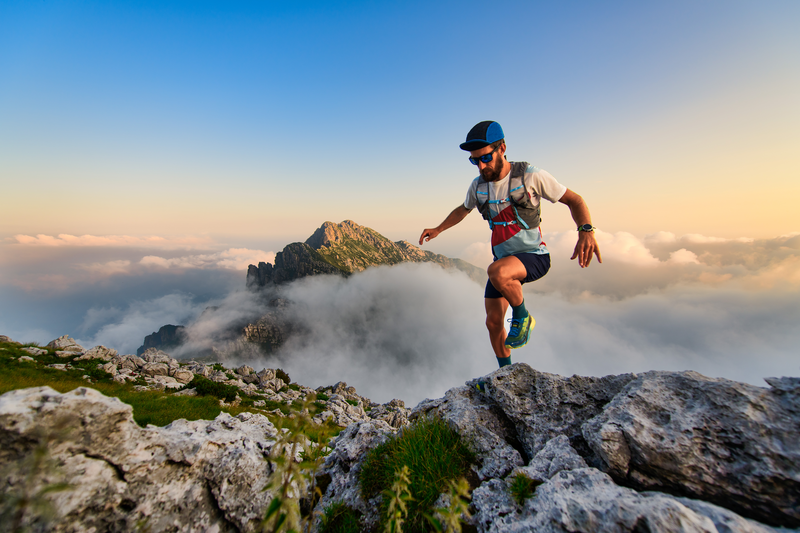
95% of researchers rate our articles as excellent or good
Learn more about the work of our research integrity team to safeguard the quality of each article we publish.
Find out more
REVIEW article
Front. Med. , 11 March 2025
Sec. Nephrology
Volume 12 - 2025 | https://doi.org/10.3389/fmed.2025.1533011
This article is part of the Research Topic Pathophysiology of Diabetic Kidney Disease View all 5 articles
Diabetes mellitus is the most common cause of chronic kidney disease leading to kidney failure and premature death. Over the years, the nomenclature of kidney involvement in diabetes mellitus has evolved, driven both by the understanding that the phenotype may be more diverse than initially thought and by pragmatism. In clinical practice, most patients with diabetes mellitus do not undergo a comprehensive work-up (including kidney biopsy and genetic testing) to exclude the presence or coexistence of additional factors or other kidney diseases. Furthermore, the inclusion criteria for successful kidney protection clinical trials that are the basis of current guidelines covered a wide range of kidney phenotypes under the label of “diabetes and kidney disease,” without requiring proactive efforts to exclude other nephropathies. The aim of this review is to provide a critical review of the most common chronic kidney disease phenotypes in the context of diabetes mellitus and discuss the evolving nomenclature. Various topics are discuss diabetic kidney disease, classic diabetic nephropathy, regression of albuminuria, rapid progression, non-albuminuric and non-proteinuric kidney disease, the connections between and the impact of aging on these phenotypes and a glimpse into future phenotypes resulting from proactive prevention rather than reactive treatment of kidney disease in diabetes.
Since the initial description of histological lesions associated with diabetes by Kimmelstiel and Wilson (1), various phenotypes of kidney disease in diabetes have been described, some with well-differentiated histological features. Concomitantly, the nomenclature has evolved, and numerous terms such as “diabetic nephropathy,” “diabetic kidney disease (DKD),” “non-diabetic kidney disease in diabetic patients,” “diabetes and chronic kidney disease (CKD),” and others have coexisted without consensus definitions (2). More recently, diverse phenotypes have been recognized, and additional terms have been proposed. Beyond the lack of uniformity, a likely significant consequence of the ambiguous and confusing terminology has been a high rate of underdiagnosis of kidney disease in patients with diabetes, which negatively impacts treatment decisions and outcomes.
The 2022 KDIGO (Kidney Disease Improving Global Outcomes) and ADA-KDIGO (American Diabetes Association- Kidney Disease Improving Global Outcomes) guidelines on kidney disease and diabetes have proposed an inclusive term for the entire spectrum of this condition: “diabetes and kidney disease” (2, 3). This paper review the most common phenotypes of CKD in the context of diabetes mellitus and discuss the evolving nomenclature. Specifically, classic diabetic nephropathy, regression of albuminuria, rapid progression, non-albuminuric and non-proteinuric kidney disease, the connections between these phenotypes, the impact of aging on these phenotypes and a glimpse into future phenotypes resulting from proactive prevention rather than reactive treatment of kidney disease in diabetes. It will also explore the clinical and epidemiological implications of the different terms and the underlying histology.
Kidney disease in diabetes was first characterized in 1936 by Kimmelstiel and Wilson based on autopsy findings in eight patients with diabetes, albuminuria, hypertension, and kidney failure (1). Median age was 59 years and a majority had a 3- to 6-year history of diabetes. This was only 13 years after the initiation of the commercial use of insulin (3). The main histological characteristic was the presence of “nodular glomerulosclerosis”. In 1946, Bell et al. (4) described a form of “diffuse glomerulosclerosis” with some controversy regarding its specificity to diabetes and varying prevalence in autopsy studies. Interestingly, almost 90 years later, nodular glomerulosclerosis and advanced diabetic glomerulosclerosis remain the only lesions that allow broad agreement between pathologists for the diagnosis of diabetic nephropathy, since pathologists examining the same kidney tissue disagree in over 50% of cases of milder glomerular lesions, and non-glomerular lesions are non-specific, with the potential exception of hyalinosis of the efferent arteriole (5).
In the 1970s and 1980s, histological changes in early stages of renal involvement in patients with diabetes were identified, such as an increased glomerular capillary filtration surface (6) and thickening of the glomerular basement membrane (GBM) (7). Additionally, some patients showed predominant ischemic changes in contrast to the classic nodular forms. Experimental models of renal artery stenosis in diabetic rats demonstrated that ischemic kidneys do not develop the classical lesions of nodular glomerulosclerosis (8, 9), a concept supported by anecdotal human evidence (10), although they do develop mesangial expansion, which is aggravated by hypertension. It was concluded that increased renal perfusion and glomerular capillary pressure contribute to the full expression of the characteristic histological lesion, “diabetic nephropathy.” By 1993 type 1 (T1DM) and type 2 diabetes (T2DM) were shown to have indistinguishable patterns of kidney injury with similar clinical and histological correlations, although patients with T2DM are usually older and may also have age-associated changes in kidney structure and function that confound the histological features and clinical presentation (Figure 1) (11). However, age is just one of multiple preexistent or concurrent factors that may impact the function and structure of the kidneys at the start or over the course of DM, beyond DM itself (Figure 2). Various clinical parameters, many of which are also cardiovascular risk factors, have been associated with the progression of kidney function decline in individuals with DM. The ARIC study (12) identified several contributors to a faster reduction in eGFR, including a high-risk APOL1 genotype, insulin use, elevated systolic blood pressure, and poor glycemic control. Findings from the Swedish National Diabetes Register also associated the onset of micro- or macroalbuminuria with older age, male sex, smoking, high body mass index, elevated systolic blood pressure, HbA1c, plasma triglycerides, and low HDL cholesterol (13).
