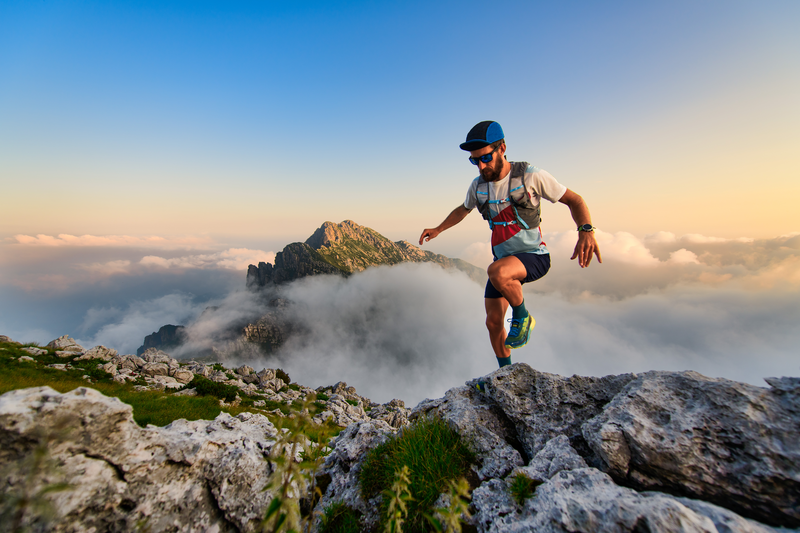
94% of researchers rate our articles as excellent or good
Learn more about the work of our research integrity team to safeguard the quality of each article we publish.
Find out more
BRIEF RESEARCH REPORT article
Front. Med. , 09 December 2024
Sec. Translational Medicine
Volume 11 - 2024 | https://doi.org/10.3389/fmed.2024.1476300
Rapid and sensitive diagnostic measures are a pre-requisite for the control of SARS-CoV-2 outbreaks. Dogs detect SARS-CoV-2-infected human individuals with high speed due to their extraordinary olfactory acuity. In the post-pandemic phase of SARS-CoV-2 it is difficult to obtain samples from infected humans for scent dog training. Established animal models for COVID-19 include hamsters and ferrets, which could overcome this shortcoming and have the advantage that samples are generated under controlled conditions. Respiratory samples from humans, hamsters and ferrets infected with SARS-CoV-2 and from ferrets infected with an H7-Influenza A virus were inactivated with β-propiolactone and presented via a device called “Detection Dog Training System” (DDTS). DDTS allows a fast, blinded, randomized, and automated sample presentation without trainer interference. Scent dogs generally showed a similar diagnostic sensitivity (DSe) and specificity (Dsp) for four tested scenarios (S1-4) and as reported previously for respiratory samples from humans. (S1) Human with COVID-19: DSe 88.1 [74.0–100.0% CI95%] and DSp 89.6 [80.6–98.5% CI95%]. (S2) Hamster with COVID-19: DSe 82.4 [74.1–90.7% CI95%] and DSp 96.7 [93.7–99.7% CI95%]. (S3) Ferret with COVID-19: DSe 86.2 [69.8–100.0% CI95%] and DSp 95.1 [89.5–100.0% CI95%]. (S4) Ferrets infected with an H7 Influenza A-virus (IAV) as a distractor: DSe 96.9 [57.2–100.0% CI95%] and DSp 89.86 [40.3–100.0% CI95%]. We provide evidence that scent dogs detect samples from SARS-CoV-2-infected hamsters and ferrets with a similar accuracy as reported for humans. The study highlights that volatile organic compound odor patterns are similar in humans, hamsters, and ferrets after SARS-CoV-2 infection, but distinct after IAV-infection.
Severe acute respiratory syndrome coronavirus 2 (SARS-CoV-2) emerged in 2019 and caused severe illness and substantial mortality in humans. Early detection of SARS-CoV-2-infected individuals is the most crucial control measure for interrupting the chain of infection. Several studies have already demonstrated the extraordinary olfactory acuity and speed of dogs in detecting SARS-CoV-2-infected individuals through disease-specific odor patterns derived from volatile organic compounds (VOCs) in different body fluids from humans. The diagnostic accuracies of scent dogs are comparable with SARS-CoV-2-specific real-time quantitative reverse transcription-PCR (RT-qPCR) (1–4).
