- 1Eye Center of the 2nd Affiliated Hospital, School of Medicine, Zhejiang University, Hangzhou, Zhejiang, China
- 2Zhejiang Provincial Key Lab of Ophthalmology, Hangzhou, Zhejiang, China
Purpose: To report a singular case of cataract caused by toad venom inoculation and to scrutinize the pathological mechanisms through proteomic sequencing of the lens specimen.
Methods: A young Chinese male presented with progressively deteriorating vision in his right eye subsequent to a history of toad venom inoculation. He was diagnosed with a toxic cataract, and underwent phacoemulsification cataract surgery. Anterior capsule, nucleus, and cortex specimens from the patient (designated as PT_CAP, PT_PHACO, and PT_CTX, respectively) and age-related cataract controls (C_CAP, C_PHACO, and C_CTX, respectively) were collected and subjected to 4D label-free quantitative proteomics.
Results: A multitude of differentially expressed proteins (DEPs) were identified in the patient’s lens compared to those in the controls. Specifically, a total of 204 DEPs were identified in PT_CAP compared to C_CAP, with MYH6, MYL2, MYL3, STAT1, and ANK1 among the foremost regulated DEPs. The DEPs of PT_CAP were principally affiliated with functions including “transportation of small molecules,” “regulation of metal ion transport,” and “import into cell.” A sum of 109 DEPs were delineated in PT_CTX compared to C_CTX, with TPM1 among the top-10 downregulated DEPs. Ninety-five DEPs were pinpointed in PT_PHACO compared to C_PHACO, with hexokinase among the top 10 downregulated DEPs. These proteins were ascertained to be linked with Na+/K+-ATPase activity.
Conclusion: This study introduced the first documented case of toxic cataract caused by toad venom inoculation. Proteomic sequencing indicated a correlation between cataract and alterations in Na+/K+-ATPase activity, providing insights for the clinical management of ocular toad venom inoculation in subsequent cases.
Introduction
Toad venom represents a frequently encountered animal toxin capable of inducing both local and systemic toxicity. Comprised of bufadienolides synthesized by the parotid glands and skin, it serves as a potential inhibitor of the sodium-potassium adenosine triphosphatase (Na+/K+-ATPase) pump. Bufotoxin, a principal toxic component, exhibits effects akin to those of digitalis (1). Systemic manifestations of toad venom poisoning encompass cyanosis, paralysis, seizures, increased salivation, vomiting, hyperkalemia, and hallucinations (1). Direct ocular exposure to toad venom may incite acute ocular toxicity, termed toad venom inoculation, manifesting as clinical symptoms and signs such as chemosis, conjunctivitis, keratitis, stromal corneal edema with Descemet’s folds, corneal dysfunction, and ocular hypotonia (1–3). In severe cases, local ocular mucosal absorption can precipitate systemic responses such as hypertension, bradycardia, and arterial constriction (4). Nonetheless, reports pertaining to the long-term ocular toxicity of toad venom remain scarce.
The present article elucidated a distinctive case of cataract resulting from toad venom exposure in our clinical observation. We conducted proteomic sequencing of the lens specimen from the affected eye to explore alterations in protein expression across various portions of the lens induced by toad venom. Our objective was to delineate the pathological mechanisms underlying cataracts triggered by toad venom inoculation and to establish a foundation for future treatment approaches for ocular toad venom exposure.
Case report
A 29-year-old Chinese male, henceforth referred to as the patient, presented to our ophthalmology clinic reporting progressively deteriorating vision in his right eye over a span of 2 years. The patient recounted a prior incident wherein toad venom had inadvertently splashed into his right eye, resulting in transient symptoms of redness and irritation. Upon examination, his best-corrected distance visual acuity (BCDVA) was 5/20 in the right eye and 20/20 in the left eye. Slit lamp microscopy evaluation of the right eye revealed noticeable posterior subcapsular opacity (PCO), graded as C1N0P4 according to the LOCS II classification (Figures 1A–C). Subsequent ocular assessments did not reveal any significant abnormalities. A diagnosis of toxic cataract in the right eye was established.
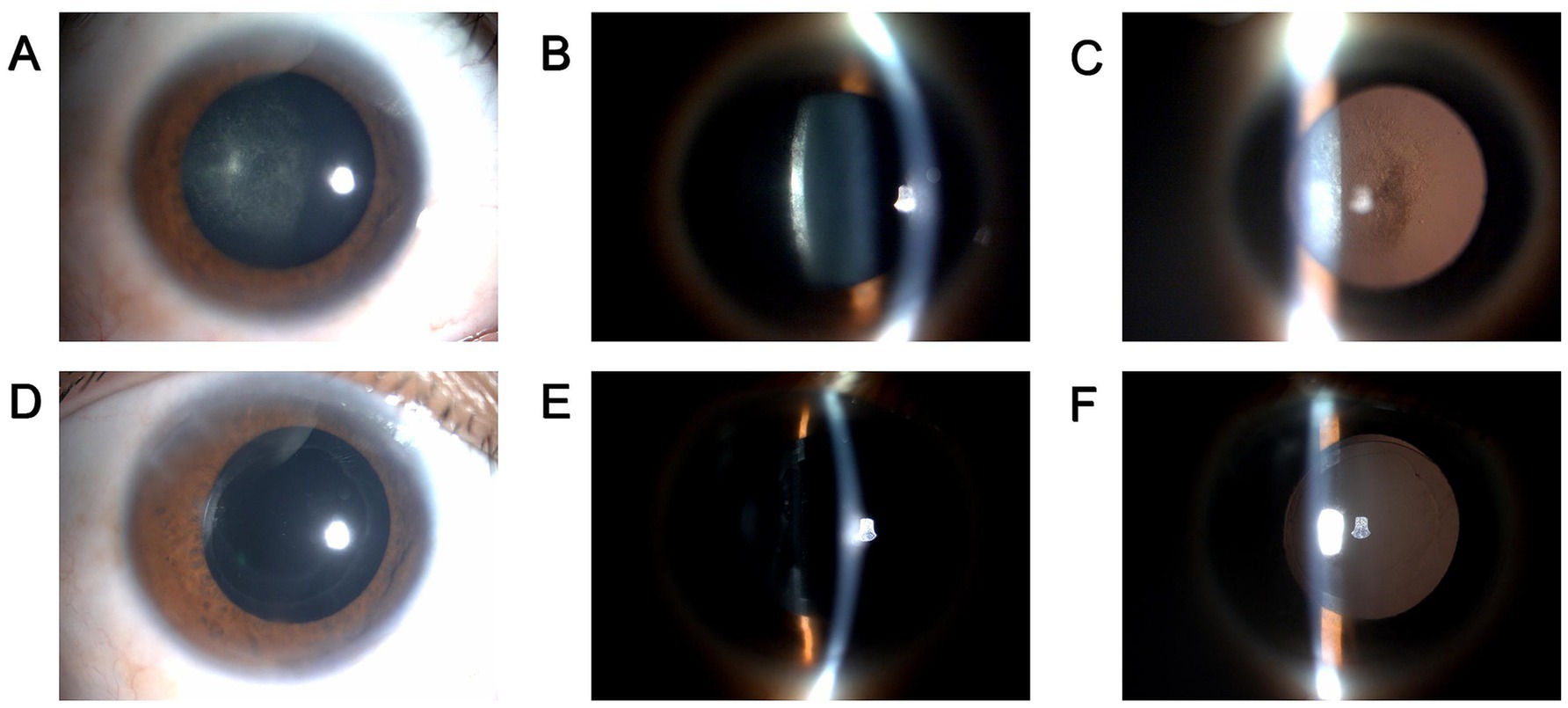
Figure 1. Preoperative and postoperative clinical data of the patient. (A–C) Slit lamp photography of the patient’s right eye before surgery. (A) diffuse illumination; (B) direct focal illumination; (C) retro illumination. (D–F) slit lamp photography of the patient’s right eye at 1 week after surgery. (D) diffuse illumination; (E) direct focal illumination; (F) retro illumination.
