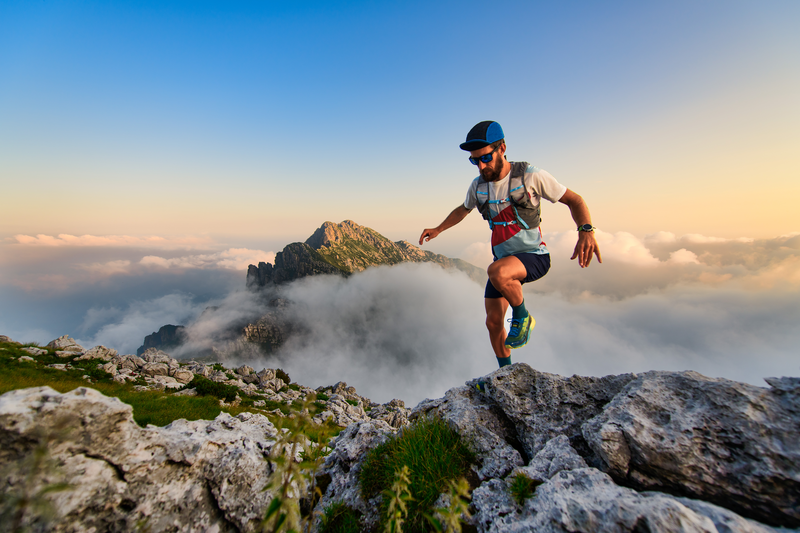
95% of researchers rate our articles as excellent or good
Learn more about the work of our research integrity team to safeguard the quality of each article we publish.
Find out more
ORIGINAL RESEARCH article
Front. Med. , 05 February 2025
Sec. Translational Medicine
Volume 12 - 2025 | https://doi.org/10.3389/fmed.2025.1522517
Purpose: The objective of this study was to investigate the impact of quercetin, a potent antioxidant, on tendon healing utilizing a rat Achilles tendon injury model.
Materials and methods: The study involved 32 male Wistar-Albino rats, randomly split into experimental (quercetin) and control groups, each with 16 rats. A bilateral Achilles tenotomy model was applied, with the experimental group receiving quercetin and the control group receiving corn oil via oral gavage from surgery until sacrifice. One Achilles tendon per rat underwent histopathological and immunohistochemical evaluations, while the other underwent biomechanical analysis.
Results: Tendons were evaluated histopathologically in terms of tenocyte, ground substance, collagen, and vascularity, and quercetin was observed to significantly increase tendon healing in the experimental group (p-values = 0.0232, 0.0128, 0.0272, 0.0307, respectively). In the immunohistochemical analysis, type I collagen, type III collagen, alpha smooth muscle actin (SMA), and Galectin-3 were evaluated, and it was observed that quercetin increased tendon healing (p-values = 0.0166, 0.0036, 0.0323, 0.0295, respectively). In the biomechanical analysis, the rupture strength was evaluated with six parameters (failure load, maximum energy, displacement, stiffness, ultimate stress, and strain), and it was observed that quercetin significantly increased the rupture strength (p-values = 0.032, 0.014, 0.026, 0.025, 0.045, 0.012, respectively).
Conclusion: Quercetin significantly enhanced tendon healing both biomechanically and immunohistochemically. However, further clinical studies are needed to understand its effects on human tendon healing, as this is the first study of its kind.
Tendon injuries, particularly those affecting the Achilles tendon, are on the rise due to the growing interest in sports and the increasing average lifespan (1–4). However, the healing process for injured tendons tends to be prolonged compared to other tissues, primarily due to factors such as low blood perfusion and slow regeneration rates (5). Consequently, there has been considerable research interest in exploring the effects of different antioxidants or anti-inflammatory agents on this challenging and protracted healing process (6–8).
Quercetin, a member of the flavonoid polyphenol class, is commonly present in nature and in the human diet. Its pharmacological properties, including cytoprotective and genoprotective effects such as anti-inflammatory, antioxidative, and antitumoral activities, have been extensively documented (9). Recent research has particularly emphasized its radical scavenging properties and potent antioxidative effects (10).
Quercetin’s antioxidative activities have been extensively studied and demonstrated through various pathways, both in vivo and in vitro (11–21). Firstly, it exerts antioxidative effects by directly neutralizing free radicals, thereby reducing potential damage to important biological components such as proteins, DNA, and lipids (22–24). Secondly, it enhances cellular protection against oxidative damage and regulates antioxidative enzymes like superoxide dismutase (SOD) and catalase (25). Additionally, quercetin can chelate metal ions, offering another mechanism to shield against oxidative damage (26). At the cellular level, studies in animal models have shown that quercetin inhibits the activation of inflammatory signaling pathways, notably MAPKs and NF-κB, in response to oxidative stress. Furthermore, it suppresses the formation of proinflammatory cytokines and chemokines (27–32). These findings underscore quercetin’s multifaceted antioxidative properties and its potential as a therapeutic agent in combating oxidative stress-related disorders.
In such studies, it is imperative to comprehend the impact of the techniques and substances employed in both the experimental and control groups on tendon healing and the mechanical properties of the tendon. Numerous studies in the literature have investigated the mechanical properties exhibited by tendons (33–35). Given that tendons are predominantly composed of an extracellular matrix, alterations in biomechanical properties serve as indicators of the healing process (36).
The bioavailability of quercetin from dietary sources typically ranges from 1 to 10%, but supplementation can increase this up to 50%, thereby enhancing its antioxidative activity (11, 37, 38). Although there are a limited number of studies on the effect of quercetin on tendon tissue (10, 39, 40), there is no study in the literature examining its effect on tendon healing, and its role in this process has not been fully elucidated. Our hypothesis posits that quercetin, as a potent antioxidative, may exert positive effects on tendon healing in rats, and our aim is to demonstrate this on an Achilles tendon injury model.
