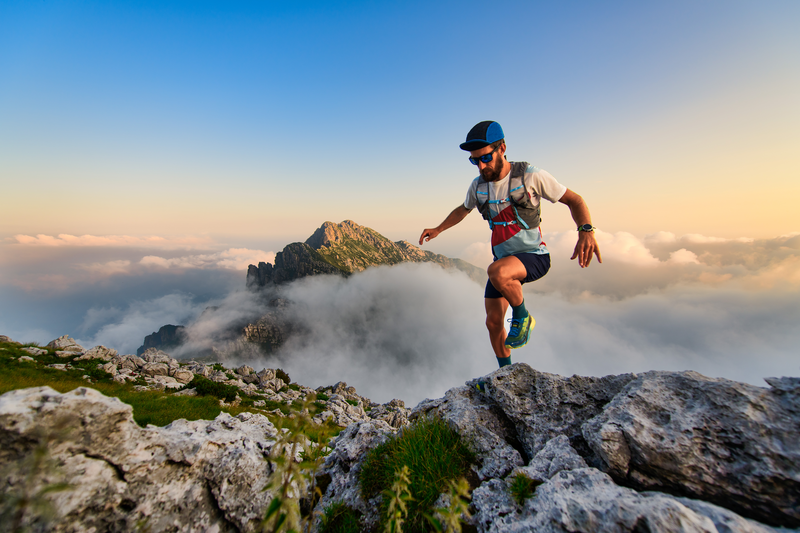
95% of researchers rate our articles as excellent or good
Learn more about the work of our research integrity team to safeguard the quality of each article we publish.
Find out more
ORIGINAL RESEARCH article
Front. Med. , 18 December 2024
Sec. Intensive Care Medicine and Anesthesiology
Volume 11 - 2024 | https://doi.org/10.3389/fmed.2024.1510997
Background: Sepsis-associated liver injury (SALI) is a critical component of sepsis-induced multiple organ dysfunction with high mortality. Identifying biomarkers for risk stratification is essential. Red cell distribution width (RDW), indicating variation in red blood cell volume, has been linked to adverse outcomes in various diseases. This study aimed to evaluate the association between RDW and 30-day mortality in SALI patients.
Methods: A retrospective cohort study was conducted using data from the Medical Information Mart for Intensive Care-IV database. Patients admitted to the intensive care unit (ICU) with SALI were included. RDW was recorded within the initial 24 h. The primary outcome was 30-day mortality. A multivariable Cox regression analysis was performed to examine the relationship between RDW and mortality.
Results: Among 529 SALI patients (mean age 68.7 years, 61.8% male), 46.1% had RDW > 15.5%. The 30-day mortality rate was 35.5%. RDW was significantly higher in non-survivors compared to survivors (17.2 ± 3.0 vs. 15.4 ± 2.3, P < 0.001). Cox regression identified RDW as an independent risk factor for 30-day mortality (HR 1.14, 95% CI 1.09 to 1.19, P < 0.001). Subgroup analyses demonstrated that the findings were consistent across the various groups.
Conclusion: Elevated RDW is independently associated with higher 30-day mortality in patients with SALI, suggesting its potential role in risk stratification and clinical management.
Sepsis, characterized by life-threatening organ dysfunction due to a dysregulated host response to infection, is a leading cause of global health burden (1). A global epidemiological study reported 48.9 million new cases of sepsis and 11.0 million sepsis-related deaths in 2017, accounting for 19.7% of global mortality (2). The liver plays a key role in maintaining metabolic balance and immune regulation (3, 4), particularly in the context of sepsis, where it is vital for orchestrating immune responses (5). Sepsis-associated liver injury (SALI) refers to liver dysfunction or injury induced by sepsis. Various factors, including pathogens, shock, excessive inflammatory responses, persistent microcirculatory failure, and oxidative stress, can lead to SALI (6). There are two main manifestations of SALI: ischemic hypoxic liver injury and sepsis-related cholestasis (7). There are no unified diagnostic criteria for SALI. The Surviving Sepsis Campaign Guidelines recommend using a total bilirubin > 2 mg/dL and an international normalized ratio (INR) > 1.5 as diagnostic criteria (8, 9). Mortality rates for SALI have been reported to be alarmingly high, ranging from 29.3 to 68% (10, 11). Given the high risk of mortality, early identification of SALI patients at risk of poor outcomes is essential for optimizing diagnostic and therapeutic strategies, ultimately improving patient prognosis.
