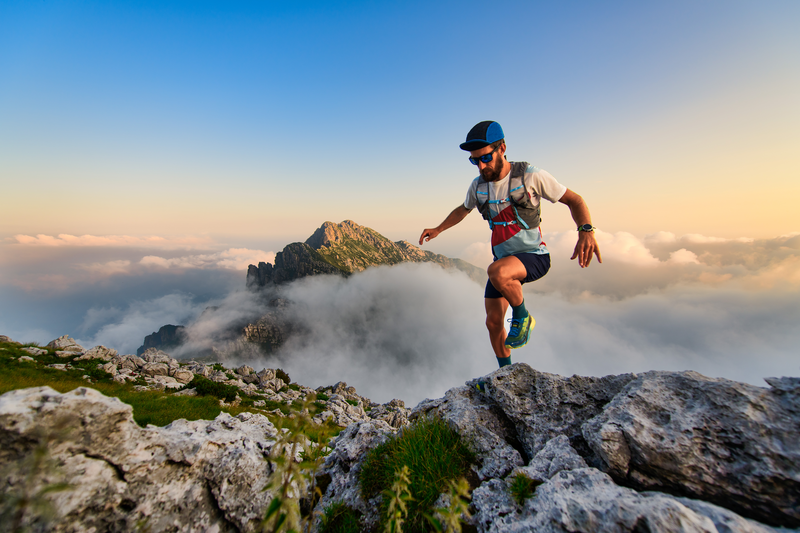
94% of researchers rate our articles as excellent or good
Learn more about the work of our research integrity team to safeguard the quality of each article we publish.
Find out more
ORIGINAL RESEARCH article
Front. Med.
Sec. Hepatobiliary Diseases
Volume 12 - 2025 | doi: 10.3389/fmed.2025.1513233
This article is part of the Research Topic Genomic Dissection and Therapy Development in Liver Cancer View all articles
The final, formatted version of the article will be published soon.
You have multiple emails registered with Frontiers:
Please enter your email address:
If you already have an account, please login
You don't have a Frontiers account ? You can register here
Background Hepatocellular carcinoma (HCC) is the third most prevalent cause of cancer-related mortality globally and the sixth most common cancer overall. It is critical to investigate new biomarkers and prognostic variables because there are currently no early diagnostic indicators. Actin-related proteins (ARPs) are involved in transcriptional regulation, chromatin remodeling, and DNA repair-all processes that have been connected to the development of cancer. However, it's still unclear how ARPs and HCC are related.Methods Through the examination of databases like The Cancer Genome Atlas (TCGA) and The International Cancer Genome Consortium (ICGC), we examined the variations in the expression of ARPs between the transcriptomes of normal tissue and HCC. Furthermore, univariate and multivariate Cox analysis were used to assess the prognostic effects of ARPs. The investigation of immune cell infiltration and possible functional enrichment followed. Additionally, tissue chips containing regional liver cancer specimens were used to confirm ACTR6 expression and the clinical impact of prognosis using an immunohistochemistry (IHC) test. Finally, to investigate the expression and function of ACTR6 in liver cancer cells, real-time qPCR (RT-qPCR) assays, CCK-8, clone creation, cell cycle, and transwell migration and invasion experiments were carried out.We found that, in addition to ACTR3C, 17 ARPs were significantly overexpressed in HCC compared with normal tissues. In both univariate and multivariate Cox models, ACTR6 and ACTL6A were identified as potential independent risk factors for the prognosis of HCC, with ACTR6 having the lowest p-value. Clinical samples also confirmed this conclusion. Furthermore, ACTR6 overexpression showed a strong connection with immune cell infiltration levels and clinical and pathological factors linked to a poor prognosis. Functionally, knocking down ACTR6 inhibited cell migration and proliferation, produced a G1 cell cycle arrest, and decreased the viability of liver cancer cells.These findings demonstrate that ACTR6 is highly expressed in HCC and is associated with poor prognosis. In addition, ACTR6 may induce immune cell infiltration and promote hepatocarcinogenesis by regulating the cell cycle.
Keywords: Hepatocellular Carcinoma, Actin-related protein family, ACTR6, Cell Cycle, Immune infiltration
Received: 18 Oct 2024; Accepted: 20 Feb 2025.
Copyright: © 2025 Wang, Song, Tang, Yue, Guo, Chen, Shen and Cao. This is an open-access article distributed under the terms of the Creative Commons Attribution License (CC BY). The use, distribution or reproduction in other forums is permitted, provided the original author(s) or licensor are credited and that the original publication in this journal is cited, in accordance with accepted academic practice. No use, distribution or reproduction is permitted which does not comply with these terms.
* Correspondence:
Mingbo Cao, People's Hospital of Zhengzhou University, Zhengzhou, China
Disclaimer: All claims expressed in this article are solely those of the authors and do not necessarily represent those of their affiliated organizations, or those of the publisher, the editors and the reviewers. Any product that may be evaluated in this article or claim that may be made by its manufacturer is not guaranteed or endorsed by the publisher.
Research integrity at Frontiers
Learn more about the work of our research integrity team to safeguard the quality of each article we publish.