- 1Centro de Investigación en Ciencias de la Salud (CICSA), FCS, Universidad Anáhuac México, Huixquilucan, Edo. de México, Mexico
- 2Facultad de Ciencias de la Salud, Universidad Anáhuac México, Huixquilucan, Edo. de México, Mexico
- 3Faculty of Medicine, Autonomous University of the State of Mexico, Toluca, State of Mexico, Mexico
- 4Secretaría de la Defensa Nacional, Escuela Militar de Graduados de Sanidad, Mexico City, Mexico
- 5Neurology Department, Mayo Clinic, Rochester, MN, United States
- 6Kazan State Medical University, Kazan, Russia
- 7School of Sport Sciences, Universidad Anáhuac México, Huixquilucan, Edo de México, Mexico
The case of a 37-year-old woman who suffered from spinal cord infarction (SI), resulting in a complete spinal cord injury (AIS A, neurological level T10), and autonomic dysfunction is presented. This study aimed to assess the effect of transcutaneous Spinal Cord Electrical Stimulation (tSCS) on improving motor, sensory, and autonomic function after SI. During the first 8 months, tSCS was applied alone, then, physical therapy (PT) was included in the sessions (tSCS+PT), until completion of 20 months. Compared to baseline, at 20 months, an increase in ISNCSCI motor (50 vs. 57) and sensory scores (light touch, 72 vs. 82; pinprick, 71 vs. 92) were observed. Neurogenic Bladder Symptoms Score (NBSS) changed from 27 at baseline to 17 at 20 months. ISAFSCI scores in sacral autonomic function improved from 0 pts (absent function) to 1 pt. (altered function) indicating better sphincter control. EMG recordings during volitional movements, including overground stepping with 80% of body weight support showed activity in gluteus medialis, tensor fascia latae, sartorius, rectus femoris, biceps femoris, tibialis anterior, and gastrocnemius medialis, indicating a partial reversion of paralysis. RMS analysis indicated higher activity during “tSCS on” compared to “tSCS off” during overground stepping in bilateral rectus femoris (p < 0.001) and gastrocnemius medialis (p < 0.01); and unilateral biceps femoris, and tibialis anterior (p < 0.001). As this is the first report on the use of tSCS in the case of SI, future studies in a case series are warranted.
1 Introduction
Spinal Cord Stroke or Spinal Cord Infarction (SI) is a rare condition that represents only 0.3%−1% of all vascular neurological conditions (1). An acute disruption of the spinal cord blood supply may result in ischemia and infarction, triggering a wide range of neurological disorders related to the vascular territory affected (2). Cohort studies reported that 45% of SI were related to perioperative procedures (i.e., aortic aneurism surgery and endovascular repair) (3), and 1.8% related to epidural anesthesia (4). Idiopathic SI cases occur in 20%−40% of the cases. In addition, MRI abnormalities are not always found (5). The severity of symptoms at a nadir after SI is related to poor prognosis, including paralysis, bladder catheterization, and pain (3–6). Reports of SI based on MRI analysis have shown anterior spinal cord compromise (ventral horn) as the most common injury pattern, while transverse infarctions are rare. In any case, more than two spinal levels are commonly affected, including in the most severe cases, from thoracic to conus medullaris (3, 7).
Transcutaneous Spinal Cord Electrical Stimulation (tSCS) is a non-invasive neuromodulation strategy aimed at restoring motor, sensory, and autonomic functions after Spinal Cord Injury (SCI) (8). Even though studies with small samples have been published, improvements in motor function (i.e., posture, volitional movements, and reduction in spasticity) have been consistently reported among the participants (9–12). Additionally, improvements in bladder capacity, voiding, and anorectal function have also been reported (13–15). To our knowledge, no studies evaluating the effect of tSCS on functional restoration after SI have been reported to date. This study aimed to evaluate the effects of tSCS on motor, sensory, and autonomic functions in a patient with complete paraplegia (flaccid paralysis, AIS-A) due to SI. Results are presented for 20 months (8 months with tSCS alone and 12 months with tSCS+) Physical therapy (PT) with evaluations every 4 months.
2 Case description
On June 23rd, 2001, the patient was admitted to emergencies after reporting nausea, right iliac fossa pain, anorexia, and fever. After clinical screening, the participant was scheduled for an open appendectomy. Consequently, an epidural block was performed at the T11–T12 level. During the postoperative hours, the patient regained consciousness and complained of loss of sensation, and weakness in both lower limbs, and absence of uresis. After 24 h, symptoms continued to evolve until the patient finally developed paraplegia. The neurological evaluation determined a complete SCI, level T10–T11 for all sensory modalities with muscle strength 0/5. Achillean and patellar reflexes were absent. A Gadolinium-based contrasted MRI (1.5 T) was subsequently performed. The findings included dorsal-lumbar syrinx (T6–T11), multiple cord injuries and epiduritis of chemical or traumatic etiology between T3–T11, and spinal edema from T8 to the medullary cone, probably related to (as indicated by the radiological interpretation) chemical myelitis due to the presence of medication in the arachnoid space, spinal cord ischemia due to vasospasm secondary to drug-induced arachnoiditis or spinal edema secondary to trauma (Figure 1A). Days after the patient was discharged with a diagnosis of SI related to an epidural anesthesia procedure. Since the diagnosis of SI, the patient attended PT sessions (5 days per week) during the first 10 years. The PT program included muscle electrostimulation, trunk exercises and passive mobilization in lower limbs. After this period, the patient gained trunk control and improved transfers. Subsequently, PT was stopped for the following 6 years. Finally, during the last 6 years (except for 2019 and 2020 due to the COVID-19 pandemic), the patient received PT in combination to muscle electrostimulation 3 days per week. None of the previous therapies have led to significant improvements in motor, sensitivity, or autonomic function below T10.