Red cell distribution width (RDW) is derived from the standard deviation of erythrocyte volume based on the mean corpuscular volume. It serves as an indicator of anisocytosis, reflecting the variation in red blood cell size (12). Oxidative stress and inflammation are believed to shorten the lifespan of red blood cells and inhibit their maturation, leading to the premature release of immature erythrocytes into the bloodstream, thereby elevating RDW (13). These processes—oxidative stress and inflammation—are also key elements in the pathophysiology of sepsis. As a result, RDW may prove useful in identifying critically ill patients who require more intensive treatment. RDW has been recognized as an important prognostic marker in various acute conditions, such as heart failure (14), pancreatitis (15), kidney injury (16), stroke (17), and sepsis (18). Elevated RDW is consistently associated with worse clinical outcomes. The objective of this study is to assess the relationship between RDW and 30-day mortality in patients with SALI, while also investigating its potential utility as a prognostic indicator.
The study population was obtained from the MIMIC-IV database. This database is an electronic health record repository that includes extensive, high-quality, deidentified data on intensive care unit (ICU) patients from the Beth Israel Deaconess Medical Center (BIDMC) in Boston, MA, USA, covering the years between 2008 and 2019. Access to this dataset was granted under certification number 58844105. The Institutional Review Board at BIDMC approved a waiver of informed consent and permitted the use of this research resource. This cohort study followed the guidelines established by the Strengthening the Reporting of Observational Studies in Epidemiology (STROBE) statement (19).
Our study encompassed all eligible ICU patients from the MIMIC-IV database. We specifically targeted adults diagnosed with sepsis-3, characterized by an increase of at least 2 points in the Sequential Organ Failure Assessment (SOFA) score as a result of a dysregulated host response to infection (1). According to the Surviving Sepsis Campaign guidelines, the criteria for diagnosing sepsis-associated liver injury (SALI) include a total bilirubin level exceeding 2 mg/dL and an INR greater than 1.5 in patients with sepsis (10). These criteria are widely recognized in the literature. The study was restricted to patients with RDW measurements obtained within the first 24 h of ICU admission. The exclusion criteria included the following: (1) individuals younger than 18 years; (2) multiple hospital admissions, excluding their initial ICU admission; (3) absence of RDW data within 24 h of ICU admission or missing information on other variables.
Data pertinent to this study were obtained from the MIMIC-IV database through Structured Query Language (SQL) queries and were subsequently stored in PostgreSQL. At the time of sepsis diagnosis, initial records were systematically collected, including demographic details such as age, sex, and race; vital signs like heart rate and mean blood pressure (MBP); and laboratory findings that encompassed RDW, white blood cell count (WBC), platelet count, hemoglobin, hematocrit, glucose, blood urea nitrogen (BUN), creatinine, and alanine aminotransferase (ALT). Additionally, comorbidities were noted, including congestive heart failure, chronic respiratory disease, diabetes, renal disease, and malignant cancer, along with disease severity metrics such as SOFA score, Charlson comorbidity index, and simplified acute physiology score (SAPS II). The SOFA score evaluates organ dysfunction across six systems, each scored from 0 to 4, with higher scores indicating worse dysfunction (1) (Supplementary Table 1). The SAPS II score is calculated within 24 h of ICU admission, using 12 physiological variables (e.g., heart rate, blood pressure, oxygenation, serum potassium) and patient factors (age, chronic conditions) (20) (Supplementary Table 2). Each variable is assigned a predefined score, with higher scores indicating a higher mortality risk. The Charlson comorbidity index assesses 17 conditions, including cardiovascular disease, diabetes, chronic kidney disease, liver disease, and cancer (21) (Supplementary Table 3). Higher scores indicate a greater comorbidity burden and higher mortality risk. Interventions, including antibiotic therapy and vasoactive agents administered on day one, were also noted. Comorbidity information was obtained using the International Classification of Diseases (ICD) coding systems.