Thirty-two male Wistar-Albino rats with an average weight of 487 g (450–525 g) and an average age of 12 weeks were utilized in this study. The rats were obtained from Selcuk University Experimental Medicine Research and Application Center (Konya, Turkey). All surgical procedures were conducted in accordance with the approval granted by the Selcuk University Experimental Medicine Research and Application Center Ethics Committee (decision number 2020/58). Power analysis indicated that a minimum of 14 rats per group were necessary to achieve statistical significance, with a predicted type 1 error of 0.05 and an efficacy power of 0.80. Environmental conditions were carefully controlled, with a temperature range of 21°C ± 2°C, humidity maintained at 55% ± 10%, lighting set at 350 lux (measured at bench level), and a 12∶12 light:dark cycle, with lights on at 0700 and off at 1900. The rats were housed in pairs in cages measuring 595 × 380 × 200 mm (Techniplast UK, 1354G Eurostandard Type IV). Throughout the study, measures were taken to ensure the animals did not experience pain, and adherence to the principles outlined in the Declaration of Helsinki was strictly observed. Two groups, comprising 16 rats each (the quercetin group and the control group), were established for histopathological, immunohistochemical, and biomechanical analyses of tendon healing. Bilateral Achilles tendon injury models were applied to all rats.
All surgical procedures performed on the rats were conducted under general anesthesia, ensuring the absence of pedal reflex. An intraperitoneal injection of 80 mg/kg ketamine hydrochloride (Narkamon 50 mg/mL, BIOVETA, Czechia) and 10 mg/kg xylazine (Xylazinbio 2% BIOVETA, Czechia) was administered for anesthesia induction. Furthermore, the rats were continuously monitored for pain sensation throughout the procedure, and additional anesthesia doses were administered as needed to maintain adequate pain control. Prior to incision, the surgical areas were shaved and cleansed with povidone-iodine (Batix®, Denizpharma, Istanbul, Turkey), and all surgical procedures were carried out under sterile conditions.
A well-established tendon injury model, widely utilized in numerous studies in the literature (6, 41, 42), was employed in this study. Following the surgical preparation of the extremity, an incision was made along the posterior line of the ankle to expose the Achilles tendon and plantaris tendon. Tenotomy was performed approximately 0,5 cm proximal to the calcaneus insertion of the Achilles tendon using a No. 15 scalpel blade. The tendons were then repaired end-to-end using the modified Kessler method with 4/0 round polypropylene monofilament sutures (TıpKimSan Limited Company, Istanbul, Türkiye). Following irrigation of the wound area with saline, the incision was closed using 3/0 polypropylene monofilament sutures (TıpKimSan Limited Company, Istanbul, Türkiye) to achieve skin integrity. The surgical procedure is illustrated in Figure 1.
No bandages, dressings, or plasters were applied to the rats postoperatively. From the early postoperative period, all rats were allowed free joint movements and were permitted to load their lower extremities. Rats in the quercetin group received a daily dose of 100 mg/kg quercetin (Sigma-Aldrich, United States), administered as a suspension in corn oil via oral gavage at the same time each day. The control group received only corn oil via oral gavage. All rats were sacrificed on the 28th day following surgery. The quercetin dosage was adjusted based on previous studies (10, 43).
Following administration of high-dose anesthesia (ketamine 75 mg/kg and xylazine 10 mg/kg), rats were euthanized by cervical dislocation. The Achilles tendons of the euthanized rats were then carefully dissected, ensuring removal distally from the bone-tendon junction of the calcaneus and proximally from the bone-tendon junctions of the femur and tibia. Subsequently, one of the Achilles tendons from each rat underwent histopathological and immunohistochemical evaluation, while the other tendon was subjected to biomechanical analysis.
The samples were dissected and kept in 10% formalin fixative solution for 3 days. Then, the tissues were left under running water overnight, and the next day they were passed through a series of 60, 70, 80, and 100% alcohol, respectively, and then taken into xylene. Tissues were embedded in paraffin to obtain thin sections, and 3 μm sections were taken with a microtome. After the sections were kept in an oven at 60°C overnight and deparaffinized, routine Hematoxylin–Eosin (HE) and Masson Trichrome (MT) staining was performed as the first step of the morphological evaluation. After staining, the tissues were photographed with an Olympus CX41 microscope (Olympus Corp., Tokyo, Japan) at 20, 40, and 100 magnifications. The most common and established system for evaluating pathological changes in tendon tissue, particularly utilized in animal experiments, is the Bonar scoring system (44–46). Tenocytes, ground substance, collagen, and vascularization were assessed using the Bonar scoring system, each out of four points. The Bonar scoring system utilizes a four-point scale; ‘0’ represents a normal tendon appearance, ‘1’ indicates mild abnormal tendon appearance (10–20% of total tissue), ‘2’ represents moderately abnormal appearance (over 20% of total tissue), and ‘3’ indicates a markedly abnormal appearance. Total scores were scored from 0 (normal tendon, strong healing) to 12 (most severe detectable pathology, poor healing) and are shown in Figures 2A–D.