A crucial step during training is to achieve the right balance between olfactory generalization and discrimination of odor patterns. Therefore, a diverse range of samples from varying symptomatic and asymptomatic COVID-19 patients at different stages of the disease is essential. Furthermore, in the post-pandemic, i.e., endemic, phase of SARS-CoV-2, it is difficult to obtain samples from infected humans. Established animal models of pathophysiological states for COVID-19 include hamsters and ferrets (5), which could overcome this shortcoming. Furthermore, if canines are able to scent SARS-CoV-2 infections in hamsters and ferrets, this would strengthen the translational character of these animal models.
Sputum, sweat and urine samples from humans previously diagnosed with COVID-19 based on SARS-CoV-2-RNA detection or from healthy volunteers were collected during the COVID-19 pandemic from 2020 to 2021 (Table 1). Hamster and ferret samples were re-used from animal experiments that were conducted for other research purposes. The animal husbandry, health monitoring, study design, experimental procedures (including for example anesthesia regimens, experimental infection, sample collection, clinical monitoring, human endpoint criteria and euthanasia) were approved by an independent ethical committee (see also section Ethical statement) and conducted as described previously (5–8). Hamsters were inoculated intranasally with phosphate buffered saline (PBS) or infected with 10^4 TCID50/ml SARS-CoV-2 (7) and sacrificed at 6 days post infection (dpi). Ferrets were infected intratracheally with 1 mL of 10^6 TCID50/ml SARS-CoV-2 (5) or with 1.5 mL of 10^5 or 10^6 plaque forming units (PFU) of an H7-Influenza A virus (IAV) or with PBS (8). Oropharyngeal swabs and nasal swabs were collected before and at 4, 7, 14 or 21 dpi after infection, while bronchioalveolar lavage fluid (BALF) samples were collected immediately after euthanasia. Specific-pathogen free ferrets served as negative control animals (5–8). An overview of the samples is provided in Table 1.
SARS-CoV-2-RNA in human and animal samples (1, 5) and H7-IAV-RNA (8, 9) in ferret samples were analyzed by real-time quantitative reverse transcription-PCR (RT-qPCR) with an internal control system (pcDNA3-EGFP, Plasmid #13031, Addgene) as described previously (1, 5, 8, 9). Briefly, 100 μL was extracted at a KingFisher 96 (Thermo Fisher Scientific) using the NucleoMagVet kit (Macherey-Nagel). A total of 2.5 μL eluate was used for amplification of SARS-CoV-2-RNA or H7-IAV-RNA using the AgPath-ID™ One-Step RT-PCR Reagent kit (Thermo Fisher Scientific, 4,387,424) at a CFX96 real-time PCR detection system (Bio-Rad) or a AriaMx Real-Time PCR System (Agilent), respectively. The RT-qPCR temperature protocols included reverse transcription for 10 min at 45°C, activation Taq for 10 min at 95°C, and 42 cycles of denaturation for 15 s at 95°C, annealing for 20 s at 57°C (SARS-CoV-2) or 56°C (H7-IAV) and elongation for 30 s at 72°C. Positive and negative controls were included in each RT-qPCR run.
The forward and reverse primers and the probes used for the H7-IAV RT-qPCR and SARS-CoV-2 assays including the EGFP assay as internal control system were as followed (sequences given 5′-3′): AYA GAA TAC AGA TWG ACC CAG T (IAV-HA7-1593-F), TAG TGC ACY GCA TGT TTC CA (IAV-HA7-1740-R), FAM-TGG TTT AGC TTC GGG GCA TCA TG-BHQ1 (AIV-HA7-1649-FAM) (8, 9); GGT AAC TGG TAT GAT TTC G (SARS2-IP4-14059F), CTG GTC AAG GTT AAT ATA GG (SARS2-IP4-14146R), FAM-TCA TAC AAA CCA CGC CAG G-BHQ1 (SARS2-IP4-14084FAM); GAC CAC TAC CAG CAG AAC AC (EGFP-1-F), GAA CTC CAG CAG GAC CAT G (EGFP2-F), HEX-AGC ACC CAG TCC GCC CTG AGC A-BHQ1 (EGFP-HEX) (1, 5).
