- 1Dongguan Tungwah Hospital, Dongguan, China
- 2Shenzhen Eye Hospital, Shenzhen Eye Institute, Shenzhen Eye Hospital Affiliated to Jinan University, Shenzhen, China
Purpose: To assess the safe, lowest effective laser power of subthreshold micropulse laser (SML) for treating acute and chronic central serous chorioretinopathy (CSC) in Chinese patients.
Methods: Patients were distinguished with acute or chronic CSC based on focal or diffuse retinal pigment epithelium (RPE) leakage on fundus fluorescein angiography (FFA), with or without widespread RPE decompensation. Patients were categorized into five groups and treated with 577 nm yellow SML according to 50% titration power. The change of best-corrected visual acuity (BCVA) and central macular thickness (CMT) were set as primary outcomes. A linear regression model assessed the correlation between different factors and outcome indicators.
Results: A total of 103 patients with 127 eyes (61 with acute CSC and 66 with chronic CSC) were enrolled. The baseline characteristics were balanced between the five groups (all p > 0.05). The decrease of CMT and the improvement of BCVA were related to the CMT at baseline (all p < 0.05). We found that the lowest effective laser power for acute CSC was 425 mW (−225.50 μm vs. −171.24 μm vs. −114.50 μm vs. −130.54 μm vs. −68.00 μm, p < 0.001), showing a significant CMT reduction at this power, but no significant increase in BCVA (−0.15 ± 0.10 logMAR vs. −0.20 ± 0.16 logMAR vs. −0.14 ± 0.11 logMAR vs. −0.17 ± 0.30 logMAR vs. −0.11 ± 0.14 logMAR, p > 0.05). For chronic CSC, the lowest effective laser power was 375 mW (p = 0.01), the change of CMT was significant in 375 mW (−93.91 ± 109.06 μm, −119.32 ± 105.56 μm, −88.67 ± 67.26 μm, −60.89 ± 106.86 μm, and −99.11 ± 157.32 μm, p = 0.04). The change of BCVA was similar trend (−0.54 ± 0.66 logMAR vs. −0.17 ± 0.23 logMAR vs. −0.10 ± 0.21 logMAR vs. −0.02 ± 0.30 logMAR vs. 0.05 ± 0.19 logMAR, p < 0.001).
Conclusion: In this study, our results suggested 425 mW and 375 mW laser power is the lowest effective SML power for treating acute and chronic CSC in Chinese patients respectively, And the power of SML for chronic CSC requires lower power than acute CSC.
Introduction
Central serous chorioretinopathy (CSC) is a common chorioretinal disease characterized by an accumulation of subretinal fluid (SRF). The exact pathogenesis is subject to debate, but it was thought to be the serous detachment of the neurosensory retina and the change in the retinal pigment epithelium (RPE) (1, 2). It commonly presents with metamorphopsia, central scotoma, and progressive visual damage. CSC was usually subdivided into acute and chronic CSC. Acute CSC is usually thought to be a self-limited disorder, which resolves within few months with minor permanent damage to vision. In comparison, chronic CSC can lead to clinically significant central vision loss and reduced quality of life (3). However, according to the research reported, the risk of recurrence for acute CSC could be about 50, and 15% might develop persistent SRF, with a duration longer than 4 months would be defined as persistent chronic CSC (4, 5), which could result in irreversible visual loss (6, 7). Therefore, acute CSC need early intervention to accelerate the resolution of SRF, shorten the disease duration and help visual acuity improvement more quickly (8).
Photodynamic therapy (PDT), laser photocoagulation, and intravitreal injection of anti-vascular endothelial growth factor (VEGF) are commonly employed clinical treatments for CSC (9). ICG-guided half-dose PDT has traditionally been regarded as the gold standard for managing CSC. However, due to the high cost and limited availability of verteporfin in China, Subthreshold Micropulse Laser (SML) has emerged as a promising alternative. Unlike conventional lasers that deliver continuous pulses, SML emits energy in a series of micropulses, which reduces the duration of heat conduction and enables repeated micropulse stimulation to promote RPE repair (10). This technique also limits the temperature increase in surrounding tissues, minimizing the risk of significant retinal damage (11, 12).
SML has become a well-established technique in the clinical management of CSC. However, more studies needs to focus on the optimal laser parameters for SML, including exposure time, spot diameter, power, and treatment area selection. While Lijun Zhou et al. have reported differences in efficacy with varying duty cycles (8), the impact of different laser energy levels still needs to be explored. Researches on SML parameters were predominantly led by European and American ophthalmologists (13, 14), with limited studies conducted in China, likely due to the relatively few SML devices available and the challenges in determining optimal treatment settings (9, 15). Additionally, Anastasia V Pilat’s work suggests racial differences in RPE thickness, with Asians exhibiting thicker RPE than Caucasians (16), implying that treatment effects and optimal SML power settings for CSC may vary between populations. Given China’s large population and the growing number of CSC patients receiving micropulse therapy, it is crucial to investigate SML parameters tailored to the Chinese population. Consequently, this study was designed to establish a cohort of 103 CSC patients in Shenzhen, China, to determine the lowest safe and effective SML power for treating acute and chronic CSC in this demographic.
Methods
Study design
This retrospective, nonrandom, comparative cohort clinical trial was conducted at the Shenzhen Eye Hospital Affiliated to Jinan University, Shenzhen, China. Institutional medical ethics committee approval was obtained (The Ethic number 2023KYPJ019). All interventions were conducted according to the tenets of the Declaration of Helsinki. All patients provided written informed consent to participate in this trial.
Participants
The patients diagnosed with CSC based on clinical characteristics and findings on multimodal imaging were enrolled in this study. Criteria for inclusion were as follows: (1) 18 years old or older; (2) Acute CSC: disease duration was less than 4 months, FFA showed rapid accumulation of fluorescein under the neuroepithelium, and fluorescein leakage was ink diffusion type or smoke type (9). (3) Chronic CSC: disease duration greater than 6 months, FFA showed single or multiple focal, diffuse leakage of fluorescein in the macular area, with or without widespread RPE decompensation (9). Criteria for exclusion were: (1) patients who received PDT or laser treatment previously for CSC; (2) patients were diagnosed with other choroidal or retinal diseases such as CNV, diabetic retinopathy, and age-related macular degeneration; (3) patients who accepted ocular surgery during the study follow-up period.