The patient underwent standard micro-incision phacoemulsification and intraocular lens (IOL) implantation procedures under topical anesthesia. A sample of the anterior capsule was collected, and the designation PT_CAP was assigned. Phacoemulsification was employed to remove the lens nucleus, and the resulting phacoemulsification fluid was collected and labeled as PT_PHACO, representing the nucleus sample given that the phacoemulsification procedure predominantly targets the nucleus. Subsequent manual irrigation and aspiration techniques were utilized to extract the residual lens cortex, with the collected sample designated as PT_CTX.
During the 1-week postoperative follow-up, clinical evaluation of the operated eye revealed a transparent cornea, a tranquil anterior chamber, and appropriately positioned IOL (Figures 1D–F). The BCDVA in the right eye improved to 20/25. Subsequent ocular examinations did not reveal any noteworthy findings. Owing to the epidemic circumstances, subsequent follow-up visits at 1 month, 3 months, 6 months, and 12 months were conducted at the local eye hospital under our team’s supervision, yielding satisfactory outcomes (details not provided).
Materials and methods
Collection of the clinical data
The study protocol strictly adhered to the ethical principles delineated in the Declaration of Helsinki and obtained approval from the Medical Ethics Committee of the Second Affiliated Hospital of Zhejiang University School of Medicine, Hangzhou, China. The patient underwent standard preoperative and postoperative evaluations, as elaborated in the Supplementary materials. All clinical data were meticulously retrieved from the electronic medical record system, with the patient’s explicit written consent duly obtained.
Surgical procedure and sample collection
The surgical procedure details and sample collection for the patient are meticulously delineated in the Case Report section. Additionally, three patients afflicted with age-related cataracts but devoid of systemic diseases were recruited as the control cohort (hereafter referred to as controls). Employing a standardized protocol identical to that of the patient, regular micro-incision phacoemulsification and IOL implantation procedures were administered to the controls. Samples of the lens anterior capsule, phacoemulsification collection fluid, and lens cortex were collected and designated as follows: Control’s lens anterior capsule samples 1, 2, and 3 (C_CAP 1, C_CAP 2, and C_CAP 3), Control’s phacoemulsification collection fluid samples 1, 2, and 3 (C_PHACO 1, C_PHACO 2, and C_PHACO 3), and Control’s lens cortex samples 1, 2, and 3 (C_CTX 1, C_CTX 2, and C_CTX 3), respectively. The procurement of specimens from both the patient and donors was conducted subsequent to the acquisition of written informed consent.
4D label-free quantitative proteomic analysis
Refer to the Supplementary materials and Methods section for detailed information.
Results
Proteomic analysis of patient’s lens versus controls
Protein extracts originating from distinct components of both the patient’s and controls’ lenses were meticulously collected for 4D Label-Free analysis, as depicted in the flow diagram presented in Figure 2A. Numerous differentially expressed proteins (DEPs) were discerned in the anterior capsule, cortex, and nucleus of the patient’s lens relative to those in the controls (Figure 2B; Supplementary Table S2). Subsequent Gene Ontology (GO), Clusters of Orthologous Groups (COG) function, and Kyoto Encyclopedia of Genes and Genomes (KEGG) pathway analyses were conducted based on all DEPs. The GO categories encompassing biological process (BP), cellular component (CC), and molecular function (MF) are depicted in Figure 2C, elucidating that “oxidation–reduction process,” “intracellular,” and “protein binding” emerged as the most prevalent terms among the BP, CC, and MF categories, respectively. COG and KEGG categories are delineated in Figures 2D,E, respectively. Additionally, a subcellular localization analysis of the DEPs was undertaken, as delineated in Figure 2F.
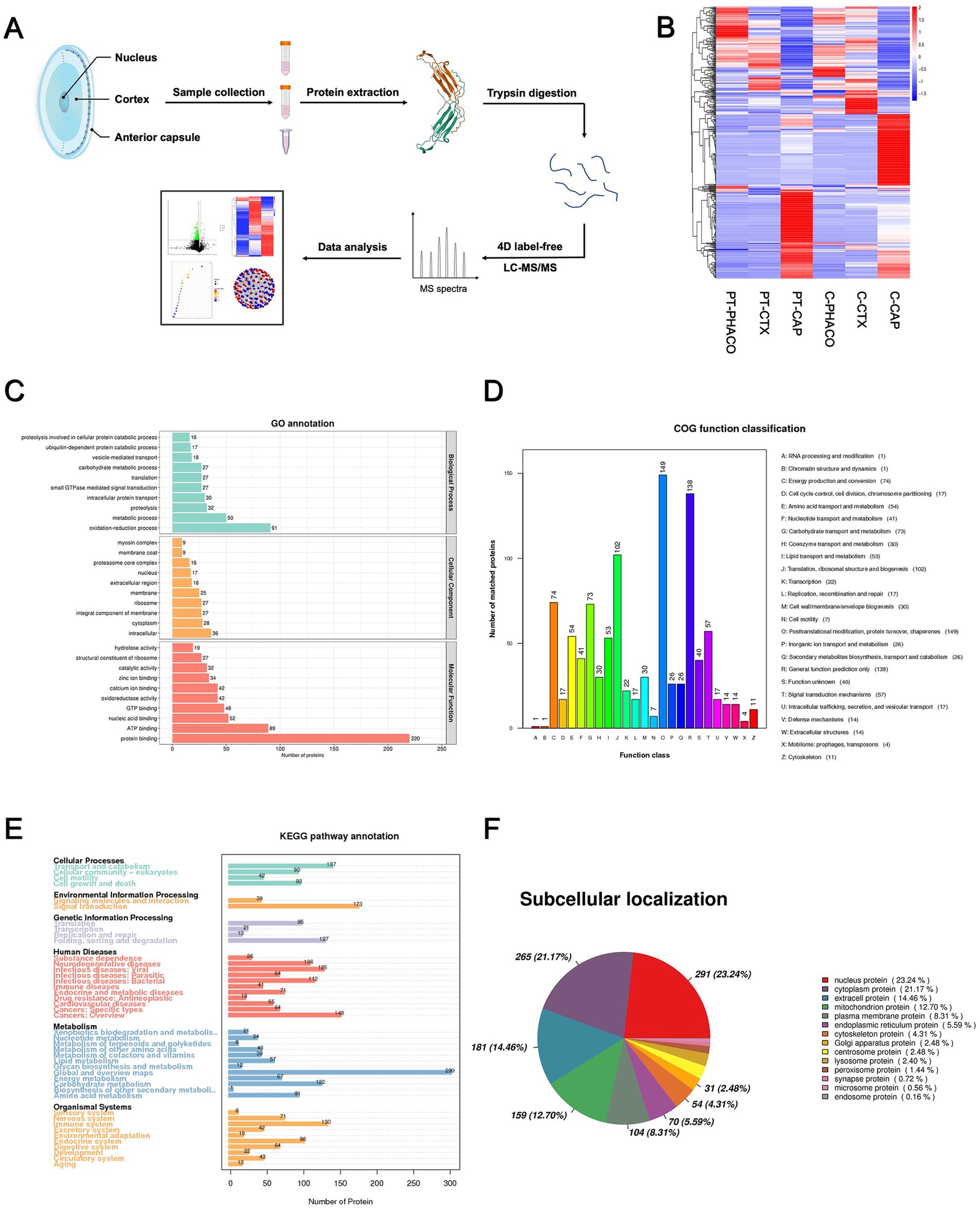
Figure 2. 4D label-free quantitative proteomics analysis of DEPs among all the different groups. (A) Flow diagram of 4D label-free quantitative proteomics analysis. (B) Heatmap generated from a hierarchical cluster analysis, illustrating the different expression profiling between patient’s and controls’ lens specimens. PT_PHACO, patient’s phacoemulsification collect fluid; PT_CTX, patient’s cortex sample; PT_CAP, patient’s anterior capsule sample; C_PHACO, controls’ phacoemulsification collect fluid; C_CTX, controls’ cortex sample; C_CAP, controls’ anterior capsule sample. (C) GO classification of the DEPs in terms of BP, CC, and MF among all the groups. (D) COG function classification of the DEPs among all the groups. (E) Enrichment of KEGG pathways among all the groups. (F) Subcellular localization of the DEPs among all the groups.