Figure 1. Age as a driver and modifier of kidney changes observed in people with diabetes mellitus (DM). The outer graph represents the prevalence of DM in high-income countries (red line). For other regions, the general shape of the curve is similar although the peak may be larger or smaller. The inner graph (purple background) represents the interquartile range (purple lines) of GFR in the general population. The green rectangle represents the age of peak prevalence of type 1 DM and the orange rectangle, the age at the peak prevalence of type 2 DM. Note that in people with type 2 DM, aging has already resulted in a GFR lower than 60 mL/min/1.73 m2 in more than 25% of people above certain age. Depending on the age at onset of DM, its impact may be different in terms of baseline GFR and age-related CKD may already be present [Information derived from references (65, 66)].
Figure 2. Age and multiple other factors may influence the phenotype of kidney involvement in diabetes mellitus (DM) beyond DM itself. These factors may be present at the start of DM or develop concurrently with DM or result from interventions (or the lack of thereof) by physicians and patients [Adapted from Oshima et al. (28)].
Thus, heterogeneity in presenting phenotype and over the course of kidney involvement in people with DM is not only possible but expected.
In the 2007 Kidney Disease Outcomes Quality Initiative (KDOQI) guidelines for diabetes and CKD, the diagnostic criteria for DKD were microalbuminuria or macroalbuminuria in the presence of diabetic retinopathy in T2DM and microalbuminuria with a duration of diabetes exceeding 10 years in T1DM. This diagnosis should be made in the absence of another cause justifying kidney disease and without features suggestive of non-diabetic kidney disease (sudden decline in glomerular filtration rate, abrupt increase in proteinuria, and others) (14). In 2010, the Renal Pathological Society (5) classified the histological lesions of diabetic nephropathy, including forms with vascular and/or tubulointerstitial predominance (15). The term “DKD” was proposed to encompass classic diabetic nephropathy with predominantly glomerular involvement and these other histological forms with predominant vascular and tubulointerstitial involvement, where albuminuria may be absent, as opposed to the term diabetic nephropathy (14). The classification also included other entities (non-diabetic kidney disease in patients with diabetes) (15). Non-proteinuric DKD is often excluded from guidelines and diagnostic algorithms, partly due to inconsistent definitions. Terms like “non-albuminuric” (albuminuria <30 mg/g) and “non-proteinuric” are misleading, as physiological levels of albuminuria and proteinuria exist. For example, tubular cells secrete uromodulin, the main protein in healthy urine, meaning true “non-proteinuric” states do not exist. Moreover, the term “non-albuminuric” is sometimes used for individuals with detectable albuminuria below 30 mg/g. The KDIGO term A1 albuminuria may be more accurate, as “non-albuminuric” could imply a complete absence of albuminuria, which is incorrect (16).
The high variability in the prevalence of kidney disease in the diabetic population can be attributed to genetic factors, socioeconomic differences, access to health care and education, compliance with treatment recommendations and biases in screening high-risk individuals, among others. Still, failure to follow screening guidance and the terminological inconsistency may also have an important role. The result is a high rate of undiagnosed kidney disease in patients with diabetes, primarily in T2DM.
All guidelines for kidney disease screening include at least an annual measurement of serum creatinine, estimating the glomerular filtration rate (eGFR) using standardized formulas, and urine albumin-creatinine ratio (UACR) starting at the time of diagnosis of T2DM and 5 years after the onset in T1DM (17). However, compliance with these recommendations is suboptimal. A recent US study showed that only 52.6% adults with T2DM had an annual albuminuria determination (18). There was a wide variability on the actual percentage depending on the type of organization and geographic area and this influenced the reported prevalence of albuminuria (and thus, of CKD) in the T2DM population. Thus, in T2DM patients with albuminuria screening rates of 20, 50, and 100%, the overall prevalence of albuminuria was 6, 15, and 30%, respectively (18).
While non-compliance with screening guidelines for kidney disease in the diabetic population is alarming, even more worrying is the low rate of physician characterization of a diagnosis of “DKD” or even the more generic “CKD” in clinical records of patients who meet diagnostic criteria based on eGFR and/or UACR results. An analysis of electronic medical records of over 15,000 T2DM patients treated at the Puerta de Hierro Majadahonda Hospital between 2009 and 2019 identified the presence of CKD based on albuminuria and/or eGFR values (estimated by the 2009 CKD-EPI equation used in a race-free manner) in 4,526 patients, of which only 1,498 (33%) had a diagnosis of “CKD,” and only 341 (7.5%) had a diagnosis of “DKD,” “diabetic nephropathy,” or “diabetic CKD” (19). The highest rate of underdiagnosis was found in patients with low (A1) albuminuria values, in women, and in the elderly, a finding consistent with other studies (20). This highlights the coexistence of two issues: (a) suboptimal screening for CKD in patients with T2DM patients, and (b) suboptimal interpretation of analytical results and translation into clinical diagnoses of “DKD” or variants of this to be reflected into health records.
The underdiagnosis of CKD in patients with diabetes leads to inaccurate epidemiological estimates and has a negative impact on the prescription of kidney protective treatment and on outcomes. Renin-angiotensin-aldosterone system (RAAS) blockers and new antidiabetic agents with cardio- and nephroprotective effects (sodium-glucose cotransporter 2 inhibitors (SGLT2i) - and glucagon-like peptide-1 receptor agonists – (GLP-1 RA)) have drastically changed the prognosis of T2DM patients with kidney disease, reducing cardiovascular events and mortality, slowing the decline of eGFR, and delaying the need for renal replacement therapy (21–23). The underdiagnosis of CKD is a missed opportunity for intervention, as demonstrated in the Swedish population where it was associated with higher prescription of nephrotoxic agents, lower rates of referral to nephrology specialists and delayed initiation of treatment with RAAS blockers or statins when indicated (20, 24). Although there is no similar analysis in the T2DM population, it is reasonable to expect a similar impact: the lack of adequate identification of kidney disease in T2DM patients would limit their access to pharmacological treatments that would definitively modify disease progression as well as cardiovascular and mortality risk and would increase the risk of exposure to nephrotoxic drugs.