All patients underwent ophthalmic examinations prior to the treatment, including best corrected visual acuity (BCVA) using LogMAR charts, dilated fundus examination, fundus photography, central macular thickness (CMT), and subretinal fluid (SRF) measured by spectral-domain optical coherence tomography (SD-OCT) (Cirrus HD OCT-5000, Zeiss, Jena, Germany), fundus fluorescein angiography (FFA), fundus auto-fluorescein (FAF) and indocyanine green angiography (ICGA) (Spectralis HRA, Heidelberg Engineering, Heidelberg, Germany). In the third month after treatment, patients were reassessed, and BCVA, fundus, and OCT examinations were performed. All treatments were performed by certified ophthalmologists with more than 20 years of experience.
Treatment procedures
The SML treatment was performed with 577 nm yellow laser system (Supra Scan, Quantell Medical, Cedex, France) by a single retina specialist (QS Chen). The laser parameter was set to 5% duty cycle, and the exposure time was 0.2 s per spot. The spot sizes 140–160 μm were used, and the total laser burns were 100–800. The multi-spot model without spacing between the spots was chosen. The photocoagulation energy for each patient was titrated at the nasal upper quadrant of the retina in the mono-spot micropulse model. The power titration would gradually increase until a visible burn was seen, and then the power was reduced by 50% for individual treatment. Multimodal imaging of a CSC patient treated with SML is depicted in Figure 1 (acute CSC) and Figure 2 (chronic CSC). After finishing SML treatment, 127 eyes were divided into five treatment groups based on the titration power: (1) below 375 mW, (2) 375 mW, (3) 400 mW, (4) 425 mW, (5) above 425 mW. The follow-up flowchart is outlined in Figure 3.
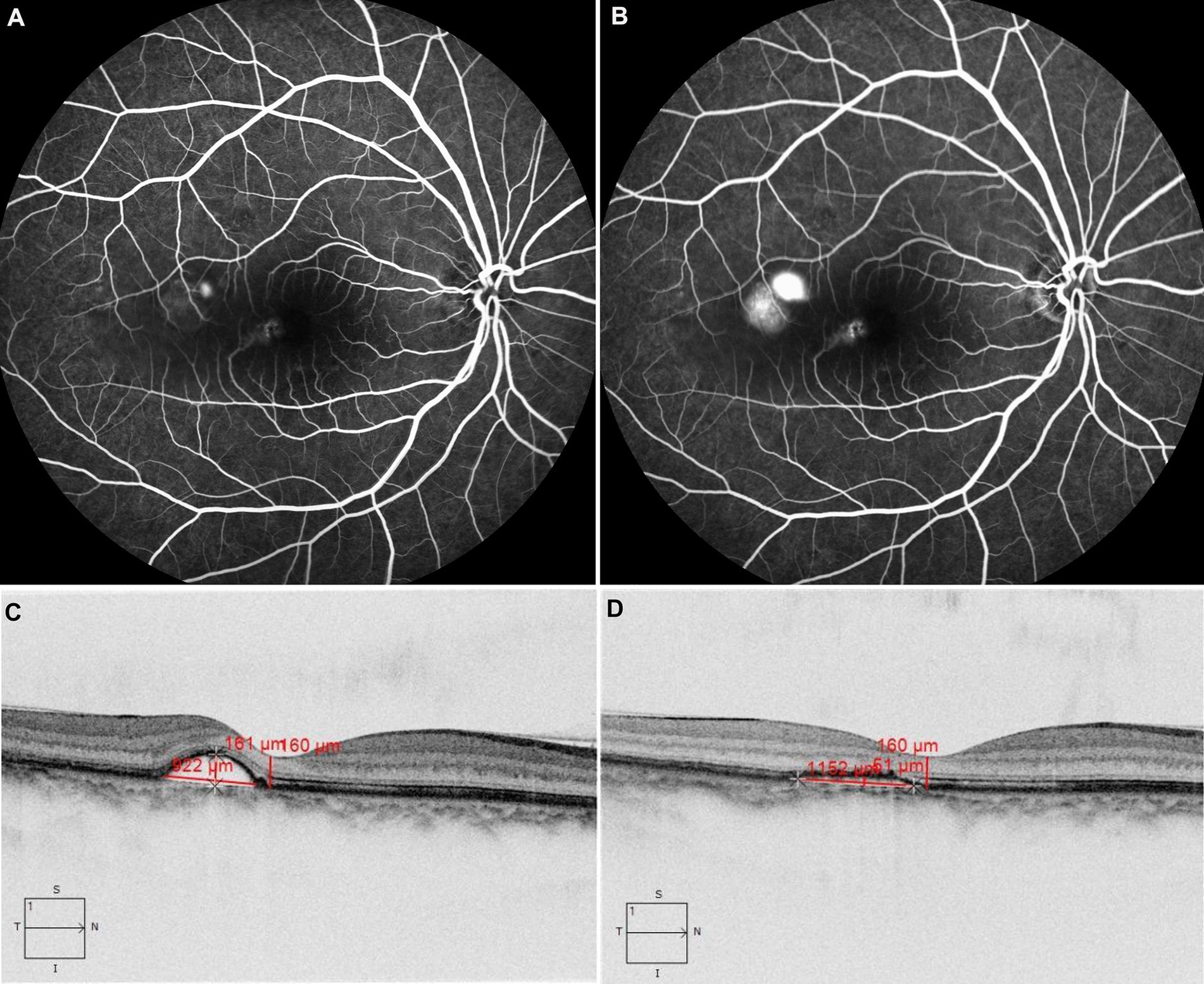
Figure 1. The patient with acute CSC received 577 nm yellow SML with 425 mW laser power. FFA revealed dye leakage located just above the macula in the early phase in panel (A) and subsequent pooling involving the macula in panel (B). OCT showed retinal neuroepithelium detachment at the temporal side of the fovea (C), after treatment, compared with that before treatment (C). OCT showed subretinal fluid was absorbed completely at the third month (D).
