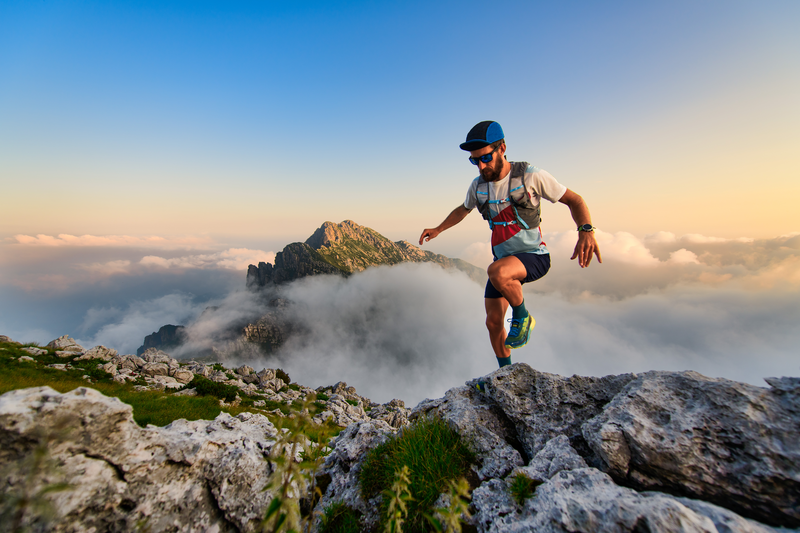
94% of researchers rate our articles as excellent or good
Learn more about the work of our research integrity team to safeguard the quality of each article we publish.
Find out more
MINI REVIEW article
Front. Med. , 20 November 2024
Sec. Infectious Diseases: Pathogenesis and Therapy
Volume 11 - 2024 | https://doi.org/10.3389/fmed.2024.1460676
Rhabdomyolysis (RML), characterized by the breakdown of skeletal muscle fibers and the release of muscle contents into the bloodstream, has emerged as a notable complication associated with Coronavirus disease 2019 (COVID-19) infection and vaccination. Studies have reported an increased incidence of RML in individuals with severe COVID-19 infection. However, the exact mechanisms remain unclear and are believed to involve the host’s immune response to the virus. Furthermore, RML has been documented as a rare adverse event following COVID-19 vaccination, particularly with mRNA vaccines. Proposed mechanisms include immune responses triggered by the vaccine and T-cell activation against viral spike proteins. This study aims to review the current literature on the incidence, pathophysiology, clinical presentation, and outcomes of RML secondary to COVID-19 infection and vaccination. We identify common risk factors and mechanisms underlying this condition by analyzing case reports, clinical studies, and pharmacovigilance data. Our findings suggest that while RML is a relatively rare adverse event, it warrants attention due to its potential severity and the widespread prevalence of COVID-19 and its vaccines. This review underscores the need for heightened clinical awareness and further research to optimize management strategies and improve patient outcomes in this context.
The SARS-CoV-2 virus triggered the Coronavirus disease 2019 (COVID-19) pandemic and has significantly impacted societies and global health systems. This disease has devastated the world, leading to several million infections and more than 7 million deaths (1) Initially, it was thought that the respiratory system was most affected by SARS-CoV-2 infection and that the virus only caused respiratory symptoms such as a mild flu-like illness or severe acute respiratory distress syndrome (ARDS), but further investigation revealed that many organs/systems could be affected in the short and long term (2). One of the complications associated with this disease is rhabdomyolysis (RML), which is increasingly recognized as a remarkable phenomenon. Previous research has shown that RML may be a possible consequence of COVID-19 (3, 4).
Numerous case studies have shown that RML is an early or later severe manifestation of COVID-19 (3, 4). Therefore, it is essential to consider the possibility of RML in individuals who have contracted COVID-19 or been vaccinated, as timely detection and intervention are crucial for managing this potentially fatal disease (5, 6). Therefore, the main objective of this study is to provide a comprehensive analysis of the epidemiology, pathophysiology, clinical presentation, laboratory observations, and therapeutic approaches related to RML as a complication of COVID-19 infection.
A structured approach was followed to gather relevant studies on RML secondary to COVID-19 infection and vaccination for this narrative review. Essential keywords and medical subject headings (MeSH) such as “COVID-19 infection,” “vaccination,” “complications,” and “rhabdomyolysis” were identified to guide the searches through major databases like PubMed and Google Scholar. A wide range of peer-reviewed articles, clinical reports, and case studies were captured. Studies published in English since the onset of the pandemic in 2019 that specifically addressed RML in relation to COVID-19 or its vaccines were included, while papers unrelated to the topic, non-peer-reviewed articles, and those beyond the scope of inquiry were excluded.
After the literature was collected, each study’s design, population, clinical outcomes, and treatment strategies were carefully reviewed. Patterns in the presentation of RML in patients with COVID-19 or following vaccination were identified, along with potential complications. Gaps in the research, such as the limited number of large-scale studies and the need for further investigation into the mechanisms underlying this condition, were noted. A synthesis of these findings was completed to provide an overview of current knowledge and insights into future research needs. This review of literature, using the SANRA (Scale for the Assessment of Narrative Review Articles) scale, resulted in a total score of 9.5/12, reflecting a high standard of quality.
RML is a pathological condition characterized by the breakdown of skeletal muscle tissue and the consequent release of intracellular muscle components, including electrolytes, enzymes, and myoglobin, into the circulatory system, potentially causing systemic complications (1). RML can arise from diverse sources, such as trauma, extended muscle compression, ischemia, drug-induced toxicity, metabolic irregularities, and infections, all of which inflict damage on muscle cell membranes, compromising the structural integrity of muscle fibers (7). A common factor between traumatic and non-traumatic forms of RML is muscle necrosis. RML can have a range of repercussions on the body, from minor elevations in muscle enzymes in the bloodstream without any symptoms to serious, potentially fatal consequences, such as acute renal injury (AKI) and abnormalities in electrolyte balance (8). In individuals with COVID-19, RML has been documented as a potential delayed complication, presenting symptoms such as fatigue, myalgia, and discomfort in the lower extremities. It may be linked to COVID-19 infection and, in rare instances, can occur after COVID-19 vaccination (9, 10). RML is prevalent, with most cases not requiring intensive care unit-level interventions. Rapid recognition and management of RML are crucial to minimizing the risk of serious complications, such as renal failure and electrolyte disturbances (11).
Numerous factors play a role in the onset of RML, including direct infiltration and toxic breakdown of muscle fibers due to various factors, such as physical trauma, strenuous exercise, medications, drugs, infectious pathogens, metabolic irregularities, electrolyte imbalances (e.g., hypokalemia, hypophosphatemia), genetic anomalies, or as a sequela of viral infections such as SARS-CoV-2 (7, 12). The distinguishing pathophysiological feature of the syndrome involves an elevation in intracellular free ionized calcium levels, stemming from either cellular energy depletion or direct rupture of the plasma membrane. This elevated intracellular calcium level triggers various proteases, enhances the contractility of skeletal muscle cells, leads to mitochondrial dysfunction, and increases the generation of reactive oxygen species, culminating in the death of skeletal muscle cells (13).
