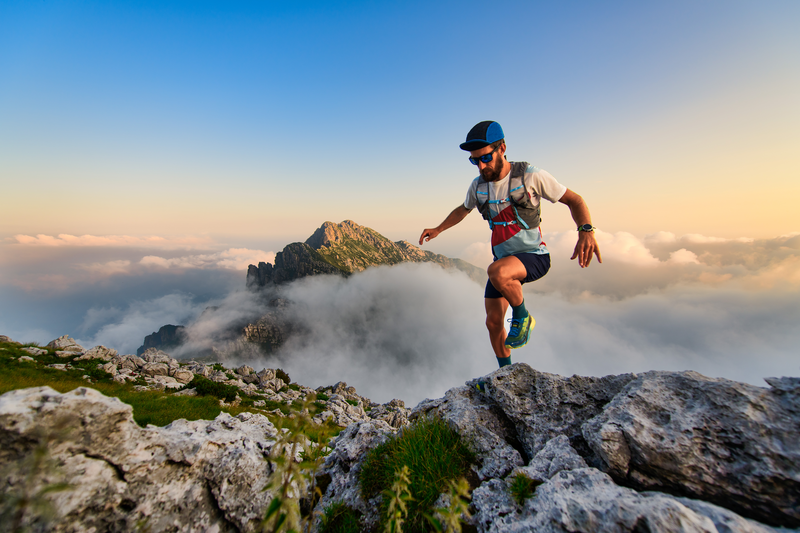
95% of researchers rate our articles as excellent or good
Learn more about the work of our research integrity team to safeguard the quality of each article we publish.
Find out more
SYSTEMATIC REVIEW article
Front. Med. , 13 September 2024
Sec. Geriatric Medicine
Volume 11 - 2024 | https://doi.org/10.3389/fmed.2024.1451180
This article is part of the Research Topic Motor Interventions: Balance and Cognition in Older Individuals View all 5 articles
Introduction: Balance is a multifactorial construct with high relevance in, e.g., everyday life activities. Apart from sensorimotor control, muscle strength and size are positively linked with balance performance. While commonly trained for via resistance training, stretch training has emerged as a potential substitution in specific conditions. However, no review has investigated potential effects of stretching on balance, yet.
Methods: PubMed, Web of Science and Scopus were searched with inception to February, 2024. Studies were included if they examined acute and/or chronic effects of any stretching type against passive and/or active controls on balance parameters – without any population-related restrictions concerning sex/gender, age, health status, activity level. Methodological quality was assessed using PEDro scale. Meta-analyses were performed if two or more studies reported on the same outcome. Certainty of evidence was determined based on GRADE criteria.
Results: Eighteen acute and eleven chronic effect studies were included. Stretching studies exhibited significant improvements for sway parameters with eyes open against passive controls of moderate magnitude for chronic (ES: 0.63, p = 0.047) and of small magnitude for acute studies (ES: 0.21, p = 0.032). Most other subgroups against passive controls as well as actively-controlled comparisons resulted in trivial and/or non-significant effects.
Conclusion: Even though some pooled effects slightly reached the level of significance, the overall results are biased by (very) low certainty of evidence (GRADE criteria downgrading for risk of bias, imprecision, publication bias). Moderators suggested by literature (strength, muscle size, flexibility, proprioception) were rarely assessed, which prevents conclusive final statements and calls for further, high quality evidence to clarify potential mechanisms–if any exist.
The ability to restore and maintain postural control during upright standing or gait is of paramount importance to master everyday life activities in all age groups, however, especially in older adults and (orthopedic) patients (1). Considering balance a multifactorial construct, the literature indicates parameters such as sensorimotor control and muscle strength to be positively linked with balance performance (2, 3). While sensorimotor function was frequently triggered by performing a variety of exercises on unstable surfaces (4) or increasing complexity in the exercise execution, strength capacity is commonly enhanced via resistance training (5).
In order to maintain or prevent loss of motor function until older age there is growing interest to develop effective balance exercise routines. The demographic change toward an aging population poses several challenges on many societies with one in particular revolving around the enhanced need for medical care and nursing. Specifically, people above the age of 65 years (yrs.) are at particular risk for falls: One third of those as well as half of people >80 yrs. olds fall at least once a year (6). While falls are first and foremost a leading cause of fatal and non-fatal injuries (6, 7) that entail substantial medical costs (8), the fear of falling alone is associated with activity restrictions and may thus be an important contributor diminishing the quality of life of community-dwelling older adults (9). Several previous works were performed to determine moderators of balance, outlining, for instance, limited physical activity as a predictor of reduced balance (10). It is well known that sedentary behavior and reduced physical activity is closely related to impaired muscular performance and sarcopenia (11, 12), making the link between maximal strength and balance performance not surprising.
The relevance of reaching high force output in the lower extremity was underlined by Cattagni et al. (13) who described strength capacity as a discriminator between fallers and non-fallers. Accordingly, the American Geriatrics Society and American Academy of Orthopedic Surgeons panel on fall prevention listed muscular weakness and muscular function impairments as the most important risk factor for falls in older adults (14) and emphasized the demand for strength-increasing exercise routines since muscle weakness – including muscle size (15), strength (16) and flexibility (17) – moderates postural control. Consequently, the current 2022 “World guidelines for falls prevention and management for older adults” (18) strongly recommend the use of individualized progressive resistance training to reduce the risk of falls, while Chang et al. (19) recently reported further increases in balance ability when strength training is combined with acupuncture interventions.
However, to ensure a safe movement execution, meaning avoidance of adverse events while exercising, and to increase training effects especially in untrained and/or unexperienced populations, training supervision is strongly recommended (20). This requirement imposes severe burdens on conditioned and older participants, thereby restricting access to prevention but also rehabilitation programs, which is considered a major contributor to muscle function and size loss (21). Consequently, there seems to be high demand for balance exercise routines that forgo the need for travel and supervision, being time- and location-independent in its application, to improve exercise commitment and adherence rates.
Recent literature raised the possibility of interchangeable use of resistance training and high volume stretching under special circumstances: While full range of motion resistance training provided comparable flexibility increases (22), high volume and intensive stretching significantly increased strength and hypertrophy (23–25). Even though the required exercise durations to induce meaningful strength and muscle size adaptations seem time-consuming for stretching interventions, Behm et al. (26) proposed its usage as a relevant resistance training alternative when integrated into daily activities such as watching television or working in the office.
While recent literature discusses the potential of replacing strength training with high-volume stretching when aiming to increase muscle strength and size (27, 28), to date, no review has examined whether stretching has acute or chronic effects on balance. Thus, the objective of this systematic review with meta-analysis was to quantify the overall available evidence for the implementation of stretching to improve balance since the literature is too scarce for population-specific calculations – and thereby explore a possible new perspective for training and therapy.
The authors conducted the systematic review following the “Reporting Items for Systematic Reviews and Meta-Analyses 2020” (PRISMA 2020) guidelines (29) and opted to register the review in the PROSPERO database which, however, was rejected following the automatic processing with possible reasons given of which either one or more applied to this review: (a) review appears to be a scoping review, (b) review revolves around sporting performance, (c) review has insufficient information in fields (usually a lack of information on data extraction, risk of bias or data synthesis methods), (d) information was submitted in non-English language.
The search term was designed under consideration of the PICOS (Patient/Population, Intervention, Comparison, Outcomes, Study design) guidelines (30). The following eligibility criteria were applied to the literature search:
1. Randomized and non-randomized studies investigating any type of stretching meeting the definition criteria listed below in comparison to a passive (non-intervened) or active (all other types of exercise such as balance training, cycling, yoga or manual therapy) control condition.
2. Studies which investigated acute (immediately following an intervention) or chronic (long-term interventions of at least two weeks of stretching with a minimum of one session per week) stretching effects, including the most common stretching types [static, dynamic, ballistic and proprioceptive neuromuscular facilitation (PNF)] (31–33).
3. Works that quantified static and dynamic balance performance outcomes via, on the one hand Y-balance reach or the star excursion test, and on the other hand, center of pressure (COP) sway measurements.
4. Studies that recruited both healthy participants and/or patients, while no restrictions were applied regarding the target population concerning sex/gender, age, health status and activity/athletic status.
Studies were excluded for the following reasons:
a. using combined interventions (stretching plus other exercise interventions except for warm-up through jogging or stationary bike),
b. having uncontrolled study designs,
c. lacking pre-post comparisons,
d. investigating parameters on an ordinal scale [e.g., the Berg-Balance-Scale, see Lima et al. (34)],
e. being of such low quality that vital aspects of the study design cannot be identified (e.g., lack of specificity regarding load control) and/or
f. not being published in English-speaking, peer-reviewed journals.