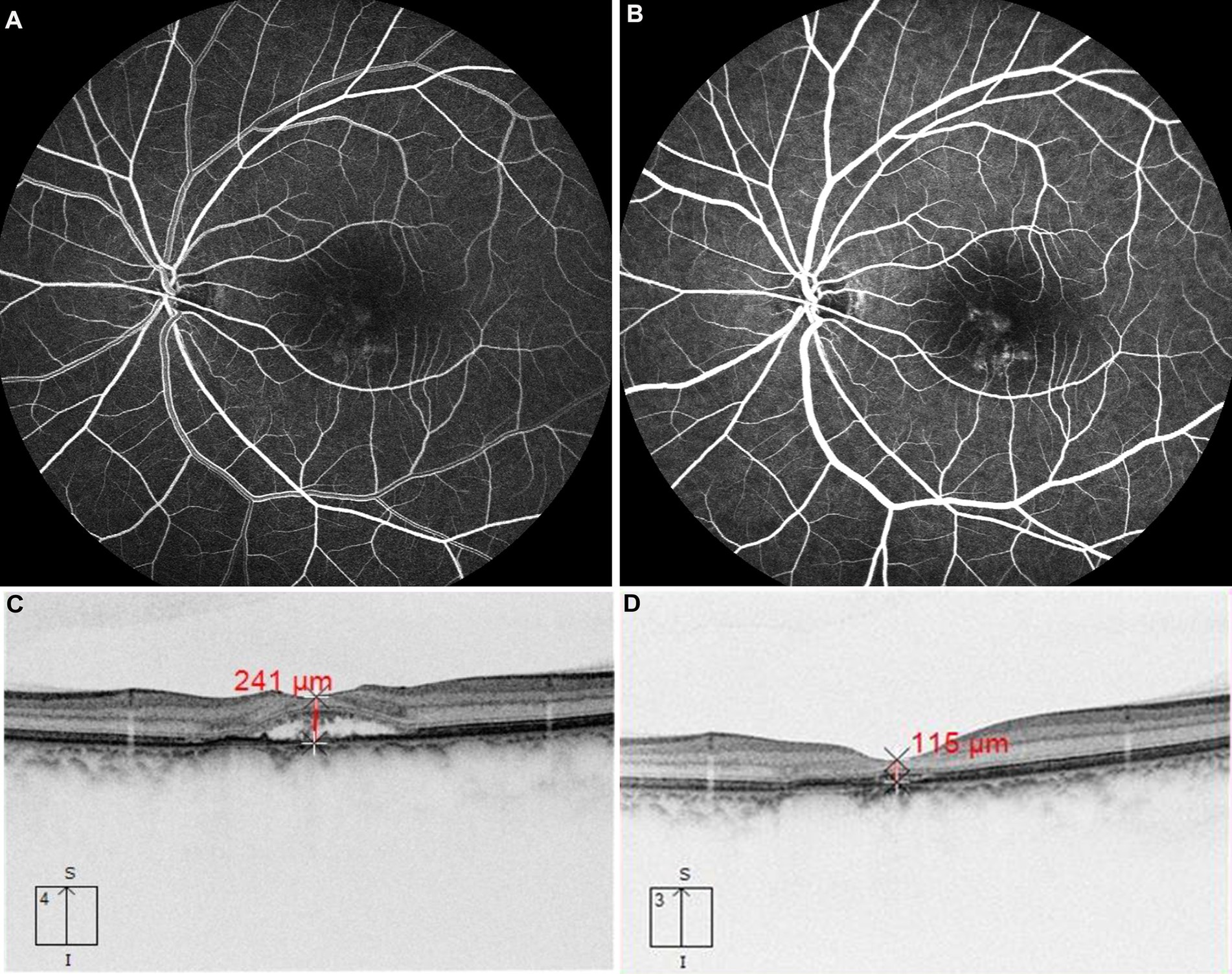
Figure 2. The patient with chronic CSC received 577 nm yellow SML with above 425 mW laser power. FFA revealed dye leakage located just below the macula in the early phase in panel (A) and subsequent pooling involving the macula in panel (B). OCT showed retinal neuroepithelium detachment at the fovea (C), after treatment, compared with that before treatment (C). OCT showed subretinal fluid was absorbed completely at the third month (D).
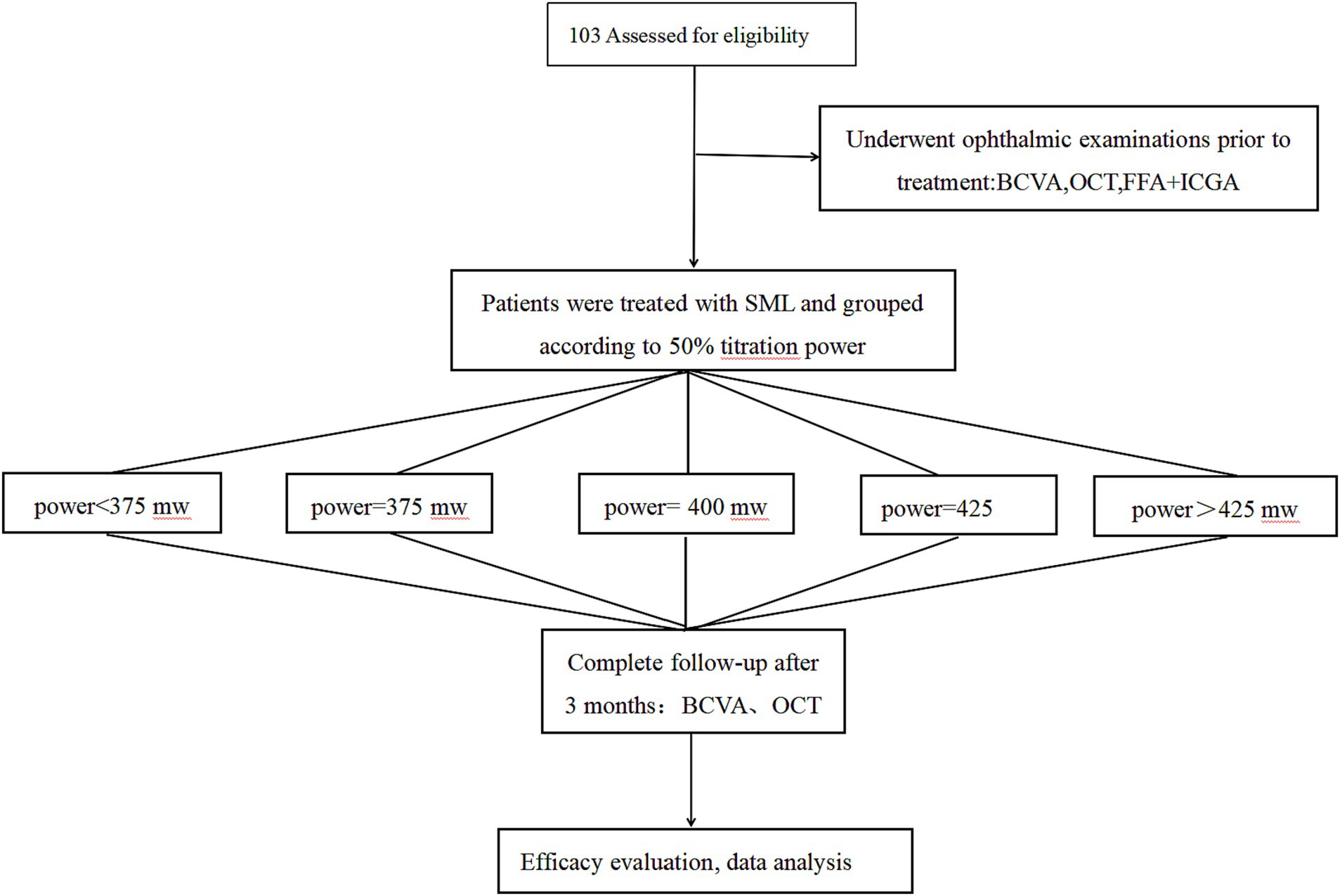
Figure 3. Study follow-up flowchart. SML, Subthreshold micropulse laser; BCVA, best-corrected visual acuity; OCT, optical coherence tomography; FFA, fundus fluorescein angiography; ICGA, indocyanine green angiography.