Since none of the patients had an RDW below the normal range of 10.5 to 15.5%, they were classified into two groups: the normal RDW group (nRDW), comprising individuals with RDW ≤ 15.5%, and the high RDW group (hRDW), which included those with RDW > 15.5%. In addition, RDW was categorized into quartiles (Q1-Q4) for sensitivity analysis.
The primary outcome was 30-day mortality. The secondary outcomes included in-hospital mortality, 90-day mortality, ventilator-free days until 28 days, vasopressor-free days until 28 days, and ICU-free days until 28 days.
Due to the retrospective design of this study, no predefined statistical analysis plan or power calculation was implemented; the sample size relied on available database data. Categorical variables are presented as frequencies and percentages, whereas continuous variables are expressed as means with standard deviations (SD) or medians with interquartile ranges (IQR). Continuous variables were assessed based on their distribution, utilizing either the Student’s t-test or the Wilcoxon rank-sum test. Categorical variables were evaluated using either Pearson’s chi-squared test or Fisher’s exact test.
Variable selection was guided by clinical relevance and existing literature. Variables with p-values < 0.1 in univariate analysis were included in the multivariate analysis (Supplementary Table 4). The effect of RDW on 30-day mortality was assessed using multivariable Cox regression models, yielding hazard ratios (HR) and 95% confidence intervals (CI) after adjusting for relevant covariates. Subgroup analyses were performed to explore interactions with covariates such as age, race, and comorbidities. Kaplan–Meier survival curves evaluated 30-day mortality across RDW categories, with significance determined by the log-rank test. Receiver operating characteristic (ROC) curves were generated to assess RDW’s predictive performance for 30-day mortality, calculated as the area under the curve (AUC). In addition, sensitivity analyses were performed by converting the types of variables and excluding special populations.
All analyses utilized R Statistical Software (Version 4.2.2, The R Foundation)1 and Free Statistics analysis platform (Version 2.0, Beijing, China). A p-value < 0.05 in a two-sided test was deemed statistically significant.
This analysis included a total of 529 patients diagnosed with SALI. The patient selection and data screening processes are illustrated in Figure 1. The baseline characteristics of patients, stratified by survival status, are presented in Table 1. The overall mean age at ICU admission was 68.7 years, and 61.8% of the patients were male. Patients in the non-survival group demonstrated higher scores on the Charlson comorbidity index, SOFA score, and SAPS II. Specifically, the RDW was significantly higher in the non-survival group compared to the survival group (17.2 vs. 15.4%, P < 0.001). Furthermore, the proportion of patients receiving vasopressin in the non-survival group was significantly greater than that in the survival group (75.5 vs. 54%, P < 0.001).
Figure 1. Flow chart of patient selection. ICU, intensive care unit; MIMIC-IV, Medical Information Mart in Intensive Care-IV; SALI, sepsis-associated liver injury; INR, international normalized ratio, RDW, red blood cell distribution width; MBP, mean blood pressure; WBC, white blood cell; ALT, alanine aminotransferase.
The overall prevalence of 30-day mortality among the cohort was found to be 35.5%. When RDW was considered as a continuous variable, the univariable Cox regression analysis revealed a significant association with 30-day mortality (crude HR 1.17; 95% CI, 1.13 to 1.23; P < 0.001). Following multivariable adjustment for factors including age, race, heart rate, MBP, creatinine, BUN, ALT, Charlson comorbidity index, SOFA, SAPS II, malignant cancer, and vasoactive agent, this association remained significant (adjusted HR 1.14; 95% CI, 1.09 to 1.19; P < 0.001) (Table 2). To further investigate this relationship, we utilized a restricted cubic spline analysis. The result indicated that, after accounting for the previously mentioned covariates, the relationship between RDW and 30-day mortality was linear (P for non-linearity = 0.340) (Supplementary Figure 1).