Proteomic analysis of anterior capsule of patient’s lens versus controls’ lens
In total, a count of 204 DEPs was recorded, comprising 92 upregulated and 112 downregulated proteins in the patient’s anterior capsule in comparison to controls, as delineated in the volcano plot (Figure 3A). The top-10 upregulated and downregulated DEPs are itemized in Tables 1, 2. The identified proteins were categorized in terms of cellular components based on GO functional analysis, with the DEPs cataloged into 19 GO terms encompassing six BPs, four CCs, and nine MFs (Figure 3B). Notably, “extracellular region” emerged as the most abundant term among the CCs, while “peptidase activity, acting on L-amino acid peptides,” “endopeptidase inhibitor activity,” and “endopeptidase activity” ranked among the top three terms within the MF category. Subsequently, KEGG enrichment analysis was conducted to explore the roles of DEPs in the anterior capsule and their impact on lens opacification (Figure 3C). The outcomes unveiled that the pathways exhibited the highest enrichment in “complement and coagulation cascades.” Moreover, InterPro (IPR) enrichment analysis was undertaken to prognosticate the functional classification of the DEPs through domain annotation, revealing that the DEPs demonstrated the highest enrichment in “immunoglobulin C1-set” (Figure 3D). Lastly, subcellular localization prediction of DEPs was executed, with the majority of proteins localized in the extracellular region (38.92%), followed by the plasma membrane (13.17%), and the nucleus (11.98%) (Figure 3E).
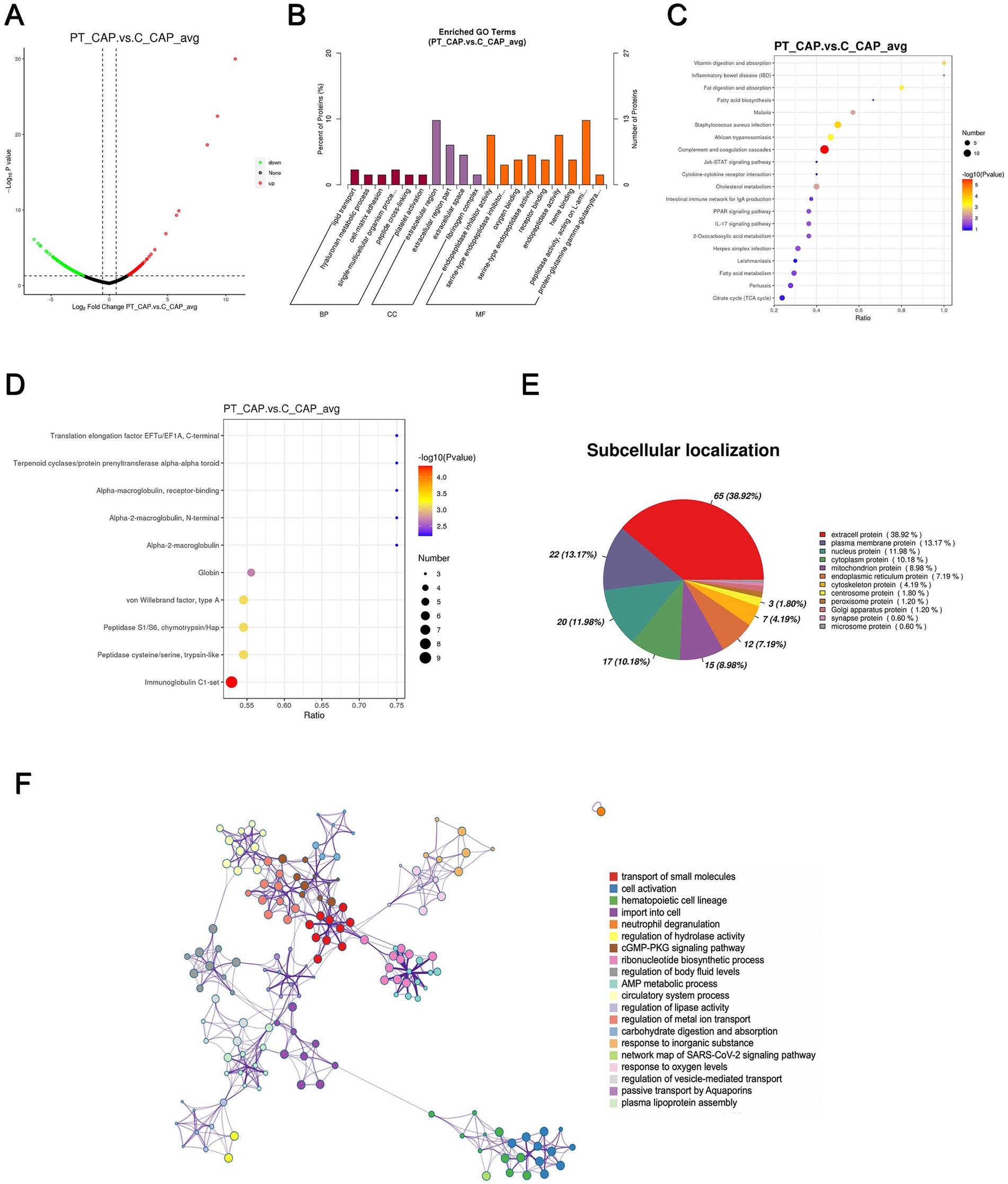
Figure 3. Quantitative proteomics analysis of DEPs between the patient’s anterior capsule sample (PT_CAP) and the controls’ anterior capsule specimens (C_CAP). (A) Volcano plot of DEPs. (B) GO classification of the DEPs between PT_CAP and C_CAP in terms of BP, CC, and MF. (C) Enrichment of KEGG pathways between PT_CAP and C_CAP. (D) IPR functional analysis of the DEPs between PT_CAP and C_CAP. (E) Subcellular localization of the DEPs between PT_CAP and C_CAP. (F) PPI network of DEPs between PT_CAP and C_CAP.
