- 1Department of Ophthalmology, Peking Union Medical College Hospital, Chinese Academy of Medical Sciences, Beijing, China
- 2Key Laboratory of Ocular Fundus Diseases, Chinese Academy of Medical Sciences & Peking Union Medical College, Beijing, China
- 3Eight-Year Medical Doctor Program, Chinese Academy of Medical Sciences & Peking Union Medical College, Beijing, China
In the rapidly evolving field of medical education, the integration of innovative technologies has become paramount to enhance the training and proficiency of future surgeons. Among these advancements, the application of 3D printing technology stands out as a useful tool in surgical training. The advantages of the 3D printing model include customization, re-usability and low-cost. The average cost of the 3D printing simulators was between $100–1000. However, there were extremely high potential labor cost during the 3D printing that hadn’t been calculated into. Additionally, in the current stage, the 3D printing simulator still have specific limitations. The most mentioned limitation was poor haptic feedback of the simulators, which was very important during the surgical training, since it is the key element for junior doctors to master practical procedures. Also, some simulators didn’t possess the integrated and elaborate structure as the human tissue, hence not the whole surgical procedures can be practiced by the trainees, and further improvement should be made. Although there are shortages, many studies have proved that 3D printing simulator can effectively reduce learning curves and is useful to enhance the trainees’ surgical skills.
1 Introduction
Three-dimensional (3D) printing technology has revolutionized our perception of how advanced technologies contribute to medical education and clinical practice. Patient-specific or personalized 3D-printed models have been increasingly used for medical applications, with research findings proving its value in different aspects. Seifert et al. (1), Nica et al. (2) and Sonkaya and Bek Kürklü (3) have conduct different 3D-printed models for oral and maxillary surgery course, Alrasheed et al. (4) and Suzuki et al. (5) developed 3D-printed models for endoscopic sinus surgery training, Barber et al. (6), Chien et al. (7), Frithioff et al. (8), Iannella et al. (9), Nguyen et al. (10) and Takahashi et al. (11) introduced various 3D-printed ear surgery simulator or 3D-printed temporal bone models, and the evaluations conducted by the studies all indicated that the 3D models were useful training/educational tool. Narayanan et al. (12) evaluated the efficacy of using 3D-printed models for endoscopic skull base surgery training, Famery et al. (13) and Vatankhah et al. (14) assessed the effectiveness of 3D-printed models for teaching ophthalmic surgeries, Nebor et al. (15), Uhl et al. (16), Zhu et al. (17), Zheng et al. (18), Encarnacion et al. (19) and Byvaltsev et al. (20) developed 3D-printed models for neurosurgery, and the studies all demonstrated the model’s effectiveness in enhancing surgical skills. Papavasiliou et al. (21) and Neijhoft et al. (22) evaluated the use of 3D-printed models for teaching orthopedic surgery, Campi et al. (23), Wong et al. (24), Reilly Scott et al. (25) and Hermans et al. (26) conducted studies of 3D-printed model for the urinary surgery training, Alshomer et al. (27) explored the use of desktop 3D printing to create an affordable training tool for microvascular anastomosis, and the approaches all represented their cost-effectiveness and helpfulness for enhancing surgical education.
In this article, we present the current 3D-printed models in surgical training. Our purpose is to demonstrate the usefulness of these models in medical education and clinical applications.
2 Search strategy
MEDLINE, EMBASE, CINAHL, ERIC, NCBI, and Web of Science were searched from Jan 1,1900 to May 30, 2024, for articles addressing 3D-printed models in surgical training. To guide our search, the search terms of “surgical training”, “3D printed model”, “curriculum”, and “education” were used combined with Boolean operators “AND”, “OR”, and “ADJACENT”. Reviews, editorials and letters would be excluded from the research. There were 58 papers founded by the search strategy, and among them, 24 papers were excluded according to the criteria of exclusion. In the end, there were 34 studies enrolled into the review.
3 The current 3D printing simulators for surgical training
3.1 3D printing simulator for oral and maxillofacial surgery
Seifert et al. (1) compared 3D-printed patient-specific models with cadaver models in an undergraduate oral and maxillary surgery course. The 3D-printed model was made to simulate the realistic extraction of the impacted third molars. Thirty-eight dental students participated, finding that 3D-printed models were significantly more realistic in anatomical accuracy and operative simulation. The 3D-printed models were also more cost-effective. However, a less fragile silicone gingiva and a better differentiation between the color of teeth and bone were the most desired suggested improvements to the 3D-printed patient individualized models. Nica et al. (2) investigated the effectiveness of using 3D-printed polymeric maxillary models in ex vivo training for dental practitioners. The technical steps of sinus augmentation and implant insertion could be performed on the corresponding 3D-printed he mi-maxillary prior to the real in vivo surgery, which included incision, flap elevation, sinus membrane elevation, flap repositioning, and suture. Results showed that the group with ex vivo training had significantly reduced operative times by 20% and similar surgical outcomes compared to the control group, demonstrating the benefit of 3D-printed models for enhancing surgical precision and efficiency. Sonkaya and Bek Kürklü (3) investigated the educational impact of a 3D-printed tooth model versus standard plastic models and extracted teeth for pre-clinical dental training. Fifty-five students rated the 3D-printed tooth model highly for its realism and usefulness in learning cavity preparation and pulp capping. The study concluded that 3D-printed models provided a cost-effective, realistic training tool, enhancing students’ practical skills before clinical practice.