Table 2. Relationship between red blood cell distribution width and 30-day morality in different models.
When RDW was treated as a categorical variable with a cutoff of 15.5%, the hRDW group exhibited a heightened risk of 30-day mortality compared with the nRDW group (adjusted HR 1.62; 95% CI, 1.17 to 2.23; P = 0.004) (Table 2). Kaplan–Meier survival curve estimates further indicated a significantly elevated risk of 30-day mortality in patients with high RDW (log-rank: P < 0.001; Figure 2). The association persisted when RDW was categorized into quartiles. Compared to individuals in Q1, those in Q4 faced a 2.71-fold increased risk of 30-day mortality (adjusted HR, 3.71; 95% CI, 2.19 to 6.28; P < 0.001; Table 2, Model 3).
Figure 2. Kaplan–Meier curve for 30-day mortality according to red blood cell distribution width (RDW). nRDW, the normal RDW group (RDW ≤ 15.5%); hRDW, the high RDW group (RDW > 15.5%).
Subgroup analyses were conducted to assess the influence of age, sex, race, congestive heart failure, diabetes, chronic pulmonary disease, renal disease, and malignant cancer on the relationship between RDW and 30-day mortality. No statistically significant interactions were identified within any subgroup (p > 0.05, Figure 3). These results indicate that the effect of RDW on 30-day mortality remains consistent across different age, race, sex, and comorbidity groups.
Figure 3. Subgroup analyses for the association of red blood cell distribution width with 30-day mortality in patients with sepsis-associated liver injury. Multivariate Cox proportional hazards models were adjusted for age, race, heart rate, mean blood pressure, creatinine, blood urea nitrogen, alanine aminotransferase, Charlson comorbidity index, sequential organ failure assessment score, simplified acute physiology score II, malignant cancer, vasoactive agent (day 1).
ROC analysis revealed that RDW possesses moderate clinical predictive value for 30-day mortality in patients with SALI, achieving an AUC of 0.70 (95% CI, 0.66 to 0.75). We subsequently compared the discriminatory ability for 30-day in-hospital mortality among RDW, SAPS II, and SOFA using ROC curves (Figure 4). RDW exhibited stronger predictive discrimination for 30-day mortality in SALI patients compared to SOFA (AUC, 0.57; 95% CI, 0.52 to 0.62; P < 0.001), but was less effective than SAPS II (AUC, 0.80; 95% CI, 0.76 to 0.84; P = 0.002) (Supplementary Figures 2–4 and Supplementary Table 5).
Figure 4. The area under the receiver operating characteristic curve (AUC-ROC) for the models including RDW, SAPS II, and SOFA score. RDW, red blood cell distribution width SOFA, Sequential Organ Failure Assessment; SAPS II, simplified acute physiology score.
Increased RDW levels were associated with a higher risk of in-hospital mortality (adjusted HR, 1.13; 95% CI, 1.07 to 1.18; P < 0.001). Similar patterns were observed for 90-day mortality. Each 1% rise in RDW was linked to a reduction of 0.81 days in ICU-free days (95% CI, −1.12 to −0.51), 0.96 days in ventilator-free days (95% CI, −1.30 to −0.62), and 0.98 days in vasopressor-free days (95% CI, −1.32 to −0.65) over a 28-day period (Table 3).
We converted non-normally distributed continuous variables, including BUN, creatinine, ALT, SOFA score, and Charlson comorbidity index, into categorical variables for sensitivity analysis. BUN, creatinine, and ALT were classified according to clinically relevant cut-offs. Normal values range from 6 to 20 mg/dL for BUN and 0.5 to 1.2 mg/dL for creatinine, and 0–40 IU/L for ALT. The SOFA score and Charlson comorbidity index were classified according to the median, which were 4 and 6, respectively. The multivariable Cox regression analysis showed that higher RDW was significantly associated with an increased risk of 30-day mortality (Supplementary Table 6).
