptibility to chronic lung diseases, also in IPF. In a study conducted by Hirano C. and coworkers, IPF patients who carry the T allele of the FAM13A polymorphism rs2609255 showed a significantly increasing mortality compared to the non-carriers (69).
The multitude of these studies suggests a strong connection between RA-ILD pathogenesis and IPF. Despite this speculation, some genetic associations may vary based on different ethnicities and must be evaluated carefully. For example, Shirai Y. et al. identified a novel RA-ILD risk locus at 7p21 that satisfied the genome-wide significance threshold (rs12702634 at RPA3-UMAD1, OR = 2.04, 95% CI 1.59 to 2.60, p = 1.5 × 10–8). The authors also found that the RA-ILD risk of the identified variant at RPA3-UMAD1 was relatively high, specifically in patients with probable and definite UIP patterns (70). RPA3 is a part of the heterotrimeric replication protein A complex (RPA), which plays an essential role in DNA replication and the cellular response to DNA damage, with a potential role in telomere maintenance. While this association seems supported in the Japanese population, the results found by Juge in 2024 were not in the same direction (71). In 883 European patients, the MUC5B rs35705950 was strongly associated with RA-ILD in all datasets, while no association between RPA3-UMAD1 rs12702634 and RA-ILD was observed. Afterward, a Japanese study conducted by Higuchi and coworkers did not confirm the association between RPA3-UMAD1 rs12702634 and RA-ILD, as previously documented by Higuchi et al. (72). The authors speculated that maybe both ethnicity and radiological pattern play a role in the development of the disease that seems extremely heterogeneous. However, further multicenter and international studies are needed to understand this complex connection between genes and ethnicity.
Another gene associated with RA-ILD is PPFIBP2. PPFIBP2 is located on chromosome 11 in the intronic region of the gene encoding tyrosine phosphatase receptor type F polypeptide-interacting protein binding protein 2. PPFIBP2 polymorphism rs6578890 seems to have the strongest association with ILD occurrence in 306 RA patients (73, 74).
In another study, the differential gene expression analysis revealed nine significantly upregulated genes in RA-ILD compared to RA without ILD: arginase 1 (ARG1), thymidylate synthetase (TYMS), sortilin 1 (SORT1), a marker of proliferation Ki-67 (MKI67), olfactomedin 4 (OLFM4), a baculoviral inhibitor of apoptosis repeat containing 5 (BIRC5), membrane-spanning 4-domains A4A (MS4A4A), C-type lectin domain family 12 member A (CLEC12A), and the long intergenic non-protein coding RNA (LINC02967). Interestingly, all these genes that are upregulated in RA-ILD vs. RA without ILD in this study are known to be involved in the pathogenesis of fibrosis. The authors also found that lower forced vital capacity (FVC) and DLCO and their decline over 6 months are associated with greater severity of RA-ILD (75). However, only a small number of patients were analyzed (n = 12), and the results presented can be managed only as a fundamental proof-of-concept.
Finally, also the polymorphisms rs11203366, rs11203367, rs874881 in PADI4, and rs1005753 in PADI2 are associated with developing ILD in patients with RA. Furthermore, the SNPs rs1748033 in PADI4, rs2057094, and rs2076615 in PADI2 were associated with RA development but not with ILD as well as haplotype in PADI4 ACTC in patients with RA-ILD. Intriguingly, the levels of PAD4 protein are increased in ILD patients (76, 77).
The clinical presentation of RA-ILD is highly variable, thus, new biomarkers and genetic variations that can predict the disease course are urgently needed. Of interest, some patients with RA-ILD develop progressive pulmonary fibrosis (PPF), characterized by an increasing worsening of the respiratory condition and consequently a higher risk of mortality (78–82). Although the development of ILD may have a substantial impact on RA prognosis, there is insufficient data for solid evidence-based guidelines. However, it is well known that RA-ILD is associated with a significant increase in mortality (83). In a US study of 582 patients, the risk of death over the follow-up period was almost 3-fold greater in patients with RA-ILD than in patients with RA alone (hazard ratio 2.86 [95% confidence interval (95% CI) 1.98–4.12]) (84). The same results were found in a prospective population-based study of 679 patients with RA-ILD and 11,722 matched patients with RA and no ILD. The 10-year mortality was 60.1% (95% CI 52.9–66.5) in the patients with RA-ILD, compared to 34.5% (95% CI 32.8–36.1) in the patients with RA and no ILD (22).