Outcome measurements
The primary outcomes included the change of BCVA and CMT from baseline to the third month. CMT was defined as the distance between the inner limiting membrane and the ellipsoid zone at the location with the maximal foveal dip on the SD-OCT scan (17). The secondary outcome included the complete absorption of SRF, defined as the CMT thinner than 220 μm in the third month.
Statistical analyses
All analyses were performed using STATA statistical software (Stata V.14.0, Stata). BCVA was converted to the logarithm of the minimal angle of resolution (LogMAR) before statistical analyses. Data were presented as ‘mean ± standard deviation’. CMT and BVCA were used as continuous variables. SML power was used as a categorical variable. The linear regression model was used to assess the association of five different kinds of SML power with different factors and outcome indicators. A p-value less than 0.05 was considered statistically significant.
Results
We enrolled 127 eyes from 103 CSC patients (76 male and 27 female) in Shenzhen Eye Hospital from December 2019 to December 2022. Sixty-one eyes (48.03%) were diagnosed with acute CSC, and 66 (51.97%) were chronic CSC. At baseline, the mean age of patients was 46.64 ± 9.71 years, the mean BCVA was 0.42 ± 0.38 logMAR, and the mean CMT was 372.09 ± 111.37 micrometers. During the three-month treatment follow-up period, For the treatments administered, the mean diameter of the SML laser beam ranged from 140 to 160 micrometers, with the mean number of laser points varying between 70 and 900. The average follow-up period after SML treatment was 3 months, and the baseline characteristics were balanced between five groups (all p > 0.05) (Supplementary Table 1). The baseline characteristics of patients in the study are shown in Table 1.
The optimal laser power for acute CSC was determined to be 425 mW (mean ± SD: −225.50 ± 109.87 μm), which resulted in the most significant decrease in change of CMT (−225.50 μm vs. −171.24 μm vs. −114.50 μm vs. −130.54 μm vs. −68.00 μm, p < 0.001) (Table 2, Figure 4), but no significant increase in BCVA (−0.15 ± 0.10 logMAR vs. −0.20 ± 0.16 logMAR vs. −0.14 ± 0.11 logMAR vs. −0.17 ± 0.30 logMAR vs. −0.11 ± 0.14 logMAR, p > 0.05). For chronic CSC, the average change in CMT for each group was −93.91 ± 109.06 μm, −119.32 ± 105.56 μm, −88.67 ± 67.26 μm, −60.89 ± 106.86 μm, and −99.11 ± 157.32 μm (p = 0.04), respectively, the results showed that the change of CMT was significant in 375 mW (mean ± SD: −119.32 ± 105.56 μm, Table 3, Figure 4). The change of BCVA was similar trend (−0.54 ± 0.66 logMAR vs. −0.17 ± 0.23 logMAR vs. −0.10 ± 0.21 logMAR vs. −0.02 ± 0.30 logMAR vs. 0.05 ± 0.19 logMAR, p < 0.001) in Table 3.