Following muscle cell injury, intracellular components such as myoglobin, enzymes (lactate dehydrogenase and creatine kinase), electrolytes (phosphate and potassium), and other proteins are released into the bloodstream (14). Myoglobin, a heme-containing protein in muscle cells, plays a significant role in the pathophysiology of RML and is among the primary substances released. Typically, myoglobin exhibits a weak binding affinity with plasma globulin and is excreted in small amounts in urine. However, myoglobin’s excessive release overwhelms the plasma proteins’ binding capacity during RML. Elevated levels of myoglobin in the blood can lead to kidney injury and contribute to the formation of toxic compounds, resulting in metabolic acidosis. Consequently, myoglobin undergoes glomerular filtration and reaches the renal tubules, potentially leading to renal impairment and dysfunction (15).
RML induces significant changes in cellular metabolism, including a surge in creatine kinase (CK) levels due to muscle cell damage; electrolyte imbalances resulting from the release of potassium, phosphate, and myoglobin into the bloodstream; and metabolic acidosis triggered by toxic compounds formed by myoglobin release. Additionally, myoglobin release can cause acute kidney injury by damaging renal tubules, whereas elevated potassium levels from muscle cell damage can lead to hyperkalemia, potentially causing cardiac arrhythmias (16). Muscle injury initiates a series of physiological processes that release extracellular calcium ions into the intracellular compartment. Excessive amounts of calcium ions in the body may result in abnormal interactions between contractile proteins, myosin, and actin. This, in turn, can contribute to the degradation and damage of muscle fibers and the overall muscle tissue (17). In typical myocytes, Na-K-ATP-ase pumps are inside the cellular membrane, facilitating the active transportation of sodium ions to the extracellular space. This process contributes to establishing a negative membrane potential (18). Additionally, it should be noted that the Na+/Ca2+ exchanger is located inside the cellular membrane. The pump facilitates the re-entry of sodium ions into the cell while concurrently transporting calcium ions out of the cell (19). This mechanism depends on adenosine triphosphate (ATP) as a primary energy source. The central mechanism underlying RML is the influx of calcium into the cell, which is triggered by ATP depletion and cell membrane breakdown. The activation of phospholipase A2, proteases, and vasoactive molecules by excess intracellular calcium leads to the generation of free radicals (20).
Most injuries tend to develop after blood flow is restored to a specific location of the damage. Reperfusion outcomes include the transportation of activated neutrophils to tissues previously deprived of blood flow, leading to the generation of free radicals originating from reactive oxygen species. These free radicals can potentially harm the cell membrane’s lipid bilayer via lipid peroxidation (21).
The traditional clinical triad associated with RML comprises muscular weakness, myalgia, and black urine (14). However, it should be noted that not all patients exhibit these symptoms (22). Numerous clinical manifestations of RML exhibit a lack of specificity and encompass a spectrum of presentations, ranging from asymptomatic cases with elevated enzyme levels to critical conditions characterized by multiple electrolyte imbalances such as hyperphosphatemia, hyperkalemia, hypocalcemia, severe depletion of intravascular volume, metabolic acidosis, and acute renal failure. The clinical presentation of classical triads includes manifestations such as myalgia, general discomfort, and the presence of urine with a characteristic tea-like coloration. Nevertheless, it has been documented that these symptoms occur in less than 10% of individuals (17, 23, 24).
The identification of RML necessitates clinical suspicion, and clinicians need to consider this diagnosis consistently, particularly in patients exhibiting a typical trio with established risk factors. Timely identification of this ailment contributes to the mitigation of associated problems (16). Measurement of serum creatine kinase (CK) level is often regarded as the most reliable and widely accepted laboratory diagnostic test (11). Serum CK levels are often below 100 U/L. No established threshold exists for creatine kinase levels; however, readings >1,000 U/L indicate RML. Many doctors choose values between three and five times the upper normal range, which is between 100 and 400 IU/L (or approximately 1,000 IU/L) (1). Elevated serum CK levels have been identified as predictive factors for the development of acute renal failure (25). However, using myoglobin as a conventional diagnostic test is limited by its short half-life. However, an elevated concentration of myoglobin in individuals suspected of having RML may indicate the first stage of the condition (24, 26). Additional diagnostic methods for RML include myoglobinuria and positive urine dipstick tests with an orthotolidine (OT) test (27).
Addressing the root cause of muscle injury is the initial step in managing RML. The primary approach to treating RML involves providing supportive care, primarily by ensuring sufficient hydration to prevent acute renal failure. Early and vigorous administration of fluid replacement with crystalloid solutions is pivotal for controlling and managing AKI resulting from RML. Electrolyte imbalance should be managed using standard medical protocols (1). Intravenous fluid resuscitation can help prevent renal problems. Hypovolemia can worsen acute renal failure. Hemodynamic monitoring is essential for cardiovascular or renal illness (28). Administering 0.9% sodium chloride solution before extricating entrapped individuals can reduce the occurrence of acute renal failure. Alkalizing urine can prevent myoglobin deposits from forming, which can lead to renal failure (29). Diuretics like mannitol can reduce muscle swelling and hypovolemia. Traditional medicines like bicarbonate therapy and dextrose-insulin are used for managing hyperkalemia (30). Hemodialysis is effective for managing electrolyte imbalances. Hemodiafiltration and continuous renal replacement approach help manage cardiovascular instabilities in patients (29).
The significance of complications associated with RML stems from the fact that the majority of clinical manifestations seen in cases of RML may be attributed to these complications. Complications can be categorized into two distinct classes: early and late complications (31). Early consequences include hyperkalemia, hypocalcemia, hepatic inflammation, cardiac arrhythmia, and cardiac arrest, as stated in the literature (17). Late concerns, on the other hand, include acute renal failure and disseminated intravascular coagulation. The spectrum of complications varies from slight increases in creatinine phosphokinase levels to severe medical crises such as compartment syndrome, intravascular fluid depletion, disseminated intravascular coagulation, pigment-induced acute kidney injury (AKI), and cardiac arrhythmias (1, 32).
COVID-19 is an infectious respiratory illness caused by the novel SARS-CoV-2. It was first identified in December 2019 in Wuhan, China, and has since spread globally, leading to a pandemic (33). The SARS-CoV-2 virus primarily spreads through respiratory droplets when an infected person coughs, sneezes, or talks. It can also spread by touching surfaces contaminated with the virus and touching one’s face, particularly the mouth, nose, or eyes (34). Several factors increase the risk of contracting COVID-19, including close contact with infected individuals, especially in enclosed spaces with poor ventilation. Other risk factors include advanced age, underlying health conditions such as cardiovascular disease, diabetes, obesity, and compromised immune systems (35). COVID-19 can lead to various complications, ranging from mild to severe. Common complications include pneumonia, ARDS, multi-organ failure, sepsis, blood clots, and neurological complications. Specific individuals, particularly older adults and those with underlying health conditions, are at higher risk of developing severe complications (36, 37).
Diagnosis of COVID-19 typically involves a combination of clinical symptoms, laboratory testing, and imaging studies. Common symptoms include fever, cough, shortness of breath, fatigue, muscle aches, loss of taste or smell, sore throat, and headache (38). Laboratory tests, such as polymerase chain reaction (PCR) or antigen tests, are used to detect the presence of the SARS-CoV-2 virus in respiratory samples (39, 40). Chest X-rays or computed tomography (CT) scans may be performed to assess lung involvement in severe cases (41). Treatment for COVID-19 varies depending on the severity of the illness. Mild cases may only require supportive care, such as rest, hydration, and over-the-counter medications to relieve symptoms. In moderate to severe cases, hospitalized patients may receive oxygen therapy, corticosteroids to reduce inflammation, antiviral medications like remdesivir, and other supportive treatments. Intensive care measures such as mechanical ventilation and extracorporeal membrane oxygenation (ECMO) may be necessary in critically ill patients (41–43).