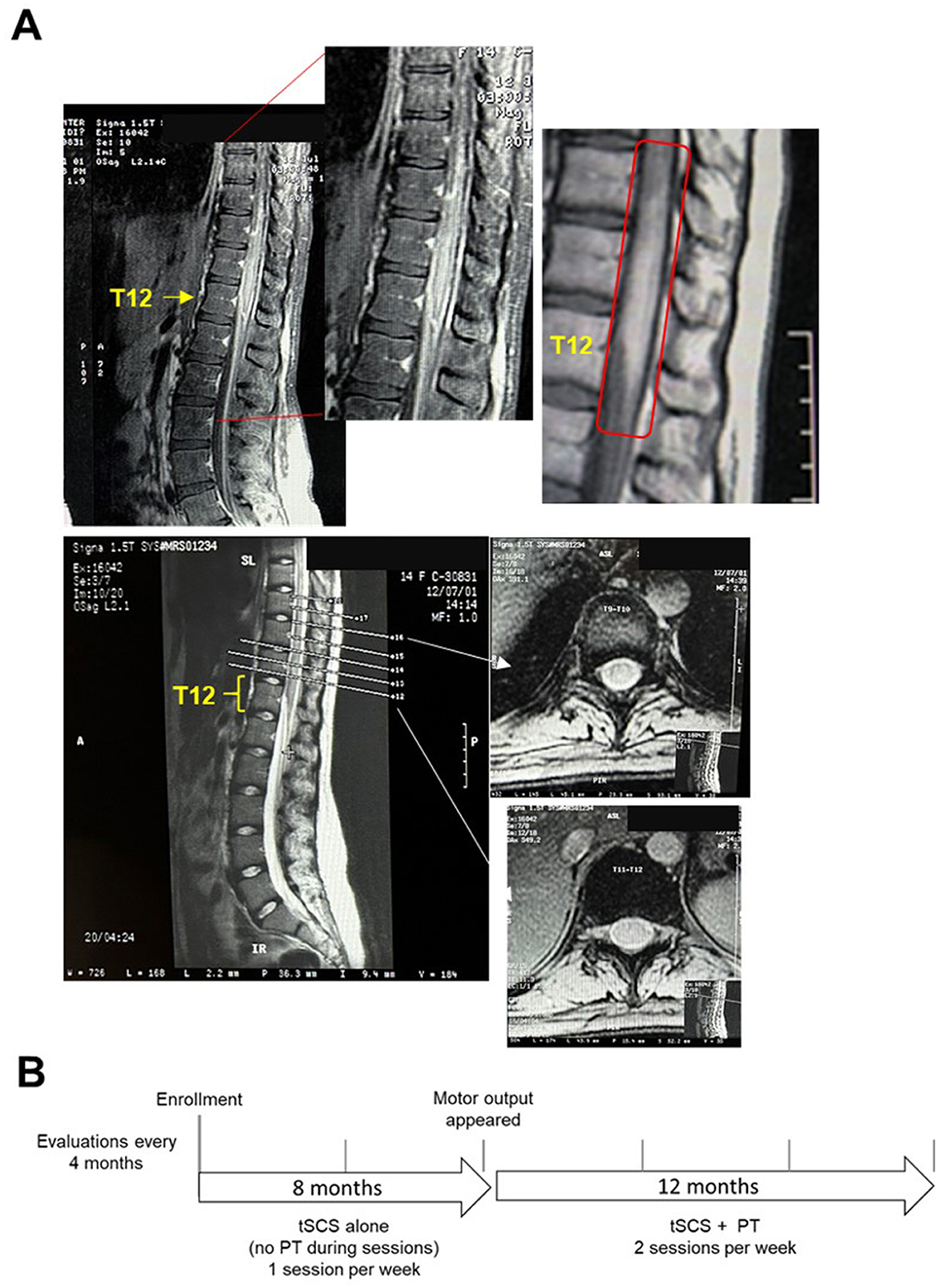
Figure 1. (A) 1.5 T MRI performed after a surgical procedure (2001) revealed an injury in T9–T12 compatible with an SI. The upper panel shows a sagittal slide and a zoomed image on the right corresponding to the lumbar enlargement. The image on the right also shows radiological abnormalities. In the lower panel, coronal slides are shown at T9–10 (upper), and T11–12 (lower) extracted from the image at the left. Findings included epiduritis and edema, which were interpreted by the radiologist. (B) The diagram showing timeline of the protocol. During the first 8 months, tSCS was applied alone, without PT. After motor output appeared around 7 months of tSCS alone, tSCS+PT was included for the following 12 months. Evaluations were applied at baseline and every 4 months.
3 Diagnostic assessment
The patient was enrolled on May, 2022, under the protocol “Evaluation of Transcutaneous Spinal Cord Stimulation on the Autonomic Functions and Quality of Life in Spinal Cord Injured Subjects” approved by the Ethics Committee of the Universidad Anáhuac México (Code 202209). The patient signed the inform consent. During the first 8 months, PT was not included during tSCS sessions due to: (a) the patient was attending PT by her own, and was decided to prevent fatigue, and (b) the primary aim was to improve autonomic and sensory function. Due to lower limb motor improvements around 8 months, tSCS+PT was implemented during sessions the following 12 months (Figure 1B).
The patient (37 years-old, 36 kg, 1.54 cm) presented severe muscle atrophy in lower limbs and reported frequent urinary infections (UI) leading to hospitalizations twice a year. The last UI requiring treatment occurred 1 month before enrollment. For bladder emptying, the patient indicated the use of clean intermittent catheterization. During the 1st week, evaluation of motor and sensory function (ISNCSCI) was performed. The SCI was classified as complete [AIS-A; neurological level (NL), T10]. Right/left motor (Upper Extremity Motor Score, UEMS; Lower Extremities Motor Score, LEMS) and sensory scores (Light Touch, LT and Pinprick, PP) at baseline (BL) are shown in Table 1. The autonomic evaluation (ISAFSCI) resulted in a total score of 11: cardiovascular, thermoregulation core body temperature and broncho-pulmonary system were found normal (2 pts. each), while the sudomotor response was found altered (1 pt.), resulting in hypohidrosis below the NL. The sacral autonomic evaluation indicated absent (0 pts.) or altered (1 pt.) functions in the bladder, bowel, and sexual function.
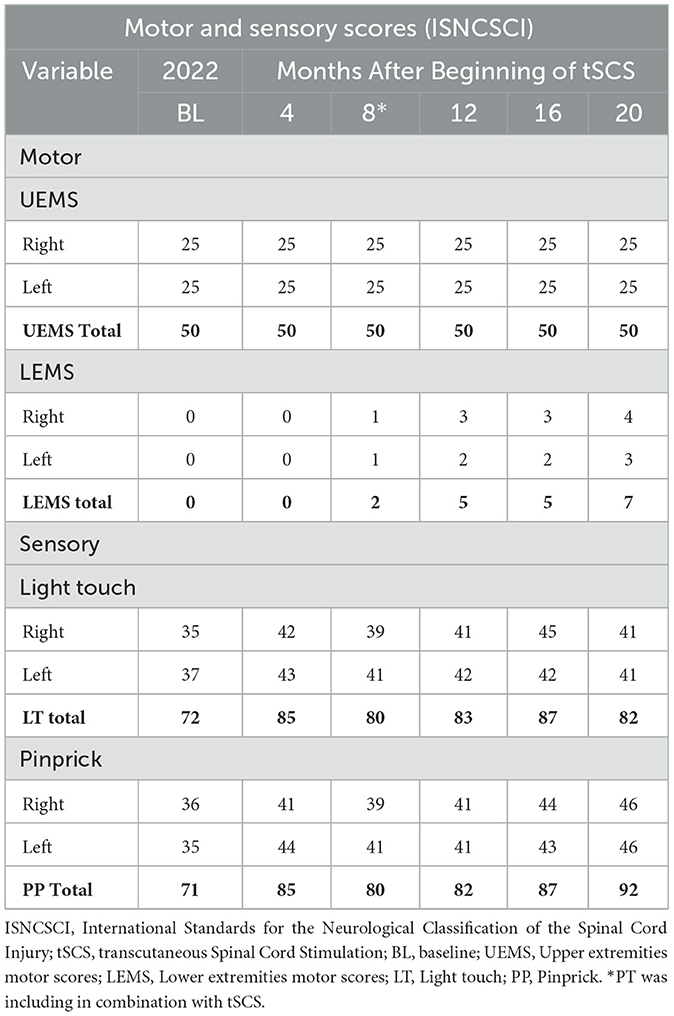
Table 1. ISNCSCI motor and sensory scores at baseline and every 4 months until 20 months completion of the intervention.