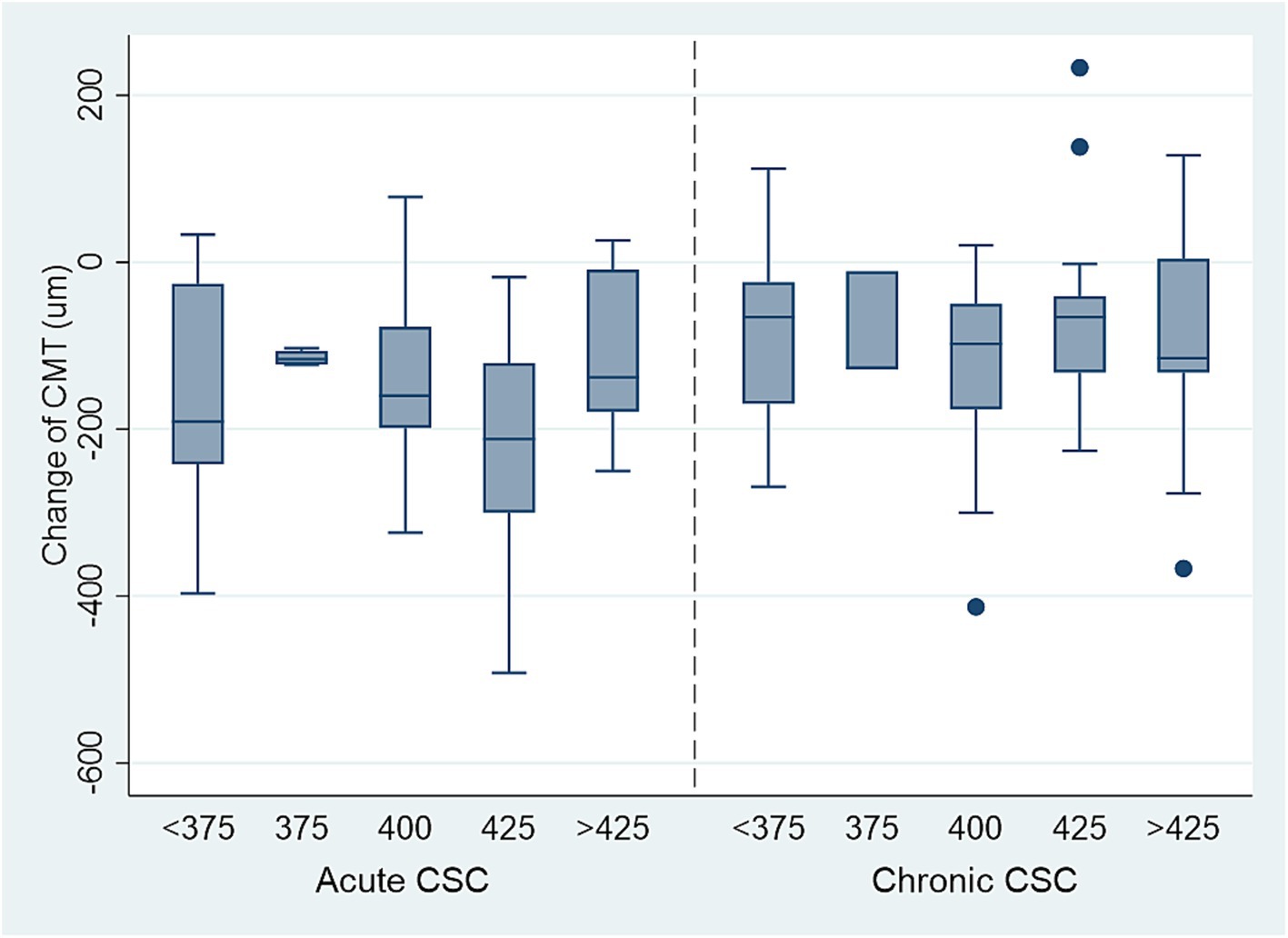
Figure 4. The decrease of CMT in acute and chronic CSC patients with different titration power (mw), 425 mW laser power is the safe, effective lowest power for treating acute CSC. The safe, effective lowest power for chronic CSC is above 425 mW.
The line regression analyses showed the more significant association between baseline age and titration power for chronic CSC patients (coef: 1.14; 95%CI: 0.10–2.18; p = 0.032) in Supplementary Table 2. Furthermore, the results of line regression suggested that the change of BCVA with chronic CSC patients was obviously significant after 375 mW SML treatment (coef: −0.52; 95%CI: −0.89 to −0.15; p = 0.007). However, for acute CSC, the changes in CMT were significantly associated with changes in BCVA (coef: 0.00; 95%CI: 0.00–0.00; p = 0.016) in Table 4.
In Table 5, we found that the change in CMT was significantly associated with CMT at baseline for acute CSC patients (coef: −0.71; 95%CI: −0.88 to −0.53; p = 0.010). In contrast, for chronic CSC, no significant correlation was found between changes in CMT and baseline characteristics (all p > 0.05). The improvement in CMT was most significant in the 375-mw group (coef: −0.52; 95%CI: −0.89 to −0.15; p = 0.007).
Discussion
Given the self-limiting nature of CSC, previous guidelines recommended monitoring patients for at least 3 months before initiating treatment. However, emerging evidence suggests that early intervention may lead to better visual outcomes than waiting for spontaneous resolution (18–20). Prompt treatment has been shown to enhance anatomical recovery and improve long-term visual outcomes (21). Various treatment modalities for CSC have been explored (22, 23), and SML has become an effective choice. Our study aimed to determine the safe lowest effective power of 577-nm yellow SML needed to treat acute and chronic CSC. We found that SML at 425 mW was a safe and effective low-power option for acute CSC, and 375 mW was optimal for chronic CSC. Meanwhile, our results showed a positive correlation between age and the required SML power for chronic CSC. The chronic CSC patients need more higher power as they get older. As far as we know, our study was the first clinical research in China that explored different SML power settings for acute and chronic CSC.
Several previous studies reported that different optimal laser parameters had profound effects on acute and chronic CSC using SML treatment (11, 24, 25). Numerous researches have predominantly examined aspects such as laser threshold percentage, wavelength, and duty cycle. For example, Lijun Zhou investigated different percentage settings for acute central serous chorioretinopathy (26). Alfred K. Yu evaluated the impact of various wavelengths on the retina through histological and differential protein expression studies (27). Jenny Wang assessed the pros and cons of varying temporal modulation strategies (28). Nonetheless, a report suggested that excessive laser power with SML might cause retinal damage and a retinal shock response despite precise titration procedures (29). Therefore, it is necessary to investigate the optimal and safe laser power settings.
The 577 nm wavelength falls within the yellow spectral range, allowing it to penetrate the lens and superficial hemorrhage while avoiding absorption by lutein, thus minimizing phototoxic effects (30). Research indicates that 577 nm laser treatment is associated with a lower risk of Bruch’s membrane rupture (31). A recent retrospective study also demonstrated that 577 nm SML can achieve outcomes comparable to conventional laser treatments without causing RPE damage (32). In our study, we treated CSC using a 577 nm yellow SML. We tested power levels ranging from 160 mW to 475 mW, divided into five groups: <375 mW, 375 mW, 400 mW, 425 mW, and >425 mW, and compared the treatment outcomes. Furthermore, our findings implied that a laser power of 425 mW was more effective than lower power levels in reducing CMT, improving BCVA, and increasing the rate of SRF absorption in cases of acute CSC. Based on these results, we considered that power levels below 425 mW may be insufficient for restoring macular and visual function, in comparison, power levels above 425 mW could lead to more significant retinal damage. Therefore, 425 mW appears to be the optimal laser power for treating acute CSC, identifying the best balance between efficacy and minimizing potential damage.
