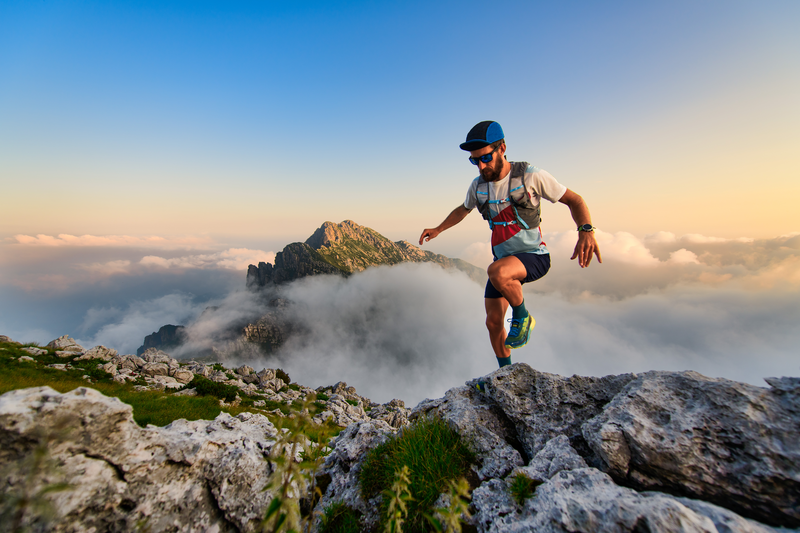
95% of researchers rate our articles as excellent or good
Learn more about the work of our research integrity team to safeguard the quality of each article we publish.
Find out more
ORIGINAL RESEARCH article
Front. Med.
Sec. Hepatobiliary Diseases
Volume 12 - 2025 | doi: 10.3389/fmed.2025.1538528
The final, formatted version of the article will be published soon.
You have multiple emails registered with Frontiers:
Please enter your email address:
If you already have an account, please login
You don't have a Frontiers account ? You can register here
Anti-tuberculosis drug-induced liver injury (ATB-DILI), caused by first-line anti-tuberculosis (anti-TB) drugs, disrupts treatment and increases the risk of drug resistance. The gut microbiota and intestinal barrier integrity play key roles in ATB-DILI susceptibility through the liver-gut axis. Probiotics, such as Bacteroides fragilis 839 (BF839), have shown therapeutic potential in modulating gut microbiota and inflammatory responses. In this study, we investigated the protective effects of BF839 on ATB-DILI in a mouse model of HRZE-induced liver injury. BF839 administration significantly alleviated HRZE-induced liver injury by reducing ALT, AST, AKP, and MDA levels, enhancing SOD and GSH levels, and improving liver histopathology. These effects were associated with restored gut microbiota diversity, enhanced intestinal barrier function, and reduced inflammatory responses. Our findings suggest that BF839 may serve as a potential preventive strategy for ATB-DILI.
Keywords: Bacteroides fragilis 839, Anti-tuberculosis drugs, liver injury, intestinal barrier, LPS/TLRs/NF-κB pathway
Received: 03 Dec 2024; Accepted: 27 Mar 2025.
Copyright: © 2025 Li, Wu, Zhang, Zhou, Li, Bai, Cao and Shi. This is an open-access article distributed under the terms of the Creative Commons Attribution License (CC BY). The use, distribution or reproduction in other forums is permitted, provided the original author(s) or licensor are credited and that the original publication in this journal is cited, in accordance with accepted academic practice. No use, distribution or reproduction is permitted which does not comply with these terms.
* Correspondence:
Xiaoxia Shi, Dalian Medical University, Dalian, China
Disclaimer: All claims expressed in this article are solely those of the authors and do not necessarily represent those of their affiliated organizations, or those of the publisher, the editors and the reviewers. Any product that may be evaluated in this article or claim that may be made by its manufacturer is not guaranteed or endorsed by the publisher.
Research integrity at Frontiers
Learn more about the work of our research integrity team to safeguard the quality of each article we publish.