The search strategy was developed based on the aforementioned eligibility criteria and applied to the three databases MEDLINE/PubMed, Web of Science, and Scopus (inception to February 2024) which was supplemented by manual search of the first 500 Google Scholar results as well as snowballing citation searching. The search terms were created based on individual database requirements, e.g., for PubMed:
(stretch*[Title/Abstract]) AND (balance[Title/Abstract] OR “postural control”[Title/Abstract] OR stability[Title/Abstract] OR proprioception[Title/Abstract]) NOT (pilates[Title/Abstract] OR dance[Title/Abstract] OR “stretch-shortening”[Title/Abstract])
The search strings for Web of Science and Scopus are listed in the Supplementary material. The databases were searched until 3 March 2024.
While there are several stretching definitions with broad extensions (22), the following definitions for static stretching, dynamic stretching and PNF were used to differentiate stretching from other interventions. In accordance with Behm (35) static stretching was defined as the lengthening of a muscle until stretch sensation/the point of discomfort and holding the muscle in a lengthened position, which can be performed passively by external devices, a partner or external weight, or actively by active movements. PNF is a stretching technique that incorporates a maximal voluntary contraction to a static stretching bout with or without antagonist contraction (contract-relax or contract-relax-antagonist-contract) (35). Interventions were considered dynamic stretching (36) if the exercise was performed as controlled back and forth movements in the end ROM, with ballistic stretching assumed a subcategory of dynamic stretching including less controlled, bouncing movements in the end ROM. Another specific subcategory of dynamic stretching was cyclic stretching, if participants performed constant velocity/angle stretching via a computerized system (37). All interventions that fit one of the aforementioned definitions were eligible for inclusion in this review.
PEDro rating was performed by two investigators (LHL & MO) in accordance with official guidelines (see Supplementary material) to assess the risk of bias (38). If no consensus was reached, a third author (KW) had the decisive vote. Additionally, risk of publication bias (39) was assessed by visual inspection of modified funnel plots, which was supplemented by the Egger’s regression test (40, 41).
Study selection and data extraction were performed by GP, MO and DJ, and consequently double-checked by LHL and KW. Each record was screened by three reviewers independently. Data was extracted from the original studies into an Excel file (Microsoft 365, Microsoft Corp., Redmond, WA, USA) using a dual control principle via screen sharing. If a study did not contain means (M) and standard deviations (SD) in writing or in graphic illustrations, data were requested via e-mail or ResearchGate from the corresponding author of the respective study. If neither the corresponding author responded, nor there was another possibility to exactly determine M and SD, the study was excluded. Effect sizes (ES) were calculated based on MDiff from pre-to post-test by applying
while pooled SDs were determined by
Accounting for multiple study (multiple outcomes of the same balance test, or different balance tests, or different stretching interventions tested against the same control) outcomes with co-variance originating from unknown sources, the robust variance estimation (RVE) meta-analysis calculation model (42) was used to pool the standardized mean differences (SMDs) and 95% confidence intervals (CIs) for acute and chronic stretching effects on balance. While comparisons with passive controls may indicate a general effectivity of the intervention, the attribution of potential effects to the specific intervention would require superior effects compared to other interventions (43). Thus, acute as well as chronic stretching effects were also compared to active, alternative control conditions. Due to the highly specific nature of balance tasks and subsequently differences that each task places on the underlying abilities needed, separate analyses were performed based on the test used to obtain the balance outcomes. Therefore, we differentiated 3 subgroups as (1) combining Y-Balance test (YBT), Star Excursion (SEBT) (and Forward reach test (FRT)), (2) sway/COP eyes open, and (3) sway/COP eyes closed. The rationale for dividing the analyses into static (sway/COP open eyes, sway/COP closed eyes) and dynamic (YBT/SEBT/FRT) balance lies in the task specificity of tests and different underlying control mechanisms such as the involvement of the neuromuscular system (44, 45). While YBT/SEBT(/FRT) demand control of the body’s center of gravity during dynamic, one-legged movement/reach, sway/COP tests quantify the extent of sway during static standing. Hereby, open-eyes and closed-eyes conditions were distinguished due to the known impact of visual feedback on static standing balance (46) that increases reliance on vestibular and proprioceptive input during eyes-closed balance performance (47). Improving postural control is of particular interest in people with functional limitations such as in older adults and patients with chronic diseases.
If possible, the analyses were further refined for stretching types (static, dynamic, PNF). To further reduce intra-study heterogeneity, stability index outcomes were removed for sensitivity analyses.
Outcome heterogeneity was assessed with τ2 and categorized in accordance to pooled effect sizes (ES), interpreted as follows: trivial: 0 ≤ ES < 0.2; small: 0.2 ≤ ES < 0.5; moderate: 0.5 ≤ ES < 0.8; and large: ES ≥ 0.8 (48). All calculations were performed using R (version 4.2.3) with the robumeta and meta package (42) under special consideration of the study design (parallel and cross-over design).
The certainty of evidence was rated adhering to the GRADE working group criteria with categorizations as “very low” (effect estimate very uncertain), “low” (further research is very likely to change the effect estimate), “moderate” (further research is likely to change the effect estimate), or “high” (further research is very unlikely to change the effect estimate) (49). Accordingly, certainty is downgraded for risk of bias (limitations in study design and execution, e.g., lacking allocation concealment or blinding of subjects and/or investigators), inconsistency of results (unexplained heterogeneity of results assessed, e.g., via τ2), indirectness of evidence (evidence stems from research that does not directly compare the interventions of interest, is not delivered to the populations of interest, and/or does not measure the outcomes of interest), imprecise data (generally, if studies include only few participants and events and have a wide CI around the estimate of the effect, e.g., when the 95%CI overlaps no effect and CI fails to exclude important harm or benefit), and publication bias (systematic over- or underestimation due to selective publication of studies assessed, e.g., via Egger’s regression and/or funnel plots), while strong evidence of association (large or very large magnitude of effect with good precision regarding the CI – see above), evidence of a dose–response gradient, and plausible confounders (confounding is expected to have influenced the result in a way that the effect is even higher when adjusted for the confounders, e.g., when confounding is expected to have reduced a demonstrated (large magnitude) effect) enabled an upgrade.
The flow chart (Figure 1) illustrates the literature search, that resulted in a total of 29 (50–78) studies of which 18 (50, 52–59, 61, 63, 65, 67, 68, 71, 73–75) investigated acute and eleven (51, 60, 62, 64, 66, 69, 70, 72, 76–78) chronic effects. Fifteen acute effect studies compared stretching to a passive control while four opposed stretching to an active control. For chronic effects, seven studies compared stretching groups to passive and five studies to active control groups (see Figure 2). More detailed study descriptions can be found in Table 1.
With a PEDro score (M ± SD) of 4.2 ± 1.8 out of 10 (range: 1 to 8 points), risk of bias was rated as fair. When differentiating for acute and chronic studies, the average score is 3.5 and 5.4, respectively, indicating that acute studies had a lower quality. While the classification fair fits the chronic studies’ quality assessment, the acute studies’ overall quality must be rated as poor. Overall, all studies provided both point measures and measures of variability (29/29), while almost all studies reported statistical between-group comparisons (26/29) and random group (for parallel group designs) or intervention sequence (for cross-over designs) allocation (22/29). While blinding of the participants and therapists was never achieved, investigator-blinding was performed in seven studies. Only seven studies concealed the allocation and only five studies adhered to the application of the intention-to-treat principle. In 13 out of 29 studies the groups were not considered similar at baseline regarding the most important prognostic factors. Only ten studies specifically stated both, the number of subjects in both pre- and post-testing and collected data from at least 85% of the initially included subjects (see Appendix Table 1).
Seven studies investigated chronic effects of stretching compared to passive controls, whereby one study (70) incorporated both passive and active controls. For the passive control comparisons, six studies (51, 60, 62, 66, 69, 70) incorporated young adults (18–65 yrs. of age). There was only one study (78) that investigated the chronic effects of stretching on older adults’ balance performance, who performed stretching for eight weeks, thrice per week with 150 s of stretch per session and found no change. Furthermore, two out of the seven studies investigated patients post ankle sprain (51) or with multiple sclerosis (70). Sensitivity analysis excluding the patient populations (due to the possibility of different neuromuscular responses for interventions in patients settings (79, 80)) resulted in non-significant results (p = 0.094–0.241).
Out of the seven studies, four investigated static standing sway/COP parameters with eyes open of which three (60, 66, 69) found a positive effect, one (70) no change and one (69) a negative effect. Hereby, Todde et al. (69) found both a large increase and large decrease for two different subgroups following different stretching interventions. Effect size pooling exhibit a moderate magnitude improvement (ES: 0.63 95%CI 0.02–1.24, p = 0.047, τ2 = 0.12) (see Figure 3). Sensitivity analysis (excluding stability index outcomes to account for the possible impact of an index ceiling effect (81–84)) resulted in small magnitude effects, however, non-significant for the same outcomes (ES: 0.44 95%CI -0.18–1.06, p = 0.091, τ2 = 0) (see Table 2).
Figure 3. Forest plot for chronic stretching effects on sway balance against passive control conditions.