Although the etiology of CSC remains unknown, it mainly affects middle-aged men and is remarkably associated with corticosteroid use, stress, and differences in ethnic and sociodemographics (33–35). In CSC, the function of RPE is mainly to maintain the outer blood-retina barrier, which was damaged due to congestion, hyper-permeability, and thickening of the choroid. The resulting loss of integrity in this barrier leads to the accumulation of SRF, which affects photoreceptor function and leads to a decrease in vision (3, 36). SML treatment may significantly improve the function of vision, which may be attributed to the fact that SML targets the RPE and further promotes SRF resolution (37). Besides, melanin in the RPE absorbs the photons’ energy, which is dissipated in the form of heat (38). This SML treatment was able to increase the expression of heat shock proteins and restore cellular function in the RPE (12). Therefore, it can be particularly relevant for treating chorioretinal diseases such as CSC. With SML treatment, the aim is to selectively target the RPE without causing visible tissue damage.
Acute CSC is characterized by local SRF accumulation in the macular area, usually without RPE detachment (12). The characteristic of chronic CSC is persistent serous neuroretinal detachment, which can be small or wide in size and multifocal in multiple leakage areas (39). A common imaging feature of atrophic RPE changes on FFA, ranging from a single localized area to extensively DARA (diffuse atrophic RPE alterations). As the disease progresses, the melanin in RPE cells gradually decreases. In this study, chronic CSC patients treated with 375 mW laser energy showed better functional outcomes, including improved BCVA, reduced CMT, and a higher rate of SRF absorption. This suggests that the optimal laser power for chronic CSC is lower than that for acute CSC, possibly due to the disease’s longer duration and the increased choroidal permeability in chronic cases. A key factor is that the SML selectively targets the RPE layer, stimulating RPE cells for repair (40, 41). The therapeutic effect of SML is enhanced when the RPE layer is thicker. However, in chronic CSC, SRF persists for 4 to 6 months, worsening RPE cell damage, leading to RPE decompensation and atrophy, which reduces the thickness of the RPE layer (42). Besides, because of the RPE damage of chronic CSC, the melanin in RPE cells gradually decreased, and fewer normal RPE cells can absorb laser energy. Therefore, chronic CSC requires lower laser energy to reduce the retinal damage caused by SML.
There are several limitations to this study. The primary limitation is the relatively short follow-up period of only three months. Since recurrences are common in CSC, this short duration may limit our ability to assess the long-term efficacy of different laser power settings. Additionally, all patients were recruited from a single institution, which could introduce selection bias. Lastly, the underlying mechanisms of how different laser power affects CSC remain unclear and warrant further investigation.
Conclusion
In conclusion, the current study suggested that 425 mW and 375 mW are the safe lowest effective power to treat acute and chronic CSC, respectively, which may provide the most optimal and safe protocol for achieving anatomical and functional improvements in CSC. It could improve clinicians to make better clinical management for CSC patients. However, these results should be confirmed by large and well-designed studies to illustrate the specific mechanism.
Data availability statement
The raw data supporting the conclusions of this article will be made available by the authors without undue reservation.
Ethics statement
The studies involving humans were approved by The Medical Research Ethics Committee of Shenzhen Eye Hospital. The studies were conducted in accordance with the local legislation and institutional requirements. The participants provided their written informed consent to participate in this study. Written informed consent was obtained from the individual(s), and minor(s)’ legal guardian/next of kin, for the publication of any potentially identifiable images or data included in this article.
Author contributions
TX: Conceptualization, Formal analysis, Investigation, Methodology, Visualization, Writing – original draft. WL: Formal analysis, Investigation, Methodology, Conceptualization, Writing – original draft. LW: Methodology, Visualization, Writing – original draft. JN: Formal analysis, Methodology, Writing – original draft. ZL: Data curation, Investigation, Visualization, Writing – original draft. YC: Data curation, Writing – original draft. XL: Writing – original draft, Methodology. SD: Conceptualization, Methodology, Writing – review & editing. QC: Conceptualization, Writing – original draft, Writing – review & editing.
Funding
The author(s) declare that no financial support was received for the research, authorship, and/or publication of this article.
Conflict of interest
The authors declare that the research was conducted in the absence of any commercial or financial relationships that could be construed as a potential conflict of interest.
Publisher’s note
All claims expressed in this article are solely those of the authors and do not necessarily represent those of their affiliated organizations, or those of the publisher, the editors and the reviewers. Any product that may be evaluated in this article, or claim that may be made by its manufacturer, is not guaranteed or endorsed by the publisher.
