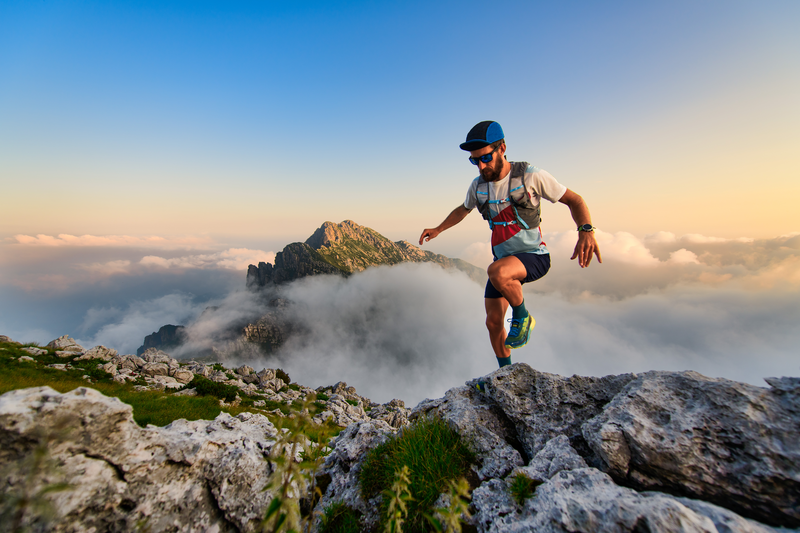
94% of researchers rate our articles as excellent or good
Learn more about the work of our research integrity team to safeguard the quality of each article we publish.
Find out more
ORIGINAL RESEARCH article
Front. Med. , 22 January 2025
Sec. Ophthalmology
Volume 11 - 2024 | https://doi.org/10.3389/fmed.2024.1507971
This article is part of the Research Topic Imaging in the Diagnosis and Treatment of Eye Diseases View all 17 articles
Background: Wet age-related macular degeneration (wAMD) is a vision-threatening eye disease worldwide. The amplitude of low-frequency fluctuation (ALFF) method was used to observe changes in spontaneous brain activity, which may help to investigate the underlying pathological mechanism of AMD.
Methods: Eighteen patients with wAMD and 18 age- and gender-matched healthy controls (HCs) were recruited. The ALFF method was used on each subject and mean ALFF values were compared between groups. The receiver operating characteristic (ROC) curve was used to compare the two groups.
Results: ALFF values in the temporal lobe and limbic lobe/parahippocampal gyrus were significantly higher than controls, while values in the postcentral gyrus were significantly lower. The under the curve of the ROC (AUC) of the three regions shows high accuracy of the diagnosis.
Conclusion: The abnormal spontaneous brain activity of patients with AMD suggests scope for the use of ALFF in the diagnosis or prognosis in AMD.
The role of the visual system is to process visual information via neural pathways connecting the eyes, optic nerve, lateral geniculate body, and visual cortex (1). Impairment of this system is a serious health problem. In recent years, a range of eye diseases, such as myopia, optic neuritis, glaucoma, and wet age-related macular degeneration (wAMD) are increasingly common. AMD, as the name suggests, is related to age, and features painless, progressive degeneration of the macular. This region is responsible for visual acuity, so the disease will seriously affect the daily life of patients, and is a very harmful disease. Globally, it is a major cause of irreversible blindness in the elderly (2). With an increase in the older population in many countries, more than 20% people may suffer from this disease (3). In China, although AMD is not the main cause of blindness, the incidence has increased in recent years. One study showed that in developed cities, the incidence of AMD has reached 15.5% (4). Regarding the cause of AMD, we know that there are many risk factors for the disease, however, the pathophysiological mechanism of AMD has not been completely elucidated (5). It is believed that it is the result of a combination of metabolism, genetics, environment, and other factors (6). Abnormalities of photoreceptor cells, retinal pigment epithelium, and Bruch’s membrane/choroid complex usually progress to cause geographic atrophy or neovascularization (7). AMD is categorized as wet or dry according to the pathological stage. wAMD is characterized by neovascularization and is the most common form.
The diagnosis of AMD is aided by examination methods such as fundus angiography, optical coherence tomography (OCT), fundus autofluorescence, and confocal laser fundus imaging. Clinical findings allow classification based on published consensus as follows (8): (1) A lack of visible drusen or abnormal pigmentation indicates no sign of AMD; (2) The presence of small drusen (<63 μm), also known as Drupelet, is considered a normal aging change without risk of progression to AMD; (3) Moderate drusen (63 ~ 125 μm) with no pigment abnormalities indicates early AMD; (4) Large drusen or a combination of moderate drusen with pigment abnormalities indicates moderate AMD; (5) Neovascular AMD or geographic atrophy should be deemed advanced AMD. The fundus of a patient with AMD is shown in Figure 1.
Figure 1. Typical fundus photographs of AMD patients. (A) Funduscopy in AMD patients; (B) Fluorescein angiography in AMD patients. AMD, age-related macular degeneration.