In addition, we performed a multivariate COX regression analysis after excluding patients with liver malignancies, anemia, and myelodysplastic syndromes, and the results remained robust (Supplementary Tables 7, 8).
In this retrospective cohort study that employed extensive public datasets, we discovered a notable correlation between RDW and 30-day mortality among patients with SALI. The multivariate Cox regression analysis, after controlling for potential confounders, consistently demonstrated an elevated risk of 30-day mortality among SALI patients with higher RDW levels. This association was consistently evident across various subgroup analyses. Additionally, the Kaplan–Meier survival curve analysis showed a greater 30-day mortality rate in the hRDW group. ROC analysis further indicated that RDW possesses moderate clinical predictive value for 30-day mortality in SALI patients. Our findings provide substantial evidence supporting the potential role of RDW as a prognostic biomarker for mortality prediction in this population.
A growing body of evidence has established a link between RDW and negative outcomes across various diseases. Numerous studies have identified RDW as an independent prognostic factor for sepsis (22, 23). Melchio et al. (14) found that RDW was a powerful marker of worse long-term outcomes in patients with acute heart failure, and its prognostic value is maintained beyond that provided by other well-established risk factors or biomarkers. O’Connell et al. (15) demonstrated that patients with RDW levels exceeding the normal upper limit faced a greater risk of inpatient mortality and an increased likelihood of requiring critical care support. In both retrospective and prospective analyses involving 258 patients with ARDS, those with RDW ≥ 14.45% exhibited significantly higher rates of acute kidney injury and 28-day mortality compared to those with RDW < 14.45% (16). Additionally, a separate study focused on identifying biomarkers for predicting in-hospital mortality in SALI patients, which involved 770 individuals, and identified a significant association between RDW and mortality (7). Our findings are consistent with these results, indicating that RDW is associated with 30-day mortality in patients with SALI and may function as a valuable prognostic marker.
Nevertheless, some researchers have raised doubts regarding the prognostic significance of RDW. Fontana et al. (24) carried out a study with 122 patients suffering from sepsis and reported no relationship between RDW and microcirculatory alterations, disease severity, the presence of shock, or survival outcomes. Another study observed that while RDW was linked to 30-day mortality in patients with severe sepsis, it did not serve as an independent predictor of mortality (25). These inconsistencies may stem from variations in study populations, the timing of measurements, and the criteria used to define abnormal RDW levels.
Various scoring systems, such as SOFA and SAPS II, are commonly used to predict outcomes in critically ill patients (26), but their effectiveness is often questioned. These scores involve multiple indicators, making them cumbersome, especially in resource-limited settings. In comparison, RDW is straightforward to acquire, quickly measurable, and offers relatively high accuracy, making it a convenient index for prognostic prediction. Our ROC analysis indicates that RDW provides a moderate prediction of 30-day mortality in SALI patients, demonstrating better predictive capability than the SOFA score while being less effective than the SAPS II score. Therefore, RDW may have a certain complement to existing biomarkers in predicting mortality. Further prospective studies are needed in the future to verify its role in clinical practice.
The exact mechanisms linking increased RDW to mortality in SALI patients remain unclear. Research has demonstrated a notable association between RDW and inflammatory markers (12). Proinflammatory cytokines may damage red blood cell membranes and inhibit maturation, resulting in the entry of larger, immature erythrocytes into circulation and subsequently raising RDW. Additionally, heightened oxidative stress could reduce erythrocyte lifespan, prompting the release of larger immature cells from the bone marrow (27). However, since this study is a retrospective observational analysis, it cannot elucidate the underlying mechanisms. More research is needed to investigate these processes and better understand the role of RDW in liver injury among septic patients.