The COVID-19 vaccination stands as a crucial tool in the global endeavor to combat the ongoing pandemic. Different COVID-19 vaccines employ diverse technologies to elicit immune responses against the SARS-CoV-2 virus (44). Different types of COVID-19 vaccines are available, including mRNA, viral vector, protein subunit, and inactivated or attenuated virus vaccines. All vaccines aim to trigger immunity against the virus and require one or two doses. Vaccination is crucial in achieving widespread immunity and controlling the spread of the disease (45, 46). The primary technologies behind COVID-19 vaccines include mRNA-based vaccines, such as those developed by Pfizer-BioNTech and Moderna, and viral vector vaccines, like the AstraZeneca and Johnson & Johnson vaccines (47). Additionally, studies have shown that COVID-19 vaccines can lead to regional immune reactions, as evidenced by changes in 18F-FDG PET/CT scans, which can help understand the vaccines’ immunogenicity (47).
COVID-19 has been associated with increased RML, characterized by the breakdown of skeletal muscle tissue and the release of muscle cell content into the bloodstream. RML is a significant complication associated with COVID-19 since it has the potential to exacerbate patients’ clinical manifestations and may result in mortality (24). It has been identified as an initial clinical presentation and subsequent outcome of SARS-CoV-2 infection (4). Several studies have documented instances of RML in COVID-19 patients, particularly in those with severe illness or complications, underscoring the importance of recognizing this potentially life-threatening manifestation of the disease (4, 48–50). The precise relationship between COVID-19 and RML remains incompletely understood; however, it is believed to be linked to the body’s immune response to the virus.
A retrospective analysis of 1,079 COVID-19 patients admitted to the ICU investigated their features and outcomes. The study discovered that acute kidney damage (AKI) was the most important predictor of mortality among patients infected with COVID-19 and undergoing rhabdomyolysis. RML was linked to a higher risk of death in ICU patients with COVID-19, with acute renal injury being the best predictor of fatal outcomes. These findings emphasize the vital need for early detection and treatment of RML in individuals with severe COVID-19 (51).
Table 1 presents the summary of variables and outcomes of case report studies of RML secondary to COVID-19 infection and vaccination (5, 6, 9, 48–50, 52–107). Table 2 summarizes cases of RML secondary to COVID-19 infection and vaccination and its associated outcomes.
Table 1. Variables and outcomes of patients with rhabdomyolysis secondary to COVID-19 infection and vaccination.
The precise mechanism linking COVID-19 infection to RML remains incompletely understood; however, it is hypothesized to encompass multiple factors, including direct infiltration of muscle cells by the virus, systemic inflammation, cytokine release syndrome, and administration of specific medications in COVID-19 management. One key aspect is the direct invasion of muscles by SARS-CoV-2, causing muscle damage and necrosis and releasing intracellular contents into the bloodstream (90). Nonetheless, two mechanisms have been delineated as complications of viral infections culminating in RML. First, the virus directly invades the muscle cells, leading to their death. Second, cytokines and immunological factors affect the host response, producing toxic effects on muscle cells (88). The mechanisms underlying virus-induced muscle degradation may involve direct viral toxicity and cytokine-mediated muscle impairment (108). Furthermore, in COVID-19 patients, immunological pathways and mechanisms may contribute to viral myositis and subsequent development of RML (82) (see Figure 1).
Figure 1. RML hypothesized the pathophysiology after vaccination for COVID-19 (Created by the author using BioRender).
RML is a notable clinical manifestation in individuals with COVID-19, manifesting either as an initial symptom or emerging at any juncture during the illness (56). The clinical manifestations of RML among COVID-19 patients can exhibit considerable variation, encompassing symptoms such as muscular discomfort, weakness, and tenderness, notably concentrated in affected muscle groups (109). Darkened urine attributable to the presence of myoglobin stemming from muscle breakdown may be observed in some instances. Additionally, individuals may report fatigue, general malaise, and challenges in mobility or executing routine activities (110). In certain instances, the discovery of RML among COVID-19 patients may be serendipitous, alongside predominant symptoms, such as cough and fever. Vigilant monitoring of creatine kinase (CK) levels is essential for the timely detection of RML (56). Instances of RML serving as the initial indication for COVID-19 have been documented in pediatric patients, underscoring the importance of heightened clinical vigilance in any patient exhibiting indications or symptoms suggestive of RML (91). In severe cases, RML can precipitate complications, such as acute kidney injury, disturbances in electrolyte balance, and cardiac dysrhythmias. Notably, not all COVID-19 patients exhibiting RML manifest overt symptoms; some instances may only be discernible via laboratory assessments revealing heightened levels of muscle enzymes in the bloodstream.
In COVID-19 patients, RML can be a complication that requires specific attention (9). Prompt recognition and management of RML in COVID-19 patients are essential to prevent further complications and optimize outcomes. Monitoring for symptoms of RML, such as muscle pain, weakness, and dark urine, is critical in COVID-19 patients to promptly address potential complications (56, 82). Patients diagnosed with RML require aggressive fluid therapy to mitigate the risk of acute kidney injury; however, it is essential to exercise caution in individuals with COVID-19 to prevent worsening of oxygenation and respiratory issues due to fluid overload. Prompt identification of this condition is imperative for effective management and prevention of potential complications (56). Treatment encompasses measures such as fluid administration, diuretic therapy, and, in severe instances, continuous renal replacement therapy (88). Timely identification and appropriate management are paramount in addressing COVID-19, underscoring the importance of precisely understanding its clinical manifestations (111).
Adverse effects associated with COVID-19 vaccination are generally mild and temporary, and serious complications are uncommon. Common side effects include discomfort, swelling, redness at the injection site, fatigue, headaches, muscle aches, chills, nausea, and fever. These effects typically subside within a few days and are comparable to those observed with routine vaccinations (112). However, there have been documented instances of RML occurring after the COVID-19 vaccination, albeit rarely. Cases have been reported in which individuals developed RML following the administration of COVID-19 mRNA vaccines, such as Comirnaty (BioNTech/Pfizer), have been reported. These cases underscore the importance of promptly identifying potential adverse reactions for appropriate management (113–115). The onset of RML after vaccination can vary, ranging from 5 to 10 days post-vaccination. Treatment typically involves intravenous fluids, with outcomes ranging from partial improvement to fatality (115). Although vaccination remains crucial for mitigating COVID-19 complications, healthcare providers should be vigilant about the rare occurrence of RML as a manageable adverse reaction to COVID-19 vaccination (116). Early recognition, diagnosis, and supportive care are crucial to ensure favorable outcomes for individuals who develop RML following vaccination (113–115).