3.2 3D printing simulator for surgery training in otolaryngology
3.2.1 3D-printed models for endoscopic sinus surgery training
Alrasheed et al. (4) developed and validated a 3D-printed model for endoscopic sinus surgery (ESS) training. The model was meant to reproduce a range of skills required for ESS, including injection of the middle turbinate; pledget insertion into the middle meatus; middle turbinate medialization and the identification of key landmarks (ethmoid bulla, maxillary ostia, frontal recess); uncinectomy; frontal recess dissection; and anterior ethmoidectomy. The study involved 20 participants, including rhinologists and otolaryngology residents, who completed 6 prespecified tasks including maxillary astronomy and frontal recess dissection on the simulator. Participants evaluated the model using survey ratings based on a 5-point Likert scale. All the participants felt that the simulator would be useful as a training/educational tool (4.6/5). The following responses were obtained: visual appearance 4.25/5; realism of materials 3.8/5; and surgical experience 3.9/5. Participants found the simulator to be a valuable training tool, suggesting its integration into rhinology training curricula. Suzuki et al. (5) evaluated the educational benefit of repetitive simulation training using 3D-printed sinus models for functional endoscopic sinus surgeries (FESS). The simulation training was started with uncinectomy and maxillary antrostomy, followed by anterior and posterior ethmoidectomy, sphenoidotomy, and frontal sinusotomy. Forty-seven otolaryngologists, categorized into experts, intermediates, and novices, performed FESS on the models. Their performance was assessed using The Objective Structured Assessment of Technical Skills (OSATS) scores and post-dissection CT scans. The study found significant differences in performance across experience levels, validating the model’s construct. Novices who underwent repetitive training showed marked improvement in OSATS scores and dissection quality, indicating that the 3D models effectively enhanced surgical skills.
3.2.2 3D-printed models for otologic surgical skill training
Barber et al. (6) developed a 3D-printed pediatric endoscopic ear surgery simulator to enhance surgical training. The simulator, designed using Computer Aided Design (CAD) software and fabricated with calcium sulfate hemihydrate powder, aimed to improve technical skills for trans canal endoscopic ear surgery (TEES). Six trainees tested the simulator, showing significant improvement in task completion times, indicating construct validity. The study concluded that the 3D-printed simulator is a cost-effective, high-fidelity tool for developing essential skills for TEES, demonstrating its potential for widespread use in otolaryngology training programs. Chien et al. (7) validated a 3D-printed human temporal bone model for otologic surgical skill training. The model was made to perform a series of otologic procedures, including cortical mastoidectomy, epitympanectomy, posterior tympanotomy, cochleostomy/round window surgery, canalplasty, canal wall down mastoidectomy, labyrinthectomy, and temporal bone resection. Seventeen otolaryngology trainees completed a series of otologic procedures using the model and rated its anatomical realism and training utility. Results showed that the model was highly realistic and useful for teaching temporal bone anatomy and surgical techniques. Although, the drill tone and color contrast were felt to be less realistic than cadaveric temporal bones, participants recommended incorporating the model into training curricula, indicating it as a viable alternative to cadaveric temporal bones due to its safety, accessibility, and effectiveness in developing surgical skills.