Supplementary material
The Supplementary material for this article can be found online at: https://www.frontiersin.org/articles/10.3389/fmed.2024.1494402/full#supplementary-material
References
1. Kaye, R, Chandra, S, Sheth, J, Boon, CJF, Sivaprasad, S, and Lotery, A. Central serous chorioretinopathy: an update on risk factors, pathophysiology and imaging modalities. Prog Retin Eye Res. (2020) 79:100865. doi: 10.1016/j.preteyeres.2020.100865
2. Fung, AT, Yang, Y, and Kam, AW. Central serous chorioretinopathy: a review. Clin Experiment Ophthalmol. (2023) 51:243–70. doi: 10.1111/ceo.14201
3. Daruich, A, Matet, A, Dirani, A, Bousquet, E, Zhao, M, Farman, N, et al. Central serous chorioretinopathy: recent findings and new physiopathology hypothesis. Prog Retin Eye Res. (2015) 48:82–118. doi: 10.1016/j.preteyeres.2015.05.003
4. Nicholson, B, Noble, J, Forooghian, F, and Meyerle, C. Central serous chorioretinopathy: update on pathophysiology and treatment. Surv Ophthalmol. (2013) 58:103–26. doi: 10.1016/j.survophthal.2012.07.004
5. Zhang, X, Lim, CZF, Chhablani, J, and Wong, YM. Central serous chorioretinopathy: updates in the pathogenesis, diagnosis and therapeutic strategies. Eye Vision. (2023) 10:33. doi: 10.1186/s40662-023-00349-y
6. Funatsu, R, Terasaki, H, Sonoda, S, Shiihara, H, Mihara, N, and Sakamoto, T. Characteristics related to visual acuity loss after successful photodynamic therapy for eyes with central serous chorioretinopathy. Am J Ophthalmol. (2023) 256:164–74. doi: 10.1016/j.ajo.2023.05.022
7. Arora, S, Maltsev, DS, Sahoo, NK, Parameshwarappa, DC, Iovino, C, Arora, T, et al. Visual acuity correlates with multimodal imaging-based categories of central serous chorioretinopathy. Eye (Lond). (2022) 36:517–23. doi: 10.1038/s41433-021-01788-4
8. Zhou, L, Chong, V, Lai, K, Huang, C, Xu, F, Gong, Y, et al. A pilot prospective study of 577-nm yellow subthreshold micropulse laser treatment with two different power settings for acute central serous chorioretinopathy. Lasers Med Sci. (2019) 34:1345–51. doi: 10.1007/s10103-019-02721-8
9. van Rijssen, TJ, van Dijk, EHC, Yzer, S, Ohno-Matsui, K, Keunen, JEE, Schlingemann, RO, et al. Central serous chorioretinopathy: towards an evidence-based treatment guideline. Prog Retin Eye Res. (2019) 73:100770. doi: 10.1016/j.preteyeres.2019.07.003
10. Malik, KJ, Sampat, KM, Mansouri, A, Steiner, JN, and Glaser, BM. Low-intensity/high-density subthreshold micropulse diode laser for chronic central serous chorioretinopathy. Retina. (2015) 35:532–6. doi: 10.1097/iae.0000000000000285
11. Maruko, I, Koizumi, H, Hasegawa, T, Arakawa, H, and Iida, T. Subthreshold 577 nm micropulse laser treatment for central serous chorioretinopathy. PLoS One. (2017) 12:e0184112. doi: 10.1371/journal.pone.0184112
12. Sramek, C, Mackanos, M, Spitler, R, Leung, LS, Nomoto, H, Contag, CH, et al. Non-damaging retinal phototherapy: dynamic range of heat shock protein expression. Invest Ophthalmol Vis Sci. (2011) 52:1780–7. doi: 10.1167/iovs.10-5917
13. Romano, MR, Parolini, B, Allegrini, D, Michalewska, Z, Adelman, R, Bonovas, S, et al. An international collaborative evaluation of central serous chorioretinopathy: different therapeutic approaches and review of literature. The European vitreoretinal society central serous chorioretinopathy study. Acta Ophthalmol. (2020) 98:e549–58. doi: 10.1111/aos.14319
14. Lotery, A, Sivaprasad, S, O'Connell, A, Harris, RA, Culliford, L, Ellis, L, et al. Eplerenone for chronic central serous chorioretinopathy in patients with active, previously untreated disease for more than 4 months (vici): a randomised, double-blind, placebo-controlled trial. Lancet. (2020) 395:294–303. doi: 10.1016/s0140-6736(19)32981-2
15. Salehi, M, Wenick, AS, Law, HA, Evans, JR, and Gehlbach, P. Interventions for central serous chorioretinopathy: a network meta-analysis. Cochrane Database Syst Rev. (2015) 2015:Cd011841. doi: 10.1002/14651858.CD011841
16. Pilat, AV, Proudlock, FA, Mohammad, S, and Gottlob, I. Normal macular structure measured with optical coherence tomography across ethnicity. Br J Ophthalmol. (2014) 98:941–5. doi: 10.1136/bjophthalmol-2013-303119
17. van Rijssen, TJ, Mohabati, D, Dijkman, G, Theelen, T, de Jong, EK, van Dijk, EHC, et al. Correlation between redefined optical coherence tomography parameters and best-corrected visual acuity in non-resolving central serous chorioretinopathy treated with half-dose photodynamic therapy. PLoS One. (2018) 13:e0202549. doi: 10.1371/journal.pone.0202549
18. Tekin, K, Sekeroglu, MA, Cankaya, AB, Teke, MY, Doguizi, S, and Yilmazbas, P. Intravitreal bevacizumab and ranibizumab in the treatment of acute central serous chorioretihopathy: a single center retrospective study. Semin Ophthalmol. (2018) 33:1–6. doi: 10.1080/08820538.2016.1228985
19. Kang, HM, Choi, JH, Koh, HJ, and Lee, SC. Long-term treatment response after intravitreal bevacizumab injections for patients with central serous chorioretinopathy. PLoS One. (2020) 15:e0238725. doi: 10.1371/journal.pone.0238725
20. Chan, WM, Lai, TY, Lai, RY, Liu, DT, and Lam, DS. Half-dose verteporfin photodynamic therapy for acute central serous chorioretinopathy: one-year results of a randomized controlled trial. Ophthalmology. (2008) 115:1756–65. doi: 10.1016/j.ophtha.2008.04.014
21. Seraj, H, Alhamoud, M, Khoshhal, MS, Alhashim, H, Alsaif, A, and Abukhashabah, A. The uses of photodynamic therapy versus anti-vascular endothelial growth factor in the management of acute central serous chorioretinopathy: a systematic review and meta-analysis. Cureus. (2023) 15:e46100. doi: 10.7759/cureus.46100
22. Chen, SN, Hwang, JF, Tseng, LF, and Lin, CJ. Subthreshold diode micropulse photocoagulation for the treatment of chronic central serous chorioretinopathy with juxtafoveal leakage. Ophthalmology. (2008) 115:2229–34. doi: 10.1016/j.ophtha.2008.08.026