The recent 2022 KDIGO guidelines on kidney disease and diabetes address this issue in their introduction (2). Most kidney disease in patients with diabetes is of diabetic etiology, except when there are other evident causes of kidney disease. Although DKD may coexist with other nephropathies and having diabetes does not protect from developing any other kidney disease, there is currently no clear consensus on the use of biomarkers, renal biopsy or genetic testing to diagnose (by excluding other causes) and classify DKD. Therefore, based on inclusion criteria for clinical trials, KDIGO guidelines opt to treat all patients with diabetes and kidney disease similarly, using solely the presence of CKD following assessment of eGFR and albuminuria as criterion to start therapy. Henceforth, this document adopts the terminology “diabetes and kidney disease” (2) (Table 1).
This approach is based on the findings of recent clinical trials with SGLT2i and GLP-1 RA, which have demonstrated clinically relevant benefits in a broad group of T2DM patients with varying eGFR levels and different rates of albuminuria, as long as they have CKD. Choosing terms that include the majority of patients with diabetes and kidney disease, regardless of clinical presentation, sends a clear and easy message for both specialists and non-specialists and will facilitate universal access to kidney protective therapies. Especially since, to date, all subgroups of CKD patients with T2DM represented in clinical trials benefited from adding SGLT2i or GLP-1 RA to their therapeutic regimen (25–27).
The 2022 KDIGO guidelines on diabetes and kidney disease explicitly discourage the use of the term “diabetic nephropathy,” considering it outdated and lacking a clear consensus for its definition (2). However, they find it appropriate to use the term “DKD,” which has traditionally been used to include most histological forms of kidney involvement in patients with diabetes, acknowledging its limitations.
The evolving terms for kidney disease in patients with diabetes reflect the phenotypic variability of kidney involvement and the limited diagnostic work-up (usually not involving kidney biopsy nor genetic studies) in most subjects. Phenotypes range from classic proteinuric forms to non-albuminuric variants with slow progression, with a range of clinical presentations with distinct renal prognoses, specific histological features, and in some cases, biomarkers that could in the future aid in their identification.
Several factors may have contributed to the evolving phenotype of kidney disease in patients with diabetes: multidrug treatment improving metabolic control, the introduction of antiproteinuric drugs, and the evolving landscape of comorbidities and risk factors (lower prevalence of smoking, higher prevalence of obesity, hypertension, and older age). This may explain the decreasing prevalence of classic forms relative to other kidney phenotypes. Oshima et al. (28) proposed four phenotypes considering the magnitude of albuminuria, the rate of loss of eGFR and their changes over time (Figure 3), and suggested associated factors and histopathological correlations. A critical overview is provided, as these phenotypes are not mutually exclusive (Figure 4).
Figure 3. Main phenotypes of chronic kidney disease in diabetes mellitus. Color of lines represents magnitude of albuminuria. Several lines in the same panel, they represent a range of possibilities [According to and adapted from reference (28)].
Figure 4. Relationships between the phenotypes of chronic kidney disease in diabetes mellitus (DM). As proposed by Oshima et al. [reference (28)], in classic DM nephropathy, albuminuria may regress when treated with kidney-protective drugs. Additionally, rapid progression characteristically occurs in natural history of classic DM nephropathy in the overt proteinuria stage. However, some patients have rapid progression with milder albuminuria (e.g., nonproteinuric patients) or even in the absence of both A2 and A3 albuminuria, i.e., non-albuminuric patients. Beware that non-albuminuric and non-proteinuric phenotypes should not be mixed up as albuminuria thresholds differ from proteinuria thresholds and have different implication for outcomes. The size of the squares does not necessarily represent the relative prevalence of the diverse phenotypes [Adapted from Oshima et al. (28)].
The clinical and histological features of “classic diabetic nephropathy” were characterized long before the development of the current anti-hypertensive and anti-diabetic drugs. It remains the predominant form of renal disease in patients with poorly controlled metabolic status and blood pressure levels. Some authors suggest that the classical histological features should be confirmed by biopsy before diagnosing diabetic nephropathy (29).
The natural history of classic diabetic nephropathy starts with an initial increase in eGFR (glomerular hyperfiltration) and development of albuminuria, which progresses over time to overt proteinuria, which may reach nephrotic range. eGFR typically declines when albuminuria is already well established and progresses to advanced stages of CKD in the absence of intervention (30). Of note, untreated classic diabetic nephropathy may progress at a mean rate of eGFR decline of 10 to 12 mL/min/1.73 m2/year once overt proteinuria is present (31, 32), i.e., it fulfills the KDIGO concept of rapid progression (eGFR slope decline faster than 5 mL/min/1.73 m2/year) (16).
The histological pattern of classic diabetic nephropathy is characterized by predominantly glomerular involvement, which is classified into four evolutionary classes (33): Thickening of the GBM (class I), mesangial expansion (class II), nodular sclerosis (class III), and advanced diabetic glomerulosclerosis (class IV).
In some patients, structural kidney changes and albuminuria may regress upon successful treatment of the underlying metabolic defect (e.g., by pancreas transplantation in T1DM) (34) or antiproteinuric treatment with RAAS blockers, SGLT2i, or GLP-1 RA (35). Regression of kidney disease features is associated with more favorable outcome than untreated or unresponsive “classic diabetic nephropathy,” as demonstrated by intervention studies (36, 37). However, in patients with type 1 diabetes, the association with favorable outcome is less clear (37). In the recent EMPA-KIDNEY clinical trial, regression of albuminuria was observed in patients with albuminuria and mildly decreased eGFR, in whom the GFR slope decline was slower than 1 mL/min/year even in participants randomized to placebo (38).
Typical histologic renal diabetic lesions (e.g., thickening of the GBM, mesangial expansion) may regress in patients with type 1 diabetes, but very slowly, requiring several years of full correction of the metabolic defect (34).
Overall, these observations demonstrate the potential reversibility of DKD and the need for comprehensive metabolic control and kidney protective medication.
However, individualizing “regression of albuminuria” or even “regression of histological evidence of kidney injury” in response to therapy as a standalone phenotype seems awkward, and it would be more realistic to characterize this improvement as “response to therapy” rather than a specific phenotype.
Osima et al. (28) describe a phenotype characterized by a rapid and early decline in eGFR in individuals with diabetes, even in the absence of albuminuria.
As indicated above, KDIGO defines rapid progression of CKD as an annual decline in GFR ≥5 mL/min/1.73 m2 and in classic texts this rapid progression was observed in untreated “classic diabetic nephropathy” after the development of overt proteinuria (31, 32), again challenging the notion that this should be considered a standalone phenotype. The prevalence of rapid progression varies widely depending on the series, ranging from 14 to 61% (39, 40). Risk factors for rapid progression include higher GFR, elevated systolic blood pressure, and the presence of albuminuria. However, assessing eGFR changes in persons with elevated eGFR is tricky since small changes in serum creatinine, within the range observed in day-to-day variability, may be associated with changes in eGFR larger than 5 mL/min/1.73 m2.