To facilitate early detection of RA-ILD, a Delphi-based consensus statement was recently proposed by a multidisciplinary team of pneumonologists, rheumatologists, and radiologists. The panel did not recommend screening all newly diagnosed RA patients. However, they strongly recommended the careful evaluation of patients with risk factors for RA-ILD, even in the absence of respiratory symptoms. Target risk factors are a history of smoking (especially more than 25 pack-years), male sex, older age, high disease activity (e.g., by a Clinical Disease Activity Index [CDAI] score > 10), and a family history of RA-ILD (85) (Figure 2). Additionally, the polymorphism of MUC5B was proposed as a new criterion. However, the predictive value of this risk score still needs validation in more extensive studies (86).
Figure 2. Risk factors associated with the development of ILD in patients with RA. GERD, gastroesophageal reflux. This picture summarizes the most frequent risk factors associated with the development of interstitial lung disease in patients with RA. This image was created by Biorender.com [Bernardinello (2025), https://BioRender.com/q67s977].
The occurrence of ILD in patients with RA is a complex and poorly understood process driven by different interacting factors, including genes and environment. However, many genes of varying effect size are likely to contribute to the predisposition to RA-ILD, although most of the reported associations await confirmation in larger studies. The identification of genetic determinants of ILD in RA is a pivotal step that would allow more precise risk stratification, while a deeper understanding of the disease pathobiology would facilitate the development of target therapies.
NB: Conceptualization, Writing – original draft, Writing – review & editing, Data curation, Investigation. MZ: Conceptualization, Investigation, Writing – review & editing. GC: Investigation, Writing – review & editing, Conceptualization. EC: Conceptualization, Writing – review & editing. EB: Investigation, Writing – review & editing. RB: Investigation, Writing – review & editing, Data curation. PS: Conceptualization, Writing – review & editing.
The author(s) declare that no financial support was received for the research, authorship, and/or publication of this article.
The authors declare that the research was conducted in the absence of any commercial or financial relationships that could be construed as a potential conflict of interest.
The author(s) declared that they were an editorial board member of Frontiers, at the time of submission. This had no impact on the peer review process and the final decision.
The authors declare that no Gen AI was used in the creation of this manuscript.
All claims expressed in this article are solely those of the authors and do not necessarily represent those of their affiliated organizations, or those of the publisher, the editors and the reviewers. Any product that may be evaluated in this article, or claim that may be made by its manufacturer, is not guaranteed or endorsed by the publisher.
1. Smolen, JS, Aletaha, D, and McInnes, IB. Rheumatoid arthritis. Lancet. (2016) 388:2023–38. doi: 10.1016/S0140-6736(16)30173-8
2. Srivastava, S, and Rasool, M. Genetics, epigenetics, and autoimmunity constitute a Bermuda triangle for the pathogenesis of rheumatoid arthritis. Life Sci. (2024) 357:123075. doi: 10.1016/j.lfs.2024.123075
3. Radu, AF, and Bungau, SG. Management of rheumatoid arthritis: an overview. Cells. (2021) 10:2857. doi: 10.3390/cells10112857