All samples were chemically inactivated with beta-propiolactone (BPL) according to Pilchová et al. (10) as shown in Figure 1B.
Figure 1. Flow chart of sample analyses. (A) Real-time reverse transcription-PCR analysis of samples to detect SARS-CoV-2-RNA and Influenza A virus (IAV)-RNA. (B) Five steps required for inactivation of SARS-CoV-2 and IAV with beta-propiolactone (BPL): samples were (i) buffered with 10% NaHCO3 and incubated for 10 min at 4°C, (ii) inactivated with BPL for 71 to 72 h at 4°C, (iii) BPL was hydrolyzed for 1 to 2 h at 37°C and (iv) stored at−80°C until presentation to the dogs for training and study purposes. (C) Sample preparation for a device called “Detection Dog Training System” (DDTS). (D) Sample presentation to dogs via DDTS. Icons in pannels (A,B) were created with Biorender.com (Agreement number FD26B6H2CJ).
Three dogs that were previously trained solely with samples from human COVID-19 patients (scenario 1, Table 1) were confronted for the first time with respiratory samples collected from hamsters (scenario 2) and ferrets (scenario 3 and 4) at different days before and after SARS-CoV-2 infection. In scenario 4, ferrets infected with an IAV (8) were included as distractor samples (Table 1). In scenario 4, only two of three dogs were available. Samples collected after infection were considered as “positive” irrespective of the PCR-result (Tables 1, 2; Figure 2).
Table 2. Diagnostic sensitivity (DSe) and specificity (DSp) for the detection of SARS-CoV-2 infection in human and animal samples by scent dogs.
Figure 2. Quantitative cycle values (Cq) of SARS-CoV-2-RNA detected by real-time reverse transcription-PCR in samples from humans, hamsters and ferrets by scenario (S) S1 to S4, species and sample matrix. (A) SARS-CoV-2-RNA in sputum, sweat and urine from human COVID-19 patients that were tested positive for SARS-CoV-2 at an earlier time point by a clinic or diagnostic laboratory (S1). (B) SARS-CoV-2-RNA in samples collected from hamsters before infection and at 6 days post infection (dpi) (S2). (C) SARS-CoV-2-RNA in samples collected from ferrets before infection and at 4 to 21 dpi (S3). (D) SARS-CoV-2-RNA in samples from ferrets as described for S3, except that in S4 dogs were additionally presented samples from ferrets infected with H7 influenza A virus as distractor. BALF, bronchioalveolar lavage fluid; LOD, limit of detection. Whiskers show median with 95% confidence intervals.
All samples were presented via a device called “Detection Dog Training System” (DDTS) (1) (Figure 1). DDTS allows a fast, blinded, randomized and automated sample presentation without trainer interference (1).
Statistical analysis was calculated with GraphPad prism (version 9), and the accuracy with Medcalc statistical software.1
Human participation was approved by ethics committee of Hannover Medical School with consent number 9042_BO_K_2020 and 9940_BO_S_2021. Animal experiments were approved by an independent ethical committee (Niedersächsisches Landesamt für Verbraucherschutz und Lebensmittelsicherheit, LAVES) and registered with the permission numbers 20-3482 (hamster), 2-3402 (SARS-CoV-2, ferrets), 20-3499 (IAV, ferrets).
SARS-CoV-2-RNA in human samples (scenario 1) was detected in one (quantitative cycle values (Cq) 31.7) of seven tested samples from COVID-19 patients that were tested positive for SARS-CoV-2 at an earlier time point by a clinic or diagnostic laboratory. S2: All samples collected from hamsters (scenario 2) at 6 dpi or ferrets (scenarios 3 and 4) at 4 to 7 dpi were positive for SARS-CoV-2-RNA (Cq 21.1 to 33.0), while no SARS-CoV-2-RNA was detected in ferret samples collected at 14 dpi, 21 dpi or before infection (Figure 2). IAV was detected in one nasal swab from one ferret collected at 4 dpi (Cq 28.6), while the nasal swabs collected at 7, 14 and 21 dpi were RT-qPCR-negative.