Figure 2. The key differences in histological changes between strong and poor healing conditions: (A) curled collagen structure, loose stromal structure, regular configuration (arrow), strong healing (HE, x100). (B) Disrupted configuration with hyalinized collagen bundles (horizontal arrow), irregular cartilaginous tissue (vertical arrow), thick vascular bundles (encircled) (HE, x100). (C) Collagen bundles containing small nuclei structures (arrow) (MT, x100). (D) Thick and irregular vascular clusters (encircled), hyalinized fibrotic areas (arrow), and disorganized, poor healing (MT, x100). (E) Regular configuration with normal collagen bundles (arrow) (IHC, x100). (F) Abnormal collagen structure with thick hyalinized areas (arrow) in irregular configuration (IHC, x100). (G) Near-normal healing characterized by thin-walled capillaries (arrow) (IHC, x100). (H) Poor healing characterized by thick-walled and irregularly distributed vascularization (arrow) (IHC, x100). (I) Clear boundaries with absence of muscle fibers between collagen bundles (arrows) in separated, strong healing (SMA IHC, x200). (J) Irregular configuration of muscle fibers (arrow) observed in tendon healing area of poor healing (SMA IHC, x200). (K) Intensely stained and regular fibroblasts with Galectin-3 (arrow) (Galectin-3 IHC, x200) (HE, Hematoxylin–Eosin; MT, Masson Trichrome; IHC, immunohistochemistry; SMA, alpha smooth muscle actin). (L) Sparsely stained and irregular fibroblasts with Galectin-3 (arrow).
Five micrometer thick sections taken from paraffin blocks with the help of a microtome were then placed on polylysine slides (Sigma, St Louis, MO, United States). After the dehydration stage, microwave application was made in citrate solution with a pH of 6 (Carlo Erba, 368,057). Sections were kept in 3% hydrogen peroxide (Dako, Glastrup, Denmark) to prevent endogenous peroxidase activity. After blocking with normal goat serum, the sections were incubated with primary antibodies, which are type I collagen (1:100, Sigma, United States), type III collagen (1:100, Sigma, United States), alpha smooth muscle actin (SMA) (1:100, RTU, Leica, BK), and Galectin-3 (1:100, RTU, Cell Marque, CA, United States). After the biotin-labeled secondary antibody (Abcam, United Kingdom) step, the tissues treated with the streptavidin-HRP kit (Santa Cruz, USA) were finally treated with 0.05% diaminobenzidine (Zymed Histostain Plus CA, United States). All washes during the staining process were performed using 1X phosphate-buffered saline (PBS, 10 mM phosphate, 137 mM NaCl, 2.7 mM KCl, pH 7.4), purchased from Sigma-Aldrich (St. Louis, MO, United States). Immunohistochemical staining results were evaluated with H-score. Staining ratio was graded semiquantitatively (0 = staining in less than 1% of cells, 1+ = staining in 1–10% of cells, 2+ = staining in 11–50% of cells, 3+ = staining in 51–80% of cells, 4+ = staining in more than 80% of cells). Staining intensity was also determined by the blind method (0 = no staining, 1 = pale, 2 = moderate, 3 = intense). Then, the total score was calculated with the formula “(1 + staining intensity/3) x staining ratio.” The highest marked immunohistochemical images for H-score are shown in Figures 2E–L.
The transverse diameters of the rat’s Achilles tendons were measured using a digital caliper (TESA Unimaster, Renens, Switzerland) (47). It was assumed that the transverse area of the Achilles tendons followed circular geometry. To ensure tissue integrity, the tendons were kept moistened with gauze soaked in a physiological solution during preparation and mounting in the materials testing equipment. The proximal tendon was delicately separated from the muscle for clamping. The length of the tendon between the grips was estimated using a caliper. A tensile test was conducted on both the experimental and control groups’ Achilles tendons (total number of samples 32). Biomechanical tests were performed using the ELISTA, TST 2500 mxe brand axial tensile testing device. The tensile tests were executed under identical conditions for each Achilles tendon sample. Figure 3 illustrates the tensile tests, with the Achilles tendons secured between the jaws of the device, one end attached to the calcaneus and the other to the musculotendinous junction. A 5 kN load cell was employed to conduct the biomechanical tests on the specimens, fixed vertically in the machine using a fixture, at a crosshead speed of 1 mm/min at room temperature. Force (N) versus elongation (mm) was recorded during the tensile test. Parameters such as failure load (N), displacement (mm), total energy (J), ultimate stress (MPa), and strain (%) were derived from the force (N)-elongation (mm) graphs at the conclusion of the biomechanical tests.
In this study, double-blinding and randomization strategies were implemented to reduce potential biases and enhance the reliability of the results. These double-blinding and randomization strategies were employed to enhance the internal validity of the study and enable the evaluation of results independently of external factors. The reliability of the obtained data and the scientific robustness of the study were supported by the integration of these methodological approaches.
The allocation of animals to groups was performed randomly using computer-based randomization methods. This helped balance potential differences among animals, initially while reducing the likelihood of systematic errors between experimental and control groups. In double-blinding, during the execution of the experiment, both the observers applying interventions to the animals and the individuals evaluating the data (microscopic preparations) were kept unaware of group assignments. This approach helped prevent potential biases in subjective assessments and data analysis.
SPSS 23 (Statistical Package for the Social Sciences) program was used for data analysis (Graph Pad Software, Inc., La Jolla, CA). Data were expressed as mean ± standard deviation (SD) or median (min–max). The skewness and kurtosis values of the data were observed to adhere to a normal distribution. Accordingly, an independent two-sample t-test (unpaired t-test) was utilized. A p-value of <0.05 was considered statistically significant.
Throughout the experiment, no complications occurred in either rat group that would disrupt standardization or necessitate exclusion, such as death, wound site infections, or tendon rerupture. Additionally, no circumstances necessitating sample exclusion were observed within groups during data analysis.
During the phases of tendon healing, structures typically observed microscopically, such as tenoblasts, inflammatory cell infiltration, collagen fibrils, and extracellular matrix, were identified in both the experimental and control groups. It was observed that tenoblasts lost their elongated appearance and alignment, while the density of extracellular matrix increased, and tenoblasts decreased in size. Inflammatory cells, newly formed collagen bundles, mature collagen bundles, and tenocytes starting to line up in wavy patterns were observed and depicted as shown in Figures 4A,B. According to the Bonar scoring, tenocytes were 2.31 ± 0.75 in the control group and 1.69 ± 0.73 in the quercetin group, indicating a significant difference between them (p = 0.0232). Ground substance was 2.56 ± 0.66 in the control group and 1.93 ± 0.68 in the quercetin group, showing a significant difference between them (p = 0.0128). Collagen was 2.44 ± 0.68 in the control group and 1.81 ± 0.83 in the quercetin group, indicating a significant difference between them (p = 0.0272). Vascularization was 2.44 ± 0.68 in the control group and 1.81 ± 0.83 in the quercetin group, showing a significant difference between them (p = 0.0307). As shown in Table 1, morphometric scoring revealed significant differences between the control and experimental groups in terms of parameters.