Since “classic diabetic nephropathy” can also progress fast, it has been proposed that the main difference compared to the “classic” phenotype is that the decline in GFR occurs early and may even occur in patients without albuminuria (41). However, we are again on thin ice since a perception of “early” occurrence does not account for prior undetected T2DM or kidney involvement or both for several years. Additionally, the observation that higher albuminuria increases the risk of rapid progression is contrary to the suggestion that this phenotype should be individualized because it can occur in normoalbuminuric individuals.
Knowledge about the histological lesion in rapid progressors is limited. Biopsies from T2DM patients showed that the arteriolar hyalinosis index was higher in the rapid decliner group (patients with an annual rate of GFR decline ≥3.0%) (42). In other series, glomerular nodular sclerosis (i.e., “classical diabetic nephropathy”) and mesangiolysis were the histological lesions that best correlated with rapid progression arguing against individualization of rapid progression as a phenotype distinct from classic diabetic nephropathy (36). Although there is abundant literature addressing the good correlation of some forms of histological lesions with the prognosis of kidney disease in T2DM, it is doubtful whether this adds any advantage over the prognostic ability of epidemiological factors (gender, age, smoking) or comorbidities (arterial hypertension, poor glycemic control, obesity) (13, 43), or even of commonly used biomarkers like plasma triglyceride levels and low high-density lipoprotein cholesterol, which have shown excellent correlation with the risk of progression of kidney disease in T2DM (13, 43, 44). Additionally, the coexistence of diabetic retinopathy with any histological form of renal disease was a good predictor of progression to kidney failure in a recent study (45).
Overall, it is believed that the speed of progression of DKD is variable because of the interaction between multiple factors, key among them being compliance with appropriate treatment and the histological or albuminuria stage of kidney disease. Once this fact is recognized, there is little basis to separate “rapid progression” as a different phenotype. It would be of interest, however, to define risk of rapid progression despite optimal therapy, since these patients would be candidates for future trials of novel add-on therapeutic approaches. Urine proteomics was helpful in this regard in the pre-SGLT2i era (46, 47), but additional studies should validate these results in the current therapeutic environment.
While both terms are frequently used interchangeably, they are not. Non-albuminuric usually means that albuminuria (expressed as UACR) is <30 mg/g (i.e., they may not have CKD if eGFR is above 60 mL/min/1.73 m2) while the concept of “non-proteinuric” is not clearly defined and may include patients with albuminuria >30 mg/g (i.e., they may have CKD even if eGFR is above 60 mL/min/1.73 m2). Thus, it is suggest that the discussion should focus on patients with albuminuria <30 mg/g and the term A1 albuminuria is preferable to “non-albuminuric.” As discussed above, the term “non-albuminuric” lacks precision since current methods allow quantification of up to 1 to 3 mg/g albuminuria and albuminuria within the A1 range is already linearly associated with diverse adverse outcomes. Thus, the prognosis information conveyed by albuminuria 1 mg/g differs from that conveyed by albuminuria 25 mg/g (48).
The prevalence of these forms is currently estimated at 20 to 40% of patients with kidney disease and either T1DM or T2DM, respectively. Clinical features that have been associated with this phenotype include female sex, hypertension, smoking, absence of diabetic retinopathy, and the use of RAAS blockers (28). However, smoking is also a risk factor for higher albuminuria (49). In general, lower albuminuria is associated with slower loss of GFR. However, up to 20% of patients in some series may progress to advanced CKD, especially those with suboptimal kidney protective treatment (50). The presence of prior cardiovascular disease appears to be associated with worse outcomes, suggesting a contribution of macrovascular disease to CKD progression.
Histological lesions are variable, ranging from those typical of “classic diabetic nephropathy” to non-specific CKD lesions with glomerular sclerosis, interstitial fibrosis, tubular atrophy, and arteriosclerosis.
Any impact of T2DM will occur on top of the age-associated loss of kidney mass and structural damage already present at the start of DM (Figure 1), on top of any associated kidney impact of the prediabetes stage and on top of ongoing age-associated loss of function and structural damage. Indeed, in healthy men, defined by a set of characteristics that includes not smoking and body mass index (BMI) <30 kg/m2 among others, the slope of measured GFR loss almost triples from age 60 to age 65 years, to −1.5 mL/min/1.73 m2, and this represents mean values, implying that age-associated GFR loss may be even faster in some healthy individuals (51). In natural history, any impact of aging would be likely be added on top of diabetes-induced kidney injury, potentially resulting in a rapid progression phenotype even in the absence of severe albuminuria. A key question for the future is whether age-associated kidney aging may be modified by therapy. In this regard, as discussed below, data from over 20,000 participants in cardiovascular outcomes trials of SGLT2i and of participants with A1 albuminuria in EMPA-KIDNEY support the notion that eGFR slopes on these drugs may be close to zero, i.e., slower than the age-associated slopes, supporting the notion that age-associated GFR loss may be responsive to therapeutic intervention, at least for the duration of current clinical trials (38, 52–54).
Most of the evidence presented in this review is common to diabetes-associated kidney disease that manifests in pediatric patients. However, it is essential to emphasize the limitations of traditional biomarkers like microalbuminuria for early detection of DKD in this population. The use of tubular biomarkers, which precede glomerular injury, alongside advanced genetic and epigenetic studies, could offer potential for early diagnosis and intervention (55).
The significance of identifying the phenotype of patients with kidney disease and diabetes is currently purely prognostic, as it does not imply therapeutic differences according to the latest KDIGO guidelines (2). However, the degree of response to therapeutic intervention is heterogeneous, as demonstrated by the STENO study (56). Although the benefit of SGLT2i and GLP-1 RA is consistent for all patients with kidney disease and diabetes, some subgroups with specific levels of GFR and albuminuria-proteinuria are underrepresented in major clinical trials. Therefore, further analysis is needed to assess the expected benefit of therapeutic intervention in particular patient groups, such as rapid progressors or non-albuminuric phenotypes with higher eGFR. Additionally, early identification of less responsive patients may feed the clinical trial pipeline for novel future interventions.
Cross-sectional GFR and albuminuria-proteinuria values provide information about the patient’s present status and associated risks but do not capture their past or future trajectory. In this context, noninvasive blood and urine markers could be helpful to more accurately stratify the risk and need for additional therapies, most of them being biomarkers of pathophysiological processes involved in the genesis or progression of diabetes-associated kidney disease, such as inflammation, oxidative stress and fibrosis. Among biomarkers with good clinical-histological correlation and potential clinical utility, the following are notable:
• Tumor Necrosis Factor Receptors TNFR1 and/or TNFR2: Circulating levels predict GFR decline and the risk of progression to advanced kidney disease in patients with kidney disease and diabetes (57). TNF is a pro-inflammatory cytokine that can induce oxidative stress by promoting the production of reactive oxygen species (ROS) and activation of TNFR1 is associated with pro-apoptotic and inflammatory signaling that increases ROS generation (58).