This study presents several strengths. Firstly, it benefits from a relatively large sample size, with data sourced from the MIMIC-IV database, a comprehensive and high-quality real-world resource. Secondly, the robustness of the findings is supported by subgroup analyses. However, there are limitations to consider. Primarily, as a retrospective study, it is prone to selection bias. Secondly, due to the limitations of public databases, we were unable to exclude all patients with diseases that could affect our results, such as secondary sclerosing cholangitis in critically ill patients (SSC-CIP). However, we have tried to exclude patients with liver diseases such as chronic liver disease/cirrhosis as much as possible to ensure the accuracy and specificity of our findings. In addition, we further excluded people with liver malignancies, anemia, and myelodysplastic syndromes and conducted a sensitivity analysis to verify the reliability of our results. Thirdly, due to the nature of our retrospective study design and the limitations inherent in the dataset we utilized, we were unable to access detailed information regarding the specific methods used for laboratory tests. Additionally, we only included RDW data for patients upon ICU admission, preventing us from evaluating how changes in RDW post-admission might influence mortality, potentially affecting result accuracy. Furthermore, despite efforts to account for confounding variables, the retrospective design leaves open the possibility of unidentified confounders impacting our findings. Lastly, the study’s design does not permit conclusions about causality between RDW and mortality.
Elevated RDW levels are significantly associated with increased 30-day mortality in patients with SALI, indicating that RDW may serve as a reliable and effective prognostic indicator for sepsis. Nonetheless, further large-scale prospective multicenter studies are required to confirm these findings.
The datasets presented in this study can be found in online repositories. The names of the repository/repositories and accession number(s) can be found below: This study used the MIMIC IV v2.2 dataset. This dataset can be found in the online repository at https://physionet.org/content/mimiciv/2.2/.
The studies involving humans were approved by the Institutional Review Boards at Beth Israel Deaconess Medical Center (2001-P-001699/14) and Massachusetts Institute of Technology (No. 0403000206). The studies were conducted in accordance with the local legislation and institutional requirements. The ethics committee/institutional review board waived the requirement of written informed consent for participation from the participants or the participants’ legal guardians/next of kin because written informed consent from participants or their legal guardians/next of kin was also waived by the ethics committee/institutional review board, as the database did not contain protected health information.
TA: Conceptualization, Data curation, Formal analysis, Funding acquisition, Investigation, Methodology, Resources, Software, Supervision, Validation, Visualization, Writing – original draft, Writing – review and editing. YH: Data curation, Methodology, Writing – review and editing. PZ: Methodology, Supervision, Writing – review and editing. MH: Project administration, Writing – review and editing.
The authors declare that no financial support was received for the research, authorship, and/or publication of this article.
We would like to express our sincere gratitude to the MIMIC database for providing the valuable data used in this study. We thank Dr. Liu Jie (People’s Liberation Army of China General Hospital, Beijing, China) and Dr. Yang Qilin (The Second Affiliated Hospital of Guangzhou Medical University, Guangzhou, Guangdong, China) for their help with this revision.
The authors declare that the research was conducted in the absence of any commercial or financial relationships that could be construed as a potential conflict of interest.
The authors declare that no Generative AI was used in the creation of this manuscript.
All claims expressed in this article are solely those of the authors and do not necessarily represent those of their affiliated organizations, or those of the publisher, the editors and the reviewers. Any product that may be evaluated in this article, or claim that may be made by its manufacturer, is not guaranteed or endorsed by the publisher.
The Supplementary Material for this article can be found online at: https://www.frontiersin.org/articles/10.3389/fmed.2024.1510997/full#supplementary-material
1. Singer M, Deutschman C, Seymour C, Shankar-Hari M, Annane D, Bauer M, et al. The third international consensus definitions for sepsis and septic shock (sepsis-3). JAMA. (2016) 315(8):801–10.
2. Rudd K, Johnson S, Agesa K, Shackelford K, Tsoi D, Kievlan D, et al. Global, regional, and national sepsis incidence and mortality, 1990-2017: Analysis for the global burden of disease study. Lancet Lond Engl. (2020) 395(10219):200–11.