Scent dogs generally showed a similar diagnostic sensitivity (DSe) and specificity (Dsp) for four tested scenarios (S1-4) (Table 2). (S1) Human with COVID-19: DSe 88.1 [74.0–100.0% CI95%] and DSp 89.6 [80.6–98.5% CI95%]. (S2) Hamster with COVID-19: DSe 82.4 [74.1–90.7% CI95%] and DSp 96.7 [93.7–99.7% CI95%]. (S3) Ferret with COVID-19: DSe 86.2 [69.8–100.0% CI95%] and DSp 95.1 [89.5–100.0% CI95%]. (S4) Ferrets infected with an H7 Influenza A-virus (IAV) as a distractor: DSe 96.9 [57.2–100.0% CI95%] and DSp 89.86 [40.3–100.0% CI95%].
Accordingly, scent dogs generally showed a similar accuracy (89–94%) (see details in Supplementary Table S1), DSe (82–97%) and DSp (90–97%) for all four scenarios, but the DSe in scenario 4 was noticeable higher (97%) compared to scenarios 1–3 (82–89%) (see details in Table 2; Supplementary Figure S1). In scenario 4, the DSe and DSp generally showed wider 95% confidence intervals compared to scenarios 1–3 (Table 2; Supplementary Figure S1). The negative predictive values (NPV) were similar for all four scenarios (95–99%), while the PPV varied between scenarios 1 and 4 (65 and 68%) in comparison to scenarios 2 and 3 (88 and 83%) (see details in Supplementary Table S2). Fisher exact tests were significant (p < 0 0.0001) for all trials, by dog and scenario (Supplementary Table S1).
Thanks to the excellent olfactory acuity of canines, scent dogs detect various odors of explosives and drugs, odors produced during disease manifestation including metabolic disorders such as different types of cancer, hyperglycemia, and VOCs released after infection with different pathogens (11, 12). During the COVID-19 pandemic, scent dogs demonstrated a high sensitivity and specificity to rapidly diagnose COVID-19 in humans (1, 13). We present here the first study to demonstrate that scent dogs detect VOC release after SARS-CoV-2 infection in samples other than humans, from animal species, such as ferrets and hamsters, with previous training solely on human samples. The accuracy, DSe and DSp of scent dogs to positively decide for different respiratory sample matrices from SARS-CoV-2 infected hamsters and ferrets after training with human samples was similar to the accuracy, DSe and DSp in human samples in our study (Table 2; Supplementary Table S1) and as reported for respiratory samples from humans before (1–3, 13). The results highlight that COVID-19 detection dogs differentiate between samples from infected and non-infected ferrets and hamsters without specific prior training with animal samples and suggests that the VOCs in human samples and laboratory animals are very similar independent from the host species. These findings strengthen the use of ferrets and hamsters as animal models for human SARS-CoV-2 infections and as a source for future canine training materials. Furthermore, comparison of VOCs in headspace air samples from humans (14, 15) and animals infected with SARS-CoV-2 and other respiratory virus using solid-phase microextraction (SPME) and gas chromatography/mass spectrometry (GC/MS) methods might illuminate species-unspecific VOCs characteristic for COVID-19. Interestingly, VOCs from animals and humans generally differ as reported for muscle tissue (16) or decomposed animals (17) and humans using SPME and GC/MS or “Human Remains Detection” dogs (16, 17).
The successful training to detect VOC-specific odor patterns may be influenced by environmental factors (11). Training with samples from experimentally infected laboratory animals could overcome challenges associated with obtaining human samples and enhance the efficiency of training detection dogs, not only for COVID-19 but also for future pandemics. Additionally, this offers novel opportunities to train dogs with a large number and variety of high-quality samples with target odors that were generated under controlled conditions. For ethical reasons and in favor of the 3R-principle to reduce, refine and replace animal experiments, no animal experiments are required to generate samples for the purpose of scent dog training (18). Collaborative networks and biobanks facilitate rapid access not only to human patient samples but also to animal samples from experiments conducted under controlled conditions, which provide comprehensive data including pathogens and disease progression (19, 20).