• Transforming Growth Factor-β1 (TGF-β1) and its natural antagonist Bone Morphogenetic Protein 7 (BMP7). They are both secretory cytokines belonging to the TGF-β superfamily; TGF-β1 is well known for its pro-fibrotic and pro-oxidative role and BMP7 is recognized as a natural antagonist to TGF-β1, with antifibrotic and anti-inflammatory properties (59, 60).
• Anti-Erythropoietin Receptor (anti-EPOr) Antibodies: These autoantibodies block the response to EPO in diabetes-associated kidney disease, anti-EPOr antibody levels correlate well with the risk of progression, even in patients without albuminuria (61).
• Combined analysis of multiple biomarkers: Advances in laboratory methods have allowed for the simultaneous analysis of multiple, even hundreds of molecules, improving predictive value compared to analyses of individual biomarkers. Several sets of systems biology or multiple biomarkers in blood and urine have been described (Table 2).
Table 2. Factors associated to phenotypes of kidney disease in people with diabetes mellitus according to Oshima et al. (28).
Similar to histological findings, biomarkers have little specificity for different kidney disease phenotypes in patients with diabetes. However, it has been suggested that combining multiple elements may improve the characterization of phenotypes (Table 2). Many experts advocate expanding the indications for renal biopsy in patients with diabetes with kidney disease to further improve risk stratification and therapeutic decision-making (62). However, it remains unclear whether expanding the indications of kidney biopsy will change the therapeutic approach and improve outcomes. Furthermore, expanding the indications of kidney biopsy will likely increase the number of worldwide deaths and complications from the procedure (63).
So far the main healthcare influence on the course of kidney disease in DM has been the prescription of appropriate treatment to optimize metabolic control and provide kidney protection after CKD had developed (64). However, evidence derived from post-hoc analyses of over 20,000 participants in cardiovascular outcomes trials of SGLT2i that had not CKD at baseline (i.e., eGFR was >60 mL/min/1.73 m2 and albuminuria <30 mg/g) support a CKD preventive effect of different SGLT2i in people with T2DM with cardiovascular disease or at high risk of cardiovascular disease [53–55]. To which extent primary prevention of CKD, i.e., proactive treatment of high-risk individuals, will further change the phenotype and histology of CKD in DM is unclear, but it will likely result in lower values of albuminuria and slower eGFR slopes.
The terminology of kidney disease in patients with diabetes has evolved toward a more inclusive nomenclature that prevents underdiagnosis of the disease. Thus, the terms “diabetes and kidney disease” and “DKD” are proposed in the latest KDIGO 2022 guidelines to denote the entire spectrum of patients who may benefit from an integrated therapeutic approach, differentiated solely based on GFR and albuminuria stages that provide further information on risk for adverse outcomes (Table 1).
The novelty of this study lies in its comprehensive exploration of the evolving nomenclature, phenotypic variability, and clinical implications of kidney disease in patients with DM. By addressing inconsistencies in terminology, the study emphasizes the significant underdiagnosis of DKD, its consequences for treatment, and its impact on patient outcomes. The study’s critical analysis of distinct DKD phenotypes—from classic diabetic nephropathy to non-albuminuric forms—offers insights into how clinical, histological, and biomarker data can refine risk stratification and guide future research. This perspective is particularly valuable in paving the way for personalized medicine and proactive prevention in diabetic kidney disease.
Consensus on nomenclature can lead to a revolution like the one initiated with the classification of CKD into 5 stages, promoted by the National Kidney Foundation more than 20 years ago. It can contribute to the accurate coding of kidney disease in diabetes, raise awareness among specialists, harmonize evidence gathering in clinical trials and evidence translation into clinical guidelines, and facilitate the early implementation of nephroprotective measures and specific treatments at all levels of care.
The presentation of kidney disease associated with diabetes is variable, and identifying the specific histological phenotype of kidney disease in patients with diabetes may have pathophysiological and prognostic implications. However, the need for renal biopsy limits this approach and the generation of evidence on its potential to change treatment and outcomes.
The phenotype of DKD is more complex than previously thought. Four main phenotypes have been proposed which display overlap between them and may be influenced by kidney aging, genetic background, comorbidities and environmental factors. Predicting rapid progression despite current kidney protective therapies, especially in non-albuminuric or non-proteinuric forms, remains a key challenge for the future, as it will allow to enroll participants in clinical trials of novel kidney protective treatments. Further changes in DKD phenotypes may be expected when the concept of primary prevention of CKD is firmly established and implemented.
MM: Conceptualization, Supervision, Visualization, Writing – original draft, Writing – review & editing. JP: Funding acquisition, Supervision, Writing – review & editing. CM-F: Investigation, Writing – review & editing. AO: Investigation, Methodology, Visualization, Writing – review & editing. JN-G: Investigation, Methodology, Supervision, Writing – review & editing.
The author(s) declare that financial support was received for the research, authorship, and/or publication of this article. This work was supported by Instituto de Salud Carlos III (research grants PI23/01518, RICORS RD24/0004/0028) through Instituto de Investigation Sanitaria Puerta de Hierro-Segovia-Arana.
The authors declare that the research was conducted in the absence of any commercial or financial relationships that could be construed as a potential conflict of interest.
The authors declare that no Gen AI was used in the creation of this manuscript.
All claims expressed in this article are solely those of the authors and do not necessarily represent those of their affiliated organizations, or those of the publisher, the editors and the reviewers. Any product that may be evaluated in this article, or claim that may be made by its manufacturer, is not guaranteed or endorsed by the publisher.