Although the mechanism underlying RML following COVID-19 vaccination has not been completely elucidated, it is proposed to be an uncommon nonspecific reaction to immunization rather than specific to mRNA or COVID-19 vaccines. Instances of RML have been reported as rare adverse events following mRNA COVID-19 vaccination, notably in patients receiving high-dose statins combined with fibrates (116). The muscle breakdown process after COVID-19 vaccination involves exposing muscles to modified contaminant agents through direct injection, provoking an immune response to the injected antigen. This immune response can induce muscle toxicity associated with the inciting agent, its constituents, and the host immune or inflammatory response. Although the precise mechanism causing damage to the injected muscle remains incompletely understood, toxic myopathy may contribute to reported pain at the vaccination site. In cases of RML post-COVID vaccination, skeletal muscle breakdown can occur following insult or injury, with potential triggers including trauma, excessive physical activity, immobilization, drug use, medications, or neuroleptic malignant syndrome (113, 116, 117). In the context of COVID-19 vaccination, instances of RML have been documented, with proposed mechanisms including autoimmune responses triggered by the vaccine and activation of CD4+ and CD8+ T cell responses against viral spike proteins (118). The proposed mechanisms for renal failure in RML include renal tubular obstruction due to myoglobin accumulation in the kidneys and free radical-mediated cytotoxicity, leading to tubular necrosis (119) (see Figure 1).
In conclusion, the increased incidence of RML in severe COVID-19 cases, likely driven by intense inflammation and potential direct viral invasion of muscle tissue, along with its rare occurrence following COVID-19 vaccination, underscores the need for heightened clinical vigilance. Early recognition and prompt management are essential to prevent serious complications, particularly through aggressive hydration and electrolyte correction. Clinicians should remain alert to the possibility of RML in patients presenting with neuromuscular symptoms post-vaccination and assess for cardiac involvement due to associated conditions like myocarditis. A thorough understanding of RML’s diverse presentations and risks in COVID-19 is critical for improving patient outcomes and enhancing safety during the pandemic.
It is important to acknowledge that the findings are primarily based on case reports, which may limit the generalizability of the conclusions drawn. Additionally, the lack of randomized controlled trials on RML associated with COVID-19 infection and vaccination presents a significant gap in the literature. Further research is necessary to investigate the processes contributing to mortality associated with RML and to develop therapies that could effectively improve patient outcomes within this group.
MK: Conceptualization, Data curation, Formal analysis, Funding acquisition, Investigation, Methodology, Project administration, Resources, Software, Supervision, Validation, Visualization, Writing – original draft, Writing – review & editing. NF: Writing – original draft. KA: Writing – original draft. RN: Writing – original draft. MaM: Writing – original draft. FJ: Writing – original draft. PK: Writing – original draft. MA: Writing – original draft, Conceptualization, Data curation, Formal analysis, Funding acquisition, Investigation, Methodology, Project administration, Resources, Software, Supervision, Validation, Visualization. MS: Writing – original draft. MoM: Writing – original draft.
The author(s) declare that no financial support was received for the research, authorship, and/or publication of this article.
The authors declare that the research was conducted in the absence of any commercial or financial relationships that could be construed as a potential conflict of interest.
All claims expressed in this article are solely those of the authors and do not necessarily represent those of their affiliated organizations, or those of the publisher, the editors and the reviewers. Any product that may be evaluated in this article, or claim that may be made by its manufacturer, is not guaranteed or endorsed by the publisher.
RML, Rhabdomyolysis; COVID-19, Coronavirus disease 2019; ARDS, Acute respiratory distress syndrome.
1. Stanley, M, Chippa, V, Aeddula, NR, Quintanilla Rodriguez, BS, and Adigun, R. Rhabdomyolysis. Treasure Island, FL: StatPearls Publishing LLC (2024).
2. Rabaan, AA, Smajlović, S, Tombuloglu, H, Ćordić, S, Hajdarević, A, Kudić, N, et al. SARS-CoV-2 infection and multi-organ system damage: a review. Biomol Biomed. (2023) 23:37–52. doi: 10.17305/bjbms.2022.7762
3. Haroun, MW, Dieiev, V, Kang, J, Barbi, M, Nia, SFM, Gabr, M, et al. Rhabdomyolysis in COVID-19 patients: a retrospective observational study. Cureus. (2021) 13:12552. doi: 10.7759/cureus.12552
4. Bawor, M, Sairam, S, Rozewicz, R, Viegas, S, Comninos, AN, and Abbara, A. Rhabdomyolysis after COVID-19 infection: a case report and review of the literature. Viruses. (2022) 14:2255. doi: 10.3390/v14102255
5. Kartalova, MJ, Cvetanovska, M, Stevanovic, M, Demiri, I, Bogoeva, ST, and Cvetanovski, V. Covid-19 pneumonia associated with rhabdomyolysis in patient with previous chronic therapy with statin: coincidence or realted conditions? J Morphol Sci. (2021) 4:6–12. doi: 10.55302/JMS2141006jk
6. Byler, J, Harrison, R, and Fell, LL. Rhabdomyolysis following recovery from severe COVID-19: a case report. Am J Case Rep. (2021) 22:e931616–1. doi: 10.12659/AJCR.931616
7. Harmelink, M. Uncommon causes of rhabdomyolysis. Crit Care Clin. (2022) 38:271–85. doi: 10.1016/j.ccc.2021.11.004
8. Kodadek, L, Carmichael Ii, SP, Seshadri, A, Pathak, A, Hoth, J, Appelbaum, R, et al. Rhabdomyolysis: an American Association for the Surgery of Trauma critical care committee clinical consensus document. Trauma Surg Acute Care Open. (2022) 7:e000836. doi: 10.1136/tsaco-2021-000836
9. Jin, M, and Tong, Q. Rhabdomyolysis as potential late complication associated with COVID-19. Emerg Infect Dis. (2020) 26:1618–20. doi: 10.3201/eid2607.200445
10. Hannah, JR, Ali, SS, Nagra, D, Adas, MA, Buazon, AD, Galloway, JB, et al. Skeletal muscles and Covid-19: a systematic review of rhabdomyolysis and myositis in SARS-CoV-2 infection. Clin Exp Rheumatol. (2022) 40:329–38. doi: 10.55563/clinexprheumatol/mkfmxt
11. Paquot, F, and Jouret, F. Diagnostic approach to rhabdomyolysis. Rev Med Liege. (2023) 78:529–32.
12. Bagley, WH, Yang, H, and Shah, KH. Rhabdomyolysis. Intern Emerg Med. (2007) 2:210–8. doi: 10.1007/s11739-007-0060-8
13. Giannoglou, GD, Chatzizisis, YS, and Misirli, G. The syndrome of rhabdomyolysis: pathophysiology and diagnosis. Eur J Intern Med. (2007) 18:90–100. doi: 10.1016/j.ejim.2006.09.020
14. Warren, JD, Blumbergs, PC, and Thompson, PD. Rhabdomyolysis: a review. Muscle Nerve. (2002) 25:332–47. doi: 10.1002/mus.10053
15. Zager, RA. Rhabdomyolysis and myohemoglobinuric acute renal failure. Kidney Int. (1996) 49:314–26. doi: 10.1038/ki.1996.48
16. Torres, PA, Helmstetter, JA, Kaye, AM, and Kaye, AD. Rhabdomyolysis: pathogenesis, diagnosis, and treatment. Ochsner J. (2015) 15:58–69.