Experimentally infected animals generally show an infection rate of IAV and SARS-CoV-2 of about 100%. Viral shedding ceases in respiratory samples after the peak of IAV and SARS-CoV-2 infection during the first to second week after infection due to virus elimination by the cellular and humoral immune responses elicited by the innate and adaptive immune systems (6–8, 21–23). Therefore, IAV-RNA and SARS-CoV-2-RNA was detected in respiratory samples until 4 or 7 dpi (Figure 2), respectively.
The results of our pilot study (scenario 2–4), indicate that dogs show a lower percentage of false negative decisions in samples collected during the first week (hamster: 3.4%; ferret: 5.0%) compared to 14 or 21 dpi (ferret: 20–21%) after SARS-CoV-2 infection. In contrast, the percentage of false positive decisions was generally higher after infection and varied between 12 and 33% (Supplementary Table S3). On the other hand, the detection of SARS-CoV-2-RNA-negative samples as positive after the peak of infection (14 and 21 dpi) indicate that “post-COVID-19” infection can also be detected by scent dogs in ferrets as previously reported for post-COVID-19 patients (3, 24).
In scenario 4, the DSe and DSp showed wider 95% confidence intervals compared to scenarios 1–3 (Table 2; Supplementary Figure S1). This can be explained by the lower number of sample presentations compared to scenarios 1–3, since only two of three dogs could be used for the trial, due to other deployments of the dogs and their handlers at the time of our study. Both dogs were able to distinguish between IAV and SARS-CoV-2 positive samples, although the proportion of false positive decisions varied between the two dogs (13% versus 2%), indicating that the training results depend on the individual dog (Supplementary Table S4). Furthermore, training with distractory samples including other respiratory pathogens to distinguish from SARS-CoV-2 positive samples is essential to optimize the DSp as suggested previously (13).
The advantage to use scent dogs in disease diagnosis generally include fast detection, high sensitivity, short training period and application for large-scale screening events at low costs. Hence, scent dog detection may be applied to individual diagnosis, but also at large-scale, for example in a pandemic situation. The disadvantages to use scent dogs is that the training and performance characteristics of scent dogs rely on various factors. The training success relies on the handler and training efficiency, which often lacks a certified standardized and validated process. Furthermore, intrinsic (e.g., anatomy, health, behavior, age) and extrinsic factors (experience, operational environment) may influence the dogs performance. The selection of the right target samples and sample diversity is essential for the training of dogs to discriminate between the target and background scent required for olfactory generalization. Last but not least, ethical considerations are paramount to ensure that the used training methods and materials are in accordance with animal welfare, the current state of research and do not harm the dogs health integrity (11, 12, 25, 26).
In the present study, we applied previously approved and standardized training methods that resulted in a high DSe and DSp to detect VOCs specific for SARS-CoV-2 infection in different matrices of samples from human COVID-19 patients. The study results confirmed that the training methods can also be successfully applied on the detection of VOCs specific for SARS-CoV-2 infection in animal samples from ferrets and hamsters. Furthermore, the study protocols were approved by an independent ethical committee in accordance with animal welfare and the samples were inactivated with BPL to preclude infection of dogs with SARS-CoV-2.
The study highlights that the VOC odor pattern released from established animal models for SARS-CoV-2 is similar to human VOCs (1–3, 13), independent from the sample matrix and distinct from VOCs released after IAV-infection. In favor of the principle of sustainability, our protocol can be adapted to future pandemics with other pathogens.
The original contributions presented in the study are included in the article/Supplementary material, further inquiries can be directed to the corresponding author.
The studies involving humans were approved by Ethics committee of Hannover Medical School. The studies were conducted in accordance with the local legislation and institutional requirements. The human samples used in this study were acquired and primarily isolated as part of our previous study for which ethical approval was obtained. Written informed consent for participation was not required from the participants or the participants’ legal guardians/next of kin in accordance with the national legislation and institutional requirements. The animal study was approved by Niedersächsisches Landesamt für Verbraucherschutz und Lebensmittelsicherheit. The study was conducted in accordance with the local legislation and institutional requirements.