Figure 4. Visualization of microscopic image samples from control and quercetin groups. (A). Graphical representation of comparison of control and quercetin groups according to Bonar histological scores (B). Graphical representation of comparison of H-scores of control and quercetin groups according to Collagen-1 and Collagen-3 in immunohistochemical evaluation (C). Graphical representation of comparison of H-scores of control and quercetin groups according to SMA and Galectin-3 in immunohistochemical evaluation (D). (HE, Hematoxylin–Eosin; MT, Masson Trichrome; SMA, Alpha smooth muscle actin).
Table 1. Tenocyte morphology and proliferation, presence or absence of ground substance, collagen bundle properties, vascularity were evaluated using Bonar scoring system in Hematoxylin–Eosin and Masson Trichrome staining.
In immunohistochemical analyses, tendon healing and ground substance were evaluated using type I collagen, type III collagen, and SMA. To evaluate the relationship between Galectin-3 and fibrosis, Galectin-3 was applied using immunohistochemical methods. The evaluation of Galectin-3 involved counting stained fibroblastic cells under a light microscope at X400 magnification. Following staining with Galectin-3, fibroblasts were differentiated from monocytes, macrophages, and neutrophil cells, and the morphological characteristics of fibroblasts were analyzed in detail. In this analysis, the number of fibroblasts exposed to Galectin-3 was determined, and morphological features associated with fibrosis were identified. As shown in Figure 4A, the region with the highest number of fibroblasts was selected as the focal point of this evaluation.
As shown in Figure 4A, areas with the highest cell count were preferred. In H-score evaluations, Collagen-1 was 78.03 ± 15.17 in the control group and 91.15 ± 14.59 in the quercetin group, indicating a significant difference between them (p = 0.0166). Collagen-3 was 150.54 ± 9.53 in the control group and 162.13 ± 11.17 in the quercetin group, showing a significant difference between them (p = 0.0166). SMA was 19.36 ± 9.78 in the control group and 29.38 ± 14.99 in the quercetin group, indicating a significant difference between them (p = 0.0323). Galectin-3 was 8.99 ± 4.90 in the control group and 13.29 ± 5.71 in the quercetin group, showing a significant difference between them (p = 0.0295). All immunohistochemical parameters are provided in Table 2 and Figures 4C,D.
The biomechanical data regarding the resistance against applied loads of Achilles tendons utilized in this study are presented in Table 3. Biomechanical test outcomes of Achilles tendons for both the quercetin and control groups are depicted in Figure 5. Analysis of failure load (N), displacement (mm), total energy (J), ultimate stress (MPa), and strain (%) data derived from the biomechanical tests for both quercetin and control group samples was conducted in detail. Initially, upon examining the average failure load, the quercetin group’s results (36.5 N) demonstrated mechanically superior outcomes compared to the control group (32.06 N) by approximately 12% (Figure 5A). Displacement and strain values exhibited greater elongation in the quercetin group, suggesting that Achilles tendons exhibit higher elongation under mechanical load in this group (Figures 5B,I). In terms of total energy, the calculation was automatically derived from the program of the testing device, representing the area under the force-strain graph. The total energy (J) and maximum energy (J) values were found to be approximately 18 and 27% higher, respectively, in the quercetin group test results compared to the control group (Figures 5C,D). One of the most critical mechanical parameters in biomechanical tests is ultimate stress. The results of this study indicated that the average ultimate stress values of the quercetin group samples were approximately 7% higher than those of the control group (Figure 5H). Stiffness refers to the resistance of a specimen against deformation when subjected to a mechanical force. It is determined by the gradient of the force-strain curve within the ‘linear’ zone. In the present study, stiffness value was calculated to be approximately 20% higher in the quercetin group compared to the control group (Figure 5G). This outcome suggests a positive healing effect of quercetin in the experimental group.
Figure 5. The comparison of biomechanical parameters in both groups: (A) failure load (N), (B) displacement (mm), (C) maximum energy (J), (D) Total energy (J), (E) Cross-sectional area (mm2), (F) Length (mm), (G) Stiffness (N/mm), (H) Ultimate stress (MPa), (I) Strain (%).
Quercetin remains a subject of ongoing research interest due to its diverse biological functions, including antioxidative, anti-inflammatory, and anticarcinogenic properties, among others. However, upon conducting our literature review, we identified a notable gap: there is currently no study investigating the healing effects of quercetin in a tendon rupture model. Building upon this observation, the primary aim of our study was to assess the impact of quercetin on tendon healing, filling this gap in the existing literature. Through this investigation, we sought to contribute valuable insights into the potential therapeutic role of quercetin in tendon injuries.
Tendon injuries are traumas frequently encountered by orthopedic surgeons that cause individual pain, disability, and institutional health expenditures (47). Although significant progress has been made in the treatment of tendon injuries with the advancing technology and developments in medicine, unsatisfactory results are still encountered in some patients. It is necessary to search for agents that can be obtained from natural products such as quercetin, which will increase tendon regeneration (8).