AMD is a disease affecting central vision, and therefore reducing the ability to drive, read, and recognize faces (9). At present, the disease cannot be cured clinically, and the current treatment method is intravitreal injection (10). However, it is understood that AMD has different pathological types, so the treatment methods also vary. Central vision is significantly affected in the late stage of GA, and most patients with dry AMD are already in the late stage. At this time, treatment for AMD is mainly based on a number of factors including the inhibition of inflammation and nutrient nerves, Nutrient nerve is a treatment method that protects photoreceptors by providing drugs. wAMD is characterized by the formation of choroidal neovascularization (CNV) and can cause subretinal exudation and bleeding. Therefore, current treatment methods for this form of the disease are mainly laser photocoagulation, photodynamic therapy, drugs, and surgery. Anti-VEGF medication is one of the standard treatments for neovascular AMD (11–13). A large body of research exists on the pathogenesis, treatment, diagnosis, and other aspects of AMD, but relatively little on the changes in brain function related to AMD. Previous studies have confirmed that brain function changes are associated with many fundus diseases, such as retinal detachment, diabetic retinopathy, hypertensive retinopathy and so on (14–16). Therefore, this study investigates brain function of patients with AMD compared with healthy controls.
The amplitude of low-frequency fluctuation (ALFF) was used to explore its potential as a tool for early diagnosis of AMD. The technology of functional magnetic resonance imaging (fMRI) offers a means by which anomalies in brain function can be assessed and allows increased understanding of pathology of the central nervous system (17–19). Resting state functional magnetic resonance imaging (rs-fMRI) has been widely applied to monitor human brain function (20). The ALFF approach is a reliable and reproducible rs-fMRI method to evaluate the spontaneous fluctuations of resting brain activity (21–28).
At present, the ALFF method is widely used in the study of eye diseases, such as blindness,1 hypertensive retinopathy (15), strabismic amblyopia (29), unilateral acute open globe injury (30), acute eye pain (31), and corneal ulcer (32). In the present study, it is used to assess brain function in patients with AMD compared with healthy controls with the aim of better understanding the pathogenesis of AMD and to explore whether this new technology can enhance early diagnosis.
Eighteen patients with AMD (8 females and 10 males) were recruited from the Department of Ophthalmology at The First Affiliated Hospital of Nanchang University (33). These subjects met the following criteria: (1) Disease onset is related to age; (2) Fundus photographs show disease-related lesions at the macula; (3) Drusen size and quantity of abnormal pigment exceeds pre-determined minimum criteria; (4) The patient has no other eye disease; (5) The patient has no brain disease with the potential to affect brain function. Patients with mental illness or with unhealthy lifestyle factors such as alcoholism or smoking were excluded.
The two groups were similar in terms of gender, age, and weight. Control subjects were included if they satisfied the following criteria: (1) no functional abnormalities found in a head MRI (e.g., cerebral hemorrhage or cerebrovascular disease); (2) no MRI contraindications (no mental in the body, no high fever, and pregnant women with younger gestational weeks); (3) There are no risk factors for AMD, such as advanced age and smoking.
The research was approved by the Human Research Ethics Committee of the First Affiliated Hospital of Nanchang University. Each participant understood the aim, technology and possible risks of the research, and signed an informed consent agreement.
The Trio 3-Tesla MR scanner (Siemens, Munich, Germany) was used. Before scanning, participants were asked to relax, close their eyes, and minimize movement (30). To obtain functional data, a 3D metamorphic gradient echo pulse sequence was used. The following parameters were used for a 176 image scan: acquisition matrix 256 × 256; field of view 250 × 250 mm; echo time 2.26 ms; repetition time 1,900 ms; thickness 1.0 mm; gap 0.5 mm; flip angle 9°. For a 240 image scan parameters were as follows: acquisition matrix 64 × 64; field of view 220 × 220 mm; thickness 4.0 mm; gap 1.2 mm; repetition time 2,000 ms; echo time 30 ms; flip angle. 90°, 29 axial.
MRIcro software (Nottingham University, Nottingham, UK) was used to classify functional data, and to identify and exclude incomplete or flawed data. Resting-state functional MRI data were preprocessed using SPM8 (Wellcome Department of Imaging Neuroscience, London, UK) (https://www.fil.ion.ucl.ac.uk/spm/) and the REST software (REST, V1.8), (http://www.restfmri.net/forum/). Briefly, preprocessing included motion correction, spatial normalization, and spatial smoothing. The remaining data were processed using DPARSFA (V2.3) (10 volumes) (http://rfmri.org/DPARSF), including space standardization, head movement correction, slice time, digital image format conversion, and smoothing with a Gaussian kernel of 6 × 6 × 6 mm3 full width at half height. To obtain a balance among signals and to make the participants adapt to the noise generated during scanning, under normal circumstances, the first 10 volumes of functional magnetic resonance images were regarded as invalid data and were excluded. Data from participants whose maximum displacement in x, y, or z dimensions exceeded 1.5 mm or whose motion angle exceeded 15° were excluded. Linear regression was used in the present study to eliminate the influence of factors such as signals originating from white matter.
SPSS software, version 20.0 (IBM Corp., Armonk, NY, USA) was used to conduct independent sample t tests. p values less than 0.05 were considered statistically significant. Paired t-tests were carried out using REST software to analyze the functional data. Gaussian random field theory was used for multiple comparison correction, and the voxel level threshold was p < 0.005. AlphaSim, part of the REST toolbox, was used for correction at a cluster size >73 voxels and a level of p < 0.05. Receiver operating characteristic (ROC) curves provided a measure of sensitivity of mean ALFF values from brain regions of the patients and the healthy control group.