Spinally Motor Evoked Potentials (SMEPs) were recorded bilaterally with the patient lying in supine position (10 kHz, LabChart 8, ADInstruments®) in the gluteus medialis (Glu), sartorius (S), vastus medialis (Vm), rectus femoris (RF), biceps femoris (BF), tibialis anterior (TA), and gastrocnemius medialis (Gm) muscles. Biphasic square pulses (500 μs per phase, 0.1 Hz, 1–28 mA, 1 mA steps, DS8R, Digitimer®) were applied by pairs of electrodes (2.5 cm diameter, MedStar) placed at T11–T12 and T12–L1 as cathodes, and electrodes (4 × 8 cm MedStar) placed bilaterally in the iliac crests as anodes. No SMEPs were observed at any muscle at BL. Surface electromyography (EMG) was recorded bilaterally in RF, BF, TA, and Gm during efforts at volitional movements in conjunction with the Jendrassik maneuver during “tSCS off” and “tSCS on” (2 kHz, 20–500 bandpass, LabChart, ADInstruments®). Electrode positioning for EMG recordings are shown in Supplementary material 3. To reduce stimulus-induced artifacts, the following process was carried out: first, two Notch filters were applied, one with a central frequency of 30 Hz and the other at 60 Hz, both with a 6 Hz bandwidth. Then, a bandpass filter was used, with a bandwidth ranging from 10 Hz to 500 Hz. A peak detector with a 60-sample lag was applied to the resulting signal to detect any residual artifact at the stimulation frequency. Once the 30 Hz peaks were identified, 7 points before and 7 points after each peak were removed. Subsequently, the 14 points (7 ms) were replaced with zeros. The signal was then processed using a moving average filter with a 10-point window, and the envelope was extracted. This method was inspired by the approach described in Hofstoetter et al. (16). Finally, the RMS (Root Mean Square) value was calculated for both “tSCS off” and “tSCS on” recordings, adjusting their lengths to match the shortest overground stepping recording, which lasted 17.45 seconds. Artifact removal, RMS and the EMG envelopes were generated in MatLab® (R2023b). Spectral analysis was performed to determine the presence of stimulus artifacts and components of EMG for each muscle.
No EMG activity was detected in any muscle when the patient was instructed to perform hip extension, flexion, adduction (ADD), and abduction (ABD); knee flexion/extension and plantar flexion/extension at BL with “tSCS off” and “tSCS on.” Bilateral H-reflexes evoked at the popliteal fossa (1 ms square pulses, 0.1 Hz, bipolar stimulation, DS8R Digitimer®) and recorded (10 kHz, LabChart, ADInstruments®) at the soleus muscle were absent as well. Due to the lack of information on tSCS on SI, we decided conservatively to start with a single weekly session for 4 months, except for a 2-week period where the patient tested COVID-19 positive. The Neurogenic Bladder Symptoms Score (NBSS), ISNCSCI, ISAFSCI, and SMEPs were evaluated at baseline and every 4 months until completion of 20 months. The set-up for SMEPs (see above) was used for the tSCS protocol.
tSCS has been applied to restore motor function in traumatic SCI. Parameters include stimulation at 30 Hz delivered at T11–T12 and T12-L1. Acute and chronic effects of tSCS have been documented and include improvements in sit-to-standing (10, 17), and voluntary motor activity (18) including stepping (16, 19). Gerasimenko et al. (20) employed tSCS delivered at T11 alone or in combination with Coxigeal stimulation to facilitate rhythmic step-like movements. Subjects were also treated with buspirone in combination with tSCS (20). tSCS aimed to improve autonomic dysfunction was chosen based on previous reports. Phillips et al., applied tSCS at 30 Hz to reduce orthostatic hypotension in four individuals with autonomic dysfunction. A single cathode was placed between T7–T8 spinous process (21). In a case report, Sachdeva et al. (22), delivered tSCS at 30 Hz to ameliorate cardiovascular dysfunction after anorectal stimulation. These authors placed the cathode at the interspinous processes T7/8 (22). In a case series, Kreydin et al., recorded anorectal contractions in acute testing (3 subjects) and chronically in 1 subject during tSCS at 30 Hz (cathode in T11–T12 and L1-2) (15). The same group employed stimulation at 30 Hz (T11–T12, L1-2) during 24 sessions in 8 weeks aimed to reduce neurogenic bladder symptoms and their consequences in 5 subjects with SCI (23). According to the above, pulses at 30 Hz were applied to complete 30 min per session with the patient lying in supine position. Current intensity was selected based on the subject's tolerance and minimal contractions in the abdominal muscles. Current intensities had a range between 15–22 mA. The subject did not experience unpleasant sensations or pain during the stimulation procedure. No responses in any muscle were observed at BL.
3.1 Motor evaluation
No motor responses were found in both legs during the first 7 months of the protocol (0 pts. LEMS Total). Early during the 8th month, when tSCS was still applied without PT, mild hip contractions were noticed. Consequently, LEMS changed from 0 pts. to 2 pts. at 8 months (Table 1). Based on motor improvement, tSCS+PT was implemented during sessions twice a week (Figure 1B). The goal of PT was to train lower limb extension movements (hip and knee extension) and trunk control to enable upright standing and overground stepping with 80% of body weight support (BWS) (Super Grúa, El Abuelo Cómodo®). The progression of PT exercises was divided into phases according to improvements in the subject (Supplementary material 1). Due to sustained motor improvements in hip muscles during the following months (Table 1), postural control and standing and overground stepping with 80% of BWS exercises were included (Supplementary material 1). Motor scores increased 5 pts. (LEMS Total) after 12 months (8 months of tSCS alone and 4 months with tSCS+PT), maintaining the same scores in the next evaluation (8 months tSCS alone and 8 months with tSCS+PT) (Table 1). Motor improvements around this time (12–16 months) led to the participant to perform ABD, ADD, and flexion of the hip during standing with BWS even with “tSCS off.” Rectified EMGs with “tSCS off” and corresponding envelopes in bilateral Glu, RF, S and tensor fasciae latae (TFL) are shown in Figure 2 (Electrode positioning is shown in Supplementary material 3). For each movement, the subject was instructed to perform 5 attempts in each leg, highlighted by the gray bars in Figures 2A, B. Glu and TFL exhibited bursting activity during hip flexion attempts in both legs, while S, and RF did not show activity (Figure 2A). During ABD-ADD attempts in the right leg, right Glu and right TFL showed bursting activity, as well as left Glu and left TFL although with lower amplitude. ABD-ADD attempts on the left leg also showed EMG bursting activity in left Glu, and left TFL, as well as right Glu and right TFL. S and RF on both legs were silent during ABD-ADD attempts (Figure 2B). In the same assessment, a movement consisting of pushing a resistance with both legs (exercise ball) during sitting position also showed EMG bursting activity in bilateral Glu and TFL and also revealed activity in the left S (Figure 2C, gray bar). Next, overground stepping with 80% of BWS was implemented during tSCS+PT sessions. A physiotherapist propelled the BWS system for safety reasons, but no assistance was provided for leg movement (Supplementary Video). EMG was recorded bilaterally in RF, BF, TA, and Gm at 10 months with tSCS+PT and 8 months with tSCS alone. With “tSCS off,” EMG activity appeared in bilateral RF, BF, Gm, and right TA, although no alternation was observed between the legs (Figure 3A). In addition, TA left did not show activity during “tSCS off.” After stimulus artifact removal, during “tSCS on,” an alternating pattern emerged bilaterally in RF, but not in the rest of the recorded muscles (Figure 3B). Gray bars in Figure 3B highlight stepping-like attempts on the right leg (R). As a periodic component persisted after artifact removal in TA left (Supplementary material 4), this muscle was excluded from RMS analysis. Comparison of the RMS with “tSCS on” vs. “tSCS off” during overground stepping showed statistical differences in RF left (p < 0.001), BF left (p < 0.001), GM left (p < 0.01), RF right (p < 0.001), TA right (p < 0.001), and Gm right (p < 0.01). No statistical differences were found in BF right (p > 0.05) (Figure 3C). TA left that was excluded from analysis due to persistent periodic artifact and absence of EMG spectral components (Supplementary material 4). The right panel in Figure 3C compares the percentage of change in RMS and demonstrate higher values during “tSCS on” vs. “tSCS off” in right and left muscles. In left RF and right RF, the difference was more than 1,000%, while in right Gm and left Gm were 202% and 412%, respectively. In the right TA, and left BF, the difference in the RMS was 384% and 329%, respectively. Finally, in right BF the difference was 161% (Figure 3C, right panel). Finally, motor scores at 20 months (8 months with tSCS alone and 12 months with tSCS+PT) increased in the right leg (4), and left leg (3) as shown in Table 1. ISNCSCI motor assessments from BL to 20 months follow-up are shown as Supplementary material 2. Single pulse stimulation delivered at Th12-12, Th12-L1 did not show SMEPS during the intervention. Bilateral H-reflexes recorded at soleus muscles were absent at all time points.