Two of the studies also investigated eyes-closed conditions that both (60, 70) found no significant change. As a consequence, the quantitative analysis reveals no changes (ES: -0.02 95%CI -0.96–0.93, p = 0.840, τ2 = 0).
The three studies investigating YBT/SEBT/FRT found an increased performance in one (62) and no change in two (51, 78). However, one (78) of these two studies used the FRT, also being the only one throughout this analysis. While the meta-analytic procedure for YBT/SEBT/FRT as well as sensitivity analysis excluding the FRT (sensitivity analysis because this test differs from YBT/SEBT as it involves mostly movement in the upper body while trying to maintain balance bipedally (85)) showed moderate improvements (ES: 0.53–0.61), these effect sizes were not significant (p = 0.440–0.560) additionally exhibiting high heterogeneity (τ2 = 1.43–2.2).
As for passive control comparisons, almost all (4 out of 5) of the included active-control studies (64, 70, 76, 77) tested young or middle-aged adults (18–65 yrs. of age). The remaining study (72) investigated effects in individuals aged 65 yrs. and older. Additionally, the majority of studies (4 out of 5) took place in clinical settings (64, 70, 72, 77). However, the sway/COP eyes open patient subgroup analysis (across different diseases) resulted in non-significant effects (p = 0.148).
Out of the five studies with active comparison groups, four investigated static standing sway/COP parameters with eyes open for which one study (77) found an improved performance and three (64, 70, 72) no change. Two of these studies additionally determined sway/COP with eyes closed whereby one found enhanced performance (77) and one no change (70). One subgroup also investigated the effects on sway/COP with eyes open of interventions solely employing static stretching (64, 72, 77). However, all calculations exhibited non-significant effects (p = 0.397–1). The remaining, fifth study (76) determined YBT/SEBT outcomes and showed performance increases.
Fifteen (15) studies investigated the acute effects of stretching compared to passive controls, whereby one of these (68) also incorporated active controls. For the passive control comparisons, all 15 studies incorporated healthy, young adults (18–65 yrs. of age), thus no results can be presented for older adult and patient populations.
Fourteen (14) studies investigated static standing sway/COP parameters with eyes open of which six (53, 55, 63, 65, 67, 75) found enhanced post-intervention balance performance, four (57, 58, 71, 74) no change and four (52, 59, 61, 73) a decreased performance. Pooling led to significant, small magnitude effects indicating beneficial effects for acute stretching on balance improvement (ES: 0.21 95%CI 0.02–0.39, p = 0.032, τ2 = 0.28) (see Table 2). Sensitivity analysis for stability index outcomes (excluding stability index outcomes to account for the possible impact of an index ceiling effect (81–84)) does not impact the effect size (ES: 0.20) but the level of significance (p = 0.066). While static and dynamic stretch subgroups yield trivial to moderate (ES: 0.04–0.52) magnitude effects that are, however, all non-significant (p = 0.086–0.537), both PNF subgroups for open-eyes outcomes show significant (p = 0.009–0.04) small magnitude effects (ES: 0.29–0.31).
Additionally, four of these 14 studies included static standing sway/COP tests with eyes closed, whereby two (53, 55) found significant positive effects, one (74) no difference and one (52) significant negative effects. The meta-analytical calculation exhibited a significant, trivial magnitude effect (ES: 0.19 95%CI 0.08–0.31, p = 0.010, τ2 = 0) when including all stretching types, with the subgroups for static and PNF stretching not reaching the level of significance (p = 0.070–0.635).
Moreover, two studies investigated YBT/SEBT outcomes both originating from static stretching interventions. While one found an increased performance (55), the other found no effects (68), pooling resulted in no significant change (p = 0.840).
All four studies (50, 54, 56, 68) incorporating active control conditions investigated young adults (18–65 yrs. of age). Out of the four studies, only one (56) used static standing sway/COP outcomes and found no effects. The remaining three studies investigated YBT/SEBT outcomes, whereby two (50, 54) showed performance increases and one (68) no change for which the meta-analytic calculation did not reach the level of significance (p = 0.550).
Modified funnel plot inspection indicated no publication bias for chronic effects, while some outliers caused a right shift of values. With p = 0.08 for chronic and p = 0.02 for acute effects, the Egger’s regression test supported these results.
Applying the GRADE criteria, certainty of evidence for the comparison of stretching studies on balance performance was initially rated as high due to the inclusion of (randomized) controlled trials. The level of evidence for chronic studies was downgraded for risk of bias (1 level, PEDro score of 5.4 being fair) and imprecision (1 level, few events and 95%CIs overlap no effect), resulting in low level of evidence meaning further research is very likely to impact the estimate of effect. The level of evidence for acute studies was downgraded for risk of bias (2 levels, PEDro score of 3.5 being poor), imprecision (1 level, few events and 95%CIs overlap no effect) and publication bias (1 level, as per visual inspection of funnel plots and the result of the Egger’s regression test), resulting in very low level of evidence meaning the effect estimate is very uncertain.
This is the first systematic review with meta-analysis that found, in accordance with the GRADE score, low level evidence for the effectiveness of chronic muscle stretching for improvements in standing postural sway (significant, moderate magnitude effects). The chronic stretching effects on dynamic balance control (YBT/SEBT) were not significant. Overall, only one of the three studies (78) on chronic effects included older individuals (over age 65 years) and two (51, 70) patients with multiple sclerosis or post ankle sprain.
Acute effects of stretching interventions exhibit very low level of evidence with significant but trivial (for closed-eyes condition) to small (for open-eyes condition) effects for standing postural sway. Acute effects on dynamic balance (YBT/SEBT) following stretching interventions were non-significant. Similar to the chronic effects, only one study (71) investigated acute effects in individuals aged 65 yrs. and older.
This review also calculated the effects of studies comparing stretching to alternative, active interventions such as self-mobilization (50), jumps (56), cycling (70), trunk stabilization exercises (77) or balance training (70). Since the meta-analysis showed non-significant effects for both acute and chronic studies opposing alternative interventions, it must be questioned whether the effects found when comparing stretching to passive controls can actually be attributed to the stretching interventions or just enhanced physical activity.
The literature provides several mechanisms that could explain stretch-induced chronic and acute adaptations in balance control, including flexibility, stiffness, strength and muscle size.
While stretching is known as the most common flexibility training method (32) it remains uncertain if changes in flexibility actually explain the improvements in balance control. Literature speculated about the role of range of motion on postural control and balance performance as flexibility-impairments might be associated with difficulties to regain standing stability following perturbations (86). Hereby, limited ankle dorsiflexion range of motion could lead to increased subtalar joint pronation (17) that in turn could increase sway due to problems in stabilization during ankle pronation or supination. However, there might be a ceiling effect once a certain threshold of “adequate” flexibility is met, depending on how balance is measured (84). Naturally, test-specifications play a major role which is true for how balance is evaluated as well: While YBT/SEBT require a subject to lower the body’s center of gravity and keep balance standing on one foot while reaching into different directions with the other, high levels of active movement and significant changes in the body’s center of gravity are not relevant for sway/COP measurements. Therefore, it seems that YBT/SEBT performance might benefit from increased flexibility to a higher degree compared to sway/COP performance. Another factor that appears un- or underinvestigated revolves around body dimensions such as the relation of upper to lower body or thigh to lower leg in sway measurement.
The three chronic stretching studies comparing stretching with passive control conditions that found unidirectional, positive effects for all measured outcomes in the stretching groups evaluated either SEBT (51), YBT (62) or postural stability measured on a force plate during jump landing (66), which can be, depending on the definition, described as dynamic balance test conditions. Due to the small samples and effect sizes, no meaningful subgroup analyses were performed in our meta-analysis to differentiate between dynamic and static balance performance.
Another parameter that could moderate the effects of stretching on balance is muscle stiffness. Stretching seems sufficient to reduce muscle stiffness parameters (87). Kim et al. (15) showed a relation of stiffness with balance performance assessed via FRT and standing balance in tandem, semi-tandem and side-by-side standing. This is in accordance with Epro et al. (16), who associated muscle-and/or tendon compliance with reduced postural control.
Unfortunately, muscle stiffness was only investigated in two of the included chronic stretching studies with passive controls showing conflicting results. While Sakai et al. (66) found decreased muscle stiffness following the intervention and increased balance performance, Gajdosik et al. (78) did neither find significant stiffness nor balance performance changes. Moreover, to the best of the authors’ knowledge, no review article investigated how stiffness affects balance.