18. Luck, RP, and Verbin, S. Rhabdomyolysis: a review of clinical presentation, etiology, diagnosis, and management. Pediatr Emerg Care. (2008) 24:262–8. doi: 10.1097/PEC.0b013e31816bc7b7
19. Cabral, BMI, Edding, SN, Portocarrero, JP, and Lerma, EV. Rhabdomyolysis. Dis Mon. (2020) 66:101015. doi: 10.1016/j.disamonth.2020.101015
20. Knochel, JP. Mechanisms of rhabdomyolysis. Curr Opin Rheumatol. (1993) 5:725–31. doi: 10.1097/00002281-199305060-00006
21. Malinoski, DJ, Slater, MS, and Mullins, RJ. Crush injury and rhabdomyolysis. Crit Care Clin. (2004) 20:171–92. doi: 10.1016/S0749-0704(03)00091-5
22. Mannix, R, Tan, ML, Wright, R, and Baskin, M. Acute pediatric rhabdomyolysis: causes and rates of renal failure. Pediatrics. (2006) 118:2119–25. doi: 10.1542/peds.2006-1352
23. Zutt, R, Van Der Kooi, A, Linthorst, G, Wanders, R, and De Visser, M. Rhabdomyolysis: review of the literature. Neuromuscul Disord. (2014) 24:651–9. doi: 10.1016/j.nmd.2014.05.005
24. Huerta-Alardín, AL, Varon, J, and Marik, PE. Bench-to-bedside review: rhabdomyolysis–an overview for clinicians. Crit Care. (2004) 9:158–12. doi: 10.1186/cc2978
25. Cervellin, G, Comelli, I, and Lippi, G. Rhabdomyolysis: historical background, clinical, diagnostic and therapeutic features. Clin Chem Lab Med. (2010) 48:749–56. doi: 10.1515/CCLM.2010.151
26. Al-Ismaili, Z, Piccioni, M, and Zappitelli, M. Rhabdomyolysis: pathogenesis of renal injury and management. Pediatr Nephrol. (2011) 26:1781–8. doi: 10.1007/s00467-010-1727-3
28. Allison, RC, and Bedsole, DL. The other medical causes of rhabdomyolysis. Am J Med Sci. (2003) 326:79–88. doi: 10.1097/00000441-200308000-00005
29. Hunter, JD, Gregg, K, and Damani, Z. Rhabdomyolysis. Contin Educ Anaesth Crit Care Pain. (2006) 6:141–3. doi: 10.1093/bjaceaccp/mkl027
30. Vanholder, R, Sever, MS, Erek, E, and Lameire, N. Rhabdomyolysis. J Am Soc Nephrol. (2000) 11:1553–61. doi: 10.1681/ASN.V1181553
31. Russell, TA. Acute renal failure related to rhabdomyolysis: pathophysiology, diagnosis, and collaborative management. Nephrol Nurs J. (2005) 32:567–75.
32. Chatzizisis, YS, Misirli, G, Hatzitolios, AI, and Giannoglou, GD. The syndrome of rhabdomyolysis: complications and treatment. Eur J Intern Med. (2008) 19:568–74. doi: 10.1016/j.ejim.2007.06.037
33. Baloch, S, Baloch, MA, Zheng, T, and Pei, X. The coronavirus disease 2019 (COVID-19) pandemic. Tohoku J Exp Med. (2020) 250:271–8. doi: 10.1620/tjem.250.271
34. Rauf, A, Abu-Izneid, T, Olatunde, A, Ahmed Khalil, A, Alhumaydhi, FA, Tufail, T, et al. COVID-19 pandemic: epidemiology, etiology, conventional and non-conventional therapies. Int J Environ Res Public Health. (2020) 17:18155. doi: 10.3390/ijerph17218155
35. Liu, T, Liang, W, Zhong, H, He, J, Chen, Z, He, G, et al. Risk factors associated with COVID-19 infection: a retrospective cohort study based on contacts tracing. Emerg Microbes Infect. (2020) 9:1546–53. doi: 10.1080/22221751.2020.1787799
36. Desai, AD, Lavelle, M, Boursiquot, BC, and Wan, EY. Long-term complications of COVID-19. Am J Physiol Cell Physiol. (2022) 322:C1–c11. doi: 10.1152/ajpcell.00375.2021
37. Lim, JT, Liang En, W, Tay, AT, Pang, D, Chiew, CJ, Ong, B, et al. Long-term cardiovascular, cerebrovascular, and other thrombotic complications in COVID-19 survivors: a retrospective cohort study. Clin Infect Dis. (2024) 78:70–9. doi: 10.1093/cid/ciad469
38. Falzone, L, Gattuso, G, Tsatsakis, A, Spandidos, DA, and Libra, M. Current and innovative methods for the diagnosis of COVID-19 infection. Int J Mol Med. (2021) 47:1–23. doi: 10.3892/ijmm.2021.4933
39. Mehrban, A, Hajikolaei, FA, Karimi, M, Khademi, R, Ansari, A, Qujeq, D, et al. Evaluation of elevated serum apelin-13 and D-dimer concentrations in individuals diagnosed with pulmonary embolism. Int J Emerg Med. (2024) 17:48. doi: 10.1186/s12245-024-00619-z
40. Hayden, MK, Hanson, KE, Englund, JA, Lee, F, Lee, MJ, Loeb, M, et al. The Infectious Diseases Society of America guidelines on the diagnosis of COVID-19: antigen testing (January 2023). Clin Infect Dis. (2024) 78:e350–84. doi: 10.1093/cid/ciad032
41. Pascarella, G, Strumia, A, Piliego, C, Bruno, F, Del Buono, R, Costa, F, et al. COVID-19 diagnosis and management: a comprehensive review. J Intern Med. (2020) 288:192–206. doi: 10.1111/joim.13091
42. Cascella, M, Rajnik, M, Aleem, A, Dulebohn, SC, and Di Napoli, R. Features, Evaluation, and treatment of coronavirus (COVID-19). Treasure Island, FL: StatPearls Publishing LLC (2024).