In our study, we utilized a total Achilles tendon injury model to investigate the healing effects of oral quercetin on this tendon. We evaluated four different histochemical parameters (tenocyte, collagen, vascularity, and ground substance) comprising the Bonar scoring system, as well as four different immunohistochemical parameters (type I collagen, type III collagen, SMA, and Galectin-3). The results indicated that all these parameters were significantly higher in the quercetin group compared to the control group. Furthermore, biomechanical tests were conducted wherein the Achilles tendons were subjected to tearing from the repaired site. Among the six biomechanical parameters measured, the quercetin group exhibited significantly higher values than the control group. Notably, there was no significant difference between the two groups in the remaining three parameters. Of particular importance, ‘failure load’ and ‘tendon rupture displacement,’ which are crucial clinical indicators, were found to be significantly higher in the quercetin group. The significant improvements observed in these biomechanical parameters, along with the histopathological and immunohistochemical data, suggest that quercetin supplementation may facilitate early mobilization, improve range of motion, and reduce adhesion formation. These findings highlight the potential therapeutic benefits of quercetin in promoting tendon healing and enhancing functional outcomes.
In their study on mice, Siddiqi and Zargar stated that quercetin led to an increase in collagen and hydroxyproline levels in the liver (48). Liang et al. (10) evaluated tendon adhesion after Achilles tendon injury in rats. They found that tendon adhesion was less in rats given quercetin. They reported that the reason for this is that quercetin, which is a powerful antioxidant, reduces the effectiveness of reactive oxygen species, which are the main mediators in fibrogenesis. In our study, we conducted Galectin-3 immunohistochemical staining to evaluate fibrogenesis, and we found a significant increase in the quercetin group compared to the control group. This indicates that quercetin inhibits fibrosis in tendon healing, which is supported by Liang et al.’s study. The higher levels of collagen in the quercetin group and the higher force required for tendon rupture in biomechanical tests may suggest tissue regeneration instead of fibrosis. Both Liang et al.’s study and our study strengthen the hypothesis that quercetin has positive effects on tendon healing due to its antioxidative properties.
Quercetin is found in the traditional Chinese herbal medicine Hippophae rhamnoides, along with isorhamnetin and kaempferol. It is known that this herbal medicine is used in the treatment of tendon injuries in Far Eastern societies (49, 50). Fu et al. (7) evaluated the effect of Hippophae rhamnoides in rats with a patellar tendon injury model. They stated that it supports the ultimate stress in patellar tendon healing by positively affecting the cytokine profile and matrix deposition in the tendon regeneration region, but further studies should be done due to the presence of more than one component in Hippophae rhamnoides. We investigated the effect of quercetin, one of the components of Hippophae rhamnoides, on tendon healing and found that quercetin increased ultimate stress in the healing of the Achilles tendon. We believe that these natural products, which are widely used in the Far East, should be given more place in the modern medical literature.
A clinical phase-1 study has reported that quercetin inhibits tyrosine kinases, which are enzymatic receptors involved in cell signaling pathways, and exhibits antineoplastic properties. In phase-2 trials, bolus intravenous administration of 2.5 g/70 kg quercetin at weekly or three-week intervals has been recommended (51, 52). Additionally, studies have suggested the use of 500 mg/day quercetin supplementation, administered twice daily for 1 month, in the treatment of prostatitis and interstitial cystitis (53, 54). We think that similar clinical studies focusing on musculoskeletal diseases should also be conducted in the future, as quercetin might yield promising therapeutic results in this area. However, prior investigations focusing on the signaling pathways involved in Achilles tendon healing, including comprehensive in vivo and in vitro studies using various animal models, are essential to provide a solid foundation for subsequent clinical trials.
The most important limitation of our study was the lack of literature information to compare our study, since no animal studies or human clinical trials on tendon healing of quercetin have been conducted before. Another limiting aspect of the study is the lack of quantitative molecular analyses of quercetin in both in vivo and in vitro settings. However, given our current knowledge, this study, being the first to examine the effect of quercetin on tendon healing, has yielded promising results for future molecular-level investigations.
It was observed that quercetin, which is easily accessible due to its widespread availability in nature and low cost, significantly increased tendon healing both biomechanically and immunohistochemically. However, since this study is the first to examine the role of quercetin in tendon healing, further investigations, including comprehensive in vivo and in vitro studies on the underlying mechanisms and signaling pathways involved in tendon healing, are necessary to establish a solid foundation before clinical studies can be conducted to evaluate its effects on humans.
The raw data supporting the conclusions of this article will be made available by the authors, without undue reservation.
The animal study was approved by Selçuk University Experimental Medicine Research and Application Center Ethics Committee. The study was conducted in accordance with the local legislation and institutional requirements.
AYu: Conceptualization, Data curation, Methodology, Project administration, Visualization, Writing – original draft, Writing – review & editing. NM: Conceptualization, Methodology, Project administration, Visualization, Writing – original draft, Writing – review & editing. ZC: Data curation, Methodology, Project administration, Supervision, Writing – review & editing. HY: Conceptualization, Data curation, Supervision, Writing – review & editing. AYı: Methodology, Project administration, Supervision, Writing – review & editing.
The author(s) declare that no financial support was received for the research, authorship, and/or publication of this article.
The authors declare that the research was conducted in the absence of any commercial or financial relationships that could be construed as a potential conflict of interest.
The authors declare that no Generative AI was used in the creation of this manuscript.
All claims expressed in this article are solely those of the authors and do not necessarily represent those of their affiliated organizations, or those of the publisher, the editors and the reviewers. Any product that may be evaluated in this article, or claim that may be made by its manufacturer, is not guaranteed or endorsed by the publisher.