The protein–protein interaction (PPI) network analysis was employed to elucidate the functional interactions among DEPs. As depicted in Figure 3F, the enriched DEP clusters encompassed various functional categories including “transportation of small molecules,” “regulation of metal ion transport,” “circulatory system process,” “ribonucleotide biosynthetic process,” and others. Furthermore, molecular complex detection (MCODE) clusters were utilized to identify densely connected network components, leading to the identification of 19 highly interconnected clusters (Supplementary Figure S1).
Proteomic analysis of the cortex of the patient’s lens compared to the controls’ lens
In total, a count of 109 DEPs, comprising 53 upregulated and 56 downregulated proteins, were observed in the patient’s cortex relative to controls, as illustrated in the volcano plot (Figure 4A). The top-10 upregulated and downregulated DEPs are itemized in Tables 3, 4. GO functional classification (Figure 4B) indicated that the DEPs were categorized into 11 GO terms, including eight BP, one CC, and two MFs. Noteworthy terms within the BPs included “regulation of cellular process” and “signal transduction.” The top-10 KEGG categories are presented in Figure 4C, revealing enrichment in pathways such as “antigen processing and presentation,” “insulin secretion,” and “staphylococcus aureus infection.” IPR enrichment analysis revealed that the DEPs were predominantly enriched in domains such as “ankyrin repeat-containing domain” and “globin” (Figure 4D). Subcellular localization prediction indicated that the majority of proteins were localized in the nucleus (21.79%), cytoplasm (16.67%), and extracellular space (14.10%) (Figure 4E).
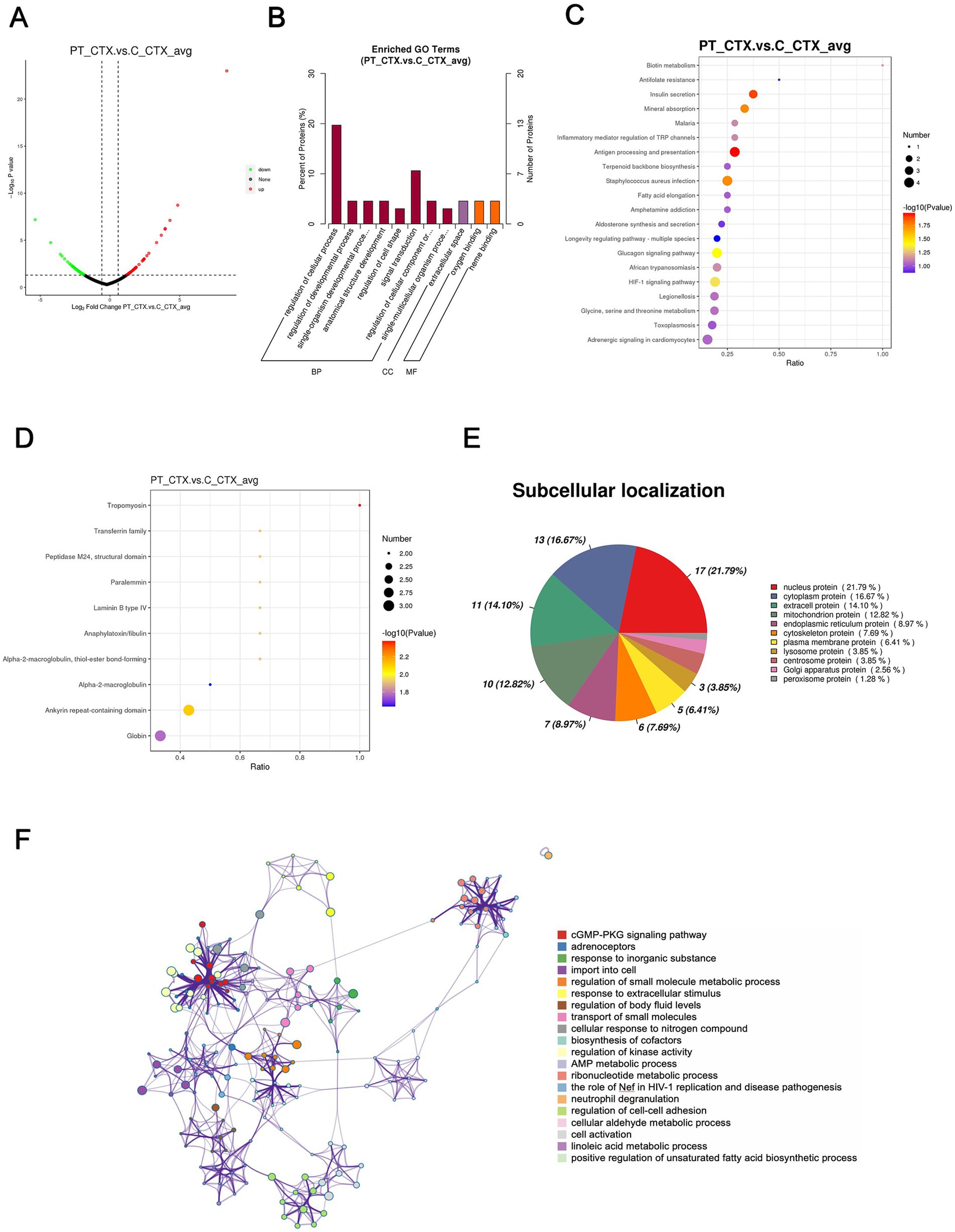
Figure 4. Quantitative proteomics analysis of DEPs between the patient’s cortex sample (PT_CTX) and the controls’ cortex specimens (C_CTX). (A) Volcano plot of DEPs. (B) GO classification of the DEPs between PT_CTX and C_CTX in terms of BP, CC, and MF. (C) Enrichment of KEGG pathways between PT_CTX and C_CTX. (D) IPR functional analysis of the DEPs between PT_CTX and C_CTX. (E) Subcellular localization of the DEPs between PT_CTX and C_CTX. (F) PPI network of DEPs between PT_CTX and C_CTX.
The PPI network analysis highlighted enriched DEP clusters primarily including “regulation of kinase activity,” “positive regulation of unsaturated fatty acid biosynthetic process,” “cGMP-PKG signaling pathway,” “transport of small molecules,” “regulation of small molecule metabolic process,” and others (Figure 4F). Six highly interconnected clusters were further identified as PPI networks (Supplementary Figure S2).