1. Kimmelstiel, P, and Wilson, C. Intercapillary lesions in the glomeruli of the Kidney. Am J Pathol. (1936) 12:83–98.7.
2. Kidney Disease: Improving Global Outcomes (KDIGO) Diabetes Work Group. KDIGO 2022 clinical practice guideline for diabetes Management in Chronic Kidney Disease. Kidney Int. (2022) 102:S1–S127. doi: 10.1016/j.kint.2022.06.008
5. Tervaert, TWC, Mooyaart, AL, Amann, K, Cohen, AH, Cook, HT, Drachenberg, CB, et al. Pathologic classification of diabetic nephropathy. J Am Soc Nephrol. (2010) 21:556–63. doi: 10.1681/ASN.2010010010
6. Kroustrup, JP, Gundersen, HJ, and Osterby, R. Glomerular size and structure in diabetes mellitus. III. Early enlargement of the capillary surface. Diabetologia. (1977) 13:207–10. doi: 10.1007/BF01219701
7. Kilo, C, Volger, N, and Williamson, JR. Muscle capillary basement membrane changes related to ageing and diabetes mellitus. Diabetes. (1972) 21:881–905. doi: 10.2337/diab.21.8.881
8. Mauer, SM, Steffes, MW, Azar, S, Sandberg, SK, and Brown, DM. The effects of Goldblatt hypertension on development of the glomerular lesions of diabetes mellitus in the rat. Diabetes. (1978) 27:738–44. doi: 10.2337/diab.27.7.738
9. Cohen, AM, Rosenmann, E, and Rosenthal, T. The Cohen diabetic (non-insulin-dependent) hypertensive rat model description of the model and pathologic findings. Am J Hypertens. (1993) 6:989–95. doi: 10.1093/ajh/6.12.989
10. Béroniade, VC, Lefebvre, R, and Falardeau, P. Unilateral nodular diabetic glomerulosclerosis: recurrence of an experiment of nature. Am J Nephrol. (1987) 7:55–9. doi: 10.1159/000167431
11. Osterby, R, Gall, MA, Schmitz, A, Nielsen, FS, Nyberg, G, and Parring, H-H. Glomerular structure and function in proteinuric Type 2 (non-insulin-dependent) diabetic patients. Diabetologia. (1993) 36:1064–70. doi: 10.1007/BF02374500
12. Warren, B, Rebholz, CM, Sang, Y, Lee, AK, Coresh, J, Selvin, E, et al. Diabetes and trajectories of estimated glomerular filtration rate: a prospective cohort analysis of the atherosclerosis risk in communities study. Diabetes Care. (2018) 41:1646–53. doi: 10.2337/dc18-0277
13. Afghahi, H, Cederholm, J, Eliasson, B, Zethelius, B, Gudbjörnsdottir, S, Hadimeri, H, et al. Risk factors for the development of albuminuria and renal impairment in type 2 diabetes--the Swedish National Diabetes Register (NDR). Nephrol Dial Transplant. (2011) 26:1236–43. doi: 10.1093/ndt/gfq535
14. KDOQI. KDOQI clinical practice guidelines and clinical practice recommendations for diabetes and Chronic Kidney Disease. Am J Nephrol. (2007) 49:S12–S154. doi: 10.1053/j.ajkd.2006.12.005
15. Fioretto, P, Mauer, M, Brocco, E, Velussi, M, Frigato, F, Muollo, B, et al. Patterns of renal injury in NIDDM patients with microalbuminuria. Diabetologia. (1996) 39:1569–76. doi: 10.1007/s001250050616
16. Kidney Disease: Improving Global Outcomes (KDIGO) CKD Work Group. KDIGO 2012 clinical practice guideline for the evaluation and Management of Chronic Kidney Disease. Kidney Int Suppl. (2013) 3:1–150. doi: 10.1038/kisup.2012.73
17. García-Maset, R, Bover, J, Segura de la Morena, J, Goicoechea Diezhandino, M, Cebollada del Hoyo, J, San Martín, JE, et al. Documento de información y consenso para la detección y manejo de la enfermedad renal crónica. Nefrologia. (2022) 42:233–64. doi: 10.1016/j.nefro.2021.07.010
18. Stempniewicz, N, Vassalotti, JA, Cuddeback, JK, Ciemins, E, Storfer-Isser, A, Sang, Y, et al. Chronic kidney disease testing among primary care patients with type 2 diabetes across 24 U.S. health care organizations. Diabetes Care. (2021) 44:2000–9. doi: 10.2337/dc20-2715
19. Marques, M, López-Sánchez, P, Tornero, F, Gargantilla, P, Maroto, A, Ortiz, A, et al. The hidden diabetic kidney disease in a university hospital-based population: a real-world data analysis. Clin Kidney J. (2022) 15:1865–71. doi: 10.1093/ckj/sfac100
20. Swartling, O, Yang, Y, Clase, CM, Fu, EL, Hecking, M, Hödlmoser, S, et al. Sex differences in the recognition, monitoring, and management of CKD in health care: an observational cohort study. J Am Soc Nephrol. (2022) 33:1903–14. doi: 10.1681/ASN.2022030373
21. Fernández-Fernandez, B, Sarafidis, P, Soler, MJ, and Ortiz, A. EMPA-KIDNEY: expanding the range of kidney protection by SGLT2 inhibitors. Clin Kidney J. (2023) 16:1187–98. doi: 10.1093/ckj/sfad082
22. Fernandez-Fernandez, B, Sarafidis, P, Kanbay, M, Navarro-Gonzalez, JF, Soler, MJ, Ortiz, A, et al. SGLT2 inhibitors for non-diabetic kidney disease: drugs to treat CKD that also improve glycaemia. Clin Kidney J. (2020) 13:728–33. doi: 10.1093/ckj/sfaa198
23. Górriz, JL, Soler, MJ, Navarro-González, JF, García-Carro, C, Puchades, MJ, D'Marco, L, et al. GLP-1 receptor agonists and diabetic Kidney Disease: a call of attention to nephrologists. J Clin Med. (2020) 9:947. doi: 10.3390/jcm9040947
24. Bosi, A, Xu, Y, Gasparini, A, Wettermark, B, Barany, P, Bellocco, R, et al. Use of nephrotoxic medications in adults with chronic kidney disease in Swedish and US routine care. Clin Kidney J. (2021) 15:442–51. doi: 10.1093/ckj/sfab210
25. Perkovic, V, Jardine, MJ, Neal, B, Bompoint, S, Heerspink, HJL, Charytan, DM, et al. Canagliflozin and renal outcomes in type 2 diabetes and nephropathy. N Engl J Med. (2019) 380:2295–306. doi: 10.1056/nejmoa1811744
26. Heerspink, HJL, Stefansson, BV, Correa-Rotter, R, Chertow, GM, Greene, T, Hou, FF, et al. Dapagliflozin in patients with Chronic Kidney Disease. N Engl J Med. (2020) 383:1436–46. doi: 10.1056/NEJMoa2024816
27. Wanner, C, Inzucchi, SE, Lachin, JM, Fitchett, D, von Eynatten, M, Mattheus, M, et al. EMPA-REG OUTCOME investigators. Empagliflozin and progression of Kidney Disease in type 2 diabetes. N Engl J Med. (2016) 375:323–34. doi: 10.1056/NEJMoa1515920
28. Oshima, M, Shimizu, M, Yamanouchi, M, Toyama, T, Hara, A, Furuichi, K, et al. Trajectories of kidney function in diabetes: a clinicopathological update. Nat Rev Nephrol. (2021) 17:740–50. doi: 10.1038/s41581-021-00462-y
29. Selby, NM, and Taal, MW. An updated overview of diabetic nephropathy: diagnosis, prognosis, treatment goals and latest guidelines. Diabetes Obes Metab. (2020) 22:3–15. doi: 10.1111/dom.14007
30. Coresh, J, Heerspink, HJL, Sang, Y, Matsushita, K, Arnlov, J, Astor, BC, et al. Change in albuminuria and subsequent risk of end-stage kidney disease: an individual participant-level consortium meta-analysis of observational studies. Lancet Diabetes Endocrinol. (2019) 7:115–27. doi: 10.1016/S2213-8587(18)30313-9
31. Viberti, GC, Bilous, RW, Mackintosh, D, and Keen, H. Monitoring glomerular function in diabetic nephropathy. A prospective study. Am J Med. (1983) 74:256–64. doi: 10.1016/0002-9343(83)90624-1
32. Nosadini, R, Velussi, M, Brocco, E, Bruseghin, M, Abaterusso, C, Saller, A, et al. Course of renal function in type 2 diabetic patients with abnormalities of albumin excretion rate. Diabetes. (2000) 49:476–84. doi: 10.2337/diabetes.49.3.476
33. Gorriz Teruel, JL, and Terrádez, L. Nefrología al día. Clínica y Anatomía Patológica de la Nefropatía Diabética. (2023). Available online at: https://www.nefrologiaaldia.org/372 (Last accessed November 1, 2023).