3. Solhi R, Lotfinia M, Gramignoli R, Najimi M, Vosough M. Metabolic hallmarks of liver regeneration. Trends Endocrinol Metab. (2021) 32(9):731–45.
5. Zhang X, Liu H, Hashimoto K, Yuan S, Zhang J. The gut-liver axis in sepsis: Interaction mechanisms and therapeutic potential. Crit Care Lond Engl. (2022) 26(1):213.
6. Lelubre C, Vincent J. Mechanisms and treatment of organ failure in sepsis. Nat Rev Nephrol. (2018) 14(7):417–27.
7. Liu Y, Sun R, Jiang H, Liang G, Huang Z, Qi L, et al. Development and validation of a predictive model for in-hospital mortality in patients with sepsis-associated liver injury. Ann Transl Med. (2022) 10(18):997.
8. Wen C, Zhang X, Li Y, Xiao W, Hu Q, Lei X, et al. An interpretable machine learning model for predicting 28-day mortality in patients with sepsis-associated liver injury. PLoS One. (2024) 19(5):e0303469. doi: 10.1371/journal.pone.0303469
9. Dellinger R, Levy M, Rhodes A, Annane D, Gerlach H, Opal S, et al. Surviving sepsis campaign: International guidelines for management of severe sepsis and septic shock: 2012. Crit Care Med. (2013) 41(2):580.
10. Yi X, Jin D, Huang S, Xie Z, Zheng M, Zhou F, et al. Association between lactate-to-albumin ratio and 28-days all-cause mortality in patients with sepsis-associated liver injury: A retrospective cohort study. BMC Infect Dis. (2024) 24(1):65. doi: 10.1186/s12879-024-08978-x
11. Zhang Z, Tan X, Shi H, Zhang H, Li J, Liao X. Bibliometric study of sepsis-associated liver injury from 2000 to 2023. World J Gastroenterol. (2024) 30(30):3609–24. doi: 10.3748/wjg.v30.i30.3609
12. Salvagno G, Sanchis-Gomar F, Picanza A, Lippi G. Red blood cell distribution width: A simple parameter with multiple clinical applications. Crit Rev Clin Lab Sci. (2015) 52(2):86–105. doi: 10.3109/10408363.2014.992064
13. Bazick H, Chang D, Mahadevappa K, Gibbons F, Christopher K. Red cell distribution width and all-cause mortality in critically ill patients. Crit Care Med. (2011) 39(8):1913–21.
14. Melchio R, Rinaldi G, Testa E, Giraudo A, Serraino C, Bracco C, et al. Red cell distribution width predicts mid-term prognosis in patients hospitalized with acute heart failure: The RDW in acute heart failure (RE-AHF) study. Intern Emerg Med. (2019) 14(2):239–47. doi: 10.1007/s11739-018-1958-z
15. O’Connell R, Boland M, O’Driscoll J, Salih A, Arumugasamy M, Walsh T, et al. Red cell distribution width and neutrophil to lymphocyte ratio as predictors of outcomes in acute pancreatitis: A retrospective cohort study. Int J Surg Lond Engl. (2018) 55:124–7.
16. Cai N, Jiang M, Wu C, He F. Red cell distribution width at admission predicts the frequency of acute kidney injury and 28-day mortality in patients with acute respiratory distress syndrome. Shock Augusta Ga. (2022) 57(3):370–7. doi: 10.1097/SHK.0000000000001840
17. Xie K, Liu L, Liang Y, Su C, Li H, Liu R, et al. Red cell distribution width: A novel predictive biomarker for stroke risk after transient ischaemic attack. Ann Med. (2022) 54(1):1167–77. doi: 10.1080/07853890.2022.2059558
18. Dankl D, Rezar R, Mamandipoor B, Zhou Z, Wernly S, Wernly B, et al. Red cell distribution width is independently associated with mortality in sepsis. Med Princ Pract. (2022) 31(2):187–94.