Different ALFF values in brain areas in AMD groups were categorized into regions of interest with rs-fMRI, and the relationship between the mean ALFF value in different brain areas and clinical performance was assessed by using correlation analysis (p < 0.05 significant differences).
AMD patients and HCs were aged 55.25 ± 4.04 years and 53.87 ± 5.16 respectively, and disease duration was 3.34 ± 2.88 months. The groups were statistically similar in age (p = 0.785) and weight (p = 0.542), but were statistically different in terms of best-corrected visual acuity (VA) of the left and right eyes (p = 0.004 and p = 0.003 respectively) (As described in the previous study (33), Supplementary Table S1).
From Figure 2 and Table 1, we can see that in comparison with HCs, the ALFF values of AMD patients were significantly higher in the temporal lobe, parahippocampal gyrus/limbic lobe, but significantly lower in the postcentral gyrus (Figure 2 and Table 2).
Figure 2. (A,B) Spontaneous brain activity in the AMD patients and the HC group. (C) Histogram of mean ALFF values in AMD and HCs groups. Warmer shades (yellow and red) represent moderate and high signal strength, respectively and blue represents lower signal strength. The signal value of the postcentral gyrus in AMD patients is lower than in controls, and on the contrary, the signal valuse of limbic lobe/ parahippocampa gyrus and temporal lobe are higher than controls. HCs, healthy controls; ALFF, the amplitude of low-frequency fluction; AMD, age-related macular degeneration.
The under the curve of the ROC (AUC) provides an indication of diagnostic accuracy. AUC ranges from 0 to 1, higher values indicating higher accuracy. From Figure 3, AUC for the temporal lobe and the limbic lobe/parahippocampal gyrus were 0.981 and 0.978, respectively, (p < 0.0001). The AUC of the postcentral gyrus was also high, at 0.941 (p<0.0001), indicating that ALFF is a reliable marker for differentiating between the two groups. The high diagnostic accuracy of ALFF suggests its potential utility in the early detection of AMD. However, further studies with larger sample sizes are needed to confirm these findings and assess the clinical utility of ALFF in conjunction with other diagnostic tools such as OCT imaging or visual acuity tests.
Figure 3. ROC curve analysis of the mean ALFF difference for altered brain regions. (A) The area under the ROC curve were 0.981, (p < 0.0001; 95% CI: 0.942–1.000) for Temporal Lobe, Limbic Lobe/ Parahippocampa Gyrus 0.978, (p < 0.0001; 95% CI: 0.940–1.000). (B) The area under the ROC curve were 0.941, (p < 0.0001; 95% CI: 0.863–1.000) for Postcentral Gyrus. AUC, area under the curve; ROC, receiver operating characteristic.
We can draw the conclusion from Figure 4, ALFF values of the limbic lobe/ parahippocampal gyrus were positively correlated with anxiety scores (r = 0.9312, p < 0.0001) and depression scores (r = 0.9729, p < 0.0001) in the AMD group.
Figure 4. Correlation between the ALFF value of limbic lobe/ parahippocampa gyrus with AS and DS. In the AMD group, we founded that the ALFF value of limbic lobe/ parahippocampa gyrus showed a positive correlation with the anxiety scores (A) (r = 0.9312, p<0.0001) and the depressed scores (B) (r = 0.9729, p<0.0001). ALFF, the amplitude of low-frequency fluction; AS, anxiety scores; DS, depressed scores; AMD, age-related macular degeneration.
In this study, we used the ALFF method to evaluate the spontaneous brain activity of AMD patients at rest, and the technology is widely used to study a variety of eye conditions, such as blindness (1), hypertensive retinopathy (15), strabismic amblyopia (29), unilateral acute open globe injury (30), acute eye pain (31), and corneal ulcer (Table 1) (32). At present, the incidence of AMD is increasing, and it is the main cause of blindness among the elderly, so it is worthy of attention. To date, research on this disease has focused on its clinical manifestations, pathogenesis, and treatment. The present study attempts to increase understanding of the disease by recording brain activity in AMD patients. The pathogenesis of AMD, which has received attention, usually leads to choroidal neovascularization, and this may cause fundal bleeding and even altered brain activity (Figure 5). The latter suggests that ALFF recordings may be useful in early diagnosis method for AMD.
Figure 5. Relationship between images and clinical performances in AMD. macular degeneration may lead to the formation of choroidal neovascularization, and there is a possibility of bleeding from the fundus and brain activities. AMD, age-related macular degeneration.