43. Bargahi, M, Alavi-Moghaddam, M, Karimi, M, Azizan, Z, Jafarzadeh, F, Javaherian, M, et al. Safety and efficacy of incentive spirometer in Covid-19 pneumonia; a randomized clinical trial. Arch Acad Emerg Med. (2024) 12:e51. doi: 10.22037/aaem.v12i1.2244
44. Abdel-Qader, DH, Abdel-Qader, H, Silverthorne, J, Kongkaew, C, Meslamani, AZA, Hayajneh, W, et al. Real-world effectiveness of four types of COVID-19 vaccines. Vaccines (Basel). (2023) 11:985. doi: 10.3390/vaccines11050985
45. Gote, V, Bolla, PK, Kommineni, N, Butreddy, A, Nukala, PK, Palakurthi, SS, et al. A comprehensive review of mRNA vaccines. Int J Mol Sci. (2023) 24:2700. doi: 10.3390/ijms24032700
46. Han, X, Xu, P, and Ye, Q. Analysis of COVID-19 vaccines: types, thoughts, and application. J Clin Lab Anal. (2021) 35:e23937. doi: 10.1002/jcla.23937
47. Ayati, N, Evans, S, Zakavi, SR, and Gruenewald, SM. Comparison between viral vector and mRNA based COVID-19 vaccination in prevalence and severity of regional immune reactions, and (18)F-FDG PET/CT features. Asia Ocean J Nucl Med Biol. (2023) 11:4–12. doi: 10.22038/AOJNMB.2022.63110.1443
48. Husain, R, Corcuera-Solano, I, Dayan, E, Jacobi, AH, and Huang, M. Rhabdomyolysis as a manifestation of a severe case of COVID-19: a case report. Radiol Case Rep. (2020) 15:1633–7. doi: 10.1016/j.radcr.2020.07.003
49. Gefen, AM, Palumbo, N, Nathan, SK, Singer, PS, Castellanos-Reyes, LJ, and Sethna, CB. Pediatric COVID-19-associated rhabdomyolysis: a case report. Pediatr Nephrol. (2020) 35:1517–20. doi: 10.1007/s00467-020-04617-0
50. Solís, JG, Pineda, AE, Minutti, PA, and Sánchez, AA. Case report: rhabdomyolysis in a patient with COVID-19: a proposed diagnostic-therapeutic algorithm. Am J Trop Med Hyg. (2020) 103:1158–61. doi: 10.4269/ajtmh.20-0692
51. Nashwan, AJ, Alassaf, A, Abujaber, AA, Al Wraidat, M, Ananthegowda, DC, Al-Kaabi, SK, et al. Rhabdomyolysis in critically ill patients with COVID-19: a retrospective study. Cureus. (2023) 15:e37333. doi: 10.7759/cureus.37333
52. Alrubaye, R, and Choudhury, H. Severe rhabdomyolysis in a 35-year-old woman with COVID-19 due to SARS-CoV-2 infection: a case report. Am J Case Rep. (2020) 21:e926733. doi: 10.12659/AJCR.926733
53. Anklesaria, Z, Frankman, J, Gordin, J, Zhan, J, and Liu, AK. Fatal rhabdomyolysis in a COVID-19 patient on rosuvastatin. Cureus. (2020) 12:11186. doi: 10.7759/cureus.11186
54. Chan, KH, Farouji, I, Hanoud, AA, and Slim, J. Weakness and elevated creatinine kinase as the initial presentation of coronavirus disease 2019 (COVID-19). Am J Emerg Med. (2020) 38:1548.e1–3. doi: 10.1016/j.ajem.2020.05.015
55. Chedid, NR, Udit, S, Solhjou, Z, Patanwala, MY, Sheridan, AM, and Barkoudah, E. COVID-19 and rhabdomyolysis. J Gen Intern Med. (2020) 35:3087–90. doi: 10.1007/s11606-020-06039-y
56. Suwanwongse, K, and Shabarek, N. Rhabdomyolysis as a presentation of 2019 novel coronavirus disease. Cureus. (2020) 12:7561. doi: 10.7759/cureus.7561
57. Mukherjee, A, Ghosh, R, and Aftab, G. Rhabdomyolysis in a patient with coronavirus disease 2019. Cureus. (2020) 12:8956. doi: 10.7759/cureus.8956
58. Anwar, H, and Al, LA. Adolescent COVID-19-associated fatal rhabdomyolysis. J Prim Care Community Health. (2020) 11:2150132720985641. doi: 10.1177/2150132720985641
59. Arai, R, Murata, N, Yamada, A, Migita, S, Koyama, Y, Morikawa, T, et al. Multimodal findings of COVID-19-related rhabdomyolysis complicated with pericarditis mimicking fulminant myocarditis. Circ Rep. (2021) 3:CR-21-0055:419–20. doi: 10.1253/circrep.CR-21-0055
60. Aria, A, Forouharnejad, K, Mortazavi, M, Omidi, A, Askari, M, Ghadimi, K, et al. COVID 19 with neurological symptoms, rhabdomyolysis and brain death: a case report. Am J Clin Exp Immunol. (2020) 9:114–7.
61. Uysal, BB, Ikitimur, H, Yavuzer, S, Islamoglu, MS, and Cengiz, M. Case report: a COVID-19 patient presenting with mild rhabdomyolysis. Am J Trop Med Hyg. (2020) 103:847–50. doi: 10.4269/ajtmh.20-0583
62. Cassim, F, Soni, AJ, and Murphy, S. Severe acute inflammatory myositis and rhabdomyolysis in paediatric SARS-CoV-2-associated MIS-C (multisystem inflammatory syndrome in children). BMJ Case Rep. (2021) 14:e243112. doi: 10.1136/bcr-2021-243112
63. Rivas-García, S, Bernal, J, and Bachiller-Corral, J. Rhabdomyolysis as the main manifestation of coronavirus disease 2019. Rheumatology. (2020) 59:2174–6. doi: 10.1093/rheumatology/keaa351
64. Bach, M, Lim, PP, Azok, J, Ruda Wessell, K, Desai, AP, and Dirajlal-Fargo, S. Anaphylaxis and rhabdomyolysis: a presentation of a pediatric patient with COVID-19. Clin Pediatr. (2021) 60:202–4. doi: 10.1177/0009922821999470
65. Azeem, A, Salat, H, and Haragsim, L. Hypophosphatemic rhabdomyolysis with moderate diabetic ketoacidosis in a COVID-19 patient needing continous renal replacement therapy. TP47 TP047 COVID and ARDS CASE reports: American Thoracic Society, p. A2470. (2021).
66. Fadel, C, Phan, N, and Kaur, A. Liberal intravenous fluid Administration in a Rare Case of severe rhabdomyolysis secondary to SARS-CoV-2. Cureus. (2021) 13:17234. doi: 10.7759/cureus.17234
67. Fadiran, O. An atypical case of COVID-19 induced pancytopenia, rhabdomyolysis and myocarditis. Cureus. (2021) 13:12455. doi: 10.7759/cureus.12455
68. Cunha, M, Pinho, I, Lopes, M, Trigueiros, F, Braz, S, and Medeiros, F. A case of corticosteroid-responsive SARS-CoV-2 related massive rhabdomyolysis. IDCases. (2020) 22:e00946. doi: 10.1016/j.idcr.2020.e00946
69. Egoryan, G, Chaudry, S, Yadav, K, Dong, T, Ozcekirdek, E, Ozen, E, et al. Dark urine as the initial manifestation of COVID-19: a case report. J Med Case Rep. (2021) 15:1–5. doi: 10.1186/s13256-021-03173-x
70. Chiba, N, Matsuzaki, M, Mawatari, T, Mizuochi, M, Sakurai, A, and Kinoshita, K. Beneficial effects of dantrolene in the treatment of rhabdomyolysis as a potential late complication associated with COVID-19: a case report. Eur J Med Res. (2021) 26:1–4. doi: 10.1186/s40001-021-00489-8
71. Khosla, SG, Nylen, ES, and Khosla, R. Rhabdomyolysis in patients hospitalized with COVID-19 infection: five case series. J Investig Med High Impact Case Rep. (2020) 8:2324709620984603. doi: 10.1177/2324709620984603
72. Wu, H-Y, Pan, C-T, Cheng, C-F, Lin, C-Y, Chang, S-N, Chen, Y-C, et al. Combined intravenous immunoglobulin and baricitinib treatment for severe COVID-19 with rhabdomyolysis: a case report. J Formos Med Assoc. (2021) 120:1777–81. doi: 10.1016/j.jfma.2021.03.014
73. Fabi, M, Guida, F, Pierantoni, L, Greco, L, di Luca, D, and Lanari, M. Severe rhabdomyolysis in a child with multisystem inflammatory syndrome: an autoimmune mechanism? Pediatr Neurol. (2021) 124:11–2. doi: 10.1016/j.pediatrneurol.2021.07.015
74. Singh-Kucukarslan, G, and Heidemann, D. Atypical presentation of COVID-19 causing rhabdomyolysis: A case report. (2020).