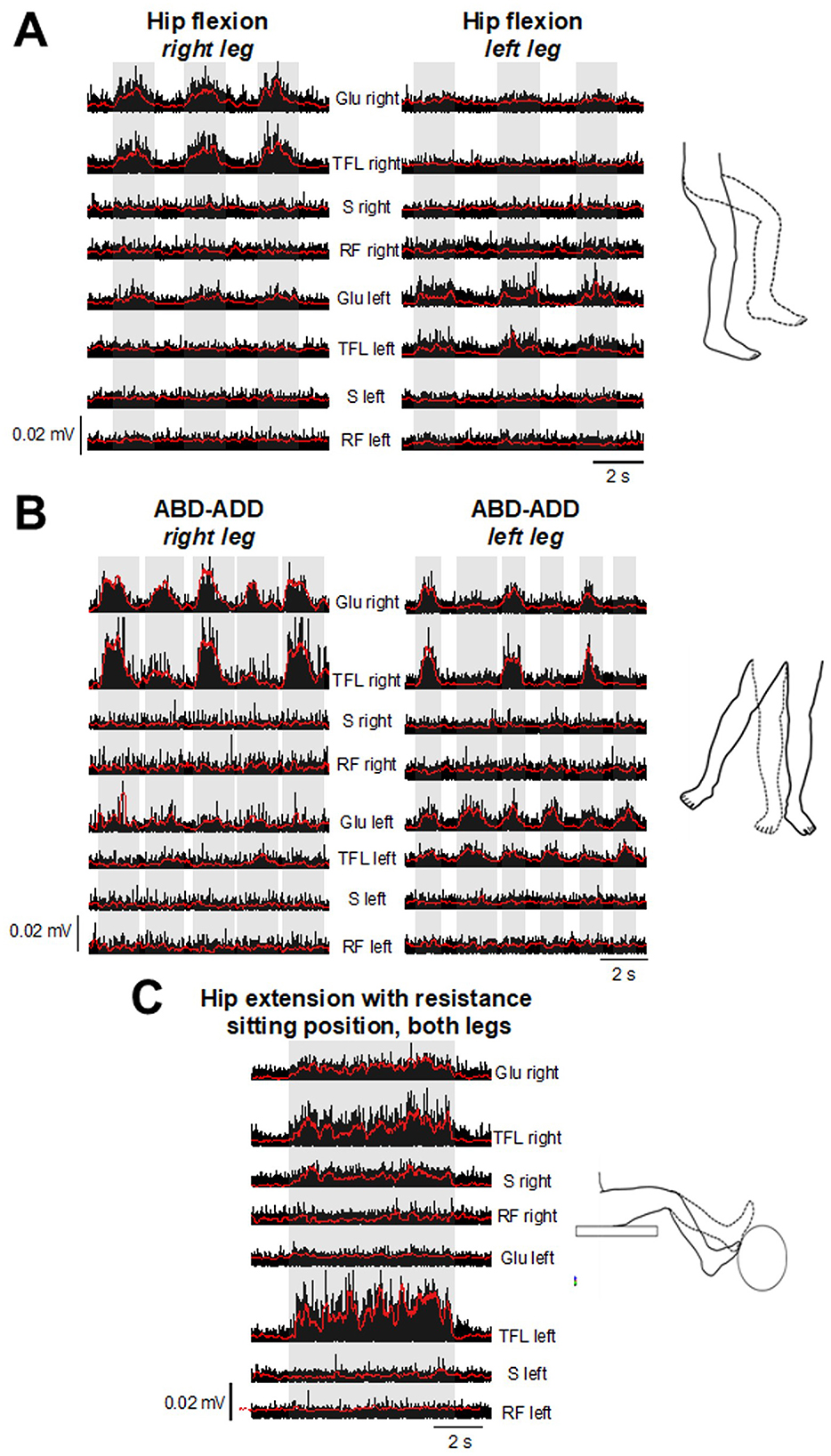
Figure 2. After 6 months of tSCS+PT (14 months from the beginning of the protocol), EMG testing with “tSCS off” showed bursting activity during attempts of hip flexion (A), alternating abduction, and adduction (B) in Glu, and TFL. (C) Slight resistance during hip and knee extension in both legs revealed activation in S. Bilateral RF did not exhibit activity in any exercise. Corresponding EMG envelopes are also shown (red traces). Glu, gluteus medialis; TFL, tensor fascia latae; RF, rectus femoris; S, sartorius.