1. Saltzman, CL, and Tearse, DS. Achilles tendon injuries. J Am Acad Orthop Surg. (1998) 6:316–25. doi: 10.5435/00124635-199809000-00007
2. Raikin, SM, Garras, DN, and Krapchev, PV. Achilles tendon injuries in a United States population. Foot Ankle Int. (2013) 34:475–80. doi: 10.1177/1071100713477621
3. Gulati, V, Jaggard, M, al-Nammari, SS, Uzoigwe, C, Gulati, P, Ismail, N, et al. Management of achilles tendon injury: a current concepts systematic review. World J Orthop. (2015) 6:380–6. doi: 10.5312/wjo.v6.i4.380
4. Egger, AC, and Berkowitz, MJ. Achilles tendon injuries. Curr Rev Musculoskelet Med. (2017) 10:72–80. doi: 10.1007/s12178-017-9386-7
5. Sarıkaya, B, Yumuşak, N, Yigin, A, Sipahioğlu, S, Yavuz, Ü, and Altay, MA. Comparison of the effects of human recombinant epidermal growth factor and platelet-rich plasma on healing of rabbit patellar tendon. Eklem Hastalik Cerrahisi. (2017) 28:92–9. doi: 10.5606/ehc.2017.55396
6. Ozer, H, Taşkesen, A, Kul, O, Selek, HY, Turanlı, S, and Köse, K. Effect of glucosamine chondroitine sulphate on repaired tenotomized rat Achilles tendons. Eklem Hastalik Cerrahisi. (2011) 22:100–6.
7. Fu, SC, Hui, CW, Li, LC, Cheuk, YC, Qin, L, Gao, J, et al. Total flavones of Hippophae rhamnoides promotes early restoration of ultimate stress of healing patellar tendon in a rat model. Med Eng Phys. (2005) 27:313–21. doi: 10.1016/j.medengphy.2004.12.011
8. Jiang, D, Gao, P, Lin, H, and Geng, H. Curcumin improves tendon healing in rats: a histological, biochemical, and functional evaluation. Connect Tissue Res. (2016) 57:20–7. doi: 10.3109/03008207.2015.1087517
9. Saccol, R, da Silveira, KL, Manzoni, AG, Abdalla, FH, de Oliveira, JS, Dornelles, GL, et al. Antioxidant, hepatoprotective, genoprotective, and cytoprotective effects of quercetin in a murine model of arthritis. J Cell Biochem. (2020) 121:2792–801. doi: 10.1002/jcb.29502
10. Liang, Y, Xu, K, Zhang, P, Zhang, J, Chen, P, He, J, et al. Quercetin reduces tendon adhesion in rat through suppression of oxidative stress. BMC Musculoskelet Disord. (2020) 21:608. doi: 10.1186/s12891-020-03618-2
11. Shahbaz, M, Naeem, H, Momal, U, Imran, M, Alsagaby, SA, Al Abdulmonem, W, et al. Anticancer and apoptosis inducing potential of quercetin against a wide range of human malignancies. International Journal of Food Properties. (2023) 26:2590–626. doi: 10.1080/10942912.2023.2252619,
12. Lokman, MS, Althagafi, HA, Alharthi, F, Habotta, OA, Hassan, AA, Elhefny, MA, et al. Protective effect of quercetin against 5-fluorouracil-induced cardiac impairments through activating Nrf2 and inhibiting NF-κB and caspase-3 activities. Environ Sci Pollut Res Int. (2023) 30:17657–69. doi: 10.1007/s11356-022-23314-z
13. Gumus, E, Sisko, A, Abas, BI, Demirkan, B, and Cevik, O. Quercetin protects mouse oocytes against chromium-induced damage in vitro and in vivo. J Trace Elem Med Biol. (2023) 75:127087. doi: 10.1016/j.jtemb.2022.127087
14. Otaki, A, Furuta, A, and Asano, KJM. Quercetin-induced enhancement of nasal epithelial cells’ ability to produce clara cell 10-kD protein in vitro and in vivo. Medicines. (2023) 10:28. doi: 10.3390/medicines10040028
15. Wang, S, Jin, X, Chen, H, Han, M, Bao, J, Niu, D, et al. Quercetin alleviates Mycoplasma gallisepticum-induced inflammatory damage and oxidative stress through inhibition of TLR2/MyD88/NF-κB pathway in vivo and in vitro. Microb Pathog. (2023) 176:106006. doi: 10.1016/j.micpath.2023.106006
16. Hashemzaei, M, Delarami Far, A, Yari, A, Heravi, RE, Tabrizian, K, Taghdisi, SM, et al. Anticancer and apoptosis-inducing effects of quercetin in vitro and in vivo. Oncol Rep. (2017) 38:819–28. doi: 10.3892/or.2017.5766
17. Shi, G-J, Li, Y, Cao, Q-H, Wu, H-X, Tang, X-Y, Gao, X-H, et al. In vitro and in vivo evidence that quercetin protects against diabetes and its complications: a systematic review of the literature. Biomed Pharmacother. (2019) 109:1085–99. doi: 10.1016/j.biopha.2018.10.130
18. Kleemann, R, Verschuren, L, Morrison, M, Zadelaar, S, Van Erk, MJ, Wielinga, PY, et al. Anti-inflammatory, anti-proliferative and anti-atherosclerotic effects of quercetin in human in vitro and in vivo models. Atherosclerosis. (2011) 218:44–52. doi: 10.1016/j.atherosclerosis.2011.04.023
19. Wang, S, Yao, J, Zhou, B, Yang, J, Chaudry, MT, Wang, M, et al. Bacteriostatic effect of quercetin as an antibiotic alternative in vivo and its antibacterial mechanism in vitro. J Food Prot. (2018) 81:68–78. doi: 10.4315/0362-028X.JFP-17-214