Maximal strength is associated with balance in, e.g., children and old adults (2), elite soccer players (88) and adolescent gymnasts (89). While Mühlbauer et al. (2) focused on healthy participants showing a positive relationship, no current systematic review investigated the role of maximum strength on balance in patients. However, there are several articles investigating the strength-balance relationship in patients with several indications. For instance, Hu et al. (90) showed that maximal strength positively influences balance after reconstruction of the anterior cruciate ligament while Yahia et al. (91) and Brech et al. (92) confirmed benefits on postural control in patients with multiple sclerosis and osteoporosis. This relationship was especially strong for the plantar flexors. Multiple studies (93, 94) emphasized the possibility of increasing calf muscle maximal strength and size via stretching. Muscle strength abilities might, therefore, explain the results of Kim et al. (15) and Epro et al. (16) who positively associated plantar flexor muscle strength and thickness with balance. While systematic reviews highlight the possibility of increasing maximal strength and muscle size via stretching (23, 25), only two studies from the present review measured maximal strength alongside the balance adaptations. However, both studies (51, 66) did not find significantly increased balance performance following four weeks of stretching. The maximal strength, hereby, did also not change. Thus, the influence of stretch-induced maximal strength or muscle size increases on postural control remains speculative.
However, due to its impact on general joint stability, it seems reasonable to hypothesize that an improved maximal strength capacity would help to positively influence postural sway and balance performance.
Even though results from Kubo et al. (95) reported no changes in electromyographic activity following stretching training, findings of Miyahara et al. (96) suggested potential neuromuscular adaptations. Accordingly, Nelson et al. (93) described maximal strength increases of 11% in the non-stretched, contralateral leg after a 12-week intervention period, which might be attributed to remote central nervous learning responsible for optimized muscle activity in general (97, 98). However, changes in muscle activation patterns increasing maximal strength do not automatically suggest transferability on balance performance to decrease postural sway.
Previous studies showed the involvement of reflex mechanisms, including the stretch-and H-reflex, to maintain or restore standing postural control (99). Furthermore, since the Golgi-tendon unit (100) and muscle spindle activity (101) are involved in muscle tension and muscle length control one may also expect changes in proprioception due to stretching. This may further adjust the center of pressure regulation during standing (102) and consequently, balance performance in general. This agrees with findings of Gruber et al. (103, 104) who reported reduced peak-to-peak amplitudes in soleus electromyography (EMG) activity in specific balance tasks and changes in H-reflex activity after balance training.
Due to findings showing diminished strength performance as an acute response to (static) stretch performance (43), it was hypothesized that stretching would also acutely reduce balance performance. Surprisingly, the present analysis found small but significant positive effects of acute stretching on balance performance compared to passive controls. While stretching is frequently reported to negatively influence reflex-responses acutely (105, 106), Behm et al. (33) reported these effects to dissipate within seconds after the induced stimulus. Consequently, their influence on subsequent balance performance was classified unlikely.
Since no significant differences in acute balance performance could be obtained when comparing stretching or other interventions, it must be questioned whether the effects found in comparison to passive controls are stretch-specific. This is in line with most recent evidence from a systematic review with meta-analysis (36) that did not find stretch-specific acute changes in flexibility and stiffness when compared to any other warm-up intervention and hypothesized that, at least with the current state of literature, any activity that increases core and muscle temperatures is sufficient to elicit these changes.
Muscle stiffness, when measured in a relaxed state, and flexibility, when measured passively, are both outcomes that are not directly linked to neural control. In contrast, acute changes in balance performance might comprise further mechanisms that alter, e.g., proprioception and cognitive awareness, which, however, are also known to be enhanced following any kind of warm-up activity (107, 108).
Thus, at the current state, the explanations of stretch-specific effects for acutely improved balance performance remain speculative. To specifically attribute potential stretching effects on balance, further research controlling alternative explanatory approaches is needed. Hereby, the post-test timing needs to be considered critically, as muscular fatigue significantly decreases balance performance (109).
Although small effects were shown for chronic stretching on balance, there are several limitations that limit the findings’ generalizability. First, the number of studies is too small for sensible subgroup analyses of chronic and acute effects in different populations. As consequence, (a) some subgroup analyses do not reach the level of significance despite exhibiting moderate effect sizes or (b) pooled effects did not distinguish between healthy adults, patients and older adults or different stretching types/routines. The authors accounted for this by performing sensitivity analyses (excluding patient populations) or further subgroups (patients only) if possible. Though, the small number of outcomes resulted in no significant effects.
Also, high levels of heterogeneity can be observed for the included studies leading to difficulties regarding the interpretation of results. This might also be due to the heterogeneous study designs regarding the stretching interventions (intensity being oftentimes not even reported, frequency and session/overall stretching volume).
While a general warm-up prior to the stretching interventions was allowed in studies examining chronic effects, the control groups oftentimes did not receive structured interventions and thus remained non-active. Thus, it cannot be ruled out that small effects could actually be attributed to the warm-up program instead of stretching. This issue is exacerbated in one study (60) with a 15 min jogging-and jumping warm up. This study design only allows a biased interpretation of the influence of stretching.
Since no studies could be found that investigated underlying mechanisms, explanations regarding adaptations in sensorimotor or neuromuscular control mechanism can only be speculated upon. Further, the number of studies and effects in older adults settings were too small for meaningful effect pooling. Therefore, the present review calculated the effects based on heterogeneous study designs, outcomes and age groups leading to concerns regarding the validity of findings for specific populations.
The scarce number of (high quality) studies for the different age groups, stretching types and outcome measures in the field underlines the need for further investigations. Future randomized controlled stretching trials should be of high(er) methodological quality and include the investigation of different balance outcomes and a broad(er) range of potential underlying factors, such as muscle size and strength, flexibility, stiffness, neuromuscular activity and proprioception, to clarify the impact of stretching on specific outcomes such as fall prevention and motor function, especially in older adults and patients.
Although some subgroups showed small to moderate magnitude balance improvements, our analysis showed no significant effects. While one possible explanation for this lack of significance is that stretching per se does not provide a sufficient stimulus to enhance balance, it could be argued that the load control parameters chosen in the included studies were inadequate to affect balance. Assuming structural parameters such as muscle strength/size (2, 15, 16, 88, 89) or stiffness (15, 16) as potential moderators for balance, recent reviews determined the used stretching intensity (24), weekly volume (duration per bout times frequency) (25) or supervision (110) to impact the stretch-induced effects. Therefore, the lack of significance could also be the result of low stretching intensities [e.g., stretching until point/sense of discomfort (62, 67, 75)], pain-free stretching (69), stretching until slight level of discomfort (71)), insufficient weekly volume [(e.g., 78)] or frequency [(e.g., 72, 77)], insufficient intervention period [(e.g., 64, 76)] or performing stretching unsupervised [most did not state supervision, (e.g., 69, 71, 73, 76, 78)], which could, in turn, be associated with insufficient intensity (110). In contrast, since Konrad et al. (32) did not find these parameters to affect stretching results on flexibility, it could be speculated that flexibility might not be the primary outcome to affect balance. As a consequence, future studies should adopt research designs that have the potential to actually affect strength and hypertrophy (stretching durations >15 min per bout on more than 5 days per week for >6 weeks (25) or moderate stiffness (thus, be performed supervised (110)) to investigate the potential role of stretch-mediated effects in balance improvements to provide robust results.
Assuming muscle strength, size and flexibility to moderate balance, there are more common training interventions to target, for example maximal strength. Indeed, LaCroix et al. (20) found resistance training to be beneficial for balance capabilities, especially when supervised, while Hu et al. (111) showed that plantar massage as well as whole body vibration training also improved static balance with comparable effect sizes (0.54 and 0.66, respectively). Obviously, the highest effect sizes were reported for specific balance training, with, for example, ES = 0.83 for dynamic balance (112). It must be noted that there are several concurrent training approaches with partly very different effect sizes in specific patient groups such as chronic ankle instability patients (113, 114), Parkinson disease (115, 116) or back pain patients (117). Therefore, it seems necessary to investigate specific balance interventions for specific group settings and explore the outcomes to determine the most effective way to enhance balance in each of these. However, due to the currently limited number of stretching studies, direct subgroup comparisons will not reveal any valuable insights.
Due to the limited number of studies, high methodological heterogeneity as well as (very) low levels of certainty in the found evidence according to the GRADE score, it is nearly impossible to provide conclusive statements for the practical relevance of stretching for balance control. Reduced muscle strength in the lower leg is associated with ankle instability, however, especially immobilized and conditioned populations face obstacles when aiming to implement resistance training in an effective and safe manner. Especially in populations with limitations in motor function, stretching could provide a safe training intervention which could be implemented as an unsupervised training. However, the practical applicability of a resistance training substitution through stretching must be further investigated using higher stretching volumes in future randomized controlled trials that are of high(er) methodological quality. Consequently, stretching performed with the required volume and intensity might not only be relevant in counteracting immobilization-related muscle strength and size decreases (118), but could help prevent functional performance impairments, i.e., balance.
The original contributions presented in the study are included in the article/Supplementary material, further inquiries can be directed to the corresponding author.
LL: Conceptualization, Data curation, Formal analysis, Methodology, Supervision, Writing – original draft, Writing – review & editing. AZ: Conceptualization, Methodology, Writing – review & editing, Supervision. GP: Data curation, Writing – review & editing, Formal analysis. MO: Data curation, Writing – review & editing, Formal analysis. DJ: Data curation, Software, Visualization, Writing – review & editing. KW: Data curation, Formal analysis, Software, Supervision, Visualization, Writing – original draft, Writing – review & editing, Methodology.