34. Fioretto, P, Steffes, MW, Sutherland, DE, Goetz, FC, and Mauer, M. Reversal of lesions of diabetic nephropathy after pancreas transplantation. N Engl J Med. (1998) 339:69–75. doi: 10.1056/NEJM199807093390202
35. Kristensen, SL, Rørth, R, Jhund, PS, Docherty, KF, Sattar, N, Preiss, D, et al. Cardiovascular, mortality, and kidney outcomes with GLP-1 receptor agonists in patients with type 2 diabetes: a systematic review and meta-analysis of cardiovascular outcome trials. Lancet Diabetes Endocrinol. (2019) 7:776–85. doi: 10.1016/S2213-8587(19)30249-9
36. Retnakaran, R, Cull, CA, Thorne, KI, Adler, AI, and Holman, RRUKPDS Study Group. Risk factors for renal dysfunction in type 2 diabetes: U.K. prospective diabetes study 74. Diabetes. (2006) 55:1832–9. doi: 10.2337/db05-1620
37. de Boer, IH, Gao, X, Cleary, PA, Bebu, I, Lachin, JM, Molitch, ME, et al. Albuminuria changes and cardiovascular and Renal outcomes in type 1 diabetes: the DCCT/EDIC study. Clin J Am Soc Nephrol. (2016) 11:1969–77. doi: 10.2215/CJN.02870316
38. Herrington, WG, Staplin, N, Wanner, C, Green, JB, Hauske, SJ, Emberson, JR, et al. Empagliflozin in patients with Chronic Kidney Disease. N Engl J Med. (2023) 388:117–27. doi: 10.1056/NEJMoa2204233
39. Yoshida, Y, Kashiwabara, K, Hirakawa, Y, Tanaka, T, Noso, S, Ikegami, H, et al. Conditions, pathogenesis, and progression of diabetic kidney disease and early decliner in Japan. BMJ Open Diabetes Res Care. (2020) 8:e000902. doi: 10.1136/bmjdrc-2019-000902
40. Furuichi, K, Shimizu, M, Yuzawa, Y, Tanaka, T, Noso, S, Ikegami, H, et al. Clinicopathological analysis of biopsy-proven diabetic nephropathy based on the Japanese classification of diabetic nephropathy. Clin Exp Nephrol. (2018) 22:570–82. doi: 10.1007/s10157-017-1485-7
41. Krolewski, AS, Niewczas, MA, Skupien, J, Gohda, T, Smiles, A, Eckfeldt, JH, et al. Early progressive renal decline precedes the onset of microalbuminuria and its progression to macroalbuminuria. Diabetes Care. (2014) 37:226–34. doi: 10.2337/dc13-0985
42. Moriya, T, Yamagishi, T, Yoshida, Y, Matsubara, M, and Ouchi, M. Arteriolar hyalinosis is related to rapid GFR decline and long-standing GFR changes observed on renal biopsies in normo-microalbuminuric type 2 diabetic patients. J Diabetes Complicat. (2021) 35:107847. doi: 10.1016/j.jdiacomp.2021.107847
43. Zoppini, G, Targher, G, Chonchol, M, Ortalda, V, Negri, C, Stoico, V, et al. Predictors of estimated GFR decline in patients with type 2 diabetes and preserved kidney function. Clin J Am Soc Nephrol. (2012) 7:401–8. doi: 10.2215/CJN.07650711
44. Kar, D, Gillies, C, Nath, M, Khunti, K, Davies, MJ, and Seidu, S. Association of smoking and cardiometabolic parameters with albuminuria in people with type 2 diabetes mellitus: a systematic review and meta-analysis. Acta Diabetol. (2019) 56:839–50. doi: 10.1007/s00592-019-01293-x
45. Bermejo, S, González, E, López-Revuelta, K, Ibernon, M, López, D, Martín-Gómez, A, et al. The coexistence of diabetic retinopathy and diabetic nephropathy is associated with worse kidney outcomes. Clin Kidney J. (2023) 16:1656–63. doi: 10.1093/ckj/sfad142
46. Schanstra, JP, Zürbig, P, Alkhalaf, A, Argiles, A, Bakker, SJ, Beige, J, et al. Diagnosis and prediction of CKD progression by assessment of urinary peptides. J Am Soc Nephrol. (2015) 26:1999–2010. doi: 10.1681/ASN.2014050423
47. Rodríguez-Ortiz, ME, Pontillo, C, Rodríguez, M, Zürbig, P, Mischak, H, and Ortiz, A. Novel urinary biomarkers for improved prediction of progressive eGFR loss in early Chronic Kidney Disease stages and in high risk individuals without Chronic Kidney Disease. Sci Rep. (2018) 8:15940. doi: 10.1038/s41598-018-34386-8
48. Hallan, SI, Matsushita, K, Sang, Y, Mahmoodi, BK, Black, C, Ishani, A, et al. Chronic Kidney Disease Prognosis Consortium. Age and association of kidney measures with mortality and end-stage renal disease. JAMA. (2012) 308:2349–60. doi: 10.1001/jama.2012.16817
49. Fernandez-Fernandez, B, Mahillo, I, Sanchez-Rodriguez, J, Carriazo, S, Sanz, AB, Sanchez-Niño, MD, et al. Gender, albuminuria and Chronic Kidney Disease progression in treated diabetic Kidney Disease. J Clin Med. (2020) 9:1611. doi: 10.3390/jcm9061611
50. Vistisen, D, Andersen, GS, Hulman, A, Persson, F, Rossing, P, and Jørgensen, ME. Progressive decline in estimated glomerular filtration rate in patients with diabetes after moderate loss in Kidney function-even without albuminuria. Diabetes Care. (2019) 42:1886–94. doi: 10.2337/dc19-0349
51. Melsom, T, Norvik, JV, Enoksen, IT, Stefansson, V, Mathisen, UD, Fuskevåg, OM, et al. Sex differences in age-related loss of Kidney function. J Am Soc Nephrol. (2022) 33:1891–902. doi: 10.1681/ASN.2022030323
52. Neuen, BL, Ohkuma, T, Neal, B, Matthews, DR, de Zeeuw, D, Mahaffey, KW, et al. Relative and absolute risk reductions in cardiovascular and Kidney outcomes with Canagliflozin across KDIGO risk categories: findings from the CANVAS program. Am J Kidney Dis. (2021) 77:23–34.e1. doi: 10.1053/j.ajkd.2020.06.018