20. Nickel, T, Hanssen, H, Sisic, Z, Pfeiler, S, Summo, C, Schmauss, D, et al. Immunoregulatory effects of the flavonol quercetin in vitro and in vivo. Eur J Nutr. (2011) 50:163–72. doi: 10.1007/s00394-010-0125-8
21. Hatahet, T, Morille, M, Hommoss, A, Devoisselle, J, Müller, R, Bégu, S, et al. Quercetin topical application, from conventional dosage forms to nanodosage forms. Eur J Pharm Biopharm. (2016) 108:41–53. doi: 10.1016/j.ejpb.2016.08.011
22. Azeem, M, Hanif, M, Mahmood, K, Ameer, N, Chughtai, FRS, and Abid, UJPB. An insight into anticancer, antioxidant, antimicrobial, antidiabetic and anti-inflammatory effects of quercetin: a review. Polym Bull. (2023) 80:241–62. doi: 10.1007/s00289-022-04091-8
23. Solnier, J, Zhang, Y, Roh, K, Kuo, YC, Du, M, Wood, S, et al. A pharmacokinetic study of different quercetin formulations in healthy participants: a diet-controlled, crossover, single-and multiple-dose pilot study. Evid Based Complement Alternat Med. (2023) 2023:2023. doi: 10.1155/2023/9727539
24. Sun, N, Jiang, X, Meng, Q, Jiang, H, Yuan, Z, Zhang, JJAB, et al. Preparation of nanoparticles loaded with quercetin and effects on bacterial biofilm and LPS-induced oxidative stress in Dugesia japonica. Appl Biochem Biotechnol. (2024) 196:32–49. doi: 10.1007/s12010-023-04543-5
25. Asgharian, P, Tazekand, AP, Hosseini, K, Forouhandeh, H, Ghasemnejad, T, Ranjbar, M, et al. Potential mechanisms of quercetin in cancer prevention: focus on cellular and molecular targets. Cancer Cell Int. (2022) 22:257. doi: 10.1186/s12935-022-02677-w
26. Eno, EA, Shagal, MH, Godfrey, OC, Ngana, OC, Ekong, JE, Benjamin, I, et al. Computational study of the interaction of metal ions (Na+, K+, Mg2+, Ca2+, and Al3+) with quercetin and its antioxidant properties. J. Indian Chem. Soc. (2023) 100:101059. doi: 10.1016/j.jics.2023.101059
27. Chanu, NR, Gogoi, P, Barbhuiya, PA, Dutta, PP, Pathak, MP, and Sen, S. Natural flavonoids as potential therapeutics in the management of diabetic wound: a review. Curr Top Med Chem. (2023) 23:690–710. doi: 10.2174/1568026623666230419102140
28. Chen, T, Zhang, X, Zhu, G, Liu, H, Chen, J, Wang, Y, et al. Quercetin inhibits TNF-α induced HUVECs apoptosis and inflammation via downregulating NF-kB and AP-1 signaling pathway in vitro. Medicine. (2020) 99:e22241. doi: 10.1097/MD.0000000000022241
29. Lai, W-W, Hsu, S-C, Chueh, F-S, Chen, Y-Y, Yang, J-S, Lin, J-P, et al. Quercetin inhibits migration and invasion of SAS human oral cancer cells through inhibition of NF-κB and matrix metalloproteinase-2/−9 signaling pathways. Anticancer Res. (2013) 33:1941–50.
30. Lee, HL, Kim, JM, Go, MJ, Kim, TY, Joo, SG, Kim, JH, et al. Protective effect of Lonicera japonica on PM2. 5-induced pulmonary damage in BALB/c mice via the TGF-β and NF-κB pathway. Antioxidants. (2023) 12:968. doi: 10.3390/antiox12040968
31. Vigneshwaran, S, Maharani, K, Sivasakthi, P, Selvan, PS, Saraswathy, S, and Priya, ESJI. Bioactive fraction of Tragia involucrata Linn leaves attenuates inflammation in Freund’s complete adjuvant-induced arthritis in Wistar albino rats via inhibiting NF-κB. Inflammopharmacology. (2023) 31:967–81. doi: 10.1007/s10787-023-01154-8
32. Wang, W, Yuan, X, Mu, J, Zou, Y, Xu, L, Chen, J, et al. Quercetin induces MGMT+ glioblastoma cells apoptosis via dual inhibition of Wnt3a/β-catenin and Akt/NF-κB signaling pathways. Phytomedicine. (2023) 118:154933. doi: 10.1016/j.phymed.2023.154933
33. Maganaris, CN, Narici, MV, and Maffulli, N. Biomechanics of the Achilles tendon. Disabil Rehabil. (2008) 30:1542–7. doi: 10.1080/09638280701785494
34. Müller, SA, Evans, CH, Heisterbach, PE, and Majewski, M. The role of the paratenon in Achilles tendon healing: a study in rats. Am J Sports. (2018) 46:1214–9. doi: 10.1177/0363546518756093
35. Yurteri, A, Mercan, N, Çelik, M, Doğar, F, Kılıç, M, Yıldırım, AJJD, et al. The effect of caffeic acid on tendon healing in rats with an Achilles tendon injury model. Jt Dis Relat Surg. (2023) 34:669–78. doi: 10.52312/jdrs.2023.1248
36. Lee, S-Y, Chieh, H-F, Lin, C-J, Jou, I-M, Sun, Y-N, Kuo, L-C, et al. Characteristics of sonography in a rat Achilles tendinopathy model: possible non-invasive predictors of biomechanics. Sci Rep. (2017) 7:5100. doi: 10.1038/s41598-017-05466-y
37. Baghel, SS, Shrivastava, N, Baghel, RS, Agrawal, P, and Rajput, SRS. A review of quercetin: antioxidant and anticancer properties. World Journal of Pharmacy and Pharmaceutical Sciences. (2012) 1:146–60.