The author(s) declare that financial support was received for the research, authorship, and/or publication of this article. The authors acknowledge the financial support by the University of Graz.
The authors declare that the research was conducted in the absence of any commercial or financial relationships that could be construed as a potential conflict of interest.
The author(s) declared that they were an editorial board member of Frontiers, at the time of submission. This had no impact on the peer review process and the final decision.
All claims expressed in this article are solely those of the authors and do not necessarily represent those of their affiliated organizations, or those of the publisher, the editors and the reviewers. Any product that may be evaluated in this article, or claim that may be made by its manufacturer, is not guaranteed or endorsed by the publisher.
The Supplementary material for this article can be found online at: https://www.frontiersin.org/articles/10.3389/fmed.2024.1451180/full#supplementary-material
CI, Confidence interval; COP, Center of pressure; FRT, Functional reach test; M, Mean; PNF, Proprioceptive neuromuscular facilitation; SEBT, Star excursion balance test; SD, Standard deviation; SMD, Standardized mean difference; YBT, Y-balance test; yrs., Years; ES, Effect size.
1. Sherrington, C, Fairhall, NJ, Wallbank, GK, Tiedemann, A, Michaleff, ZA, Howard, K, et al. Exercise for preventing falls in older people living in the community. Cochrane Database Syst Rev. (2019) 2019:CD012424. doi: 10.1002/14651858.CD012424.pub2
2. Muehlbauer, T, Gollhofer, A, and Granacher, U. Associations between measures of balance and lower-extremity muscle strength/Power in healthy individuals across the lifespan: a systematic review and meta-analysis. Sport Med. (2015) 45:1671–92. doi: 10.1007/s40279-015-0390-z
3. Fitzpatrick, R, and McCloskey, DI. Proprioceptive, visual and vestibular thresholds for the perception of sway during standing in humans. J Physiol. (1994) 478:173–86. doi: 10.1113/jphysiol.1994.sp020240
4. Behm, DG, Muehlbauer, T, Kibele, A, and Granacher, U. Effects of strength training using unstable surfaces on strength, Power and balance performance across the lifespan: a systematic review and meta-analysis. Sport Med. (2015) 45:1645–69. doi: 10.1007/s40279-015-0384-x
5. Currier, BS, McLeod, JC, Banfield, L, Beyene, J, Welton, NJ, D’Souza, AC, et al. Resistance training prescription for muscle strength, hypertrophy and physical function in healthy adults: a systematic review and Bayesian network meta-analysis. Br J Sports Med. (2023) 57:1211–1220. doi: 10.1136/bjsports-2023-106807
6. Phelan, EA, and Ritchey, K. Fall prevention in community-dwelling older adults. Ann Intern Med. (2018) 169:ITC81–96. doi: 10.7326/AITC201812040
7. Kruschke, C, and Butcher, HK. Evidence-based practice guideline: fall prevention for older adults. J Gerontol Nurs. (2017) 43:15–21. doi: 10.3928/00989134-20171016-01
8. Florence, CS, Bergen, G, Atherly, A, Burns, E, Stevens, J, and Drake, C. Medical costs of fatal and nonfatal falls in older adults. J Am Geriatr Soc. (2018) 66:693–8. doi: 10.1111/jgs.15304
9. Schoene, D, Heller, C, Aung, YN, Sieber, CC, Kemmler, W, and Freiberger, E. A systematic review on the influence of fear of falling on quality of life in older people: is there a role for falls? Clin Interv Aging. (2019) 14:701–19. doi: 10.2147/CIA.S197857
10. Allison, LK, Painter, JA, Emory, A, Whitehurst, P, and Raby, A. Participation restriction, not fear of falling, predicts actual balance and mobility abilities in rural community-dwelling older adults. J Geriatr Phys Ther. (2013) 36:13–23. doi: 10.1519/JPT.0b013e3182493d20
11. Bowden Davies, KA, Pickles, S, Sprung, VS, Kemp, GJ, Alam, U, Moore, DR, et al. Reduced physical activity in young and older adults: metabolic and musculoskeletal implications. Ther Adv Endocrinol Metab. (2019) 10:204201881988882–15. doi: 10.1177/2042018819888824
12. Suwa, M, Imoto, T, Kida, A, and Yokochi, T. Early reduction in toe flexor strength is associated with physical activity in elderly men. J Phys Ther Sci. (2016) 28:1472–7. doi: 10.1589/jpts.28.1472
13. Cattagni, T, Scaglioni, G, Laroche, D, Van Hoecke, J, Gremeaux, V, and Martin, A. Ankle muscle strength discriminates fallers from non-fallers. Front Aging Neurosci. (2014) 6:1–7. doi: 10.3389/fnagi.2014.00336
14. American Geriatrics Society Panel on Falls in Older Persons. “Guideline for the prevention of falls in older persons”: essential reading. Age Ageing. (2002) 49:664–72. doi: 10.1046/j.1532-5415.2001.49115.x
15. Kim, N, Park, J, Shin, H, and Bae, Y. Gastrocnemius medial head stiffness is associated with potential fall risk in community-dwelling older adults. Healthc. (2022) 10:785. doi: 10.3390/healthcare10050785
16. Epro, G, McCrum, C, Mierau, A, Leyendecker, M, Brüggemann, GP, and Karamanidis, K. Effects of triceps surae muscle strength and tendon stiffness on the reactive dynamic stability and adaptability of older female adults during perturbed walking. J Appl Physiol. (2018) 124:1541–9. doi: 10.1152/japplphysiol.00545.2017
17. Nakagawa, TH, and Petersen, RS. Relationship of hip and ankle range of motion, trunk muscle endurance with knee valgus and dynamic balance in males. Phys Ther Sport. (2018) 34:174–9. doi: 10.1016/j.ptsp.2018.10.006
18. Task force on global guidelines for falls in older adults. World guidelines for falls prevention and management for older adults: a global initiative. Age Ageing. (2022) 51:afac205. doi: 10.1093/ageing/afac205
19. Chang, S, Tan, Y, Cheng, L, Zhou, L, Wang, B, and Liu, H. Effect of strength training with additional acupuncture on balance, ankle sensation, and isokinetic muscle strength in chronic ankle instability among college students. Front Physiol. (2024) 15:1–10. doi: 10.3389/fphys.2024.1324924
20. Lacroix, A, Hortobágyi, T, Beurskens, R, and Granacher, U. Effects of supervised vs. unsupervised training programs on balance and muscle strength in older adults: a systematic review and Meta-analysis. Sport Med. (2017) 47:2341–61. doi: 10.1007/s40279-017-0747-6
21. Morie, M, Reid, KF, Miciek, R, Lajevardi, N, Choong, K, Krasnoff, JB, et al. Habitual physical activity levels are associated with performance in measures of physical function and mobility in older men. J Am Geriatr Soc. (2010) 58:1727–33. doi: 10.1111/j.1532-5415.2010.03012.x
22. Alizadeh, S, Daneshjoo, A, Zahiri, A, Anvar, SH, Goudini, R, Hicks, JP, et al. Resistance training induces improvements in range of motion: a systematic review and Meta-analysis. Sport Med. (2023) 53:707–22. doi: 10.1007/s40279-022-01804-x
23. Arntz, F, Markov, A, Behm, DG, Behrens, M, Negra, Y, Nakamura, M, et al. Chronic effects of static stretching exercises on muscle strength and Power in healthy individuals across the lifespan: a systematic review with multi-level Meta-analysis. Sport Med. (2023) 53:723–45. doi: 10.1007/s40279-022-01806-9
24. Panidi, I, Donti, O, Konrad, A, Dinas, PC, Terzis, G, Mouratidis, A, et al. Muscle architecture adaptations to static stretching training: a systematic review with Meta-analysis. Sport Med-Open. (2023) 9:47. doi: 10.1186/s40798-023-00591-7
25. Warneke, K, Lohmann, LH, Behm, DG, Wirth, K, Keiner, M, Schiemann, S, et al. Effects of chronic static stretching on maximal strength and muscle hypertrophy: a systematic review and Meta-analysis with Meta-regression. Sport Med-Open. (2024) 10:706. doi: 10.1186/s40798-024-00706-8
26. Behm, DG, Granacher, U, Warneke, K, Aragão-Santos, JC, Da Silva-Grigoletto, ME, and Konrad, A. Minimalist training: Is lower dosage or intensity resistance training effective to improve physical fitness? A Narrative Review. Sport Med. (2023) 54:289–302. doi: 10.1007/s40279-023-01949-3
27. Wohlann, T, Warneke, K, Kalder, V, Behm, DG, Schmidt, T, and Schiemann, S. Influence of 8-weeks of supervised static stretching or resistance training of pectoral major muscles on maximal strength, muscle thickness and range of motion. Eur J Appl Physiol. (2024) 124:1885–93. doi: 10.1007/s00421-023-05413-y
28. Warneke, K, Wirth, K, Keiner, M, Lohmann, LH, Hillebrecht, M, Brinkmann, A, et al. Comparison of the effects of long-lasting static stretching and hypertrophy training on maximal strength, muscle thickness and flexibility in the plantar flexors. Eur J Appl Physiol. (2023) 123:1773–87. doi: 10.1007/s00421-023-05184-6
29. Page, MJ, McKenzie, JE, Bossuyt, PM, Boutron, I, Hoffmann, TC, Mulrow, CD, et al. The PRISMA 2020 Statement: an updated guideline for reporting systematic reviews. BMJ. (2021) 372:71. doi: 10.1136/bmj.n71
30. Eriksen, MB, and Frandsen, TF. The impact of patient, intervention, comparison, outcome (PICO) as a search strategy tool on literature search quality: a systematic review. J Med Libr Assoc. (2018) 106:420–31. doi: 10.5195/jmla.2018.345
31. Thomas, E, Bianco, A, Paoli, A, and Palma, A. The relation between stretching typology and stretching duration: The effects on range of motion. Int J Sports Med. (2018) 39:243–54. doi: 10.1055/s-0044-101146
32. Konrad, A, Alizadeh, S, Daneshjoo, A, Anvar, SH, Graham, A, Zahiri, A, et al. Chronic effects of stretching on range of motion with consideration of potential moderating variables: a systematic review with meta-analysis. J Sport Heal Sci. (2023) 13:186–94. doi: 10.1016/j.jshs.2023.06.002
33. Behm, DG, Kay, AD, Trajano, GS, and Blazevich, AJ. Mechanisms underlying performance impairments following prolonged static stretching without a comprehensive warm-up. Eur J Appl Physiol. (2021) 121:67–94. doi: 10.1007/s00421-020-04538-8
34. Lima, CA, Ricci, NA, Nogueira, EC, and Perracini, MR. The berg balance scale as a clinical screening tool to predict fall risk in older adults: a systematic review. Physiother (United Kingdom). (2018) 104:383–94. doi: 10.1016/j.physio.2018.02.002
35. Behm, DG. The science and physiology of flexibility and stretching: Implications and applications in sport performance and health. London: Routledge (2018).