75. Foster, A, Khan, Z, Siddiqui, A, Singh, S, Atere, M, and Nfonoyim, JM. It’s complicated: a case report on a COVID-19-positive HIV patient presenting with rhabdomyolysis and acute kidney injury. SAGE Open Med Case Rep. (2020) 8:2050313X20965423. doi: 10.1177/2050313X20965423
76. Valente-Acosta, B, Moreno-Sanchez, F, Fueyo-Rodriguez, O, and Palomar-Lever, A. Rhabdomyolysis as an initial presentation in a patient diagnosed with COVID-19. BMJ Case Rep. (2020) 13:e236719. doi: 10.1136/bcr-2020-236719
77. Chong, WH, and Saha, B. Severe acute respiratory syndrome coronavirus 2 (SARS-CoV-2) associated with rhabdomyolysis and acute kidney injury (AKI). Am J Med Sci. (2020) 360:738–9. doi: 10.1016/j.amjms.2020.07.032
78. Samies, NL, Pinninti, S, and James, SH. Rhabdomyolysis and acute renal failure in an adolescent with coronavirus disease 2019. J Pediatr Infect Dis Soc. (2020) 9:507–9. doi: 10.1093/jpids/piaa083
79. Mahmood, UA, Mermis, JD, Khan, N-M, and El Atrouni, W. Rhabdomyolysis with COVID-19. Infect Dis Clin Pract. (2021) 29:e129:–e130. doi: 10.1097/IPC.0000000000000968
80. Legrand, F, Chong-Nguyen, C, and Ghanem, N. Myopericarditis, rhabdomyolysis, and acute hepatic injury: sole expression of a SARS-CoV-2 infection. Circulation. Cardiovasc Imaging. (2020) 13:e010907. doi: 10.1161/CIRCIMAGING.120.010907
81. Mah, TJ, Lum, YH, and Fan, BE. Coronavirus disease 2019 presenting with rhabdomyolysis. Proc Singapore Healthc. (2021) 30:68–70. doi: 10.1177/2010105820943911
82. Meegada, S, Muppidi, V, Wilkinson, DC III, Siddamreddy, S, and Katta, SK. Coronavirus disease 2019-induced rhabdomyolysis. Cureus. (2020) 12:10123. doi: 10.7759/cureus.10123
83. Jahnke, VS, Poloni, JAT, Neves, CAM, Peter, C, Thompson, CE, and Rotta, LN. Acute kidney injury associated with rhabdomyolysis in a patient with COVID-19. Brazilian. J Nephrol. (2021) 44:443–6. doi: 10.1590/2175-8239-jbn-2020-0170
84. Li, APZ, Thomas, S, Gokmen, R, and Kariyawasam, D. Rhabdomyolysis and severe biphasic disturbance of calcium homeostasis secondary to COVID-19 infection. BMJ Case Rep. (2021) 14:e239611. doi: 10.1136/bcr-2020-239611
85. Murillo, F, Ramos, G, Del Pozo, JL, Valdez, LM, and Zagaceta, J. SARS-CoV-2 infection with associated rhabdomyolysis and probable myocarditis. Eur J Case Rep Intern Med. (2020) 7:1867. doi: 10.12890/2020_001867
86. Patel, V, Alzghoul, B, and Kalra, SS. COVID-19 infection and severe rhabdomyolysis. Baylor University medical center proceedings. New York:: Taylor and Francis (2021).
87. Su, D, and Kamangar, N. Late-onset rhabdomyolysis in SARS-COV-2. Chest. (2020) 158:A982. doi: 10.1016/j.chest.2020.08.913
88. Taxbro, K, Kahlow, H, Wulcan, H, and Fornarve, A. Rhabdomyolysis and acute kidney injury in severe COVID-19 infection. BMJ Case Rep. (2020) 13:e237616. doi: 10.1136/bcr-2020-237616
89. Tram, N, Chiodini, B, Montesinos, I, Vicinanza, A, Beretta-Piccoli, X, Gubbelmans, N, et al. Rhabdomyolysis and acute kidney injury as leading COVID-19 presentation in an adolescent. Pediatr Infect Dis J. (2020) 39:e314–5. doi: 10.1097/INF.0000000000002853
90. Fujita, K, Kanai, O, Nanba, K, Esaka, N, Hata, H, Seta, K, et al. Acute rhabdomyolysis in a young woman with moderate COVID-19. IDCases. (2021) 25:e01212. doi: 10.1016/j.idcr.2021.e01212
91. Gilpin, S, Byers, M, Byrd, A, Cull, J, Peterson, D, Thomas, B, et al. Rhabdomyolysis as the initial presentation of SARS-CoV-2 in an adolescent. Pediatrics. (2021) 147:273. doi: 10.1542/peds.2020-019273
92. Heidarpour, M, Vakhshoori, M, Haghighatpanah, MA, Ashrafi, L, Khorvash, F, and Iraj, B. Rhabdomyolysis plus hypocalcemia and diabetic ketoacidosis as concurrent rare COVID-19 manifestations. Case Rep Med. (2021) 2021:1–6. doi: 10.1155/2021/6625086
93. Zhang, Q, Shan, KS, Minalyan, A, O'Sullivan, C, and Nace, T. A rare presentation of coronavirus disease 2019 (COVID-19) induced viral myositis with subsequent rhabdomyolysis. Cureus. (2020) 12:8074. doi: 10.7759/cureus.8074
94. Flato, UAP, Ferreira, KV, Biteli, P, Reina, DOB, Reina, FTR, Vernaschi, FT, et al. Rabdomyolysis as a serious complication of COVID-19. AIMS Bioeng. (2021) 8:165–72. doi: 10.3934/bioeng.2021015
95. Taneska, AC, Trajceska, L, Milenkova, M, Kuzmanovski, N, Ristovska, V, Rambabova-Busletikj, I, et al. Rhabdomyolysis and acute kidney injury in a patient with severe form of Covid-19 pneumonia-a case report. BANTAO J. (2020) 2020:82.