CS: Conceptualization, Data curation, Formal analysis, Funding acquisition, Investigation, Methodology, Project administration, Resources, Software, Supervision, Validation, Visualization, Writing – original draft, Writing – review & editing. FT: Conceptualization, Data curation, Formal analysis, Funding acquisition, Investigation, Methodology, Project administration, Resources, Software, Supervision, Validation, Visualization, Writing – original draft, Writing – review & editing. SM: Data curation, Formal analysis, Investigation, Methodology, Software, Writing – review & editing. NH: Methodology, Writing – review & editing, Investigation. VP: Investigation, Methodology, Writing – review & editing. KW: Investigation, Methodology, Writing – review & editing. SC: Investigation, Methodology, Writing – review & editing. CM: Investigation, Methodology, Writing – review & editing. AV: Investigation, Methodology, Writing – review & editing. MK-B: Investigation, Methodology, Writing – review & editing. HV: Conceptualization, Funding acquisition, Investigation, Methodology, Project administration, Resources, Supervision, Validation, Writing – review & editing.
The author(s) declare that financial support was received for the research, authorship, and/or publication of this article. The study was supported by the COVID-19-Research Network of the State of Lower Saxony (COFONI) with funding from the Ministry of Science and Culture of Lower Saxony, Germany (14-76403-184). The funder had no role in study design, data collection and analysis, decision to publish, or preparation of the manuscript. We acknowledge financial support by the Open Access Publication Fund of the University of Veterinary Medicine Hannover, Foundation.
We thank our dog handlers Lisa Strahl and Jessica Wollrab for their assistance in dog training and conducting the study. We would like to acknowledge the excellent technical laboratory assistance by Rouwen Stucke, Karola Schlote, Katharina Meyer and Victoria Lechler and the animal keepers of the RIZ for their dedicated animal care.
The authors declare that the research was conducted in the absence of any commercial or financial relationships that could be construed as a potential conflict of interest.
The author(s) declared that they were an editorial board member of Frontiers, at the time of submission. This had no impact on the peer review process and the final decision.
All claims expressed in this article are solely those of the authors and do not necessarily represent those of their affiliated organizations, or those of the publisher, the editors and the reviewers. Any product that may be evaluated in this article, or claim that may be made by its manufacturer, is not guaranteed or endorsed by the publisher.
The Supplementary material for this article can be found online at: https://www.frontiersin.org/articles/10.3389/fmed.2024.1476300/full#supplementary-material
1. Jendrny, P, Schulz, C, Twele, F, Meller, S, Von Köckritz-Blickwede, M, Osterhaus, ADME, et al. Scent dog identification of samples from COVID-19 patients - A pilot study. BMC Infect Dis. (2020) 20:536. doi: 10.1186/s12879-020-05281-3
2. Jendrny, P, Twele, F, Meller, S, Schulz, C, von Köckritz-Blickwede, M, Osterhaus, ADME, et al. Scent dog identification of SARS-CoV-2 infections in different body fluids. BMC Infect Dis. (2021) 21:707. doi: 10.1186/s12879-021-06411-1
3. Twele, F, ten Hagen, NA, Meller, S, Schulz, C, Osterhaus, A, Jendrny, P, et al. Detection of post-COVID-19 patients using medical scent detection dogs—a pilot study. Front Med. (2022) 9:1824. doi: 10.3389/fmed.2022.877259
4. Meller, S, Caraguel, C, Twele, F, Charalambous, M, Schoneberg, C, Chaber, AL, et al. Canine olfactory detection of SARS-CoV-2-infected humans—a systematic review. Ann Epidemiol. (2023) 85:68–85. doi: 10.1016/j.annepidem.2023.05.002
5. Ciurkiewicz, M, Armando, F, Schreiner, T, de Buhr, N, Pilchová, V, Krupp-Buzimikic, V, et al. Ferrets are valuable models for SARS-CoV-2 research. Vet Pathol. (2022) 59:661–72. doi: 10.1177/03009858211071012
6. Pilchová, V, Gerhauser, I, Armando, F, Wirz, K, Schreiner, T, de Buhr, N, et al. Characterization of young and aged ferrets as animal models for SARS-CoV-2 infection with focus on neutrophil extracellular traps. Front Immunol. (2023) 14:14. doi: 10.3389/fimmu.2023.1283595
7. Wirz, K, Schulz, C, Sobbeler, F, Armando, F, Beythien, G, Gerhauser, I, et al. A new methodology for the oxygen measurement in lung tissue of an aged ferret model proves hypoxia during COVID-19. Am J Respir Cell Mol Biol. (2024) 71:146–53. doi: 10.1165/rcmb.2024-0005MA
8. Schulz, C, Wirz, K, Pilchová, V, Soebbeler, F, Beythien, G, Armando, F, et al., Neutrophil extracellular traps-related host factors associated with acute respiratory distress syndrome (ARDS)-like disease in a ferret model of H7N7-influenza virus infection. In: G Mazzuoli-Weber and M Köckritz-Blickwedevon, editors. Tagung der DVG-Fachgruppe Physiologie & Biochemie ; (2024) Hannover, Germany. Gießen: Verlag der DVG Service GmbH.