4. Deane, KD, Demoruelle, MK, Kelmenson, LB, Kuhn, KA, Norris, JM, and Holers, VM. Genetic and environmental risk factors for rheumatoid arthritis. Best Pract Res Clin Rheumatol. (2017) 31:3–18. doi: 10.1016/j.berh.2017.08.003
5. GBD 2021 Rheumatoid Arthritis Collaborators. Global, regional, and national burden of rheumatoid arthritis, 1990-2020, and projections to 2050: a systematic analysis of the global burden of disease study 2021. Lancet Rheumatol. (2023) 5:e594–610. doi: 10.1016/S2665-9913(23)00211-4
6. Gabriel, SE. The epidemiology of rheumatoid arthritis. Rheum Dis Clin N Am. (2001) 27:269–81. doi: 10.1016/s0889-857x(05)70201-5
7. Silman, AJ, and Pearson, JE. Epidemiology and genetics of rheumatoid arthritis. Arthritis Res. (2002) 4:S265–72. doi: 10.1186/ar578
8. Guo, Q, Wang, Y, Xu, D, Nossent, J, Pavlos, NJ, and Xu, J. Rheumatoid arthritis: pathological mechanisms and modern pharmacologic therapies. Bone Res. (2018) 6:15. doi: 10.1038/s41413-018-0016-9
9. Schett, G. Autoimmunity as a trigger for structural bone damage in rheumatoid arthritis. Mod Rheumatol. (2017) 27:193–7. doi: 10.1080/14397595.2016.1265907
10. Komatsu, N, and Takayanagi, H. Mechanisms of joint destruction in rheumatoid arthritis - immune cell-fibroblast-bone interactions. Nat Rev Rheumatol. (2022) 18:415–29. doi: 10.1038/s41584-022-00793-5
11. Steiner, G, and Toes, REM. Autoantibodies in rheumatoid arthritis - rheumatoid factor, anticitrullinated protein antibodies and beyond. Curr Opin Rheumatol. (2024) 36:217–24. doi: 10.1097/BOR.0000000000001006
12. Rönnelid, J, Turesson, C, and Kastbom, A. Autoantibodies in rheumatoid arthritis - laboratory and clinical perspectives. Front Immunol. (2021) 12:685312. doi: 10.3389/fimmu.2021.685312
13. Figus, FA, Piga, M, Azzolin, I, McConnell, R, and Iagnocco, A. Rheumatoid arthritis: extra-articular manifestations and comorbidities. Autoimmun Rev. (2021) 20:102776. doi: 10.1016/j.autrev.2021.102776
14. Azam, AT, Odeyinka, O, Alhashimi, R, Thoota, S, Ashok, T, Palyam, V, et al. Rheumatoid arthritis and associated lung diseases: a comprehensive review. Cureus. (2022) 14:e22367. doi: 10.7759/cureus.22367
15. Kadura, S, and Raghu, G. Rheumatoid arthritis-interstitial lung disease: manifestations and current concepts in pathogenesis and management. Eur Respir Rev. (2021) 30:210011. doi: 10.1183/16000617.0011-2021
16. Laria, A, Lurati, AM, Zizzo, G, Zaccara, E, Mazzocchi, D, Re, KA, et al. Interstitial lung disease in rheumatoid arthritis: a practical review. Front Med (Lausanne). (2022) 9:837133. doi: 10.3389/fmed.2022.837133
17. Spagnolo, P, Lee, JS, Sverzellati, N, Rossi, G, and Cottin, V. The lung in rheumatoid arthritis: focus on interstitial lung disease. Arthritis Rheumatol. (2018) 70:1544–54. doi: 10.1002/art.40574
18. Fazeli, MS, Khaychuk, V, Wittstock, K, Han, X, Crocket, G, Lin, M, et al. Rheumatoid arthritis-associated interstitial lung disease: epidemiology, risk/prognostic factors, and treatment landscape. Clin Exp Rheumatol. (2021) 39:1108–18. doi: 10.55563/clinexprheumatol/h9tc57
19. Wang, HF, Wang, YY, Li, ZY, He, PJ, Liu, S, and Li, QS. The prevalence and risk factors of rheumatoid arthritis-associated interstitial lung disease: a systematic review and meta-analysis. Ann Med. (2024) 56:2332406. doi: 10.1080/07853890.2024.2332406
20. Joy, GM, Arbiv, OA, Wong, CK, Lok, SD, Adderley, NA, Dobosz, KM, et al. Prevalence, imaging patterns and risk factors of interstitial lung disease in connective tissue disease: a systematic review and meta-analysis. Eur Respir Rev. (2023) 32:220210. doi: 10.1183/16000617.0210-2022