96. Farouji, A, Hellou, R, and Peretz, A. Asymptomatic rhabdomyolysis in a young adult with COVID-19. Cureus. (2021) 13. doi: 10.7759/cureus.18039
97. Rosato, C, Bolondi, G, Russo, E, Oliva, A, Scognamiglio, G, Mambelli, E, et al. Clinical, electromyographical, histopathological characteristics of COVID-19 related rhabdomyolysis. SAGE Open Med Case Rep. (2020) 8:2050313X20983132. doi: 10.1177/2050313X20983132
98. Sathappan, A, Wachs, D, and Morgenstein, C. A potential complication of COVID-19: a case of non-traumatic Rhabdomyolisis. J Med Cases. (2020) 11:296–8. doi: 10.14740/jmc3546
99. Shanbhag, A, Manaktala, PS, Rizvi, H, Frey, K, and Narayanan, R. COVID-19 presenting as severe rhabdomyolysis with normal renal function. Cureus. (2020) 12:9556. doi: 10.7759/cureus.9556
100. Singh, B, Kaur, P, Mechineni, A, and Maroules, M. Rhabdomyolysis in COVID-19: report of four cases. Cureus. (2020) 12:10686. doi: 10.7759/cureus.10686
101. Chetram, VK, Ahmad, AI, Farid, S, and Sood, T. Acute kidney injury secondary to rhabdomyolysis and COVID-19: a case report and literature review. Case Rep Nephrol. (2021) 2021:1–8. doi: 10.1155/2021/5528461
102. Mughal, MS, Kaur, IP, Alhashemi, R, Rehman, R, and Du, D. Acute viral myositis complicated by rhabdomyolysis: a sole manifestation of COVID-19 infection. J Community Hosp Intern Med Perspect. (2021) 11:289–91. doi: 10.1080/20009666.2021.1878601
103. Kontou, M, Kakleas, K, Kimioni, V, Georgiadi, D, Spoulou, V, and Michos, A. Rhabdomyolysis and coronavirus disease-2019 in children: a case report and review of the literature. Pediatr Investig. (2022) 6:135–9. doi: 10.1002/ped4.12320
104. Yu, R, Chen, C-R, Evans, D, Qing, X, Gotesman, M, Chandramohan, G, et al. Glucose-6-phosphate dehydrogenase deficiency presenting with rhabdomyolysis in a patient with coronavirus disease 2019 pneumonia: a case report. J Med Case Rep. (2022) 16:1–5. doi: 10.1186/s13256-022-03322-w
105. Donati, G, Abenavoli, C, Vischini, G, Cenacchi, G, Costa, R, Pasquinelli, G, et al. Sickle cell trait and SARS-CoV-2-induced rhabdomyolysis: a case report. Am J Case Rep. (2022) 23:e934220–1. doi: 10.12659/AJCR.934220
106. Buckholz, AP, Kaplan, A, Rosenblatt, RE, and Wan, D. Clinical characteristics, diagnosis, and outcomes of 6 patients with COVID-19 infection and rhabdomyolysis. Mayo Clinic proceedings: Amsterdam, Netherlands: Elsevier (2020).
107. Philippe, C, Misquitta, D, and Niedermier, J. Rhabdomyolysis in young adult male stabilized on mirtazapine and with history of COVID-19 infection. CNS Spectr. (2022) 27:234–5. doi: 10.1017/S1092852922000347
108. Fodili, F, and Van Bommel, E. Severe rhabdomyolysis and acute renal failure following recent Coxsackie B virus infection. Neth J Med. (2003) 61:177–9.
109. Wong, CKH, Wong, JYH, Tang, EHM, Au, CH, and Wai, AKC. Clinical presentations, laboratory and radiological findings, and treatments for 11,028 COVID-19 patients: a systematic review and meta-analysis. Sci Rep. (2020) 10:19765. doi: 10.1038/s41598-020-74988-9
110. Baeza-Trinidad, R. Rhabdomyolysis: a syndrome to be considered. Med Clin (Barc). (2022) 158:277–83. doi: 10.1016/j.medcli.2021.09.025
111. Tajmirriahi, M, Sami, R, Mansourian, M, Khademi, N, Hosseini, N-s, Dehghan, M, et al. The clinical manifestation and outcome of COVID-19 in patients with a history of ischemic heart disease; a retrospective case-control study. BMC Cardiovasc Disord. (2023) 23:32526. doi: 10.1186/s12872-023-03256-1
112. Pormohammad, A, Zarei, M, Ghorbani, S, Mohammadi, M, Razizadeh, MH, Turner, DL, et al. Efficacy and safety of COVID-19 vaccines: a systematic review and meta-analysis of randomized clinical trials. Vaccine. (2021) 9:467. doi: 10.3390/vaccines9050467
113. Pucchio, A, Akiva, MH, Evangeliou, H, Papenburg, J, and Salvadori, MI. Severe rhabdomyolysis secondary to COVID-19 mRNA vaccine in a teenager. Pediatr Nephrol. (2023) 38:1979–83. doi: 10.1007/s00467-022-05808-7
114. Unger, K, Ponte, CD, and Anderson, D. A possible case of COVID-19 booster vaccine–associated rhabdomyolysis and acute kidney injury. J Pharm Technol. (2022) 38:247–50. doi: 10.1177/87551225221093944
115. Ruijters, VJ, van der Meulen, MF, van Es, MA, Smit, T, and Hoogendijk, JE. Rhabdomyolysis after COVID-19 Comirnaty vaccination: a case report. Case Rep Neurol. (2022) 14:429–32. doi: 10.1159/000527599
116. Imhof, C, Messchendorp, AL, Martiniere, H, and Gansevoort, RT. Severe rhabdomyolysis after COVID-19 vaccine. Ann Intern Med. (2022) 1:e220215. doi: 10.7326/aimcc.2022.0215
117. Sheka, M, Coattrenec, Y, Lorenzini, KI, and Nendaz, M. A severe case of rhabdomyolysis after Moderna mRNA anti-COVID-19 vaccine with a literature review. Clin Case Rep. (2023) 11:e7184. doi: 10.1002/ccr3.7184
118. Ajmera, KM. Fatal case of rhabdomyolysis post-COVID-19 vaccine. Infect Drug Resist. (2021) 14:3929–35. doi: 10.2147/IDR.S331362
Keywords: rhabdomyolysis, autoimmunity, COVID-19, coronavirus vaccine, vaccine side effects, vaccine complications
Citation: Karimi M, Faal Hamedanchi N, Ansari K, Nahavandi R, Mazdak M, Javaherchian F, Koochaki P, Asadi Anar M, Shirforoush Sattari M and Mohamaditabar M (2024) Rhabdomyolysis secondary to COVID-19 infection and vaccination: a review of literature. Front. Med. 11:1460676. doi: 10.3389/fmed.2024.1460676
Received: 09 July 2024; Accepted: 06 November 2024;
Published: 20 November 2024.
Edited by:
Diego Ripamonti, Papa Giovanni XXIII Hospital, ItalyReviewed by:
Abdulqadir J. Nashwan, Hamad Medical Corporation, QatarCopyright © 2024 Karimi, Faal Hamedanchi, Ansari, Nahavandi, Mazdak, Javaherchian, Koochaki, Asadi Anar, Shirforoush Sattari and Mohamaditabar. This is an open-access article distributed under the terms of the Creative Commons Attribution License (CC BY). The use, distribution or reproduction in other forums is permitted, provided the original author(s) and the copyright owner(s) are credited and that the original publication in this journal is cited, in accordance with accepted academic practice. No use, distribution or reproduction is permitted which does not comply with these terms.
*Correspondence: Mehdi Karimi, S2FyaW1pOTAxMEBnbWFpbC5jb20=: Mahsa Asadi Anar, bWFoc2EuYm96QGdtYWlsLmNvbQ==
†ORCID: Mehdi Karimi, http://orcid.org/0009-0006-4388-0214
Reza Nahavandi, http://orcid.org/0000-0001-7564-2348
Mahsa Shirforoush Sattari, http://orcid.org/0000-0003-1218-7645
Mona Mohamaditabar, http://orcid.org/0000-0003-1183-3799
Disclaimer: All claims expressed in this article are solely those of the authors and do not necessarily represent those of their affiliated organizations, or those of the publisher, the editors and the reviewers. Any product that may be evaluated in this article or claim that may be made by its manufacturer is not guaranteed or endorsed by the publisher.
Research integrity at Frontiers
Learn more about the work of our research integrity team to safeguard the quality of each article we publish.