36. Warneke, K, Plöschberger, G, Lohmann, LH, Lichtenstein, E, Jochum, D, Siegel, SD, et al. Foam rolling and stretching do not provide superior acute flexibility and stiffness improvements compared to any other warm-up intervention: a systematic review with meta-analysis. J Sport Heal Sci. (2024) 13:509–20. doi: 10.1016/j.jshs.2024.01.006
37. Nordez, A, Casari, P, Mariot, JP, and Cornu, C. Modeling of the passive mechanical properties of the musculo-articular complex: acute effects of cyclic and static stretching. J Biomech. (2009) 42:767–73. doi: 10.1016/j.jbiomech.2008.12.019
38. de Morton, NA. The PEDro scale is a valid measure of the methodological quality of clinical trials: a demographic study. Aust J Physiother. (2009) 55:129–33. doi: 10.1016/S0004-9514(09)70043-1
39. Afonso, J, Ramirez-Campillo, R, Clemente, FM, Büttner, FC, and Andrade, R. The perils of misinterpreting and misusing “publication Bias” in meta-analyses: an education review on funnel plot-based methods. Sport Med. (2024) 54:257–69. doi: 10.1007/s40279-023-01927-9
40. Mavridis, D, and Salanti, G. Exploring and accounting for publication bias in mental health: a brief overview of methods. Evid Based Ment Health. (2014) 17:11–5. doi: 10.1136/eb-2013-101700
41. Fernández-Castilla, B, Declercq, L, Jamshidi, L, Beretvas, SN, Onghena, P, and van den Noortgate, W. Visual representations of meta-analyses of multiple outcomes: extensions to forest plots, funnel plots, and caterpillar plots. Methodology. (2020) 16:299–315. doi: 10.5964/meth.4013
42. Fisher, Z, and Tipton, E. Robumeta: an R-package for robust variance estimation in meta-analysis. ar Xiv. (2015)
43. Warneke, K, and Lohmann, LH. Revisiting the stretch-induced force deficit – a systematic review with multilevel meta-analysis of acute effects. J Sport Heal Sci. (2024) 13:805–19. doi: 10.1016/j.jshs.2024.05.002
44. Kiss, R, Schedler, S, and Muehlbauer, T. Associations between types of balance performance in healthy individuals across the lifespan: a systematic review and meta-analysis. Front Physiol. (2018) 1366:9. doi: 10.3389/fphys.2018.01366
45. Ringhof, S, and Stein, T. Biomechanical assessment of dynamic balance: specificity of different balance tests. Hum Mov Sci. (2017) 58:140–7. doi: 10.1016/j.humov.2018.02.004
46. Rougier, P. The influence of having the eyelids open or closed on undisturbed postural control. Neurosci Res. (2003) 47:73–83. doi: 10.1016/S0168-0102(03)00187-1
47. Isableu, B, Hlavackova, P, Diot, B, and Vuillerme, N. Regularity of center of pressure trajectories in expert gymnasts during bipedal closed-eyes quiet standing. Front Hum Neurosci. (2017) 11:1–7. doi: 10.3389/fnhum.2017.00317
48. Faraone, SV. Interpreting estimates of treatment effects: implications for managed care. P T. (2008) 33:700–11.
49. GRADE working group. Grading quality of evidence and strength of recommendations. BMJ. (2004) 328:1490. doi: 10.1136/bmj.328.7454.1490
50. Akdag, MB, Badilli, FS, and Akkus, Z. Acute effects of dynamic stretching and self mobilization exercises on balance and proprioception. J Int Dent Med Res. (2021) 14:1210–8.
51. Alahmari, KA, Silvian, P, Ahmad, I, Reddy, RS, Tedla, JS, Kakaraparthi, VN, et al. Effectiveness of low-frequency stimulation in proprioceptive neuromuscular facilitation techniques for post ankle sprain balance and proprioception in adults: a randomized controlled trial. Biomed Res Int. (2020) 2020:1–13. doi: 10.1155/2020/9012930
52. Ghram, A, Damak, M, and Costa, PB. Effect of acute contract-relax proprioceptive neuromuscular facilitation stretching on static balance in healthy men. Sci Sport. (2017) 32:e1–7. doi: 10.1016/j.scispo.2016.06.005
53. Ghram, A, Yahia, A, Damak, M, Safaei Motlagh, A, Jribi, S, and Costa, PB. Effects of different types of proprioceptive neuromuscular facilitation stretching on dynamic balance control. Sport Sci Health. (2020) 16:451–8. doi: 10.1007/s11332-020-00623-9
54. Jouira, G, Srihi, S, Ben, WF, Rebai, H, and Sahli, S. Dynamic balance in athletes with intellectual disability: effect of dynamic stretching and plyometric warm-ups. J Sport Rehabil. (2021) 30:401–7. doi: 10.1123/jsr.2020-0100
55. Jung, EY, Jung, JH, Cho, HY, and Kim, SH. Effects of plantar flexor stretching on static and dynamic balance in healthy adults. Int J Environ Res Public Health. (2023) 20:1462. doi: 10.3390/ijerph20021462
56. Kim, K, Lee, T, Kang, G, Kwon, S, Choi, S, and Park, S. The effects of diverse warm-up exercises on balance. J Phys Ther Sci. (2014) 26:1601–3. doi: 10.1589/jpts.26.1601
57. Leblebici, H, Yarar, H, Aydin, EM, Zorlu, Z, Ertas, U, and Kingir, ME. The acute effects of different stretching on dynamic balance performance. Int J Sport Stud. (2017) 7:153–9.
58. Il, LK, Nam, HC, and Jung, KS. Effects on hamstring muscle extensibility, muscle activity, and balance of different stretching techniques. J Phys Ther Sci. (2014) 26:209–13. doi: 10.1589/jpts.26.209
59. Lima, BN, Lucareli, PRG, Gomes, WA, Silva, JJ, Bley, AS, Hartigan, EH, et al. The acute effects of unilateral ankle plantar flexors static-stretching on postural sway and gastrocnemius muscle activity during single-leg balance tasks. J Sport Sci Med. (2014) 13:564–70.
