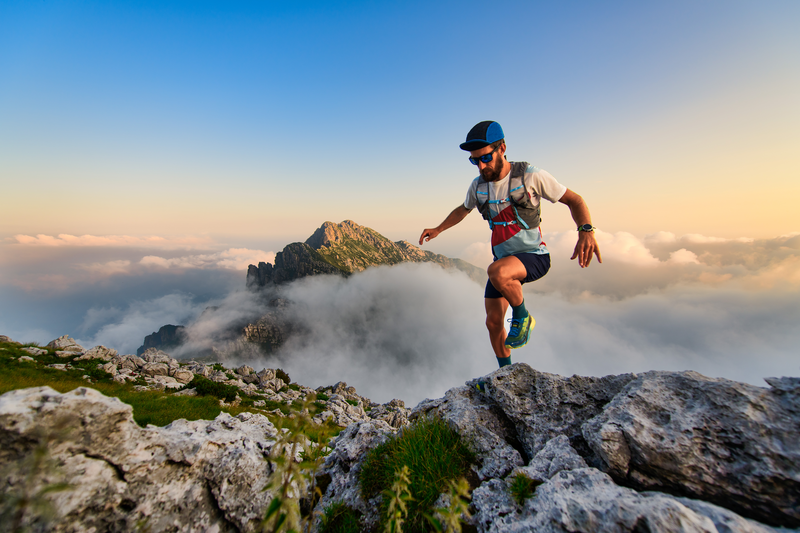
94% of researchers rate our articles as excellent or good
Learn more about the work of our research integrity team to safeguard the quality of each article we publish.
Find out more
STUDY PROTOCOL article
Front. Med.
Sec. Geriatric Medicine
Volume 12 - 2025 | doi: 10.3389/fmed.2025.1537515
This article is part of the Research Topic Expanding Therapeutic Horizons with Non-Invasive Vagus Nerve Stimulation View all 5 articles
The final, formatted version of the article will be published soon.
You have multiple emails registered with Frontiers:
Please enter your email address:
If you already have an account, please login
You don't have a Frontiers account ? You can register here
Objective:This study innovatively employs transcutaneous auricular vagus nerve stimulation (taVNS), a non-invasive physical therapy, to intervene in elderly patients with chronic insomnia(CI) comorbid with functional dyspepsia(FD). This research aims to provide a novel, neuromodulation-based precision therapeutic approach characterized by favorable safety and tolerability for integrated management of geriatric comorbidities.Methods/Design:This double-blind randomized controlled trial will enroll 60 elderly patients (60-85 years) meeting ICSD-3 criteria for CI and Rome IV criteria for FD. Using block randomization with computer-generated sequences, eligible participants will be allocated 1:1 to either active taVNS group (n=30) or sham control group (n=30). The CFDA-certified taVNS (Model tVNS501, Reach Medical, China; Registration No. SuXieZhun20212090050) will be positioned at standardized anatomical sites: the concha cymba ( the inferior margin of the intersection between the superior and inferior crura of the antihelix within the cymba conchae), electrical stimulation will deliver with fixed parameters (frequency: 80 Hz, pulse width: 100 μs, pulse 40-60s). The active group will receive validated taVNS parameters, while the sham group will undergo identical procedures without electrical output. Interventions will be administered 30 minutes per session, 5 sessions weekly for 3 consecutive weeks. Primary endpoints include changes in Pittsburgh Sleep Quality Index (PSQI) and Nepean Dyspepsia Symptom Index (NDSI) at week 3. Secondary outcomes encompass Insomnia Severity Index (ISI), 36-Item Short Form Survey (SF-36), and serum biomarkers (pro-inflammatory cytokines IL-1β, IL-4, IL-6, TNF-α, hs-CRP, TGF-β; DA, 5-HT, NE, Glutamate (Glu), γ-aminobutyric acid (GABA)). Safety profiles will be systematically evaluated using CTCAE v5.0 criteria, with all adverse events documented throughout the study.Discussion: This study mitigate the adverse effects associated with the significant side effects of oral medications in elderly patients with CI comorbid with FD. It seeks to scientifically validate the clinical efficacy of taVNS therapy, elucidate its underlying anti-inflammatory and neuromodulatory mechanisms, and establish a multimodal evidence chain integrating "efficacy-inflammation-neuromodulation." By doing so, this research provides a novel, convenient, scientifically validated, effective, and safe non-pharmacological therapeutic approach for elderly patients with CI and FD, it offers innovative insights and methodologies for the development of pharmaceuticals, medical devices, and related products.
Keywords: Auricular, Vagus Nerve Stimulation, elderly patients, Chronic insomnia, functional dyspepsia, randomized controlled trial
Received: 01 Dec 2024; Accepted: 07 Mar 2025.
Copyright: © 2025 Zhou, Tang, WANG, Huang, Zeng, Liu and Wang. This is an open-access article distributed under the terms of the Creative Commons Attribution License (CC BY). The use, distribution or reproduction in other forums is permitted, provided the original author(s) or licensor are credited and that the original publication in this journal is cited, in accordance with accepted academic practice. No use, distribution or reproduction is permitted which does not comply with these terms.
* Correspondence:
Xing Tang, Sichuan Integrative Medicine Hospital, Chengdu, 610041, Sichuan Province, China
Disclaimer: All claims expressed in this article are solely those of the authors and do not necessarily represent those of their affiliated organizations, or those of the publisher, the editors and the reviewers. Any product that may be evaluated in this article or claim that may be made by its manufacturer is not guaranteed or endorsed by the publisher.
Research integrity at Frontiers
Learn more about the work of our research integrity team to safeguard the quality of each article we publish.