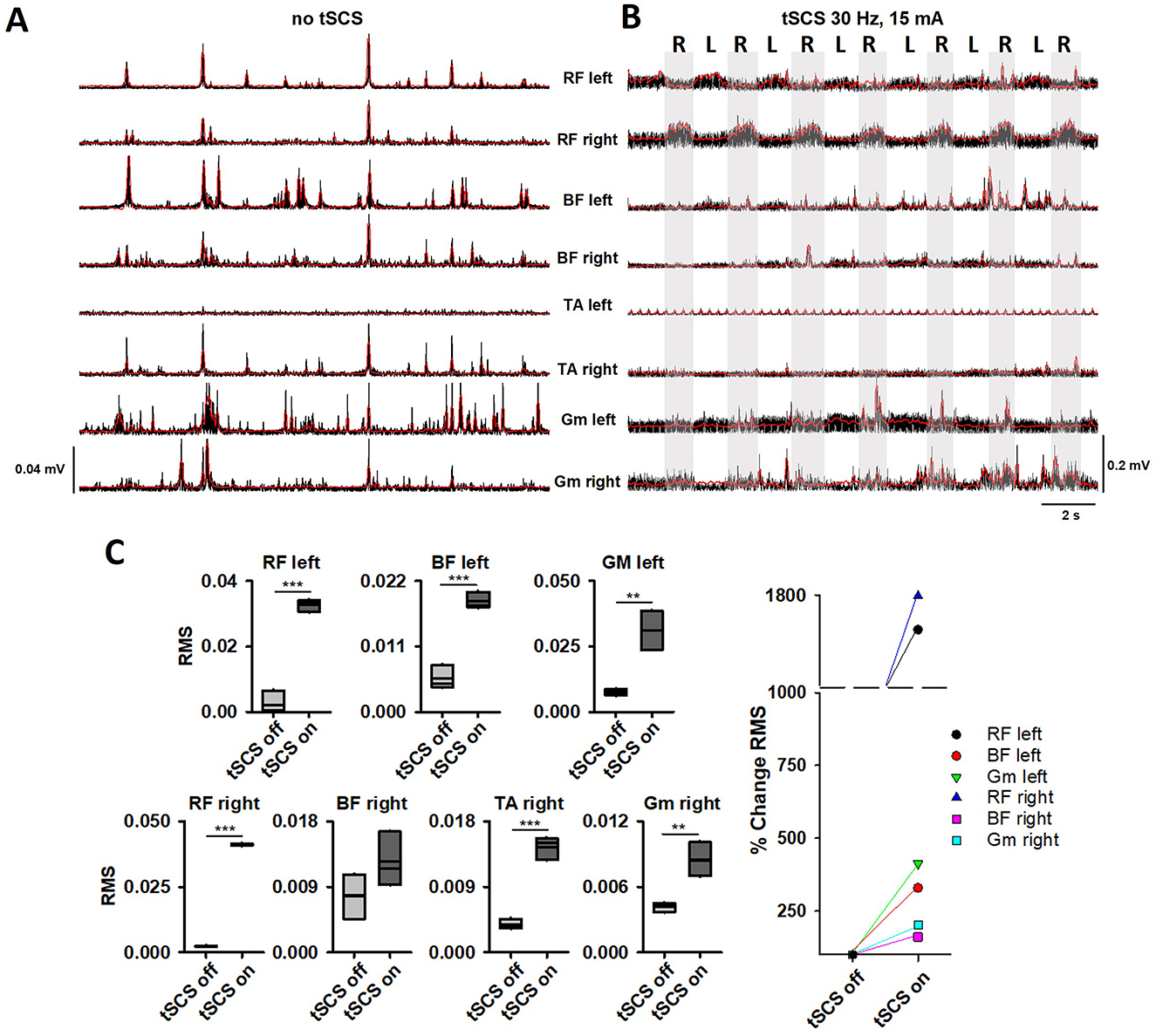
Figure 3. EMG and corresponding envelopes (red traces) during overground stepping with 80% of BWS are shown in bilateral RF, BF, TA, and Gm, with “tSCS off” (no tSCS) (A) and “tSCS on” (30 Hz, 15 mA) (B) at 10 months of tSCS+PT (18 months from the beginning of the protocol). (C) Comparisons of RMS with “tSCS off” and “tSCS on” from recordings in (A, B). Statistically significant difference was found in all tested muscles (**p < 0.01, ***p < 0.001). The percentage of change (%) of the RMS is shown in the right plot. RF, rectus femoris; BF, biceps femoris; TA, tibialis anterior; Gm, gastrocnemius medialis.
3.2 Sensory evaluation
Improvements in sensory function were observed after 4 months of tSCS alone compared to BL, in LT Total (72 pts. vs. 85 pts.), and PP Total (71 pts. vs. 85 pts.). At 8 months, LT sensory scores slightly decreased compared to 4 months: LT Total (80 pts.), PP Total (80 pts.). In the following evaluations (with tSCS+PT), sensory scores continuously improved at the 12th month (LT Total, 83 pts., PP Total, 82 pts.), and 16th month (LT Total, 87 pts., PP Total, 87 pts). The final evaluation in the 20th month revealed a decrease in light touch compared to the previous evaluation (LT Total, 82 pts.), but an increase in PP Total (92 pts.) (Table 1). ISNCSCI sensory assessments from BL to 20 months follow-up are shown as Supplementary material 2.
3.3 Autonomic function evaluation
NBSS total score decreased from 27 (BL) to 17 (20 months), representing a significant clinical change (24). In general, the patient reported an improvement of sphincter control, and continence, eliminating the recurrence of UI. Sacral Autonomic Function (ISAFSCI) changes from BL to 20 months included: improved ability to prevent bladder leakage (0 vs. 1), awareness of bowel fullness (0 vs. 1), and ability to prevent bowel leakage (0 vs. 1).
4 Discussion
The cause of SI can be defined in < 50% of the cases of this condition (1). Partial or total recovery occurs after a few months, depending on the severity of the injury (3–6). In this case report, we present a patient who suffered an SI 23 years ago. Even though trunk control improved after many years with PT, the patient's diagnosis was discouraging as minimal improvements in motor, sensory, or autonomic functions below T11 following the SI occurred. Due to a lack of reports on tSCS in SI, we implemented a conservative, exploratory protocol consisting of one session per week, considering that the participant was attending PT on her own (3 times per week), absence of SMEPs and H reflex, flaccid paralysis, and multiple radiological findings from T3 to conus medullaris (see “Case Report”). To the best of our knowledge, this is the first case of an SI in an AIS-ASIA grade A subject treated with tSCS.
Motor, sensory, and autonomic improvements after traumatic SCI have been reported with tSCS (10–19, 23). tSCS has demonstrated improvement in urinary function with 3 sessions per week during 8 weeks in patients that suffered traumatic SCI (23), and in anorectal function in three subjects and one participant sub-chronically (5 days per week, during 5 weeks) (15). Other studies, including AIS-A and B patients, also demonstrated motor improvement in upper and lower limbs during tSCS applied between 1 to 4 months (12). Although the participant in this case report received fewer sessions per week compared to other studies, our results suggest that even a low number of sessions per week could have a positive impact on a long-term following. After 4 months of tSCS alone, the patient noticed improvements in sensory function, specifically in the hip (L1 dermatome), pelvic floor, and gluteal region (L1, S3). Improvements were more evident after 8 months of tSCS alone and coincided with moderate motor output improvements in hip muscles (Table 1). Consequently, a tSCS+PT program was started, and improvements led to EMG bursting during motor testing, even in the absence of tSCS (Figure 2). Interestingly, muscle activity shown in Figure 2 does not correspond to typical muscle patterns. For example, during right hip flexion (Figure 2A), extensor muscle Glu and weak hip abductor TFL were active, as well as the Glu left. The same pattern was observed during hip flexion in the left leg (Figure 2A), where left Glu, TFL became active, as well as right Glu. Right Glu and TFL were active during ABD-ADD attempts in the right leg. Notably, during ABD-ADD attempts in the left leg, right Glu, TFL were active along with left Glu, TFL (Figure 2B). During movement execution as shown in Figure 2C, right S became active, including right Glu and TFL and left Glu. Although several muscles were tested during the hip abduction/adduction/flexion and extension movements, the muscles shown in Figure 2 were the only muscles active around the 16th month. After overground stepping with 80% of BWS was included as part of tSCS+PT sessions, bilateral RF, BF, and right TA and Gm exhibited activity, although the emerging pattern did not correspond to a typical stepping pattern (Figure 3, Supplementary Video). For example, during steps in the right leg, antagonist RF and BF and TA and Gm were coactive. In the left leg, RF and BF were coactive as well while no activity was observed in the left TA and Gm (Figure 3). During movement attempts, both shown in Figures 2, 3, the subject had difficulties to command the muscles, and considering that some muscles became active after 22 years of paralysis, it is conceivable that a typical motor pattern can be altered not only at spinal cord level but in supraspinal structures. The evaluation of motor pattern execution including supraspinal commands is far beyond the scope of this case report, and will be analyzed in the future. In addition, sustained sensory improvements (light touch and pinprick) were also observed (Table 1).