60. Mel’nikov, AA, Smirnova, PA, Nikolaev, RY, Podolyaka, OB, and Andreeva, AM. Influence of stretching training of the lower limbs on postural stability. Hum Physiol. (2021) 47:270–81. doi: 10.1134/S0362119721030129
61. Oba, K, Ohta, M, Mani, H, Suzuki, T, Ogasawara, K, and Samukawa, M. The effects of static stretching on dynamic postural control during maximum forward leaning task. J Mot Behav. (2021) 55:594–602. doi: 10.1080/00222895.2021.1909529
62. Alimoradi, M, Sahebozamani, M, Hosseini, E, Konrad, A, and Noorian, S. The effect on flexibility and a variety of performance tests of the addition of 4 weeks of soleus stretching to a regular dynamic stretching routine in amateur female soccer players. Sports. (2023) 11:138. doi: 10.3390/sports11070138
63. Oba, K, Kyotani, N, Tanaka, M, Komatsuzaki, M, Kasahara, S, Ogasawara, K, et al. Acute effects of static and dynamic stretching for ankle plantar flexors on postural control during the single-leg standing task. Sport Biomech. (2023) 1–11. doi: 10.1080/14763141.2023.2298967
64. Park, D, Lee, JH, Kang, TW, and Cynn, HS. Four-week training involving ankle mobilization with movement versus static muscle stretching in patients with chronic stroke: a randomized controlled trial. Top Stroke Rehabil. (2018) 26:81–6. doi: 10.1080/10749357.2018.1550614
65. Ryan, EE, Rossi, MD, and Lopez, R. The effects of the contract relax-antagonist-contract form of proprioceptive neuromuscular facilitation stretching on postural stability. J Strength Cond Res. (2010) 24:1888–94. doi: 10.1519/JSC.0b013e3181ddad9d
66. Sakai, S, Maeda, N, Sasadai, J, Kotoshiba, S, Anami, K, Tashiro, T, et al. Effect of 4-week cyclic stretching program on muscle properties and physical performance in healthy adult men. J Sports Med Phys Fitness. (2020) 60:37–44. doi: 10.23736/S0022-4707.19.09870-0
67. Szafraniec, R, Chromik, K, Poborska, A, and Kawczyński, A. Acute effects of contract-relax proprioceptive neuromuscular facilitation stretching of hip abductors and adductors on dynamic balance. Peer J. (2018) 6:e6108–12. doi: 10.7717/peerj.6108
68. Thomas, E, Ficarra, S, Scardina, A, Bellafiore, M, Palma, A, Maksimovic, N, et al. Positional transversal release is effective as stretching on range of movement, performance and balance: a cross-over study. BMC Sports Sci Med Rehabil. (2022) 14:1–11. doi: 10.1186/s13102-022-00599-8
69. Todde, F, Carcangiu, A, Pusceddu, N, Di Giacomo, A, and Tocco, F. The effects of two different 8-week stretching protocols on postural stability in amateur sportsmen. Sport Sci Health. (2022) 18:545–9. doi: 10.1007/s11332-021-00842-8
70. Tollár, J, Nagy, F, Tóth, BE, Török, K, Szita, K, Csutorás, B, et al. Exercise effects on multiple sclerosis quality of life and clinical-motor symptoms. Med Sci Sports Exerc. (2020) 52:1007–14. doi: 10.1249/MSS.0000000000002228
71. Wallmann, HW, Player, KR, and Bugnet, M. Acute effects of static stretching on balance in young versus elderly adults. Phys Occup Ther Geriatr. (2012) 30:301–15. doi: 10.3109/02703181.2012.719076
72. Ayán, C, Bidaurrazaga-Letona, I, Martin, A, Lejonagoitia-Garmendia, M, Torres-Unda, J, and Esain, I. Effects of stretching vs. in hatha yoga people with mild to moderate Parkinson’s disease: a randomized controlled trial. Sci Sport. (2023) 38:631–5. doi: 10.1016/j.scispo.2022.09.010
73. Behm, DG, Bambury, A, Cahill, F, and Power, K. Effect of acute static stretching on force, balance, reaction time, and movement time. Med Sci Sports Exerc. (2004) 36:1397–402. doi: 10.1249/01.MSS.0000135788.23012.5F
74. Coratella, G, Longo, S, Rampichini, S, Doria, C, Borrelli, M, Limonta, E, et al. Passive stretching decreases muscle efficiency in balance tasks. PLoS One. (2021) 16:e0256656. doi: 10.1371/journal.pone.0256656
75. Costa, PB, Graves, BS, Whitehurst, M, and Jacobs, PL. The acute effects of different durations of static stretching on dynamic balance performance. J Strength Cond Res. (2009) 23:141–7. doi: 10.1519/JSC.0b013e31818eb052
76. Espí-López, GV, López-Martínez, S, Inglés, M, Serra-Añó, P, and Aguilar-Rodríguez, M. Effect of manual therapy versus proprioceptive neuromuscular facilitation in dynamic balance, mobility and flexibility in field hockey players. A randomized controlled trial. Phys Ther Sport. (2018) 32:173–9. doi: 10.1016/j.ptsp.2018.04.017
77. Fontana Carvalho, AP, Dufresne, SS, Rogério De Oliveira, M, Couto Furlanetto, K, Dubois, M, Dallaire, M, et al. Effects of lumbar stabilization and muscular stretching on pain, disabilities, postural control and muscle activation in pregnant woman with low back pain. Eur J Phys Rehabil Med. (2020) 56:297–306. doi: 10.23736/S1973-9087.20.06086-4
78. Gajdosik, RL, Vander Linden, DW, McNair, PJ, Williams, AK, and Riggin, TJ. Effects of an eight-week stretching program on the passive-elastic properties and function of the calf muscles of older women. Clin Biomech. (2005) 20:973–83. doi: 10.1016/j.clinbiomech.2005.05.011
79. Alghadir, AH, Iqbal, ZA, Iqbal, A, Ahmed, H, and Ramteke, SU. Effect of chronic ankle sprain on pain, range of motion, proprioception, and balance among athletes. Int J Environ Res Public Heal. (2020) 17:1–10. doi: 10.3390/ijerph17155318
80. McGinley, MP, Goldschmidt, CH, and Rae-Grant, AD. Diagnosis and treatment of multiple sclerosis: a review. JAMA. (2021) 325:765–79. doi: 10.1001/jama.2020.26858
81. Downs, S, Marquez, J, and Chiarelli, P. The berg balance scale has high intra-and inter-rater reliability but absolute reliability varies across the scale: a systematic review. J Physiother. (2013) 59:93–9. doi: 10.1016/S1836-9553(13)70161-9
82. Park, KN, Yang, M, Yoo, TG, and Kim, SH. One-leg standing and Y-balance test performance in elderly fallers and nonfallers. Top Geriatr Rehabil. (2020) 36:92–6. doi: 10.1097/TGR.0000000000000262
83. Liu, Q, and Wang, L. T-test and ANOVA for data with ceiling and/or floor effects. Behav Res Methods. (2021) 53:264–77. doi: 10.3758/s13428-020-01407-2
84. Rahlf, AL, John, C, Hamacher, D, and Zech, A. Effects of a 10 vs. 20-min injury prevention program on neuromuscular and functional performance in adolescent football players. Front Physiol. (2020) 11:1–9. doi: 10.3389/fphys.2020.578866
85. Cavanaugh, JT, Shinberg, M, Ray, L, Shipp, KM, Kuchibhatla, M, and Schenkman, M. Kinematic characterization of standing reach: comparison of younger vs. older subjects. Clin Biomech. (1999) 14:271–9. doi: 10.1016/S0268-0033(98)00074-6
86. Shafizadegan, Z, Baharlouei, H, Khoshavi, O, Garmabi, Z, and Fereshtenejad, N. Evaluating the short term effects of kinesiology taping and stretching of gastrocnemius on postural control: a randomized clinical trial. J Bodyw Mov Ther. (2020) 24:196–201. doi: 10.1016/j.jbmt.2019.11.003
87. Takeuchi, K, Nakamura, M, Fukaya, T, Konrad, A, and Mizuno, T. Acute and long-term effects of static stretching on muscle-tendon unit stiffness: a systematic review and Meta-analysis. J Sport Sci Med. (2023). 22:465–75. doi: 10.52082/jssm.2023.465
88. Śliwowski, R, Marynowicz, J, Jadczak, Ł, Grygorowicz, M, Kalinowski, P, and Paillard, T. The relationships between knee extensors/flexors strength and balance control in elite male soccer players. Peer J. (2021) 9:e12461–15. doi: 10.7717/peerj.12461
89. Kyselovičová, O, Zemková, E, Péliová, K, and Matejová, L. Isokinetic leg muscle strength relationship to dynamic balance reflects gymnast-specific differences in adolescent females. Front Physiol. (2023) 13:1–17. doi: 10.3389/fphys.2022.1084019
90. Hu, S, Ma, X, Ma, X, Sun, W, Zhou, Z, Chen, Y, et al. Relationship of strength, joint kinesthesia, and plantar tactile sensation to dynamic and static postural stability among patients with anterior cruciate ligament reconstruction. Front Physiol. (2023) 14:1112708. doi: 10.3389/fphys.2023.1112708
91. Yahia, A, Ghroubi, S, Mhiri, C, and Elleuch, MH. Relationship between muscular strength, gait and postural parameters in multiple sclerosis. Ann Phys Rehabil Med. (2011) 54:144–55. doi: 10.1016/j.rehab.2011.02.004
92. Brech, GC, Alonso, AC, Luna, NMS, and Greve, JM. Correlation of postural balance and knee muscle strength in the sit-to-stand test among women with and without postmenopausal osteoporosis. Osteoporos Int. (2013) 24:2007–13. doi: 10.1007/s00198-013-2285-x
93. Nelson, AG, Kokkonen, J, Winchester, JB, Kalani, W, Peterson, K, Kenly, MS, et al. A 10-week stretching program increases strength in the contralateral muscle. J Strength Cond Res. (2012) 26:832–6. doi: 10.1519/JSC.0b013e3182281b41
94. Warneke, K, Brinkmann, A, Hillebrecht, M, and Schiemann, S. Influence of long-lasting static stretching on maximal strength, muscle thickness and flexibility. Front Physiol. (2022) 13:1–13. doi: 10.3389/fphys.2022.878955
95. Kubo, K, Kanehisa, H, and Fukunaga, T. Effects of resistance and stretching training programmes on the viscoelastic properties of human tendon structures in vivo. J Physiol. (2002) 538:219–26. doi: 10.1113/jphysiol.2001.012703
96. Miyahara, Y, Naito, H, Ogura, Y, Katamoto, S, and Aoki, J. Effects of proprioceptive neuromuscular facilitation stretching and static stretching on maximal voluntary contraction. J Strength Cond Res. (2013) 27:195–201. doi: 10.1519/JSC.0b013e3182510856
97. Zhou, S. Chronic neural adaptations to unilateral exercise: mechanisms of cross education. Exerc Sport Sci Rev. (2000) 28:177–84.