53. Mosenzon, O, Raz, I, Wiviott, SD, Schechter, M, Goodrich, EL, Yanuv, I, et al. Dapagliflozin and prevention of Kidney Disease among patients with type 2 diabetes: post hoc analyses from the DECLARE-TIMI 58 trial. Diabetes Care. (2022) 45:2350–9. doi: 10.2337/dc22-0382
54. Levin, A, Perkovic, V, Wheeler, DC, Hantel, S, George, JT, von Eynatten, M, et al. Empagliflozin and cardiovascular and Kidney outcomes across KDIGO risk categories: post hoc analysis of a randomized, double-blind, placebo-controlled, multinational trial. Clin J Am Soc Nephrol. (2020) 15:1433–44. doi: 10.2215/CJN.14901219
55. Muntean, C, Starcea, IM, and Banescu, C. Diabetic kidney disease in pediatric patients: a current review. World J Diabetes. (2022) 13:587–99. doi: 10.4239/wjd.v13.i8.587
56. Gaede, P, Lund-Andersen, H, Parving, HH, and Pedersen, O. Effect of a multifactorial intervention on mortality in type 2 diabetes. N Engl J Med. (2008) 358:580–91. doi: 10.1056/NEJMoa0706245
57. Niewczas, MA, Gohda, T, Skupien, J, Smiles, AM, Walker, WH, Rosetti, F, et al. Circulating TNF receptors 1 and 2 predict ESRD in type 2 diabetes. J Am Soc Nephrol. (2012) 23:507–15. doi: 10.1681/ASN.2011060627
58. Fischer, R, and Maier, O. Interrelation of oxidative stress and inflammation in neurodegenerative disease: role of TNF. Oxid Med Cell Longev. 2015:10813. doi: 10.1155/2015/610813
59. Wong, MG, Perkovic, V, Woodward, M, Chalmers, J, Li, Q, Hillis, GS, et al. Circulating bone morphogenetic protein-7 and transforming growth factor-β1 are better predictors of renal end points in patients with type 2 diabetes mellitus. Kidney Int. (2013) 83:278–84. doi: 10.1038/ki.2012.383
60. Chen, HY, Chou, HC, Ho, YJ, Chang, SJ, Liao, EC, Wei, YS, et al. Characterization of tgf-β by induced oxidative stress in human trabecular meshwork cells. Antioxidants. (2021) 10:1–31. doi: 10.3390/antiox10010107
61. Hara, A, Furuichi, K, Koshino, A, Yasuda, H, Tran, TTT, Iwata, Y, et al. Clinical and pathological significance of autoantibodies to erythropoietin receptor in type 2 diabetic patients with CKD. Kidney Int Rep. (2017) 3:133–41. doi: 10.1016/j.ekir.2017.08.017
62. Gesualdo, L, Fiorentino, M, Conserva, F, and Pontrelli, P. Should we enlarge the indication for kidney biopsy in patients with diabetes? The pro part. Clin Kidney J. (2023) 17:sfad266. doi: 10.1093/ckj/sfad266
63. Ortiz, A. Should we enlarge the indication for kidney biopsy in diabetics? The con part. Clin Kidney J. (2023) 17:sfad267. doi: 10.1093/ckj/sfad267
64. De Boer, IH, Khunti, K, Sadusky, T, Tuttle, KR, Neumiller, JJ, Rhee, CM, et al. Diabetes management in chronic kidney disease: a consensus report by the American Diabetes Association (ADA) and Kidney Disease: improving global outcomes (KDIGO). Kidney Int. (2022) 102:974–89. doi: 10.1016/j.kint.2022.08.012
65. Friberg, L, Gasparini, A, and Carrero, JJ. A scheme based on ICD-10 diagnoses and drug prescriptions to stage chronic kidney disease severity in healthcare administrative records. Clin Kidney J. (2018) 11:254–8. doi: 10.1093/ckj/sfx085
Keywords: chronic kidney disease, diabetes mellitus, diabetic nephropathy, diabetic kidney disease, phenotypes
Citation: Marques M, Portolés J, Mora-Fernández C, Ortiz A and Navarro-González JF (2025) Nomenclature of renal involvement in diabetes mellitus: unify to manage diversity. Front. Med. 12:1533011. doi: 10.3389/fmed.2025.1533011
Received: 22 November 2024; Accepted: 20 February 2025;
Published: 11 March 2025.
Edited by:
Pedro Henrique Franca Gois, The University of Queensland, AustraliaReviewed by:
Edwin Castillo Velarde, Hospital Base Guillermo Almenara Irigoyen, PeruCopyright © 2025 Marques, Portolés, Mora-Fernández, Ortiz and Navarro-González. This is an open-access article distributed under the terms of the Creative Commons Attribution License (CC BY). The use, distribution or reproduction in other forums is permitted, provided the original author(s) and the copyright owner(s) are credited and that the original publication in this journal is cited, in accordance with accepted academic practice. No use, distribution or reproduction is permitted which does not comply with these terms.
*Correspondence: José Portolés, am9zZW0ucG9ydG9sZXNAc2FsdWQubWFkcmlkLm9yZw==; Juan F. Navarro-González,am5hdmdvbkBnb2JpZXJub2RlY2FuYXJpYXMub3Jn
†ORCID: José Portolés, orcid.org/0000-0002-2114-1385
Juan F. Navarro-González, orcid.org/0000-0002-5015-7474
Disclaimer: All claims expressed in this article are solely those of the authors and do not necessarily represent those of their affiliated organizations, or those of the publisher, the editors and the reviewers. Any product that may be evaluated in this article or claim that may be made by its manufacturer is not guaranteed or endorsed by the publisher.
Research integrity at Frontiers
Learn more about the work of our research integrity team to safeguard the quality of each article we publish.