Proteomic analysis of the nucleus of the patient’s lens versus the controls’ lens
Ninety-five DEPs, comprising 51 upregulated and 44 downregulated proteins, were identified in the patient’s nucleus compared to controls, as illustrated in the volcano plot (Figure 5A). The top-10 upregulated and downregulated DEPs are listed in Tables 5, 6. GO functional classification (Figure 5B) indicated that DEPs were categorized into 24 GO terms, including 14 BPs, four CCs, and six MFs. Notably, “proteolysis,” “cellular component organization or biogenesis,” “cellular component biogenesis,” and “organelle organization” were the top four terms in the BPs. “Cytoskeleton” and “endopeptidase inhibitor activity” emerged as the most abundant terms in the CCs and MFs, respectively. The top 10 KEGG categories (Figure 5C) identified enriched pathways such as “mineral absorption,” “HIF-1 signaling pathway,” and “thyroid hormone synthesis.” IPR enrichment analysis revealed that the DEPs were predominantly enriched in the “AAA + ATPase domain” (Figure 5D). Subcellular localization prediction indicated that most proteins were located in the nucleus (31.51%), extracellular space (23.29%), and cytoplasm (19.18%) (Figure 5E).
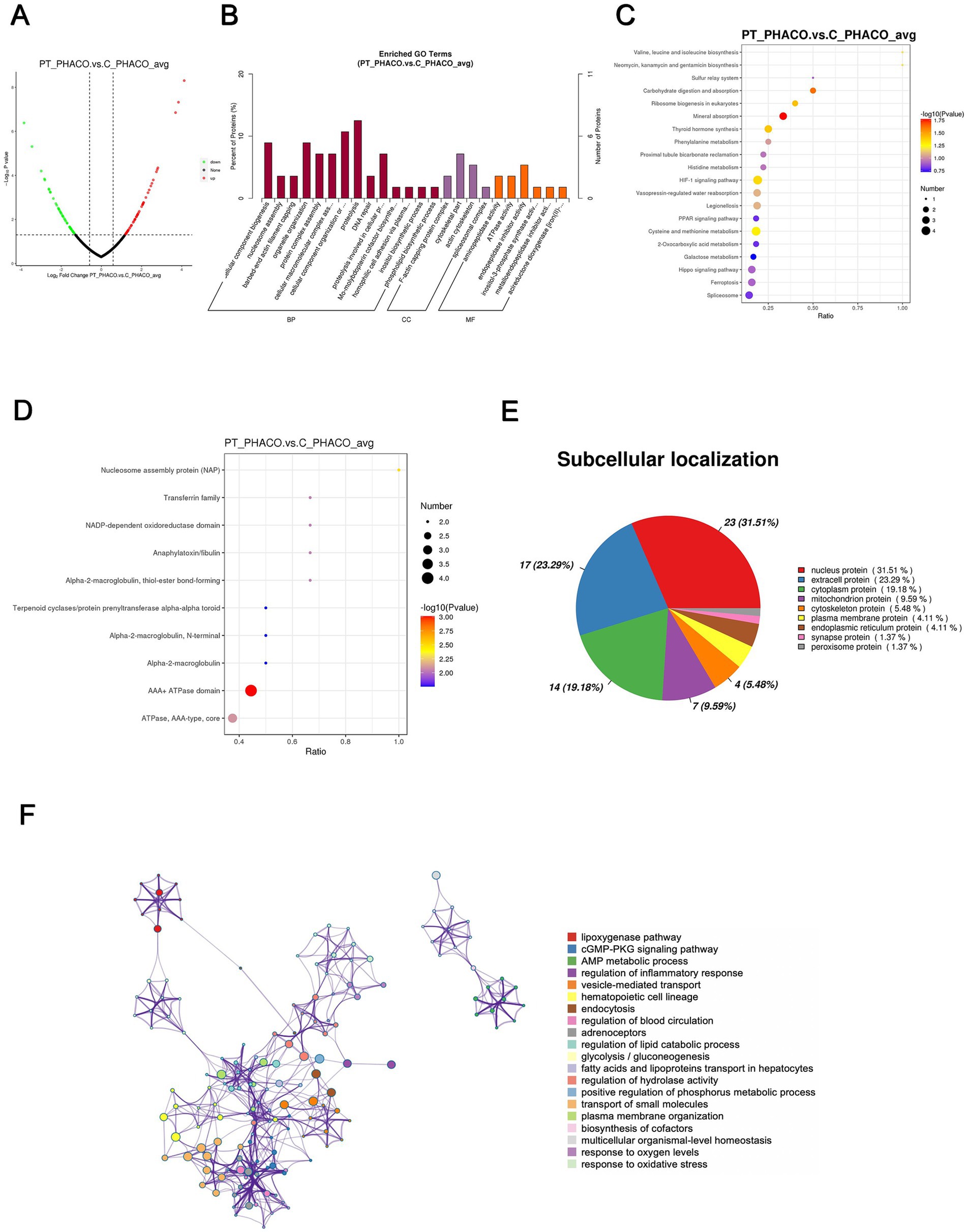
Figure 5. Quantitative proteomics analysis of DEPs between the patient’s and the controls’ phacoemulsification collect fluid. PT_PHACO, patient’s phacoemulsification collect fluid; C_PHACO, controls’ phacoemulsification collect fluid. (A) Volcano plot of DEPs. (B) GO classification of the DEPs between PT_PHACO and C_PHACO in terms of BP, CC, and MF. (C) Enrichment of KEGG pathways between PT_PHACO and C_PHACO. (D) IPR functional analysis of the DEPs between PT_PHACO and C_PHACO. (E) Subcellular localization of the DEPs between PT_PHACO and C_PHACO. (F) PPI network of DEPs between PT_PHACO and C_PHACO.
The PPI network analysis revealed enriched DEP clusters primarily including “transport of small molecules,” “regulation of hydrolase activity,” “hematopoietic cell lineage,” and others (Figure 5F). Nine highly interconnected clusters were further identified as PPI networks (Supplementary Figure S3).
Common DEPs in the anterior capsule, cortex, and nucleus of the patient’s lens versus controls
To elucidate the shared pathological mechanisms underlying lens opacification across each component of the lens, five commonly identified DEPs, A0A024R3E3 (APOA1), A0A140VKF3, P02787 (TF), Q6PIL8, and V9HWA9, within the anterior capsule, cortex, and nucleus of the patient’s lens were subjected to further analysis in comparison to controls (Table 7; Supplementary Figure S4). Notably, while all five DEPs exhibited downregulation in PT-CAP relative to C-CAP, they demonstrated upregulation in the cortex and nucleus of the patient’s lens compared to controls, with the exception of V9HWA9.
Discussion
This study unveiled the first documented instance of a toxic cataract induced by the inoculation of toad venom. Through proteomic sequencing and bioinformatics analyses, the lens sample extracted from the affected eye revealed a multitude of DEPs in comparison to the control group afflicted with non-toxic cataracts. Notably, the study identified five DEPs commonly expressed across the lens’s anterior capsule, cortex, and nucleus.
Venom ophthalmia, the ocular inoculation of venom, has been reported in association with a variety of animals, including snakes (5–9), toads (1–4), hornets (10–13), corals (14, 15), jellyfish, and anemones (16, 17). Rare instances involve spiders (18), caterpillars (19), moths (19), and walking sticks (20). The typical ocular manifestations resulting from these venoms include blepharitis, conjunctivitis, keratitis, corneal endothelial dysfunction, periorbital edema, iritis, or uveitis (5–10, 12, 14, 18, 20). More severe cases can lead to corneal erosions, ulceration, perforation, corneal decompensation, corneal and conjunctival neovascularization, cycloplegia, mydriasis, iris atrophy, ocular hypertonia or hypotonia, cataract, lens subluxation, lens abscess, optic neuropathy, and branch retinal artery occlusion (1, 2, 11–13, 15, 16). Specifically, ocular clinical manifestations from toad venom exposure may include chemosis, conjunctivitis, keratitis, stromal corneal edema with Descemet folds, corneal dysfunction, and ocular hypotonia (1–3). Very rarely, systemic reactions, including hypertension, bradycardia, and constriction of renal, mesenteric, and celiac arteries, may occur due to mucosal absorption of toad venom (4). Prior to this study, cases of cataracts caused by toad venom have not been reported.