98. Zhou, S, Zhang, SS, and Crowley-McHattan, ZJ. A scoping review of the contralateral effects of unilateral peripheral stimulation on neuromuscular function. PLoS One. (2022) 17:1–40.
99. Shimba, S, Kawashima, N, Ohta, Y, Yamamoto, SI, and Nakazawa, K. Enhanced stretch reflex excitability in the soleus muscle during passive standing posture in humans. J Electromyogr Kinesiol. (2010) 20:406–12. doi: 10.1016/j.jelekin.2009.04.003
100. Moore, JC. The Golgi tendon organ: a review and update. Am J Occup Ther. (1984) 38:227–36. doi: 10.5014/ajot.38.4.227
101. Zalkind, VI. Regularity of muscle spindle receptor discharges at a different muscle length. Neurosci Behav Physiol. (1984) 14:318–26. doi: 10.1007/BF01149618
102. Banks, RW, Ellaway, PH, Prochazka, A, and Proske, U. Secondary endings of muscle spindles: structure, reflex action, role in motor control and proprioception. Exp Physiol. (2021) 106:2339–66. doi: 10.1113/EP089826
103. Gruber, M, Gruber, SBH, Taube, W, Schubert, M, Beck, SC, and Gollhofer, A. Differential effects of ballistic versus sensorimotor training on rate of force development and neural activation in humans. J Strength Cond Res. (2007) 21:274–82. doi: 10.1519/00124278-200702000-00049
104. Gruber, M, Taube, W, Gollhofer, A, Beck, S, Amtage, F, and Schubert, M. Training-specific adaptations of H- and stretch reflexes in human soleus muscle. J Mot Behav. (2007) 39:68–78. doi: 10.3200/JMBR.39.1.68-78
105. Avela, J, Kyröläinen, H, and Komi, PV. Altered reflex sensitivity after repeated and prolonged passive muscle stretching. J Appl Physiol. (1999) 86:1283–91. doi: 10.1152/jappl.1999.86.4.1283
106. Mrachacz-Kersting, N, and Sinkjaer, T. Reflex and non-reflex torque responses to stretch of the human knee extensors. Exp Brain Res. (2003) 151:72–81. doi: 10.1007/s00221-003-1483-8
107. Daneshjoo, A, Mokhtar, AH, Rahnama, N, and Yusof, A. The effects of comprehensive warm-up programs on proprioception, static and dynamic balance on male soccer players. PLoS One. (2012) 7:1–10. doi: 10.1371/journal.pone.0051568
108. Mehl, J, Diermeier, T, Herbst, E, Imhoff, AB, Stoffels, T, Zantop, T, et al. Evidence-based concepts for prevention of knee and ACL injuries. 2017 guidelines of the ligament committee of the German knee society (DKG). Arch Orthop Trauma Surg. (2018) 138:51–61. doi: 10.1007/s00402-017-2809-5
109. Zech, A, Steib, S, Hentschke, C, Eckhardt, H, and Pfeifer, K. Effects of localized and general fatigue on static and dynamic postural control in male team handball athletes. J Strength Cond Res. (2012) 26:1162–8. doi: 10.1519/JSC.0b013e31822dfbbb
110. Warneke, K, Lohmann, LH, Plöschberger, G, and Konrad, A. Critical evaluation and recalculation of current systematic reviews with meta-analysis on the effects of acute and chronic stretching on passive properties and passive peak torque. Eur J Appl Physiol. (2024). doi: 10.1007/s00421-024-05564-6
111. Hu, X, Liao, J, Hu, X, Zeng, Z, and Wang, L. Effects of plantar-sensory treatments on postural control in chronic ankle instability: a systematic review and meta-analysis. PLoS One. (2023) 18:e0287689. doi: 10.1371/journal.pone.0287689
112. Mollà-Casanova, S, Inglés, M, and Serra-Añó, P. Effects of balance training on functionality, ankle instability, and dynamic balance outcomes in people with chronic ankle instability: systematic review and meta-analysis. Clin Rehabil. (2021) 35:1694–709. doi: 10.1177/02692155211022009
113. Guo, Y, Cheng, T, Yang, Z, Huang, Y, Li, M, and Wang, T. A systematic review and meta-analysis of balance training in patients with chronic ankle instability. Syst Rev. (2024) 13:1–10. doi: 10.1186/s13643-024-02455-x
114. Koshino, Y, and Kobayashi, T. Effects of conservative interventions on static and dynamic balance in individuals with chronic ankle instability: a systematic review and Meta-analysis. Arch Phys Med Rehabil. (2023) 104:673–85. doi: 10.1016/j.apmr.2022.10.014
115. Qian, Y, Fu, X, Zhang, H, Yang, Y, and Wang, G. Comparative efficacy of 24 exercise types on postural instability in adults with Parkinson’s disease: a systematic review and network meta-analysis. BMC Geriatr. (2023) 23:1–16. doi: 10.1186/s12877-023-04239-9
116. Shen, X, Wong-Yu, ISK, and Mak, MKY. Effects of exercise on falls, balance, and gait ability in Parkinson’s disease. Neurorehabil Neural Repair. (2016) 30:512–27. doi: 10.1177/1545968315613447
117. Dal Farra, F, Arippa, F, Arru, M, Cocco, M, Porcu, E, Tramontano, M, et al. Effects of exercise on balance in patients with non-specific low back pain: a systematic review and meta-analysis. Eur J Phys Rehabil Med. (2022) 58:423–34. doi: 10.23736/S1973-9087.21.07293-2
Keywords: stretching, postural control, center of pressure, sway, Y-balance
Citation: Lohmann LH, Zech A, Plöschberger G, Oraže M, Jochum D and Warneke K (2024) Acute and chronic effects of stretching on balance: a systematic review with multilevel meta-analysis. Front. Med. 11:1451180. doi: 10.3389/fmed.2024.1451180
Received: 18 June 2024; Accepted: 19 August 2024;
Published: 13 September 2024.
Edited by:
Warren G. Darling, The University of Iowa, United StatesCopyright © 2024 Lohmann, Zech, Plöschberger, Oraže, Jochum and Warneke. This is an open-access article distributed under the terms of the Creative Commons Attribution License (CC BY). The use, distribution or reproduction in other forums is permitted, provided the original author(s) and the copyright owner(s) are credited and that the original publication in this journal is cited, in accordance with accepted academic practice. No use, distribution or reproduction is permitted which does not comply with these terms.
*Correspondence: Lars Hubertus Lohmann, bG9obWFubmxhcnNAZ214LmRl
†ORCID: Lars Hubertus Lohmann, http://orcid.org/0000-0002-5990-2290
Astrid Zech, http://orcid.org/0000-0001-7147-4113
Gerit Plöschberger, http://orcid.org/0009-0003-4551-585X
Manuel Oraže, http://orcid.org/0009-0000-2835-2225
Daniel Jochum, http://orcid.org/0000-0002-8478-4185
Konstantin Warneke, http://orcid.org/0000-0003-4964-2867
Disclaimer: All claims expressed in this article are solely those of the authors and do not necessarily represent those of their affiliated organizations, or those of the publisher, the editors and the reviewers. Any product that may be evaluated in this article or claim that may be made by its manufacturer is not guaranteed or endorsed by the publisher.
Research integrity at Frontiers
Learn more about the work of our research integrity team to safeguard the quality of each article we publish.