21. England, BR, and Hershberger, D. Management issues in rheumatoid arthritis-associated interstitial lung disease. Curr Opin Rheumatol. (2020) 32:255–63. doi: 10.1097/BOR.0000000000000703
22. Hyldgaard, C, Hilberg, O, Pedersen, AB, Ulrichsen, SP, Løkke, A, Bendstrup, E, et al. A population-based cohort study of rheumatoid arthritis-associated interstitial lung disease: comorbidity and mortality. Ann Rheum Dis. (2017) 76:1700–6. doi: 10.1136/annrheumdis-2017-211138
23. Koduri, G, and Solomon, JJ. Identification, monitoring, and Management of Rheumatoid Arthritis-Associated Interstitial Lung Disease. Arthritis Rheumatol. (2023) 75:2067–77. doi: 10.1002/art.42640
24. Zhou, W, Zheng, J, Yuan, M, Yuan, L, Jia, X, and Liu, H. Differentially expressed lncRNAs in peripheral blood mononuclear cells from middle-aged female patients with rheumatoid arthritis-associated interstitial lung disease. Clin Rheumatol. (2020) 39:2281–9. doi: 10.1007/s10067-020-04977-w
25. Palmucci, S, Galioto, F, Fazio, G, Ferlito, A, Cancemi, G, Di Mari, A, et al. Clinical and radiological features of lung disorders related to connective-tissue diseases: a pictorial essay. Insights Imaging. (2022) 13:108. doi: 10.1186/s13244-022-01243-2
26. Ohno, Y, Koyama, H, Yoshikawa, T, and Seki, S. State-of-the-art imaging of the lung for connective tissue disease (CTD). Curr Rheumatol Rep. (2015) 17:69. doi: 10.1007/s11926-015-0546-8
27. Yoo, H, Hino, T, Hwang, J, Franks, TJ, Han, J, Im, Y, et al. Connective tissue disease-related interstitial lung disease (CTD-ILD) and interstitial lung abnormality (ILA): evolving concept of CT findings, pathology and management. Eur J Radiol Open. (2022) 9:100419. doi: 10.1016/j.ejro.2022.100419
28. Akiyama, M, and Kaneko, Y. Pathogenesis, clinical features, and treatment strategy for rheumatoid arthritis-associated interstitial lung disease. Autoimmun Rev. (2022) 21:103056. doi: 10.1016/j.autrev.2022.103056
29. Allen, RJ, Stockwell, A, Oldham, JM, Guillen-Guio, B, Schwartz, DA, Maher, TM, et al. Genome-wide association study across five cohorts identifies five novel loci associated with idiopathic pulmonary fibrosis. Thorax. (2022) 77:829–33. doi: 10.1136/thoraxjnl-2021-218577
30. Faruque, M, Wanschers, M, Ligtenberg, AJ, Laine, ML, and Bikker, FJ. A review on the role of salivary MUC5B in oral health. J Oral Biosci. (2022) 64:392–9. doi: 10.1016/j.job.2022.09.005
31. Seibold, MA, Wise, AL, Speer, MC, Steele, MP, Brown, KK, Loyd, JE, et al. A common MUC5B promoter polymorphism and pulmonary fibrosis. N Engl J Med. (2011) 364:1503–12. doi: 10.1056/NEJMoa1013660
32. Zhang, Q, Wang, Y, Qu, D, Yu, J, and Yang, J. The possible pathogenesis of idiopathic pulmonary fibrosis considering MUC5B. Biomed Res Int. (2019) 2019:1–12. doi: 10.1155/2019/9712464
33. Biondini, D, Cocconcelli, E, Bernardinello, N, Lorenzoni, G, Rigobello, C, Lococo, S, et al. Prognostic role of MUC5B rs35705950 genotype in patients with idiopathic pulmonary fibrosis (IPF) on antifibrotic treatment. Respir Res. (2021) 22:98. doi: 10.1186/s12931-021-01694-z
34. van der Vis, JJ, Prasse, A, Renzoni, EA, Stock, CJW, Caliskan, C, Maher, TM, et al. MUC5B rs35705950 minor allele associates with older age and better survival in idiopathic pulmonary fibrosis. Respirology. (2023) 28:455–64. doi: 10.1111/resp.14440