Previous studies have established that toad venom primarily contains noxious substances such as bufadienolides (including bufofagins and bufotoxins with digitalis-like properties), bufotenines (exhibiting serotonin-like effects), and lipophilic alkaloids. Bufadienolides, acting as cardioactive substances, exert digitalis-like effects by inhibiting the Na+/K+-ATPase pump (21). This pump’s proper function is integral to the health of corneal endothelial cells, the ciliary body, and the iris, thereby playing a vital role in maintaining corneal transparency and regulating aqueous humor secretion (22, 23). Due to their highly lipophilic nature, bufadienolides can readily penetrate corneal layers, even with intact epithelium (24), potentially leading to corneal dysfunction and ocular hypotony. Furthermore, research suggests that systemic digoxin toxicity can also manifest as corneal edema, Descemet’s folds, and decreased intraocular pressure (24), thus reinforcing the previously described mechanisms. Despite the well-documented effects of toad venom, whether it induces cataracts through these mechanisms remains to be elucidated.
The present study conducted proteomic sequencing and bioinformatics analysis of lens specimens from a patient with cataracts induced by toad venom toxicity. A set of DEPs was identified in the patient’s lens compared to the control group. Notably, five DEPs were found to be commonly expressed across the anterior capsule, cortex, and nucleus of the lens: A0A024R3E3 (APOA1), A0A140VKF3, P02787 (TF), Q6PIL8, and V9HWA9. Previous reports have demonstrated that incubation of erythrocyte membranes with APOA1 results in decreased Na+/K+-ATPase activity (25). Additionally, research focusing on bufalin, one of the active constituents of toad venom and a member of cardiac steroids (CSs), confirmed the involvement of the Na+/K+-ATPase signalosome in the cytotoxicity of CSs. This research suggests that Apolipoprotein E regulates the sensitivity of cells to CSs by influencing the formation and function of the Na+/K+-ATPase signalosome (26). Another study highlights that CSs may cause the retention of TF in the early endosome. It is also suggested that CSs can increase acidification and the retention of recycled membrane within the early endosome or alter the sorting of the TF sensor to a more acidic compartment, such as the late endosome or lysosome (27).
In addition to the commonly identified DEPs in the anterior capsule, cortex, and nucleus of the lens, the present study unveiled several DEPs within each group’s top 10 rankings, which were associated with the functionality of Na+/K+-ATPase. Notably, the top three up-regulated DEPs in PT_CAP compared to C_CAP were MYH6, MYL2, and MYL3, as detailed in Table 1. It has been elucidated in prior research that myosin phosphatase (MP) interacts with Na+/K+-ATPase, thereby catalyzing the dephosphorylation of its inhibitory phosphorylation sites, thus designating this protein as a novel MP substrate (28). Furthermore, STAT1 demonstrates a notable upregulation in PT_CAP in comparison to C_CAP (Table 1). Studies underscore that interferon-gamma (IFN-g) significantly mitigates Na+/K+-ATPase activity through a cascade of transduction mechanisms set in motion by IFN-g, entailing the activation of PKC downstream of STAT1 phosphorylation, alongside the Raf-1, MEK, ERK2, and p38 MAPK pathways, in a complex sequence of events (29).
Ankyrin-1 (ANK1) was markedly downregulated in PT_CAP compared to C_CAP (Table 2). ANK1 exhibits a direct and high-affinity binding to recombinant peptides of the α1 subunit of Na+/K+-ATPase (30, 31). Ankyrin B (AnkB) functions as an adaptor protein, orchestrating the assembly of Na+/K+-ATPase and Na+/Ca2+ exchanger within the AnkB macromolecular complex (32). Mutations occurring within the established interaction motifs of Na+/K+-ATPase with AnkB and caveolin-1 are anticipated to perturb plasma membrane targeting, localization patterns, and the diffusion behavior of the enzyme (33).
Tropomyosin 1 (TPM1) displayed a significant downregulation in PT_CTX compared to C_CTX, as delineated in Table 4. Ouabain, a cardiac glycoside, exerts inhibitory effects on Na+/K+-ATPase, with its sensitivity being heightened by tropomyosin and calcium (34, 35). Moreover, hexokinase manifested a significant downregulation in PT_PHACO compared to C_PHACO, as indicated in Table 6. Investigations have elucidated that hexokinase interacts with ATP1A4, the testis-specific isoform of Na+/K+-ATPase, within a non-raft interactome (36).
Na+/K+-ATPase assumes a pivotal role in the maintenance of circulatory sodium homeostasis and regulation of hydrostatic pressure within the lens, primarily achieved by expelling Na+ ions into the extracellular space against their concentration gradient, thereby facilitating ionic flux to the equator (37). Notably, the activity of Na+/K+-ATPase exhibits variance between the two distinct cell types constituting the lens, namely epithelial cells and fibers, with epithelial cells displaying a higher specific activity compared to fibers. This discrepancy likely contributes to the observed differential expression of Na+/K+-ATPase-related DEPs across various lens sections.
Although the precise mechanisms await comprehensive elucidation, a robust correlation exists between aberrant lens sodium accumulation and cortical opacification observed in age-related human cataracts. Extant studies have implicated processes such as oxidation and glycation within aging lens fibers in diminishing Na+/K+-ATPase activity (38). Furthermore, experimental investigations in mice have demonstrated that inhibition of Na+/K+-ATPase pump activity can precipitate diverse biological and pathological phenomena, including cataractogenesis (39). Thus, it is plausible to infer that toad venom-induced cataracts may stem from Na+/K+-ATPase activity inhibition, perturbing ionic homeostasis within the lens. PPI network analysis of the DEPs in PT_CAP underscored their predominant association with functions such as “transportation of small molecules,” “regulation of metal ion transport,” and “import into cell,” thereby lending further credence to this hypothesis. However, a deeper comprehension of these mechanisms necessitates further investigation. While surgical intervention currently stands as the primary therapeutic modality for cataracts (40), the insights gleaned from these findings held promise for informing the prevention and management of toxic cataracts engendered by toad venom in future therapeutic endeavors.
This study is subject to certain limitations, primarily stemming from constraints related to the availability of patient specimens. Firstly, due to the rarity of such cases, the study group comprised only a single patient’s lens sample. Secondly, owing to the limited volume of protein specimens, conducting further validation of the DEPs was not feasible. Thirdly, concerning the impact of long-term sample storage on the accuracy of test results, lens samples from age-related cataract patients who underwent surgery on the same day were included as the control group, rather than congenital cataract patients of similar age as the patient in the present case, since the latter are relatively rare. Additionally, as the patient did not promptly seek medical attention following exposure to the toad venom, it remained impossible to ascertain the presence of any transient conditions such as low intraocular pressure, keratitis, or uveitis at the time of examination.