9. Kalthoff, D, Bogs, J, Harder, T, Grund, C, Pohlmann, A, Beer, M, et al. Nucleic acid-based detection of influenza A virus subtypes H7 and N9 with a special emphasis on the avian H7N9 virus. Eur Secur. (2014) 19:20731. doi: 10.2807/1560-7917.ES2014.19.10.20731
10. Pilchová, V, Kandiyil Prajeeth, C, Jendrny, P, Twele, F, Meller, S, Pink, I, et al. β-Propiolactone (BPL)-inactivation of SARS-co-V-2: in vitro validation with focus on saliva from COVID-19 patients for scent dog training. J Virol Methods. (2023) 317:114733. doi: 10.1016/j.jviromet.2023.114733
11. Meller, S, Al Khatri, MSA, Alhammadi, HK, Álvarez, G, Alvergnat, G, Alves, LC, et al. Expert considerations and consensus for using dogs to detect human SARS-CoV-2-infections. Front Med. (2022) 9:3590. doi: 10.3389/FMED.2022.1015620/BIBTEX
12. Edwards, TL, Browne, CM, Schoon, A, Cox, C, and Poling, A. Animal olfactory detection of human diseases: guidelines and systematic review. J Vet Behav. (2017) 20:59–73. doi: 10.1016/J.JVEB.2017.05.002
13. ten Hagen, NA, Twele, F, Meller, S, Jendrny, P, Schulz, C, von Köckritz-Blickwede, M, et al. Discrimination of SARS-CoV-2 infections from other viral respiratory infections by scent detection dogs. Front Med. (2021) 8:2245. doi: 10.3389/fmed.2021.749588
14. Feuerherd, M, Sippel, AK, Erber, J, Baumbach, JI, Schmid, RM, Protzer, U, et al. A proof of concept study for the differentiation of SARS-CoV-2, hCoV-NL63, and IAV-H1N1 in vitro cultures using ion mobility spectrometry. Sci Rep. (2021) 11:20143. doi: 10.1038/S41598-021-99742-7
15. Crespo-Cajigas, J, Gokool, VA, Ramírez Torres, A, Forsythe, L, Abella, BS, Holness, HK, et al. Investigating the use of SARS-CoV-2 (COVID-19) odor expression as a non-invasive diagnostic tool—pilot study. Diagnostics. (2023) 13:13. doi: 10.3390/DIAGNOSTICS13040707
16. Li, X-b, Guo, C-h, Qi, Y-h, Lu, W-h, Xu, G-t, Wang, B-y, et al. Identification of volatile organic compounds in muscle tissues of different species based on headspace-gas-chromatography ion-mobility spectrometry. Legal Med. (2022) 59:102132. doi: 10.1016/J.LEGALMED.2022.102132
17. Cablk, ME, Szelagowski, EE, and Sagebiel, JC. Characterization of the volatile organic compounds present in the headspace of decomposing animal remains, and compared with human remains. Forensic Sci Int. (2012) 220:118–25. doi: 10.1016/J.FORSCIINT.2012.02.007
18. Richter, A, Wirz, K, Pilchová, V, Schulz, C, Volz, A, and von Köckritz-Blickwede, M. Quality management and animal welfare as culture of Care for Animal Experiments under biosafety Level-3, taking COVID-19 as a practical example. J App Animal Ethics Res. (2024) 1:1–27. doi: 10.1163/25889567-BJA10047
19. COFONI (2024). Central COFONI technology platform | Universitätsmedizin Göttingen. Available at: https://www.umg.eu/en/cofoni/technology-platform. [Accessed August 04, 2024]
20. HUB (2024). Medizinische Hochschule Hannover: Hannover Unified Biobank HUB. Available at: https://www.mhh.de/institute-zentren-forschungseinrichtungen/hannover-unified-biobank-hub. [Accessed August 04, 2024]
21. Abouelkhair, MA, and Kennedy, M. Basic virology In: DS McVey, M Kennedy, MM Chengappa, and RP Wilkes, editors. Veterinary Microbiology (2022). 35–40. doi: 10.1002/9781119650836.ch4