tSCS aims to activate spinal circuits below the injury level, providing augmentation of the excitability to the cervical (11, 25, 26), lumbar enlargements (16, 17, 27), or both (19). It is possible that changes in autonomic and sensory function during the first 8 months with tSCS alone in addition to PT (3 times per week on her own) contributed to motor improvements observed later. In previous studies, tSCS was applied in cervical or lumbar enlargements, allowing that preserved, but “dormant” circuits can be activated through tSCS (8, 11, 20, 25). In our study, tSCS was applied at T11 to L1, corresponding to the levels affected by the SI (see “Case Report” and Figure 1A). Motor, sensory, and autonomic improvements in the patient may be explained by residual networks that subserved the mechanisms of neural restoration. Animal and human studies with electrical spinal stimulation have described potential mechanisms for spinal cord restoration, including plasticity, re-engaging neurons that lost supraspinal input, generation of new connections [reviewed in (28, 29), and recently, a reduction of excitability driven by a specific subpopulation of neurons that promote stepping recovery have been shown (30)]. These mechanisms seem to be facilitated by locomotor training, engaging residual supraspinal inputs to the spinal cord (31).
In this case report, no H-reflex (and myotatic reflexes below injury level) or SMEPs were observed at any time point. Motor thresholds depend on spinal level stimulation at the lumbar enlargement, according to motor pool distribution (27, 32). Previous reports in healthy subjects describing spatial-temporal characteristics of SMEPs components used tSCS currents up to 100 mA; however, motor thresholds were achieved around 20 mA, stimulating T11–T12 and T12-L1 in tibialis anterior and medial gastrocnemius as shown in (27). In this case report, the maximal intensity applied for SMEPs testing was 28 mA at 0.1 Hz. Current intensities above 20 mA were perceived as unpleasant by the subject, limiting perhaps the appearance of SMEPs in recorded muscles. In this sense, one limitation of this study is that the current intensity applied during tSCS was not selected based on the threshold of motor-evoked responses as reported in previous reports. Interestingly, muscles tested during SMEPs in this study including RF, BF, and GM exhibited bursting activity after 18 months (Figure 3). Emeliannikov et al., found that in injuries at Th10-L2, SMEPs as well as H reflex were absent in 3 out of 10 subjects (33). Similarly, Shapkova et al., did not find H-reflex in 7/19 subjects with and SMEPs were absent in 2 out of 19 participants, although details on AIS, and neurological level of these 2 subjects were not provided (34). The absence of evoked potentials could be explained by several factors (not mutually exclusive): reflex responses (i.e., SMEPs) could be limited by still dormant spinal circuits, partial damage in motor pools, concomitant chronic injury in peripheral nerves and muscles and likely due to cauda equina syndrome (35, 36). Additional studies correlating SI areas (imaging) and muscles showing activity (electrophysiology) will be performed in the following months. Electrophysiological testing (SMEPs, EMG, and H-reflex) will also be carry out in the future.
Bladder and anorectal function improvements with the use of tSCS have been reported (13, 15, 23). Although in this study we did not evaluate objective parameters, the patient reported a reduction in leaks and improvements in continence both in bladder and bowel function, replicating results from those studies. Importantly, the severity and frequency of UI were reduced, particularly for the last 8 months of tSCS+PT. Burning pain in lower limbs was occasionally perceived in some areas of lower limbs. Pain did not appear during or immediately after tSCS sessions, lasted no more than 1 day, and resolved without medication. We may speculate that pain episodes may be related to the following central mechanisms: transient, aberrant connections in the dorsal horn, plastic changes related to dorsal horn neuron spontaneous firing, sensitization due to continuous afferent input (reviewed in West et al. (37)), and a peripheral mechanism related to muscle fatigue (muscle soreness).
The therapeutic mechanisms of tSCS on motor, sensory, and autonomic function after SCI remain elusive, although recent findings have been reviewed (38–40). Findings from animal studies have provided some insights into plastic changes in the nervous system, mainly derived from epidural stimulation (ES). For example, excitatory interneurons located in the intermedia laminae of the spinal cord have shown re-organizing properties relevant for walking recovery (30). ES also promoted a higher count of neural cells and subcellular markers associated to regeneration and recovery in a rodent model of SCI (41). Since the basic mechanisms of tSCS are similar to those of ES (32, 42, 43), tSCS may exhibit comparable properties in restoring lost functions after SCI. In addition, it was recently described that tSCS reduces hyperreflexia, spasticity and enhances motor output in a rodent model of SCI explained by a prevention of chloride imbalance related to cotransporters in the spinal cord (44). Although a clinical case, the results presented here show that the use of tSCS could be explored in other lower motor neuron syndromes, which present symptoms such as hyporeflexia, weakness, and muscle atrophy similar to those exhibited by the subject of this study. Although the classification of lower motor neuron syndromes includes hereditary, immune, and even sporadic patterns (45), patients suffering from these diseases could benefit from tSCS in the future.
5 Conclusion
This is the first report of tSCS used as a therapy to ameliorate motor, sensory, and autonomic dysfunction following SI. Improvements in motor, sensory (light touch and pinprick), and autonomic functions (bowel and bladder) were observed after 22 years of paraplegia with tSCS applied alone (the first 8 months) and with combined tSCS+PT during the following 12 months. No serious adverse events of tSCS were noticed. Bilateral EMG activity were observed, indicating a partial reversion of paralysis.
Data availability statement
The raw data supporting the conclusions of this article will be made available by the authors, without undue reservation.
Ethics statement
The studies involving humans were approved by the Research and Ethics Committee, Universidad Anáhuac México. The studies were conducted in accordance with the local legislation and institutional requirements. The participants provided their written informed consent to participate in this study. Written informed consent was obtained from the individual(s) for the publication of any potentially identifiable images or data included in this article.
Author contributions
FL: Conceptualization, Data curation, Investigation, Supervision, Writing – review & editing. CR: Data curation, Investigation, Writing – review & editing. MA: Data curation, Investigation, Writing – review & editing. GR: Investigation, Writing – review & editing. EM: Investigation, Writing – review & editing. AP-C: Investigation, Writing – review & editing, Formal analysis. JF: Investigation, Writing – review & editing, Resources. AI: Investigation, Resources, Writing – review & editing, Funding acquisition, Project administration, Supervision. IL: Writing – review & editing, Conceptualization, Data curation, Methodology, Validation. CC: Conceptualization, Data curation, Methodology, Validation, Writing – review & editing, Formal analysis, Funding acquisition, Investigation, Project administration, Resources, Supervision, Visualization, Writing – original draft.
Funding
The author(s) declare financial support was received for the research, authorship, and/or publication of this article. This work was supported by the Universidad Anáhuac México.