38. Baruah, MM, Khandwekar, AP, and Sharma, N. Quercetin modulates Wnt signaling components in prostate cancer cell line by inhibiting cell viability, migration, and metastases. Tumour Biol. (2016) 37:14025–34. doi: 10.1007/s13277-016-5277-6
39. Semis, HS, Gur, C, Ileriturk, M, Kandemir, FM, and Kaynar, O. Evaluation of therapeutic effects of quercetin against achilles tendinopathy in rats via oxidative stress, inflammation, apoptosis, autophagy, and metalloproteinases. Am J Sports Med. (2022) 50:486–98. doi: 10.1177/03635465211059821
40. Yoshikawa, T, Mifune, Y, Inui, A, Nishimoto, H, Yamaura, K, Mukohara, S, et al. Quercetin treatment protects the Achilles tendons of rats from oxidative stress induced by hyperglycemia. BMC Musculoskelet Disord. (2022) 23:1–13. doi: 10.1186/s12891-022-05513-4
41. Eren, Y, Adanır, O, Dinçel, YM, Genç, E, Arslan, YZ, and Çağlar, A. Effects of low molecular weight heparin and rivaroxaban on rat Achilles tendon healing. Eklem Hastalik Cerrahisi. (2018) 29:13–9. doi: 10.5606/ehc.2018.54577
42. Aydın, BK, Altan, E, Acar, MA, Erkoçak, ÖF, and Ugraş, S. Effect of Ankaferd blood stopper® on tendon healing: an experimental study in a rat model of Achilles tendon injury. Eklem Hastali Cerrahisi. (2015) 26:31–7. doi: 10.5606/ehc.2015.08
43. Niu, YB, Yang, YY, Xiao, X, Sun, Y, Zhou, YM, Zhang, YH, et al. Quercetin prevents bone loss in hindlimb suspension mice via stanniocalcin 1-mediated inhibition of osteoclastogenesis. Acta Pharmacol Sin. (2020) 41:1476–86. doi: 10.1038/s41401-020-00509-z
44. Maffulli, N, Longo, UG, Franceschi, F, Rabitti, C, and Denaro, V. Movin and Bonar scores assess the same characteristics of tendon histology. Clin Orthop Relat Res. (2008) 466:1605–11. doi: 10.1007/s11999-008-0261-0
45. Fearon, A, Dahlstrom, JE, Twin, J, Cook, J, and Scott, A. The Bonar score revisited: region of evaluation significantly influences the standardized assessment of tendon degeneration. J Sci Med Sport. (2014) 17:346–50. doi: 10.1016/j.jsams.2013.07.008
46. Zabrzyński, J, Gagat, M, Huri, G, Łapaj, Ł, Paczesny, Ł, Zielińska, W, et al. Therapeutic advances in tendinopathy quantified microscopically using Bonar score, with a special reference to PRP therapy—a systematic review of experimental. Studies. (2021) 11:4973. doi: 10.3390/app11114973
47. Thomopoulos, S, Parks, WC, Rifkin, DB, and Derwin, KA. Mechanisms of tendon injury and repair. J Orthop Res. (2015) 33:832–9. doi: 10.1002/jor.22806
48. Siddiqi, NJ, and Zargar, S. Effect of quercetin on cadmium fluoride-induced alterations in hydroxyproline/collagen content in mice liver. Connect Tissue Res. (2014) 55:234–8. doi: 10.3109/03008207.2014.900551
49. Yang, F, Quan, J, Zhang, TY, and Ito, Y. Multidimensional counter-current chromatographic system and its application. J Chromatogr A. (1998) 803:298–301. doi: 10.1016/S0021-9673(97)01273-9
50. Xing, J, Yang, B, Dong, Y, Wang, B, Wang, J, and Kallio, HP. Effects of sea buckthorn (Hippophaë rhamnoides L.) seed and pulp oils on experimental models of gastric ulcer in rats. Fitoterapia. (2002) 73:644–50. doi: 10.1016/S0367-326X(02)00221-6
51. Ferry, DR, Smith, A, Malkhandi, J, Fyfe, DW, deTakats, PG, Anderson, D, et al. Phase I clinical trial of the flavonoid quercetin: pharmacokinetics and evidence for in vivo tyrosine kinase inhibition. Clin Cancer Res. (1996) 2:659–68.
52. Okamoto, T. Safety of quercetin for clinical application (review). Int J Mol Med. (2005) 16:275–8. doi: 10.3892/ijmm.16.2.275
53. Shoskes, DA, Zeitlin, SI, Shahed, A, and Rajfer, J. Quercetin in men with category III chronic prostatitis: a preliminary prospective, double-blind, placebo-controlled trial. Urology. (1999) 54:960–3. doi: 10.1016/S0090-4295(99)00358-1
Keywords: biomechanical, histopathological, immunohistochemical, polyphenols, quercetin
Citation: Yurteri A, Mercan N, Çelik ZE, Yaykaşlı H and Yıldırım A (2025) Does quercetin affect tendon healing? An experimental study in a rat model of Achilles tendon injury. Front. Med. 12:1522517. doi: 10.3389/fmed.2025.1522517
Received: 04 November 2024; Accepted: 24 January 2025;
Published: 05 February 2025.
Edited by:
Federica Fulceri, University of Pisa, ItalyReviewed by:
Yanchun Wang, Banan People’s Hospital of Chongqing, ChinaCopyright © 2025 Yurteri, Mercan, Çelik, Yaykaşlı and Yıldırım. This is an open-access article distributed under the terms of the Creative Commons Attribution License (CC BY). The use, distribution or reproduction in other forums is permitted, provided the original author(s) and the copyright owner(s) are credited and that the original publication in this journal is cited, in accordance with accepted academic practice. No use, distribution or reproduction is permitted which does not comply with these terms.
*Correspondence: Numan Mercan, bnVtYW5tZXJjYW5AZ21haWwuY29t
Disclaimer: All claims expressed in this article are solely those of the authors and do not necessarily represent those of their affiliated organizations, or those of the publisher, the editors and the reviewers. Any product that may be evaluated in this article or claim that may be made by its manufacturer is not guaranteed or endorsed by the publisher.
Research integrity at Frontiers
Learn more about the work of our research integrity team to safeguard the quality of each article we publish.