In our previous research, we observed different fMRI parameters in different studies. In previous study, the regional homogeneity (ReHo) method was used to investigate levels of cerebral homogeneity in individuals with AMD. Mean ReHo values at the cingulate gyrus and the superior frontal gyrus were negatively correlated with clinical symptoms (33). The present research results show that compared with HC, AMD patients have higher ALFF values in the temporal lobe/parahippocampal gyrus, and limbic lobe, and lower ALFF values in the postcentral gyrus area (Figure 6). The higher ALFF values observed in AMD patients could be a result of compensatory neural activity in response to visual loss. As the brain reorganizes due to sensory deprivation, areas traditionally involved in visual processing, such as the occipital cortex, may exhibit increased activity. This phenomenon has been observed in other forms of sensory loss and may reflect heightened neural recruitment in regions involved in sensory processing and cognitive compensation (34–36). The decreased ALFF values observed in the postcentral gyrus could reflect cortical reorganization associated with sensory deprivation. As patients with AMD experience visual loss, compensatory mechanisms might lead to a decrease in sensory processing in the somatosensory cortex, or alterations in the cross-modal plasticity between sensory areas may contribute to this effect (37). Further investigation is needed to confirm these mechanisms. The increased ALFF values observed in the temporal lobe and limbic system may reflect compensatory neural activity linked to cortical reorganization due to visual loss. Cross-modal plasticity is a well-established phenomenon where the brain adapts to sensory deprivation by enhancing neural activity in non-visual areas, such as the temporal lobe (38). Additionally, the limbic system, which is involved in emotional processing, might undergo functional alterations in response to the emotional and cognitive impacts of AMD, including anxiety, depression, or cognitive decline (39). This could contribute to the observed increased ALFF values in these regions.
Figure 6. The mean ALFF values of altered brain regions. Compared with the HCs, the ALFF values of the following regions were decreased to various extents: 1- Postcentral Gyrus (t = 4.8468). Compared with the HCs, the ALFF values of the following regions were increased to various extents: 2- Limbic Lobe/ Parahippocampa Gyrus (t = −4.8052), 3- Temporal Lobe (t = −4.398). HCs, healthy controls; BA, Brodmann’s area.
The temporal lobe is a complex brain area and is involved in various cognitive functions, such as language, memory, hearing, semantic processing, and vision (40). Previous research suggests that the temporal lobe has a role in epilepsy and autism (41), though these studies may have been affected by selective bias. One study found that picture naming triggered activation of the left posterior and inferior parts of the temporal lobe, suggesting that connected activity of parts of the temporal lobe was related to better naming performance (42). The posterior middle temporal gyrus is thought to represent a key connection between initial speech processing and subsequent semantic processing (43). Vaz et al. (44) reported that the process of episodic memory retrieval involves connection between the medial temporal lobe memory system and neocortex, and this connection may be the basis of memory retrieval. This is consistent with findings that the medial temporal lobe plays a vital role in human intermittent memory (32, 45, 46). Together with studies showing that the temporal lobe may be related to facial recognition (47), this body of research highlights the role of the temporal lobe in multiple functions. The present study found that temporal lobe ALFF values are higher in patients with AMD than in controls, indicating that these functions may be abnormal in AMD (Table 3).
The parahippocampal gyrus is the core part of the limbic system, which plays a significant part in emotion and memory (48), especially visual memory (49), but not familiarity (50). Karanian et al. (51) found that false memory generated more activity in the parahippocampal cortex than true memory, suggesting that this area mediates context rather than sensory processing. Other research has shown that parahippocampus activity is related to negative emotions (52). In the present study, the ALFF value at the parahippocampal gyrus was higher in AMD patients than in controls, which may indicate overactivation of the limbic system, possibly accompanied by negative emotion.
In addition to emotion, the limbic system processes sensory input from the internal and external environment related to movement and cognitive functions (53). Because the limbic lobe is involved in the sense of smell and related functions, it is also called the rhinencephalon, or olfactory brain (54). Higher ALFF values of the limbic lobe in AMD may be accompanied by changes in smell, cognition, and other functions.
Finally, in the present study ALFF values at the postcentral gyrus are reduced in AMD patients compared with controls, which is contrary to previous observations, the possible reasons are the different sample sizes between this study and previous studies, as well as the factors affecting the experimental results of the subject’s own conditions. The postcentral gyrus is located in the main somatosensory cortex (S1) and plays an important role in sensation (55). A functional imaging study showed that the S1 activity observed during voluntary movement of the limbs is related to the activity of the movement area, and is not triggered by passive movement (56). Functional imaging has also shown that hemiplegic cerebral palsy is related to abnormal processing in the ipsilateral primitive somatosensory cortex (57). Studies have proposed that the S1 cortex is important in the location and discrimination of pain (58), and the plasticity of S1 plays a role in neuropathic pain (59). Lenoir et al. (60) found that S1 is involved in sensing vibration and in nociception. Together with these findings, the observation of low postcentral gyrus ALFF values in AMD patients in the present study suggests that these patients have limited function related to central posterior gyrus functional activity.
In addition to the above results, we also conducted a comparative study on the emotional-related clinical manifestations of AMD patients. The results show that in limbic lobe/ parahippocampa gyrus, the ALFF value is positively correlated with the AS and DS scores, which may indicate spontaneously hyperactive brain regions may be functionally active and cause emotional problems such as anxiety and depressed. Therefore, we can assume that areas with low spontaneous activity in the brain may trigger the opposite behavior.
Despite the AMD findings were explored in this study, there are some limitations. The ROC curve analysis demonstrated promising results, with a high AUC value, indicating the potential of ALFF as a diagnostic biomarker for AMD. However, these findings should be interpreted cautiously given the relatively small sample size, and further validation in larger cohorts is necessary. Future studies could also explore the combined use of ALFF with other clinical biomarkers, such as visual acuity or OCT, to enhance diagnostic precision and improve clinical outcomes. Meanwhile, potential confounding factors, such as AMD somatotype, could influence ALFF results. Future studies should stratify AMD patients into subgroups based on these factors to explore their effect on brain activity and better understand the underlying mechanisms of altered ALFF in AMD. We recognize that the relatively small sample size in this study may limit the statistical power and generalizability of the findings. Future studies with larger, multicenter cohorts are needed to validate these results and explore the broader applicability of ALFF as a diagnostic marker for AMD.