Acknowledgments
We thank the patient for the resilience and commitment to the protocol. We also thank PT Leticia Manzo Rivera, MD Ana Karen Almada, and Aimee Rebolledo for their assistance during PT sessions. Our special recognition to PT Eliud Piña Eslava, for his interest and following of the patient during our protocol.
Conflict of interest
The authors declare that the research was conducted in the absence of any commercial or financial relationships that could be construed as a potential conflict of interest.
Publisher's note
All claims expressed in this article are solely those of the authors and do not necessarily represent those of their affiliated organizations, or those of the publisher, the editors and the reviewers. Any product that may be evaluated in this article, or claim that may be made by its manufacturer, is not guaranteed or endorsed by the publisher.
Supplementary material
The Supplementary Material for this article can be found online at: https://www.frontiersin.org/articles/10.3389/fmed.2024.1459835/full#supplementary-material
References
1. Romi F, Naess H. Spinal cord infarction in clinical neurology: a review of characteristics and long-term prognosis in comparison to cerebral infarction. Eur Neurol. (2016) 76:95–8. doi: 10.1159/000446700
2. Sliwa JA, Maclean IC. Ischemic myelopathy: a review of spinal vasculature and related clinical syndromes. Arch Phys Med Rehabil. (1992) 73:365–72. doi: 10.1016/0003-9993(92)90011-K
3. Robertson CE, Brown RD, Wijdicks EFM, Rabinstein AA. Recovery after spinal cord infarcts: long-term outcome in 115 patients. Neurology. (2012) 78:114–21. doi: 10.1212/WNL.0b013e31823efc93
4. Nedeltchev K, Loher TJ, Stepper F, Arnold M, Schroth G, Mattle HP, et al. Long-term outcome of acute spinal cord ischemia syndrome. Stroke. (2004) 35:560–5. doi: 10.1161/01.STR.0000111598.78198.EC
5. Rigney L, Cappelen-Smith C, Sebire D, Beran RG, Cordato D. Nontraumatic spinal cord ischaemic syndrome. J Clin Neurosci. (2015) 22:1544–9. doi: 10.1016/j.jocn.2015.03.037
6. Masson C. Spinal cord infarction: clinical and magnetic resonance imaging findings and short term outcome. J Neurol Neurosurg Psychiatry. (2004) 75:1431–5. doi: 10.1136/jnnp.2003.031724
7. Yadav N, Pendharkar H, Kulkarni GB. Spinal cord infarction: clinical and radiological features. J Stroke Cerebrovasc Dis. (2018) 27:2810–21. doi: 10.1016/j.jstrokecerebrovasdis.2018.06.008
8. Gerasimenko Y, Gorodnichev R, Moshonkina T, Sayenko D, Gad P, Reggie Edgerton V. Transcutaneous electrical spinal-cord stimulation in humans. Ann Phys Rehabil Med. (2015) 58:225–31. doi: 10.1016/j.rehab.2015.05.003
9. Hofstoetter US, Freundl B, Danner SM, Krenn MJ, Mayr W, Binder H, et al. Transcutaneous spinal cord stimulation induces temporary attenuation of spasticity in individuals with spinal cord injury. J Neurotrauma. (2020) 37:481–93. doi: 10.1089/neu.2019.6588
10. Alam M, Ling YT, Wong AYL, Zhong H, Edgerton VR, Zheng Y. Reversing 21 years of chronic paralysis via non-invasive spinal cord neuromodulation: a case study. Ann Clin Transl Neurol. (2020) 7:829–38. doi: 10.1002/acn3.51051
11. Inanici F, Brighton LN, Samejima S, Hofstetter CP, Moritz CT. Transcutaneous spinal cord stimulation restores hand and arm function after spinal cord injury. IEEE Trans Neural Syst Rehabil Eng. (2021) 29:310–9. doi: 10.1109/TNSRE.2021.3049133
12. Tefertiller C, Rozwod M, VandeGriend E, Bartelt P, Sevigny M, Smith AC. Transcutaneous electrical spinal cord stimulation to promote recovery in chronic spinal cord injury. Front Rehabil Sci. (2022) 2:740307. doi: 10.3389/fresc.2021.740307
13. Gad PN, Kreydin E, Zhong H, Latack K, Edgerton VR. Non-invasive neuromodulation of spinal cord restores lower urinary tract function after paralysis. Front Neurosci. (2018) 12:432. doi: 10.3389/fnins.2018.00432
14. Gad P, Zhong H, Edgerton VR, Kreydin E. Home-Based SCONE TM therapy improves symptoms of neurogenic bladder. Neurotrauma Rep. (2021) 2:165–8. doi: 10.1089/neur.2020.0061
15. Kreydin E, Zhong H, Lavrov I, Edgerton VR, Gad P. The effect of non-invasive spinal cord stimulation on anorectal function in individuals with spinal cord injury: a case series. Front Neurosci. (2022) 16:816106. doi: 10.3389/fnins.2022.816106
16. Hofstoetter US, Krenn M, Danner SM, Hofer C, Kern H, McKay WB, et al. Augmentation of voluntary locomotor activity by transcutaneous spinal cord stimulation in motor-incomplete spinal cord-injured individuals: augmentation of locomotion by tSCS in incomplete SCI. Artif Organs. (2015) 39:E176–86. doi: 10.1111/aor.12615
17. Sayenko DG, Rath M, Ferguson AR, Burdick JW, Havton LA, Edgerton VR, et al. Self-assisted standing enabled by non-invasive spinal stimulation after spinal cord injury. J Neurotrauma. (2019) 36:1435–50. doi: 10.1089/neu.2018.5956
18. Meyer C, Hofstoetter US, Hubli M, Hassani RH, Rinaldo C, Curt A, et al. Immediate effects of transcutaneous spinal cord stimulation on motor function in chronic, sensorimotor incomplete spinal cord injury. J Clin Med. (2020) 9:3541. doi: 10.3390/jcm9113541
19. Siu R, Brown EH, Mesbah S, Gonnelli F, Pisolkar T, Edgerton VR, et al. Novel noninvasive spinal neuromodulation strategy facilitates recovery of stepping after motor complete paraplegia. J Clin Med. (2022) 11:3670. doi: 10.3390/jcm11133670
20. Gerasimenko YP, Lu DC, Modaber M, Zdunowski S, Gad P, Sayenko DG, et al. Noninvasive reactivation of motor descending control after paralysis. J Neurotrauma. (2015) 32:1968–80. doi: 10.1089/neu.2015.4008
21. Phillips AA, Squair JW, Sayenko DG, Edgerton VR, Gerasimenko Y, Krassioukov AV. An autonomic neuroprosthesis: noninvasive electrical spinal cord stimulation restores autonomic cardiovascular function in individuals with spinal cord injury. J Neurotrauma. (2018) 35:446–51. doi: 10.1089/neu.2017.5082
22. Sachdeva R, Nightingale TE, Pawar K, Kalimullina T, Mesa A, Marwaha A, et al. Noninvasive neuroprosthesis promotes cardiovascular recovery after spinal cord injury. Neurotherapeutics. (2021) 18:1244–56. doi: 10.1007/s13311-021-01034-5
23. Kreydin E, Zhong H, Latack K, Ye S, Edgerton VR, Gad P. Transcutaneous electrical spinal cord neuromodulator (TESCoN) improves symptoms of overactive bladder. Front Syst Neurosci. (2020) 14:1. doi: 10.3389/fnsys.2020.00001