35. Juge, PA, Lee, JS, Ebstein, E, Furukawa, H, Dobrinskikh, E, Gazal, S, et al. MUC5B promoter variant and rheumatoid arthritis with interstitial lung disease. N Engl J Med. (2018) 379:2209–19. doi: 10.1056/NEJMoa1801562
36. Juge, PA, Granger, B, Debray, MP, Ebstein, E, Louis-Sidney, F, Kedra, J, et al. A risk score to detect subclinical rheumatoid arthritis-associated interstitial lung disease. Arthritis Rheumatol. (2022) 74:1755–65. doi: 10.1002/art.42162
37. Joo, YB, Ahn, SM, Bang, SY, Park, Y, Hong, SJ, Lee, Y, et al. MUC5B promoter variant rs35705950, rare but significant susceptibility locus in rheumatoid arthritis-interstitial lung disease with usual interstitial pneumonia in Asian populations. RMD Open. (2022) 8:e002790. doi: 10.1136/rmdopen-2022-002790
38. Leavy, OC, Kawano-Dourado, L, Stewart, ID, Quint, JK, Solomon, JJ, Borie, R, et al. Rheumatoid arthritis and idiopathic pulmonary fibrosis: a bidirectional Mendelian randomisation study. Thorax. (2024) 79:538–44. doi: 10.1136/thorax-2023-220856
39. Kim, EJ, Collard, HR, and King, TE. Rheumatoid arthritis-associated interstitial lung disease: the relevance of histopathologic and radiographic pattern. Chest. (2009) 136:1397–405. doi: 10.1378/chest.09-0444
40. Matson, S, Lee, J, and Eickelberg, O. Two sides of the same coin? A review of the similarities and differences between idiopathic pulmonary fibrosis and rheumatoid arthritis-associated interstitial lung disease. Eur Respir J. (2021) 57:2002533. doi: 10.1183/13993003.02533-2020
41. Rossiello, F, Jurk, D, Passos, JF, and d'Adda di Fagagna, F. Telomere dysfunction in ageing and age-related diseases. Nat Cell Biol. (2022) 24:135–47. doi: 10.1038/s41556-022-00842-x
42. Revy, P, Kannengiesser, C, and Bertuch, AA. Genetics of human telomere biology disorders. Nat Rev Genet. (2023) 24:86–108. doi: 10.1038/s41576-022-00527-z
43. Niewisch, MR, Beier, F, and Savage, SA. Clinical manifestations of telomere biology disorders in adults. Hematology Am Soc Hematol Educ Program. (2023) 2023:563–72. doi: 10.1182/hematology.2023000490
44. Molina-Molina, M, and Borie, R. Clinical implications of telomere dysfunction in lung fibrosis. Curr Opin Pulm Med. (2018) 24:440–4. doi: 10.1097/MCP.0000000000000506
45. Borie, R, Kannengiesser, C, Antoniou, K, Bonella, F, Crestani, B, Fabre, A, et al. European Respiratory Society statement on familial pulmonary fibrosis. Eur Respir J. (2023) 61:2201383. doi: 10.1183/13993003.01383-2022
46. Borie, R, and Renzoni, E. Pulmonary fibrosis associated with telomere-related gene mutations: a complex inheritance. Respirology. (2021) 26:1098–100. doi: 10.1111/resp.14168
47. Huang, D, Lin, S, He, J, Wang, Q, and Zhan, Y. Association between COVID-19 and telomere length: a bidirectional Mendelian randomization study. J Med Virol. (2022) 94:5345–53. doi: 10.1002/jmv.28008
48. Bernardinello, N, Crestani, B, Spagnolo, P, Ghanem, M, Homps-Legrand, M, Morer, L, et al. Is telomere length a predictor of long-term survival in patients with COVID-19 pneumonia? Respir Med Res. (2023) 84:101048. doi: 10.1016/j.resmer.2023.101048
49. Wei, D, Jiang, Y, Cheng, J, Wang, H, Sha, K, and Zhao, J. Assessing the association of leukocyte telomere length with ankylosing spondylitis and rheumatoid arthritis: a bidirectional Mendelian randomization study. Front Immunol. (2023) 14:1023991. doi: 10.3389/fimmu.2023.1023991
50. Juge, PA, Borie, R, Kannengiesser, C, Gazal, S, Revy, P, Wemeau-Stervinou, L, et al. Shared genetic predisposition in rheumatoid arthritis-interstitial lung disease and familial pulmonary fibrosis. Eur Respir J. (2017) 49:1602314. doi: 10.1183/13993003.02314-2016