In conclusion, our study demonstrates that ALFF alterations in specific brain regions, including the occipital cortex, temporal lobe, and limbic system, could serve as potential biomarkers for the early detection of age-related macular degeneration (AMD). These findings suggest that changes in spontaneous brain activity may reflect neural adaptations to sensory deprivation. Future studies should explore the longitudinal dynamics of ALFF changes as AMD progresses, and investigate the potential of ALFF in combination with other diagnostic tools, such as OCT and visual acuity assessments, to enhance early diagnosis and therapeutic monitoring.
The datasets used and analyzed during the current study are available corresponding author on reasonable request. The corresponding author should be contacted if someone wants to request the data from this study.
All research methods were approved by the Medical Ethics Committee of the First Affiliated Hospital of Nanchang University (CDYFY- LL- 2021039) and complied with the Helsinki Declaration. The whole study was carried out in accordance with the approved guidelines. The patients/participants provided their written informed consent to participate in this study.
YZ: Conceptualization, Writing – original draft. JH: Data curation, Writing – original draft. QL: Methodology, Writing – original draft. SX: Formal analysis, Writing – original draft. MK: Investigation, Writing – original draft. HW: Formal analysis, Writing – original draft. JZ: Software, Validation, Writing – original draft. QY: Conceptualization, Writing – review & editing. GT: Supervision, Writing – review & editing. YS: Supervision, Writing – review & editing.
The author(s) declare that no financial support was received for the research, authorship, and/or publication of this article.
The authors declare that the research was conducted in the absence of any commercial or financial relationships that could be construed as a potential conflict of interest.
The authors declare that no Generative AI was used in the creation of this manuscript.
All claims expressed in this article are solely those of the authors and do not necessarily represent those of their affiliated organizations, or those of the publisher, the editors and the reviewers. Any product that may be evaluated in this article, or claim that may be made by its manufacturer, is not guaranteed or endorsed by the publisher.
The Supplementary material for this article can be found online at: https://www.frontiersin.org/articles/10.3389/fmed.2024.1507971/full#supplementary-material
AMD, Age-related macular degeneration; ALFF, The amplitude of low-frequency fluctuation; HCs, Healthy controls; ROC, The receiver operating characteristic; rs-fMRI, Resting state functional magnetic resonance imaging; AUC, The under the curve of the ROC.
1. Li, Q, Huang, X, Ye, L, Wei, R, Zhang, Y, Zhong, YL, et al. Altered spontaneous brain activity pattern in patients with late monocular blindness in middle-age using amplitude of low-frequency fluctuation: a resting-state functional MRI study. Clin Interv Aging. (2016) 11:1773–80. doi: 10.2147/CIA.S117292
2. Thomas, CJ, Mirza, RG, and Gill, MK. Age-related macular degeneration. Med Clin North Am. (2021) 105:473–91. doi: 10.1016/j.mcna.2021.01.003
3. Lim, LS, Mitchell, P, Seddon, JM, Holz, FG, and Wong, TY. Age-related macular degeneration. Lancet. (2012) 379:1728–38. doi: 10.1016/S0140-6736(12)60282-7
4. Qi, HJ, Li, XX, Zhang, JY, and Zhao, MW. Efficacy and safety of ranibizumab for wet age-related macular degeneration in Chinese patients. Int J Ophthalmol. (2017) 10:91–7. doi: 10.18240/ijo.2017.01.15
5. Schnabolk, G. Systemic inflammatory disease and AMD comorbidity. Adv Exp Med Biol. (2019) 1185:27–31. doi: 10.1007/978-3-030-27378-1_5
6. Al-Zamil, WM, and Yassin, SA. Recent developments in age-related macular degeneration: a review. Clin Interv Aging. (2017) 12:1313–30. doi: 10.2147/CIA.S143508
7. Kijlstra, A, and Berendschot, TT. Age-related macular degeneration: a complementopathy? Ophthalmic Res. (2015) 54:64–73. doi: 10.1159/000432401