In summary, this study presented a unique case of ocular toad venom inoculation, representing the first documented instance of toxic cataract induced by inadvertent exposure to toad venom splash. Furthermore, proteomic sequencing was conducted on the lens specimen obtained from the patient, aimed at unraveling the underlying pathological mechanisms. The findings suggested a potential association between the development of cataract and alterations in Na+/K+-ATPase activity. This research offered valuable insights and might serve as a guiding framework for the clinical management of ocular toad venom inoculation in the future.
Data availability statement
The datasets presented in this study can be found in online repositories. The names of the repository/repositories and accession number(s) can be found below: http://www.proteomexchange.org/, PXD058328.
Ethics statement
The studies involving humans were approved by the Medical Ethics Committee of the Second Affiliated Hospital of Zhejiang University School of Medicine, Hangzhou, China. The studies were conducted in accordance with the local legislation and institutional requirements. The participants provided their written informed consent to participate in this study. Written informed consent was obtained from the individual(s) for the publication of any potentially identifiable images or data included in this article.
Author contributions
DL: Conceptualization, Validation, Writing – review & editing, Data curation, Formal analysis, Investigation, Methodology, Visualization, Writing – original draft. SN: Data curation, Formal analysis, Investigation, Methodology, Validation, Visualization, Writing – review & editing. JX: Data curation, Formal analysis, Investigation, Methodology, Validation, Writing – review & editing. SZ: Data curation, Formal analysis, Investigation, Methodology, Validation, Writing – review & editing. J-WX: Formal analysis, Investigation, Methodology, Writing – review & editing, Visualization. YF: Formal analysis, Investigation, Methodology, Writing – review & editing, Validation. CS: Formal analysis, Investigation, Methodology, Validation, Writing – review & editing. WX: Validation, Writing – review & editing, Conceptualization, Funding acquisition, Project administration, Resources, Supervision.
Funding
The author(s) declare that financial support was received for the research, authorship, and/or publication of this article. This study was supported by research grants from the National Key Research and Development Program of China (2020YFE0204400), the National Nature Science Foundation of China (82271042), and the Zhejiang Province Key Research and Development Program (2023C03090).
Conflict of interest
The authors declare that the research was conducted in the absence of any commercial or financial relationships that could be construed as a potential conflict of interest.
Generative AI statement
The author(s) declare that no Gen AI was used in the creation of this manuscript.
Publisher’s note
All claims expressed in this article are solely those of the authors and do not necessarily represent those of their affiliated organizations, or those of the publisher, the editors and the reviewers. Any product that may be evaluated in this article, or claim that may be made by its manufacturer, is not guaranteed or endorsed by the publisher.
Supplementary material
The Supplementary material for this article can be found online at: https://www.frontiersin.org/articles/10.3389/fmed.2024.1537770/full#supplementary-material
References
1. Pejic, R, Simic Prskalo, M, and Simic, J. Ocular Hypotonia and transient decrease of vision as a consequence of exposure to a common toad poison. Case Rep Ophthalmol Med. (2020) 2020:2983947–3. doi: 10.1155/2020/2983947
2. Lopez-Lopez, JM, Sanabria, MR, and de Prada, SJ. Ocular toxicity caused by toad venom. Cornea. (2008) 27:236–7. doi: 10.1097/ICO.0b013e31815b8317
3. Forrester, MB. Pediatric exposures to Bombina toads reported to poison centers. Pediatr Emerg Care. (2018) 34:25–6. doi: 10.1097/PEC.0000000000000719
4. Henricksen, K. The danger of kissing toads: fire-bellied toad exposure and assessment parameters in children. J Emerg Nurs. (1999) 25:235–7. doi: 10.1016/s0099-1767(99)70212-6
5. Jalink, MB. Ocular complications of spitting cobra venom. Indian J Ophthalmol. (2020) 68:2632–3. doi: 10.4103/ijo.IJO_1164_20
6. Jalink, MB, and Wisse, RPL. A guide to direct (Peri)ocular injuries caused by snakes: systematic review and treatment advice. Am J Trop Med Hyg. (2022) 107:968–73. doi: 10.4269/ajtmh.22-0289
7. Tsai, TH, Lin, CC, Mao, YC, Hung, CL, Yang, YC, Yang, CC, et al. Naja atra venom-spit ophthalmia in Taiwan: an epidemiological survey from 1990 to 2016. J Chin Med Assoc. (2020) 83:77–83. doi: 10.1097/JCMA.0000000000000223
8. Chang, KC, Huang, YK, Chen, YW, Chen, MH, Tu, AT, and Chen, YC. Venom Ophthalmia and ocular complications caused by Snake venom. Toxins (Basel). (2020) 12:10.3390/toxins12090576. doi: 10.3390/toxins12090576
9. Chu, ER, Weinstein, SA, White, J, and Warrell, DA. Venom ophthalmia caused by venoms of spitting elapid and other snakes: report of ten cases with review of epidemiology, clinical features, pathophysiology and management. Toxicon. (2010) 56:259–72. doi: 10.1016/j.toxicon.2010.02.023
10. Ono, T, Takahashi, S, Hisai, T, Kato, M, Mori, Y, Nejima, R, et al. Endothelial dysfunction of the cornea after exposure to sprayed venom from hornets. Cutan Ocul Toxicol. (2023) 42:185–9. doi: 10.1080/15569527.2023.2227902
11. Hirano, K, and Tanikawa, A. Ocular injury caused by the sprayed venom of the Asian Giant hornet (Vespa mandarinia). Case Rep Ophthalmol. (2020) 11:430–5. doi: 10.1159/000508911
12. Laborde-Casterot, H, Darrouzet, E, Le Roux, G, Labadie, M, Delcourt, N, de Haro, L, et al. Ocular lesions other than stings following yellow-legged hornet (Vespa velutina nigrithorax) projections, as reported to French poison control centers. JAMA Ophthalmol. (2021) 139:105–8. doi: 10.1001/jamaophthalmol.2020.4877
13. Reisman, RE. Unusual reactions to insect stings. Curr Opin Allergy Clin Immunol. (2005) 5:355–8. doi: 10.1097/01.all.0000173782.35283.b6
14. Farooq, AV, Gibbons, AG, Council, MD, Harocopos, GJ, Holland, S, Judelson, J, et al. Corneal toxicity associated with aquarium coral Palytoxin. Am J Ophthalmol. (2017) 174:119–25. doi: 10.1016/j.ajo.2016.10.007