19. von Elm E, Altman D, Egger M, Pocock S, Gøtzsche P, Vandenbroucke J, et al. The strengthening the reporting of observational studies in epidemiology (STROBE) statement: Guidelines for reporting observational studies. Lancet Lond Engl. (2007) 370(9596):1453–7.
20. Le Gall J, Lemeshow S, Saulnier FA. new simplified acute physiology score (SAPS II) based on a european/north american multicenter study. JAMA. (1993) 270(24):2957–63.
21. Charlson M, Pompei P, Ales K, MacKenzie CRA. new method of classifying prognostic comorbidity in longitudinal studies: development and validation. J Chronic Dis. (1987) 40(5):373–83.
22. Jiang Y, Jiang F, Kong F, An M, Jin B, Cao D, et al. Inflammatory anemia-associated parameters are related to 28-day mortality in patients with sepsis admitted to the ICU: A preliminary observational study. Ann Intensive Care. (2019) 9(1):67. doi: 10.1186/s13613-019-0542-7
23. Kim Y, Song J, Kim E, Choi H, Jeong W, Jung I, et al. A simple scoring system using the red blood cell distribution width, delta neutrophil index, and platelet count to predict mortality in patients with severe sepsis and septic shock. J Intensive Care Med. (2019) 34(2):133–9.
24. Fontana V, Spadaro S, Bond O, Cavicchi F, Annoni F, Donadello K, et al. No relationship between red blood cell distribution width and microcirculatory alterations in septic patients. Clin Hemorheol Microcirc. (2017) 66(2):131–41. doi: 10.3233/CH-160154
25. Jandial A, Kumar S, Bhalla A, Sharma N, Varma N, Varma S. Elevated red cell distribution width as a prognostic marker in severe sepsis: A prospective observational study. Indian J Crit Care Med. (2017) 21(9):552–62.
27. Karabulut B, Arcagok B. New diagnostic possibilities for early onset neonatal sepsis: Red cell distribution width to platelet ratio. Fetal Pediatr Pathol. (2020) 39(4):297–306. doi: 10.1080/15513815.2019.1661051
28. Vincent JL, Moreno R, Takala J, Willatts S, De Mendonça A, Bruining H, et al. The SOFA (Sepsis-related Organ Failure Assessment) score to describe organ dysfunction/failure. On behalf of the Working Group on Sepsis-Related Problems of the European Society of Intensive Care Medicine. Intensive Care Med. (1996) 22:707–10. doi: 10.1007/BF01709751
Keywords: sepsis-associated liver injury, red cell distribution width, 30-day mortality, critical illness, sepsis
Citation: Ao T, Huang Y, Zhen P and Hu M (2024) Association between red cell distribution width and 30-day mortality in patients with sepsis-associated liver injury: a retrospective cohort study. Front. Med. 11:1510997. doi: 10.3389/fmed.2024.1510997
Received: 14 October 2024; Accepted: 29 November 2024;
Published: 18 December 2024.
Edited by:
Iokfai Cheang, Nanjing Medical University, ChinaReviewed by:
Vlatka Sotošek, University of Rijeka, CroatiaCopyright © 2024 Ao, Huang, Zhen and Hu. This is an open-access article distributed under the terms of the Creative Commons Attribution License (CC BY). The use, distribution or reproduction in other forums is permitted, provided the original author(s) and the copyright owner(s) are credited and that the original publication in this journal is cited, in accordance with accepted academic practice. No use, distribution or reproduction is permitted which does not comply with these terms.
*Correspondence: Ming Hu, aG15eDIwMTJAc2luYS5jb20=
Disclaimer: All claims expressed in this article are solely those of the authors and do not necessarily represent those of their affiliated organizations, or those of the publisher, the editors and the reviewers. Any product that may be evaluated in this article or claim that may be made by its manufacturer is not guaranteed or endorsed by the publisher.
Research integrity at Frontiers
Learn more about the work of our research integrity team to safeguard the quality of each article we publish.