8. Gheorghe, A, Mahdi, L, and Musat, O. Age-related macular degeneration. Rom J Ophthalmol. (2015) 59:74–7.
9. Ferris, FL 3rd, Wilkinson, CP, Bird, A, Chakravarthy, U, Chew, E, Csaky, K, et al. Beckman Initiative for Macular Research classification committee. Clinical classifi- cation of age-related macular degeneration. Ophthalmology. (2013) 120:844–51. doi: 10.1016/j.ophtha.2012.10.036
10. DeAngelis, MM, Owen, LA, Morrison, MA, Morgan, DJ, Li, M, Shakoor, A, et al. Genetics of age-related macular degeneration (AMD). Hum Mol Genet. (2017) 26:R45–50. doi: 10.1093/hmg/ddx228
11. Staels, W, Heremans, Y, Heimberg, H, and de Leu, N. VEGF-A and blood vessels: a beta cell perspective. Diabetologia. (2019) 62:1961–8. doi: 10.1007/s00125-019-4969-z
12. Han, KY, Chang, JH, Lee, H, and Azar, DT. Proangiogenic interactions of vascular endothelial MMP14 with VEGF receptor 1 in VEGFA-mediated corneal angiogenesis. Invest Ophthalmol Vis Sci. (2016) 57:3313–22. doi: 10.1167/iovs.16-19420
13. Tah, V, Orlans, HO, Hyer, J, Casswell, E, Din, N, Sri Shanmuganathan, V, et al. Anti-VEGF therapy and the retina: an update. J Ophthalmol. (2015) 2015:1–13. doi: 10.1155/2015/627674
14. Chen, SY, Cai, GQ, Liang, RB, Yang, QC, Min, YL, Ge, QM, et al. Regional brain changes in patients with diabetic optic neuropathy: a resting-state functional magnetic resonance imaging study. Quant Imaging Med Surg. (2021) 11:2125–37. doi: 10.21037/qims-20-453
15. Chen, MJ, Wu, SN, Shu, HY, Ge, QM, Pan, YC, Zhang, LJ, et al. Spontaneous functional changes in specific cerebral regions in patients with hypertensive retinopathy: a resting-state functional magnetic resonance imaging study. Aging (Albany NY). (2021) 13:13166–78. doi: 10.18632/aging.202999
16. Shao, Y, Yang, L, Zhu, PW, Su, T, Zhou, XZ, Li, B, et al. Functional connectivity density alterations in middle-age retinal detachment patients. Brain Behav. (2021) 11:e01783. doi: 10.1002/brb3.1783
17. Bekiesińska-Figatowska, M, Helwich, E, Rutkowska, M, Stankiewicz, J, and Terczyńska, I. Magnetic resonance imaging of neonates in the magnetic resonance compatible incubator. Arch Med Sci. (2016) 12:1064–70. doi: 10.5114/aoms.2016.61913
18. Liu, H, and Wang, X. Correlation of iron deposition and change of gliocyte metabolism in the basal ganglia region evaluated using magnetic resonance imaging techniques: an in vivo study. Arch Med Sci. (2016) 12:163–71. doi: 10.5114/aoms.2016.57593
19. Wang, Y, Shao, Y, Shi, WQ, Jiang, L, Wang, XY, Zhu, PW, et al. The predictive potential of altered spontaneous brain activity patterns in diabetic retinopathy and nephropathy. EPMA J. (2019) 10:249–59. doi: 10.1007/s13167-019-00171-4
20. Fox, MD, and Greicius, M. Clinical applications of resting state functional connectivity. Front Syst Neurosci. (2010) 4:19. doi: 10.3389/fnsys.2010.00019
21. Zhang, Y, Zhu, C, Chen, H, Duan, X, Lu, F, Li, M, et al. Frequency-dependent alterations in the amplitude of low-frequency fluctuations in social anxiety disorder. J Affect Disord. (2015) 174:329–35. doi: 10.1016/j.jad.2014.12.001
22. Hoptman, MJ, Zuo, XN, Butler, PD, Javitt, DC, D'Angelo, D, Mauro, CJ, et al. Amplitude of low-frequency oscillations in schizophrenia: a resting state fMRI study. Schizophr Res. (2010) 117:13–20. doi: 10.1016/j.schres.2009.09.030
23. Zuo, XN, di, A, Kelly, C, Shehzad, Z, Gee, D, Klein, D, et al. The oscillating brain: complex and reliable. NeuroImage. (2010) 49:1432–45. doi: 10.1016/j.neuroimage.2009.09.037
24. Turner, JA, Damaraju, E, van Erp, TG, Mathalon, DH, Ford, JM, Voyvodic, J, et al. A multi-site resting state fMRI study on the amplitude of low frequency fluctuations in schizophrenia. Front Neurosci. (2013) 7:137. doi: 10.3389/fnins.2013.00137
25. Xu, J, Rees, G, Yin, X, Song, C, Han, Y, Ge, H, et al. Spontaneous neuronal activity predicts intersubject variations in executive control of attention. Neuroscience. (2014) 263:181–92. doi: 10.1016/j.neuroscience.2014.01.020
26. Dong, M, Li, J, Shi, X, Gao, S, Fu, S, Liu, Z, et al. Altered baseline brain activity in experts measured by amplitude of low frequency fluctuations (ALFF): a resting state fMRI study using expertise model of acupuncturists. Front Hum Neurosci. (2015) 9:99. doi: 10.3389/fnhum.2015.00099
27. Zang, YF, He, Y, Zhu, CZ, Cao, QJ, Sui, MQ, Liang, M, et al. Altered baseline brain activity in children with ADHD revealed by resting-state functional MRI. Brain and Development. (2007) 29:83–91. doi: 10.1016/j.braindev.2006.07.002
28. Shao, Y, Cai, FQ, Zhong, YL, Huang, X, Zhang, Y, Hu, PH, et al. Altered intrinsic regional spontaneous brain activity in patients with optic neuritis: a resting-state functional magnetic resonance imaging study. Neuropsychiatr Dis Treat. (2015) 11:3065–73. doi: 10.2147/NDT.S92968