24. Welk B, Lenherr S, Elliott S, Stoffel J, Presson AP, Zhang C, et al. The Neurogenic Bladder Symptom Score (NBSS): a secondary assessment of its validity, reliability among people with a spinal cord injury. Spinal Cord. (2018) 56:259–64. doi: 10.1038/s41393-017-0028-0
25. Gad P, Lee S, Terrafranca N, Zhong H, Turner A, Gerasimenko Y, et al. Non-invasive activation of cervical spinal networks after severe paralysis. J Neurotrauma. (2018) 35:2145–58. doi: 10.1089/neu.2017.5461
26. Barss TS, Parhizi B, Mushahwar VK. Transcutaneous spinal cord stimulation of the cervical cord modulates lumbar networks. J Neurophysiol. (2020) 123:158–66. doi: 10.1152/jn.00433.2019
27. Sayenko DG, Atkinson DA, Dy CJ, Gurley KM, Smith VL, Angeli C, et al. Spinal segment-specific transcutaneous stimulation differentially shapes activation pattern among motor pools in humans. J Appl Physiol. (2015) 118:1364–74. doi: 10.1152/japplphysiol.01128.2014
28. Samejima S, Henderson R, Pradarelli J, Mondello SE, Moritz CT. Activity-dependent plasticity and spinal cord stimulation for motor recovery following spinal cord injury. Exp Neurol. (2022) 357:114178. doi: 10.1016/j.expneurol.2022.114178
29. Martin JH. Neuroplasticity of spinal cord injury and repair. In: Handbook of Clinical Neurology. Elsevier (2022). p. 317–330. doi: 10.1016/B978-0-12-819410-2.00017-5
30. Kathe C, Skinnider MA, Hutson TH, Regazzi N, Gautier M, Demesmaeker R, et al. The neurons that restore walking after paralysis. Nature. (2022) 611:540–7. doi: 10.1038/s41586-022-05385-7
31. Jo HJ, Perez MA. Corticospinal-motor neuronal plasticity promotes exercise-mediated recovery in humans with spinal cord injury. Brain. (2020) 143:1368–82. doi: 10.1093/brain/awaa052
32. Hofstoetter US, Freundl B, Binder H, Minassian K. Common neural structures activated by epidural and transcutaneous lumbar spinal cord stimulation: elicitation of posterior root-muscle reflexes. PLoS ONE. (2018) 13:e0192013. doi: 10.1371/journal.pone.0192013
33. Emeliannikov DV, Shapkova EY, Moshonkina TR, Gerasimenko YP. Evaluation of lumbar enlargement motor neuron excitability: comparison between h-reflex and transcutaneous spinal cord stimulation. Hum Physiol. (2016) 42:258–261. doi: 10.1134/S0362119716030075
34. Shapkova EY, Pismennaya EV, Emelyannikov DV, Ivanenko Y. Exoskeleton walk training in paralyzed individuals benefits from transcutaneous lumbar cord tonic electrical stimulation. Front Neurosci. (2020) 14:416. doi: 10.3389/fnins.2020.00416
35. Misulis KE, Zimmerman EE. Spinal Cord. In: Neurologic Localization and Diagnosis. Elsevier (2023). p. 107–117. doi: 10.1016/B978-0-323-81280-1.00017-2
36. Tyger R. Spine. In: Orthopaedics for Physician Assistants. Elsevier (2022). p. 1–38. doi: 10.1016/B978-0-323-70984-2.00001-6
37. West SJ, Bannister K, Dickenson AH, Bennett DL. Circuitry and plasticity of the dorsal horn – toward a better understanding of neuropathic pain. Neuroscience. (2015) 300:254–75. doi: 10.1016/j.neuroscience.2015.05.020
38. Laskin JJ, Waheed Z, Thorogood NP, Nightingale TE, Noonan VK. Spinal cord stimulation research in the restoration of motor, sensory, and autonomic function for individuals living with spinal cord injuries: a scoping review. Arch Phys Med Rehabil. (2022) 103:1387–97. doi: 10.1016/j.apmr.2022.01.161
39. Lin A, Shaaya E, Calvert JS, Parker SR, Borton DA, Fridley JS. A review of functional restoration from spinal cord stimulation in patients with spinal cord injury. Neurospine. (2022) 19:703–34. doi: 10.14245/ns.2244652.326
40. Ievins A, Moritz CT. Therapeutic stimulation for restoration of function after spinal cord injury. Physiology. (2017) 32:391–8. doi: 10.1152/physiol.00010.2017
41. Angelin LG, Carreño MNP, Otoch JP, De Resende JCF, Arévalo A, Motta-Teixeira LC, et al. Regeneration and plasticity induced by epidural stimulation in a rodent model of spinal cord injury. Int J Mol Sci. (2024) 25:9043. doi: 10.3390/ijms25169043
42. Calvert JS, Gill ML, Linde MB, Veith DD, Thoreson AR, Lopez C, et al. Voluntary modulation of evoked responses generated by epidural and transcutaneous spinal stimulation in humans with spinal cord injury. J Clin Med. (2021) 10:4898. doi: 10.3390/jcm10214898
43. Mayr W, Krenn M, Dimitrijevic MR. Epidural and transcutaneous spinal electrical stimulation for restoration of movement after incomplete and complete spinal cord injury. Curr Opin Neurol. (2016) 29:721–6. doi: 10.1097/WCO.0000000000000382
44. Malloy DC, Côté M-P. Multi-session transcutaneous spinal cord stimulation prevents chloride homeostasis imbalance and the development of hyperreflexia after spinal cord injury in rat. Exp Neurol. (2024) 376:114754. doi: 10.1016/j.expneurol.2024.114754
Keywords: spinal cord infarction, spinal cord injury, transcutaneous spinal cord stimulation, non-invasive neuromodulation, spinal cord stroke, paraplegia, physical therapy
Citation: León F, Rojas C, Aliseda MJ, del Río G, Monzalvo E, Pliego-Carrillo A, Figueroa J, Ibarra A, Lavrov I and Cuellar CA (2024) Case report: Combined transcutaneous spinal cord stimulation and physical therapy on recovery of neurological function after spinal cord infarction. Front. Med. 11:1459835. doi: 10.3389/fmed.2024.1459835
Received: 04 July 2024; Accepted: 14 October 2024;
Published: 06 November 2024.
Edited by:
Simon M. Danner, Drexel University, United StatesReviewed by:
Claudia A. Angeli, Kessler Foundation, United StatesKaren Minassian, Medical University of Vienna, Austria
Copyright © 2024 León, Rojas, Aliseda, del Río, Monzalvo, Pliego-Carrillo, Figueroa, Ibarra, Lavrov and Cuellar. This is an open-access article distributed under the terms of the Creative Commons Attribution License (CC BY). The use, distribution or reproduction in other forums is permitted, provided the original author(s) and the copyright owner(s) are credited and that the original publication in this journal is cited, in accordance with accepted academic practice. No use, distribution or reproduction is permitted which does not comply with these terms.
*Correspondence: Carlos A. Cuellar, Y2FybG9zLmN1ZWxsYXJyYSYjeDAwMDQwO2FuYWh1YWMubXg=