51. Natalini, JG, England, BR, Baker, JF, Chen, Q, Singh, N, Mahajan, TD, et al. Associations between shortened telomeres and rheumatoid arthritis-associated interstitial lung disease among U.S. Veterans Respir Med. (2022) 201:106943. doi: 10.1016/j.rmed.2022.106943
52. Doyle, TJ, Juge, PA, Peljto, AL, Lee, S, Walts, AD, Esposito, AJ, et al. Short peripheral blood leukocyte telomere length in rheumatoid arthritis-interstitial lung disease. Thorax. (2024) 79:182–5. doi: 10.1136/thorax-2023-220022
53. Tomos, I, Karakatsani, A, Manali, ED, Kottaridi, C, Spathis, A, Argentos, S, et al. Telomere length across different UIP fibrotic-interstitial lung diseases: a prospective Greek case-control study. Pulmonology. (2022) 28:254–61. doi: 10.1016/j.pulmoe.2020.11.005
54. Lee, YH, and Bae, SC. Association between shortened telomere length and rheumatoid arthritis: a meta-analysis. Z Rheumatol. (2018) 77:160–7. doi: 10.1007/s00393-016-0209-9
55. Steer, SE, Williams, FMK, Kato, B, Gardner, JP, Norman, PJ, Hall, MA, et al. Reduced telomere length in rheumatoid arthritis is independent of disease activity and duration. Ann Rheum Dis. (2007) 66:476–80. doi: 10.1136/ard.2006.059188
56. Jee, AS, Sheehy, R, Hopkins, P, Corte, TJ, Grainge, C, Troy, LK, et al. Diagnosis and management of connective tissue disease-associated interstitial lung disease in Australia and New Zealand: a position statement from the Thoracic Society of Australia and new Zealand. Respirology. (2021) 26:23–51. doi: 10.1111/resp.13977
57. Medhasi, S, and Chantratita, N. Human leukocyte antigen (HLA) system: genetics and association with bacterial and viral infections. J Immunol Res. (2022) 2022:1–15. doi: 10.1155/2022/9710376
58. Dedmon, LE. The genetics of rheumatoid arthritis. Rheumatology (Oxford). (2020) 59:2661–70. doi: 10.1093/rheumatology/keaa232
59. Sharma, SD, Leung, SH, and Viatte, S. Genetics of rheumatoid arthritis. Best Pract Res Clin Rheumatol. (2024) 38:101968. doi: 10.1016/j.berh.2024.101968
60. McInnes, IB, and Schett, G. The pathogenesis of rheumatoid arthritis. N Engl J Med. (2011) 365:2205–19. doi: 10.1056/NEJMra1004965
61. MacGregor, AJ, Snieder, H, Rigby, AS, Koskenvuo, M, Kaprio, J, Aho, K, et al. Characterizing the quantitative genetic contribution to rheumatoid arthritis using data from twins. Arthritis Rheum. (2000) 43:30–7. doi: 10.1002/1529-0131(200001)43:1<30::AID-ANR5>3.0.CO;2-B
62. Florescu, A, Gherghina, FL, Mușetescu, AE, Pădureanu, V, Roșu, A, Florescu, MM, et al. Novel biomarkers, diagnostic and therapeutic approach in rheumatoid arthritis interstitial lung disease-a narrative review. Biomedicines. (2022) 10:1367. doi: 10.3390/biomedicines10061367
63. Furukawa, H, Oka, S, Shimada, K, Sugii, S, Ohashi, J, Matsui, T, et al. Association of human leukocyte antigen with interstitial lung disease in rheumatoid arthritis: a protective role for shared epitope. PLoS One. (2012) 7:e33133. doi: 10.1371/journal.pone.0033133
64. Jönsson, E, Ljung, L, Norrman, E, Freyhult, E, Ärlestig, L, Dahlqvist, J, et al. Pulmonary fibrosis in relation to genetic loci in an inception cohort of patients with early rheumatoid arthritis from northern Sweden. Rheumatology (Oxford). (2022) 61:943–52. doi: 10.1093/rheumatology/keab441
65. Oldham, JM, Ma, SF, Martinez, FJ, Anstrom, KJ, Raghu, G, Schwartz, DA, et al. MUC5B, and the response to N-acetylcysteine among individuals with idiopathic pulmonary fibrosis. Am J Respir Crit Care Med. (2015) 192:1475–82. doi: 10.1164/rccm.201505-1010OC