29. Wang, YN, Pan, YC, Shu, HY, Zhang, LJ, Li, QY, Ge, QM, et al. Altered spontaneous brain activity patterns in children with strabismic amblyopia after low-frequency repetitive transcranial magnetic stimulation: a resting-state functional magnetic resonance imaging study. Front Hum Neurosci. (2022) 16:790678. doi: 10.3389/fnhum.2022.790678
30. Tan, G, Huang, X, Ye, L, Wu, AH, He, LX, Zhong, YL, et al. Altered spontaneous brain activity patterns in patients with unilateral acute open globe injury using amplitude of low-frequency fluctuation: a functional magnetic resonance imaging study. Neuropsychiatr Dis Treat. (2016) 12:2015–20. doi: 10.2147/NDT.S110539
31. Kang, HH, Shu, YQ, Yang, L, Zhu, PW, Li, D, Li, QH, et al. Measuring abnormal intrinsic brain activities in patients with retinal detachment using amplitude of low-frequency fluctuation: a resting-state fMRI study. Int J Neurosci. (2019) 129:681–6. doi: 10.1080/00207454.2018.1554657
32. Shi, WQ, Wu, W, Ye, L, Jiang, N, Liu, WF, Shu, YQ, et al. Altered spontaneous brain activity patterns in patients with corneal ulcer using amplitude of low-frequency fluctuation: an fMRI study. Exp Ther Med. (2019) 18:125–32. doi: 10.3892/etm.2019.7550
33. Liu, QY, Pan, YC, Shu, HY, Zhang, LJ, Li, QY, Ge, QM, et al. Brain activity in age-related macular degeneration patients from the perspective of regional homogeneity: a resting-state functional magnetic resonance imaging study. Front Aging Neurosci. (2022) 14:865430. doi: 10.3389/fnagi.2022.865430
34. Liu, T, Cheung, SH, Schuchard, RA, Glielmi, CB, Hu, X, He, S, et al. Incomplete cortical reorganization in macular degeneration. Invest Ophthalmol Vis Sci. (2010) 51:6826–34. doi: 10.1167/iovs.09-4926
35. Norman, LJ, Hartley, T, and Thaler, L. Changes in primary visual and auditory cortex of blind and sighted adults following 10 weeks of click-based echolocation training. Cereb Cortex. (2024) 34:239. doi: 10.1093/cercor/bhae239
36. Voss, P. Brain (re)organization following visual loss. Wiley Interdiscip Rev Cogn Sci. (2019) 10:e1468. doi: 10.1002/wcs.1468
37. Mowad, TG, Willett, AE, Mahmoudian, M, Lipin, M, Heinecke, A, Maguire, AM, et al. Compensatory cross-modal plasticity persists after sight restoration. Front Neurosci. (2020) 14:291. doi: 10.3389/fnins.2020.00291
38. Amedi, A, Stern, WM, Camprodon, JA, Bermpohl, F, Merabet, L, Rotman, S, et al. Shape conveyed by visual-to-auditory sensory substitution activates the lateral occipital complex. Nat Neurosci. (2007) 10:687–9. doi: 10.1038/nn1912
39. Zeni, ALB, Camargo, A, and Dalmagro, AP. Lutein prevents corticosterone-induced depressive-like behavior in mice with the involvement of antioxidant and neuroprotective activities. Pharmacol Biochem Behav. (2019) 179:63–72. doi: 10.1016/j.pbb.2019.02.004
40. Yang, L, Yan, Y, Wang, Y, Hu, X, Lu, J, Chan, P, et al. Gradual disturbances of the amplitude of low-frequency fluctuations (ALFF) and fractional ALFF in alzheimer spectrum. Front Neurosci. (2018) 12:975. doi: 10.3389/fnins.2018.00975
41. Arain, AM, Azar, NJ, Lagrange, AH, McLean, M, Singh, P, Sonmezturk, H, et al. Temporal lobe origin is common in patients who have undergone epilepsy surgery for hypermotor seizures. Epilepsy Behav. (2016) 64:57–61. doi: 10.1016/j.yebeh.2016.09.019
42. Trimmel, K, van Graan, AL, Caciagli, L, Haag, A, Koepp, MJ, Thompson, PJ, et al. Left temporal lobe language network connectivity in temporal lobe epilepsy. Brain. (2018) 141:2406–18. doi: 10.1093/brain/awy164
43. Middlebrooks, EH, Yagmurlu, K, Szaflarski, JP, Rahman, M, and Bozkurt, B. A contemporary framework of language processing in the human brain in the context of preoperative and intraoperative language mapping. Neuroradiology. (2017) 59:69–87. doi: 10.1007/s00234-016-1772-0
44. Vaz, AP, Inati, SK, Brunel, N, and Zaghloul, KA. Coupled ripple oscillations between the medial temporal lobe and neocortex retrieve human memory. Science. (2019) 363:975–8. doi: 10.1126/science.aau8956
45. Miller, JF, Neufang, M, Solway, A, Brandt, A, Trippel, M, Mader, I, et al. Neural activity in human hippocampal formation reveals the spatial context of retrieved memories. Science. (2013) 342:1111–4. doi: 10.1126/science.1244056
46. Eichenbaum, H. On the integration of space, time, and memory. Neuron. (2017) 95:1007–18. doi: 10.1016/j.neuron.2017.06.036
47. Op de Beeck, HP, Dicarlo, JJ, Goense, JB, Grill-Spector, K, Papanastassiou, A, Tanifuji, M, et al. Fine-scale spatial organization of face and object selectivity in the temporal lobe: do functional magnetic resonance imaging, optical imaging, and electrophysiology agree? J Neurosci. (2008) 28:11796–801. doi: 10.1523/JNEUROSCI.3799-08.2008