22. Schlottau, K, Rissmann, M, Graaf, A, Schön, J, Sehl, J, Wylezich, C, et al. SARS-CoV-2 in fruit bats, ferrets, pigs, and chickens: an experimental transmission study. Lancet Microbe. (2020) 1:e218–25. doi: 10.1016/S2666-5247(20)30089-6
23. Halwe, NJ, Hamberger, L, Sehl-Ewert, J, Mache, C, Schön, J, Ulrich, L, et al. Bat-borne H9N2 influenza virus evades MxA restriction and exhibits efficient replication and transmission in ferrets. Nat Commun. (2024) 15:3450–8. doi: 10.1038/s41467-024-47455-6
24. Grandjean, D, Salmon, D, Slama, D, Gallet, C, Julien, C, Seyrat, E, et al. Screening for SARS-CoV-2 persistence in long COVID patients using sniffer dogs and scents from axillary sweats samples. J Clin Trials. (2022) 12:1–5. doi: 10.35248/2167-0870.22.S16.002
25. Hall, NJ, Johnston, AM, Bray, EE, Otto, CM, MacLean, EL, and Udell, MAR. Working dog training for the twenty-first century. Front Vet Sci. (2021) 8:646022. doi: 10.3389/fvets.2021.646022
Keywords: scent dog, COVID-19, SARS-CoV-2, diagnosis, animal experiment, ferret, hamster
Citation: Schulz C, Twele F, Meller S, ten Hagen NA, Pilchová V, Wirz K, Clever S, Meyer zu Natrup C, Volz A, von Köckritz-Blickwede M and Volk HA (2024) Scent dogs identify SARS-CoV-2-infections in respiratory samples from experimentally infected ferrets and hamsters—a pilot study. Front. Med. 11:1476300. doi: 10.3389/fmed.2024.1476300
Received: 05 August 2024; Accepted: 19 November 2024;
Published: 09 December 2024.
Edited by:
Levon Abrahamyan, Montreal University, CanadaReviewed by:
Michał Dzięcioł, Wroclaw University of Environmental and Life Sciences, PolandCopyright © 2024 Schulz, Twele, Meller, ten Hagen, Pilchová, Wirz, Clever, Meyer zu Natrup, Volz, von Köckritz-Blickwede and Volk. This is an open-access article distributed under the terms of the Creative Commons Attribution License (CC BY). The use, distribution or reproduction in other forums is permitted, provided the original author(s) and the copyright owner(s) are credited and that the original publication in this journal is cited, in accordance with accepted academic practice. No use, distribution or reproduction is permitted which does not comply with these terms.
*Correspondence: Holger A. Volk, aG9sZ2VyLnZvbGtAdGloby1oYW5ub3Zlci5kZQ==
†Present addresses: Claudia Schulz, Infectiology and Virology, Center of Pathobiology, Department of Biological Sciences and Pathobiology, University of Veterinary Medicine Vienna, Vienna, Austria
Veronika Pilchová, Division of Virology, Department of Veterinary Sciences, Ludwig Maximilian University of Munich, Munich, Germany
‡These authors have contributed equally to this work
Disclaimer: All claims expressed in this article are solely those of the authors and do not necessarily represent those of their affiliated organizations, or those of the publisher, the editors and the reviewers. Any product that may be evaluated in this article or claim that may be made by its manufacturer is not guaranteed or endorsed by the publisher.
Research integrity at Frontiers
Learn more about the work of our research integrity team to safeguard the quality of each article we publish.