23. Yeo, JH, Oh, R, Kim, YJ, Kim, JG, Yoon, YH, and Lee, JY. Choroidal neovascularization secondary to central serous chorioretinopathy: oct angiography findings and risk factors. J Ophthalmol. (2020) 2020:7217906. doi: 10.1155/2020/7217906
24. Breukink, MB, Mohabati, D, van Dijk, EH, den Hollander, AI, de Jong, EK, Dijkman, G, et al. Efficacy of photodynamic therapy in steroid-associated chronic central serous chorioretinopathy: a case-control study. Acta Ophthalmol. (2016) 94:565–72. doi: 10.1111/aos.13059
25. Wood, EH, Karth, PA, Sanislo, SR, Moshfeghi, DM, and Palanker, DV. Nondamaging retinal laser therapy for treatment of central serous chorioretinopathy: what is the evidence? Retina. (2017) 37:1021–33. doi: 10.1097/iae.0000000000001386
26. van Dijk, EHC, Fauser, S, Breukink, MB, Blanco-Garavito, R, Groenewoud, JMM, Keunen, JEE, et al. Half-dose photodynamic therapy versus high-density subthreshold micropulse laser treatment in patients with chronic central serous chorioretinopathy: the place trial. Ophthalmology. (2018) 125:1547–55. doi: 10.1016/j.ophtha.2018.04.021
27. Vignesh, TP, Maitray, A, Sen, S, Chakrabarti, A, Kannan, NB, and Ramasamy, K. Subthreshold micro-pulse yellow laser and eplerenone drug therapy in chronic central serous chorio-retinopathy patients: a comparative study. Semin Ophthalmol. (2020) 35:237–45. doi: 10.1080/08820538.2020.1809682
28. Scholz, P, Ersoy, L, Boon, CJ, and Fauser, S. Subthreshold micropulse laser (577 nm) treatment in chronic central serous Chorioretinopathy. Ophthalmol J. (2015) 234:189–94. doi: 10.1159/000439600
29. Sun, Z, Huang, Y, Nie, C, Wang, Z, Pei, J, Lin, B, et al. Efficacy and safety of subthreshold micropulse laser compared with threshold conventional laser in central serous chorioretinopathy. Eye (Lond). (2020) 34:1592–9. doi: 10.1038/s41433-019-0692-8
30. Mrejen, S, Balaratnasingam, C, Kaden, TR, Bottini, A, Dansingani, K, Bhavsar, KV, et al. Long-term visual outcomes and causes of vision loss in chronic central serous chorioretinopathy. Ophthalmology. (2019) 126:576–88. doi: 10.1016/j.ophtha.2018.12.048
31. Zhou, L, Li, T, Lai, K, Huang, C, Xu, F, Zhu, Z, et al. Subretinal fibrin absorption after 577-nm subthreshold micropulse laser therapy in a Csc case: a brief report. Lasers Med Sci. (2018) 33:1175–8. doi: 10.1007/s10103-017-2316-5
32. Yadav, NK, Jayadev, C, Mohan, A, Vijayan, P, Battu, R, Dabir, S, et al. Subthreshold micropulse yellow laser (577 nm) in chronic central serous chorioretinopathy: safety profile and treatment outcome. Eye (Lond). (2015) 29:258–65. doi: 10.1038/eye.2014.315
33. Brinks, J, van Dijk, EHC, Tsonaka, R, Meijer, OC, and Boon, CJF. Sex hormones in males and females with active central serous Chorioretinopathy. Ophthalmol J. (2022) 245:555–62. doi: 10.1159/000526052
34. de Jong, EK, Breukink, MB, Schellevis, RL, Bakker, B, Mohr, JK, Fauser, S, et al. Chronic central serous chorioretinopathy is associated with genetic variants implicated in age-related macular degeneration. Ophthalmology. (2015) 122:562–70. doi: 10.1016/j.ophtha.2014.09.026
35. van Dijk, EHC, Schellevis, RL, van Bergen, MGJM, Breukink, MB, Altay, L, Scholz, P, et al. Association of a haplotype in the Nr3c2 gene, encoding the mineralocorticoid receptor, with chronic central serous chorioretinopathy. JAMA Ophthalmol. (2017) 135:446–51. doi: 10.1001/jamaophthalmol.2017.0245
36. Liu, JH, Peter, DO, Faldalen Guttormsen, MS, Hossain, MK, Gerking, Y, Veruki, ML, et al. The mosaic of Aii Amacrine cell bodies in rat retina is indistinguishable from a random distribution. Vis Neurosci. (2022) 39:E004. doi: 10.1017/S0952523822000025
37. Ozkaya, A, Alkin, Z, Ozveren, M, Yazici, AT, and Taskapili, M. The time of resolution and the rate of recurrence in acute central serous chorioretinopathy following spontaneous resolution and low-fluence photodynamic therapy: a case-control study. Eye (Lond). (2016) 30:1005–10. doi: 10.1038/eye.2016.79
38. Sivaprasad, S, Elagouz, M, McHugh, D, Shona, O, and Dorin, G. Micropulsed diode laser therapy: evolution and clinical applications. Surv Ophthalmol. (2010) 55:516–30. doi: 10.1016/j.survophthal.2010.02.005
39. Mohabati, D, van Rijssen, TJ, van Dijk, EH, Luyten, GP, Missotten, TO, Hoyng, CB, et al. Clinical characteristics and long-term visual outcome of severe phenotypes of chronic central serous chorioretinopathy. Clin Ophthalmol. (2018) 12:1061–70. doi: 10.2147/OPTH.S160956
40. Weenink, AC, Borsje, RA, and Oosterhuis, JA. Familial chronic central serous chorioretinopathy. Ophthalmol J. (2001) 215:183–7. doi: 10.1159/000050855
41. Tsay, K, Safari, S, Abu-Samra, A, Kremers, J, and Tzekov, R. Pre-stimulus bioelectrical activity in light-adapted erg under blue versus white background. Vis Neurosci. (2023) 40:E004. doi: 10.1017/S0952523823000032
Keywords: central serous chorioretinopathy, subthreshold micropulse laser, central macular thickness, laser power, treatment
Citation: Xie T, Li W, Wang L, Ning J, Li Z, Chen Y, Lin X, Du S and Chen Q (2024) The safe lowest effective power of subthreshold micropulse laser treatment in Chinese patients with acute or chronic central serous chorioretinopathy. Front. Med. 11:1494402. doi: 10.3389/fmed.2024.1494402
Edited by:
Qi Dai, Wenzhou Medical University, ChinaReviewed by:
Honghua Yu, Guangdong Provincial People’s Hospital, ChinaMinwen Zhou, Shanghai General Hospital, China
Copyright © 2024 Xie, Li, Wang, Ning, Li, Chen, Lin, Du and Chen. This is an open-access article distributed under the terms of the Creative Commons Attribution License (CC BY). The use, distribution or reproduction in other forums is permitted, provided the original author(s) and the copyright owner(s) are credited and that the original publication in this journal is cited, in accordance with accepted academic practice. No use, distribution or reproduction is permitted which does not comply with these terms.
*Correspondence: Qingshan Chen, Q3FzMTk2N0BzaW5hLmNvbQ==; Shaolin Du, ZHUuc2hhb2xpbkBob3RtYWlsLmNvbQ==
†These authors have contributed equally to this work