66. Bonella, F, Campo, I, Zorzetto, M, Boerner, E, Ohshimo, S, Theegarten, D, et al. Potential clinical utility of MUC5B und TOLLIP single nucleotide polymorphisms (SNPs) in the management of patients with IPF. Orphanet J Rare Dis. (2021) 16:111. doi: 10.1186/s13023-021-01750-3
67. Whalen, W, Berger, K, Kim, JS, Simmons, W, Ma, SF, Kaner, RJ, et al. TOLLIP SNP and antimicrobial treatment effect in idiopathic pulmonary fibrosis. Am J Respir Crit Care Med. (2024) 210:508–11. doi: 10.1164/rccm.202312-2224LE
68. Isshiki, T, Koyama, K, Homma, S, Sakamoto, S, Yamasaki, A, Shimizu, H, et al. Association of rs3750920 polymorphism in TOLLIP with clinical characteristics of fibrosing interstitial lung diseases in Japanese. Sci Rep. (2021) 11:16250. doi: 10.1038/s41598-021-95869-9
69. Hirano, C, Ohshimo, S, Horimasu, Y, Iwamoto, H, Fujitaka, K, Hamada, H, et al. FAM13A polymorphism as a prognostic factor in patients with idiopathic pulmonary fibrosis. Respir Med. (2017) 123:105–9. doi: 10.1016/j.rmed.2016.12.007
70. Shirai, Y, Honda, S, Ikari, K, Kanai, M, Takeda, Y, Kamatani, Y, et al. Association of the RPA3-UMAD1 locus with interstitial lung diseases complicated with rheumatoid arthritis in Japanese. Ann Rheum Dis. (2020) 79:1305–9. doi: 10.1136/annrheumdis-2020-217256
71. Juge, PA, Sparks, JA, Gazal, S, Ebstein, E, Borie, R, Debray, MP, et al. RPA3-UMAD1 rs12702634 and rheumatoid arthritis-associated interstitial lung disease in European ancestry. Rheumatol Adv Pract. (2024) 8:rkae059. doi: 10.1093/rap/rkae059
72. Higuchi, T, Oka, S, Furukawa, H, Shimada, K, and Tohma, S. Lack of association of rs12702634 in RPA3-UMAD1 with interstitial lung diseases in Japanese rheumatoid arthritis patients. Biomark Insights. (2022) 17:17. doi: 10.1177/11772719221091758
73. Hayashi, S, Matsubara, T, Fukuda, K, Maeda, T, Funahashi, K, Hashimoto, M, et al. A genome-wide association study identifying single nucleotide polymorphisms in the PPFIBP2 gene was predictive for interstitial lung disease in rheumatoid arthritis patients. Rheumatol Adv Pract. (2022) 6:rkac088. doi: 10.1093/rap/rkac088
74. Vermant, M, Goos, T, Gogaert, S, De Cock, D, Verschueren, P, and Wuyts, WA. Are genes the missing link to detect and prognosticate RA-ILD? Rheumatol Adv Pract. (2023) 7:rkad023. doi: 10.1093/rap/rkad023
75. Wierczeiko, A, Linke, M, Friedrich, JP, Koch, J, Schwarting, A, Krause, A, et al. A call for gene expression analysis in whole blood of patients with rheumatoid arthritis (RA) as a biomarker for RA-associated interstitial lung disease. J Rheumatol. (2024) 51:130–3. doi: 10.3899/jrheum.2023-0588
76. Nava-Quiroz, KJ, Rojas-Serrano, J, Pérez-Rubio, G, Buendia-Roldan, I, Mejía, M, Fernández-López, JC, et al. Molecular factors in PAD2 (PADI2) and PAD4 (PADI4) are associated with interstitial lung disease susceptibility in rheumatoid arthritis patients. Cells. (2023) 12:2235. doi: 10.3390/cells12182235
77. Nava-Quiroz, KJ, Rojas-Serrano, J, Pérez-Rubio, G, Buendia Roldan, I, Mejía, M, Fernández-López, JC, et al. Single-nucleotide variants in PADI2 and PADI4 and ancestry informative markers in interstitial lung disease and rheumatoid arthritis among a Mexican mestizo population. Data. (2024) 9:5. doi: 10.3390/data9010005
78. Olson, AL, Swigris, JJ, Sprunger, DB, Fischer, A, Fernandez-Perez, ER, Solomon, J, et al. Rheumatoid arthritis-interstitial lung disease-associated mortality. Am J Respir Crit Care Med. (2011) 183:372–8. doi: 10.1164/rccm.201004-0622OC