48. Catani, M, Dell'acqua, F, and Thiebaut de Schotten, M. A revised limbic system model for memory, emotion and behaviour. Neurosci Biobehav Rev. (2013) 37:1724–37. doi: 10.1016/j.neubiorev.2013.07.001
49. Düzel, E, Habib, R, Rotte, M, Guderian, S, Tulving, E, and Heinze, HJ. Human hippocampal and parahippocampal activity during visual associative recognition memory for spatial and nonspatial stimulus configurations. J Neurosci. (2003) 23:9439–44. doi: 10.1523/JNEUROSCI.23-28-09439.2003
50. Eichenbaum, H, Yonelinas, AP, and Ranganath, C. The medial temporal lobe and recognition memory. Annu Rev Neurosci. (2007) 30:123–52. doi: 10.1146/annurev.neuro.30.051606.094328
51. Karanian, JM, and Slotnick, SD. False memory for context activates the parahippocampal cortex. Cogn Neurosci. (2014) 5:186–92. doi: 10.1080/17588928.2014.938035
52. Chan, E, Baumann, O, Bellgrove, MA, and Mattingley, JB. Negative emotional experiences during navigation enhance parahippocampal activity during recall of place information. J Cogn Neurosci. (2014) 26:154–64. doi: 10.1162/jocn_a_00468
53. Zhao, D, Zhang, Z, Lu, H, Cheng, S, Si, B, and Feng, X. Learning Cognitive Map Representations for Navigation by Sensory-Motor Integration. IEEE Trans Cybern. (2022) 52:508–21. doi: 10.1109/TCYB.2020.2977999
54. Roxo, MR, Franceschini, PR, Zubaran, C, Kleber, FD, and Sander, JW. The limbic system conception and its historical evolution. ScientificWorldJournal. (2011) 11:2428–41. doi: 10.1100/2011/157150
55. Kaukoranta, E, Hämäläinen, M, Sarvas, J, and Hari, R. Mixed and sensory nerve stimulations activate different cytoarchitectonic areas in the human primary somatosensory cortex SI. Neuromagnetic recordings and statistical considerations. Exp Brain Res. (1986) 63:60–6.
56. Cui, F, Arnstein, D, Thomas, RM, Maurits, NM, Keysers, C, and Gazzola, V. Functional magnetic resonance imaging connectivity analyses reveal efference-copy to primary somatosensory area, BA2. PLoS One. (2014) 9:e84367. doi: 10.1371/journal.pone.0084367
57. Papadelis, C, Butler, EE, Rubenstein, M, Sun, L, Zollei, L, Nimec, D, et al. Reorganization of the somatosensory cortex in hemiplegic cerebral palsy associated with impaired sensory tracts. Neuroimage Clin. (2018) 17:198–212. doi: 10.1016/j.nicl.2017.10.021
58. Bushnell, MC, Duncan, GH, Hofbauer, RK, Ha, B, Chen, JI, and Carrier, B. Pain perception: is there a role for primary somatosensory cortex? Proc Natl Acad Sci USA. (1999) 96:7705–9. doi: 10.1073/pnas.96.14.7705
59. Seifert, F, and Maihöfner, C. Central mechanisms of experimental and chronic neuropathic pain: findings from functional imaging studies. Cell Mol Life Sci. (2009) 66:375–90. doi: 10.1007/s00018-008-8428-0
Keywords: the amplitude of low-frequency fluctuation, age-related macular degeneration, spontaneous brain activity, functional magnetic resonance imaging, resting brain activity
Citation: Zhang YY, Hu JY, Ling Q, Xu SH, Kang M, Wei H, Zou J, Yi Q, Tan G and Shao Y (2025) The amplitude of low frequency fluctuation and spontaneous brain activity alterations in age-related macular degeneration. Front. Med. 11:1507971. doi: 10.3389/fmed.2024.1507971
Received: 08 October 2024; Accepted: 17 December 2024;
Published: 22 January 2025.
Edited by:
Shujun Wang, Hong Kong Polytechnic University, Hong Kong SAR, ChinaReviewed by:
Neetu Kushwah, Harvard Medical School, United StatesCopyright © 2025 Zhang, Hu, Ling, Xu, Kang, Wei, Zou, Yi, Tan and Shao. This is an open-access article distributed under the terms of the Creative Commons Attribution License (CC BY). The use, distribution or reproduction in other forums is permitted, provided the original author(s) and the copyright owner(s) are credited and that the original publication in this journal is cited, in accordance with accepted academic practice. No use, distribution or reproduction is permitted which does not comply with these terms.
*Correspondence: Yi Shao, ZnJlZWJlZTk5QDE2My5jb20=; Gang Tan, dGFuZ2FuZzk5QGhvdG1haWwuY29t; Quanyong Yi, eWlxdWFueW9uZ3RlYW1AMTYzLmNvbQ==
†These authors have contributed equally to this work
Disclaimer: All claims expressed in this article are solely those of the authors and do not necessarily represent those of their affiliated organizations, or those of the publisher, the editors and the reviewers. Any product that may be evaluated in this article or claim that may be made by its manufacturer is not guaranteed or endorsed by the publisher.
Research integrity at Frontiers
Learn more about the work of our research integrity team to safeguard the quality of each article we publish.