15. Croskey, A, Trautman, W, Barton, D, Ratay, MK, and Shulman, J. Ocular injury from saltwater coral palytoxin. Am J Emerg Med. (2024) 75:197.e1–3. doi: 10.1016/j.ajem.2023.10.027
16. Glasser, DB, Noell, MJ, Burnett, JW, Kathuria, SS, and Rodrigues, MM. Ocular jellyfish stings. Ophthalmology. (1992) 99:1414–8. doi: 10.1016/s0161-6420(92)31800-7
17. Ruiz, Y, Fuchs, J, Beuschel, R, Tschopp, M, and Goldblum, D. Dangerous reef aquaristics: Palytoxin of a brown encrusting anemone causes toxic corneal reactions. Toxicon. (2015) 106:42–5. doi: 10.1016/j.toxicon.2015.09.001
18. Isbister, GK. Acute conjunctival inflammation following contact with squashed spider contents. Am J Ophthalmol. (2003) 136:563–4. doi: 10.1016/s0002-9394(03)00277-0
19. Hossler, EW. Caterpillars and moths. Dermatol Ther. (2009) 22:353–66. doi: 10.1111/j.1529-8019.2009.01247.x
20. Paysse, EA, Holder, S, and Coats, DK. Ocular injury from the venom of the southern walkingstick. Ophthalmology. (2001) 108:190–1. doi: 10.1016/s0161-6420(00)00432-2
21. Daly, JW. The chemistry of poisons in amphibian skin. Proc Natl Acad Sci USA. (1995) 92:9–13. doi: 10.1073/pnas.92.1.9
22. Li, Z, Duan, H, Li, W, Jia, Y, Zhang, S, Zhao, C, et al. Nicotinamide inhibits corneal endothelial mesenchymal transition and accelerates wound healing. Exp Eye Res. (2019) 184:227–33. doi: 10.1016/j.exer.2019.04.012
23. Delamere, NA. Ciliary body and ciliary epithelium. Adv Organ Biol. (2005) 10:127–48. doi: 10.1016/S1569-2590(05)10005-6
24. Smith, JL, and Mickatavage, RC. The ocular effects of topical Digitalis. Am J Ophthalmol. (1963) 56:889–94. doi: 10.1016/0002-9394(63)92324-9
25. Panin, LE, Ryazantseva, NV, Novitskii, VV, and Tokareva, NV. Apolipoprotein A-I possessing activity of defensin proteins modifies structure of plasma membranes. Bull Exp Biol Med. (2005) 140:406–9. doi: 10.1007/s10517-005-0504-y
26. Liu, M, Feng, LX, Sun, P, Liu, W, Mi, T, Lei, M, et al. Knockdown of Apolipoprotein E enhanced sensitivity of Hep3B cells to cardiac steroids via regulating Na+/K+-ATPase signalosome. Mol Cancer Ther. (2016) 15:2955–65. doi: 10.1158/1535-7163.MCT-15-0961
27. Feldmann, T, Glukmann, V, Medvenev, E, Shpolansky, U, Galili, D, Lichtstein, D, et al. Role of endosomal Na+-K+-ATPase and cardiac steroids in the regulation of endocytosis. Am J Physiol Cell Physiol. (2007) 293:C885–96. doi: 10.1152/ajpcell.00602.2006
28. Major, E, Keller, I, Horvath, D, Tamas, I, Erdodi, F, and Lontay, B. Smoothelin-like protein 1 regulates the thyroid hormone-induced homeostasis and remodeling of C2C12 cells via the modulation of myosin phosphatase. Int J Mol Sci. (2021) 22:10.3390/ijms221910293. doi: 10.3390/ijms221910293
29. Magro, F, Fraga, S, Ribeiro, T, and Soares-da-Silva, P. Intestinal Na+-K+-ATPase activity and molecular events downstream of interferon-gamma receptor stimulation. Br J Pharmacol. (2004) 142:1281–92. doi: 10.1038/sj.bjp.0705895
30. Devarajan, P, Scaramuzzino, DA, and Morrow, JS. Ankyrin binds to two distinct cytoplasmic domains of Na,K-ATPase alpha subunit. Proc Natl Acad Sci USA. (1994) 91:2965–9. doi: 10.1073/pnas.91.8.2965
31. Davis, JQ, and Bennett, V. The anion exchanger and Na+K(+)-ATPase interact with distinct sites on ankyrin in in vitro assays. J Biol Chem. (1990) 265:17252–6. doi: 10.1016/S0021-9258(17)44896-4
32. Skogestad, J, Aronsen, JM, Tovsrud, N, Wanichawan, P, Hougen, K, Stokke, MK, et al. Coupling of the Na+/K+-ATPase to Ankyrin B controls Na+/Ca2+ exchanger activity in cardiomyocytes. Cardiovasc Res. (2020) 116:78–90. doi: 10.1093/cvr/cvz087
33. Junghans, C, Vukojevic, V, Tavraz, NN, Maksimov, EG, Zuschratter, W, Schmitt, FJ, et al. Disruption of Ankyrin B and Caveolin-1 interaction sites alters Na(+),K(+)-ATPase membrane diffusion. Biophys J. (2017) 113:2249–60. doi: 10.1016/j.bpj.2017.08.053
34. Lelievre, LG, Potter, JD, Piascik, M, Wallick, ET, Schwartz, A, Charlemagne, D, et al. Specific involvement of calmodulin and non-specific effect of tropomyosin in the sensitivity to ouabain of Na+,K+-ATPase in murine plasmocytoma cells. Eur J Biochem. (1985) 148:13–9. doi: 10.1111/j.1432-1033.1985.tb08800.x
35. Charlemagne, D, Leger, J, Schwartz, K, Geny, B, Zachowski, A, and Lelievre, L. Role of tropomyosin in the sensitivity of (Na+ + K+) ATPase to ouabain. C R Seances Acad Sci D. (1979) 288:963–6.
36. Rajamanickam, GD, Kastelic, JP, and Thundathil, JC. Testis-specific isoform of Na/K-ATPase (ATP1A4) Interactome in raft and non-raft membrane fractions from capacitated bovine sperm. Int J Mol Sci. (2019) 20:10.3390/ijms20133159. doi: 10.3390/ijms20133159
37. Giannone, AA, Li, L, Sellitto, C, and White, TW. Physiological mechanisms regulating Lens transport. Front Physiol. (2021) 12:818649. doi: 10.3389/fphys.2021.818649
38. Delamere, NA, and Tamiya, S. Expression, regulation and function of Na,K-ATPase in the lens. Prog Retin Eye Res. (2004) 23:593–615. doi: 10.1016/j.preteyeres.2004.06.003
39. Jung, J, Ryu, S, Ki, IA, Woo, HA, and Lee, K. Some biological consequences of the inhibition of Na,K-ATPase by translationally controlled tumor protein (TCTP). Int J Mol Sci. (2018) 19:10.3390/ijms19061657. doi: 10.3390/ijms19061657
Keywords: toad venom, venom inoculation, toxic cataract, 4D label-free quantitative proteomics, Na+/K+-ATPase
Citation: Lyu D, Ni S, Xu J, Zhu S, Xu J-W, Feng Y, Shi C and Xu W (2025) Ocular inoculation of toad venom: toxic cataract and proteomic profiling. Front. Med. 11:1537770. doi: 10.3389/fmed.2024.1537770
Edited by:
Dahu Wang, Shanghai University of Traditional Chinese Medicine, ChinaReviewed by:
Ratnakar Tripathi, University of Missouri, United StatesCao Gu, Second Military Medical University, China
Copyright © 2025 Lyu, Ni, Xu, Zhu, Xu, Feng, Shi and Xu. This is an open-access article distributed under the terms of the Creative Commons Attribution License (CC BY). The use, distribution or reproduction in other forums is permitted, provided the original author(s) and the copyright owner(s) are credited and that the original publication in this journal is cited, in accordance with accepted academic practice. No use, distribution or reproduction is permitted which does not comply with these terms.
*Correspondence: Wen Xu, eHV3ZW4yMDAzQHpqdS5lZHUuY24=