79. Farquhar, HJ, Beckert, N, Beckert, L, Edwards, AL, Matteson, EL, Frampton, C, et al. Survival of adults with rheumatoid arthritis associated interstitial lung disease - a systematic review and meta-analysis. Semin Arthritis Rheum. (2023) 60:152187. doi: 10.1016/j.semarthrit.2023.152187
80. Juge, PA, Wemeau, L, Ottaviani, S, Desjeux, G, Zhuo, J, Vannier-Moreau, V, et al. Increased mortality in patients with RA-associated interstitial lung disease: data from a French administrative healthcare database. RMD Open. (2023) 9:e003491. doi: 10.1136/rmdopen-2023-003491
81. Nieto, MA, Rodriguez-Nieto, MJ, Sanchez-Pernaute, O, Romero-Bueno, F, Leon, L, Vadillo, C, et al. Mortality rate in rheumatoid arthritis-related interstitial lung disease: the role of radiographic patterns. BMC Pulm Med. (2021) 21:205. doi: 10.1186/s12890-021-01569-5
82. Solomon, JJ, Chung, JH, Cosgrove, GP, Demoruelle, MK, Fernandez-Perez, ER, Fischer, A, et al. Predictors of mortality in rheumatoid arthritis-associated interstitial lung disease. Eur Respir J. (2016) 47:588–96. doi: 10.1183/13993003.00357-2015
83. Sullivan, DI, and Ascherman, DP. Rheumatoid arthritis-associated interstitial lung disease (RA-ILD): update on prevalence, risk factors, pathogenesis, and therapy. Curr Rheumatol Rep. (2024) 26:431–49. doi: 10.1007/s11926-024-01155-8
84. Bongartz, T, Nannini, C, Medina-Velasquez, YF, Achenbach, SJ, Crowson, CS, Ryu, JH, et al. Incidence and mortality of interstitial lung disease in rheumatoid arthritis: a population-based study. Arthritis Rheum. (2010) 62:1583–91. doi: 10.1002/art.27405
85. Hackner, K, Hütter, L, Flick, H, Grohs, M, Kastrati, K, Kiener, H, et al. Screening for rheumatoid arthritis-associated interstitial lung disease-a Delphi-based consensus statement. Z Rheumatol. (2024) 83:160–8. doi: 10.1007/s00393-023-01464-w
Keywords: interstitial lung disease, idiopathic pulmonary fibrosis, rheumatoid arthritis, genetics, MUC5B
Citation: Bernardinello N, Zen M, Castelli G, Cocconcelli E, Balestro E, Borie R and Spagnolo P (2025) Navigating interstitial lung disease associated with rheumatoid arthritis (RA-ILD): from genetics to clinical landscape. Front. Med. 12:1542400. doi: 10.3389/fmed.2025.1542400
Received: 09 December 2024; Accepted: 12 February 2025;
Published: 24 February 2025.
Edited by:
Eirini Vasarmidi, University of Crete, GreeceReviewed by:
Nikita Khmelinskii, Lisbon Academic Medical Center, PortugalCopyright © 2025 Bernardinello, Zen, Castelli, Cocconcelli, Balestro, Borie and Spagnolo. This is an open-access article distributed under the terms of the Creative Commons Attribution License (CC BY). The use, distribution or reproduction in other forums is permitted, provided the original author(s) and the copyright owner(s) are credited and that the original publication in this journal is cited, in accordance with accepted academic practice. No use, distribution or reproduction is permitted which does not comply with these terms.
*Correspondence: Nicol Bernardinello, bmljb2wuYmVybmFyZGluZWxsb0B1bmlwZC5pdA==
Disclaimer: All claims expressed in this article are solely those of the authors and do not necessarily represent those of their affiliated organizations, or those of the publisher, the editors and the reviewers. Any product that may be evaluated in this article or claim that may be made by its manufacturer is not guaranteed or endorsed by the publisher.
Research integrity at Frontiers
Learn more about the work of our research integrity team to safeguard the quality of each article we publish.