Frithioff et al. (8) investigated the effectiveness of 3D-printed temporal bone models compared to virtual reality (VR) simulation for mastoidectomy training. Eighteen otorhinolaryngology residents trained on 3D-printed models outperformed a control group that trained on VR simulations by 29% during subsequent cadaveric dissection. Although, the present models lack realistic representation of soft tissue, such as the facial nerve, dura and sigmoid sinus, this study demonstrated that 3D-printed models could improve technical skills more effectively than VR simulations, highlighting their value as a supplementary tool for traditional cadaver training. Iannella et al. (9) introduced the ‘SAPIENS’ 3D-printed temporal bone model, developed for educational and surgical simulation purposes. This model was created through a multidisciplinary collaboration and demonstrated high anatomical accuracy and it can stimulate mastoidectomy, epitympanotomy, posterior tympanotomy, and canal wall down technique. Evaluations by experienced ears, nose, and throat (ENT) surgeons indicated that the model closely resembled human temporal bone anatomy, with particular strengths in simulating mastoidectomy and middle ear dissection. Although, the 3D-printed models may not fully replicate the sensation of real tissue or bone, the questionnaire-based assessment by five experienced ENT surgeons still yielded a relatively high average total score of 49.4 ± 1.8 out of 61. The study concluded that the ‘SAPIENS’ model is a valuable tool for otologic surgery training, offering a cost-effective and realistic alternative to cadaveric bones. Nguyen et al. (10) modified a commercially available 3D-printed temporal bone model to include force sensors for stapes fixation surgery training. This study involved six junior and seven senior surgeons performing piston prosthesis placement and crimping tasks. The results showed that senior surgeons applied significantly lower forces to the stapes compared to junior surgeons, highlighting the model’s potential as a training tool to help residents self-evaluate their progress. The modified model demonstrated high fidelity in anatomical representation, offering an effective means for teaching delicate middle ear surgeries. Takahashi et al. (11) addressed the limitations of conventional 3D-printed temporal bone models by making slight manual modifications. These modifications included creating drainage holes for removing excess materials, converting ossicles ligaments to bony structures, and differentially coloring small and soft tissue structures. The model was evaluated through macroscopic and endoscopic inspections, Computed tomography (CT) imaging, and surgical performance assessments by 20 otolaryngologists. The study found that the model was highly reproducible for most anatomical structures, though the stapes, tympanic sinus, and mastoid air cells were less satisfactory. Surgeons rated the model highly for tactile sensation and anatomical accuracy, suggesting its utility for surgical training.
3.2.3 3D-printed models for skull base surgery training
Narayanan et al. (12) evaluated the efficacy of using 3D-printed models with preexisting pathology for endoscopic skull base surgery training. The study involved 15 ENT surgeons participating in a workshop where they used models created from Magnetic Resonance Imaging (MRI) and CT imaging data of a patient with platybasia and basilar invagination. The models allowed surgeons to practice complex procedures such as skull base drilling and navigation. Participants rated the models highly for their anatomical accuracy and the realism of surgical tasks, indicating that these models are valuable tools for structured, simulation-based surgical training.
3.3 3D printing simulator for ophthalmology surgery
Famery et al. (13) introduced a new wet lab model for Descemet membrane endothelial keratoplasty (DMEK) using an artificial anterior chamber equipped with a 3D-printed iris. This DMEK wet lab model offered a teaching technique that permit to perform all DMEK surgical steps. It also offered the possibility of varying the surgical difficulty by changing the anterior chamber depth. This model, tested by both beginners and experienced surgeons, allowed for the complete simulation of DMEK procedures, including graft preparation, insertion, and orientation. The study found that experienced surgeons performed significantly faster and with higher performance scores compared to beginners. The model proved to be a realistic, resource-sparing teaching tool, effectively replicating surgical steps and accommodating adjustable surgical difficulties. Vatankhah et al. (14) assessed the effectiveness of 3D-printed models for teaching orbital anatomy, anomalies, and fractures to ophthalmology residents. In a quasi-experimental study, 24 residents were divided into control and intervention groups, with the latter using 3D-printed models for training. Results indicated that the intervention group showed significant improvement in post test scores compared to the control group, particularly among first-year residents. This study highlighted the positive impact of 3D modeling on learning outcomes, demonstrating that 3D-printed models are effective tools for enhancing anatomical understanding and surgical skills.
3.4 3D printing simulator for neurosurgery
3.4.1 3D printing simulator for dural repair, cranioplasty and intracerebral hematoma (ICH) evacuation surgery
Nebor et al. (15) developed a 3D-printed model for primary dural repair via an endoscopic endonasal corridor to enhance surgical training. Using an Ultimaker 2++ printer, they created a cranial base and nasal cavity replica fitted with chicken skin to simulate dura mater. Twenty-six participants, categorized by experience levels, performed suturing tasks using the model. Most participants rated the model highly for improving technical skills and endoscopic proficiency, particularly in dural suturing. Novices showed significant improvement in task completion time, demonstrating the model’s effectiveness as a cost-efficient training tool. Uhl et al. (16) conducted a study on the use of 3D-printed models for surgical simulation of cranioplasty in craniosynostosis. Ten neurosurgeons with less than five years of experience were divided into two groups: one trained traditionally with cadaveric models and the other with 3D-printed models. The study found that surgeons using 3D-printed models showed better accuracy in bone reshaping and fixation and improved overall performance compared to those trained traditionally. Participants reported that the 3D models provided a realistic and controlled environment for practicing surgical techniques, enhancing their understanding and skills. Zhu et al. (17) developed a 3D-printed model for training endoscopic and exoscopic intracerebral hematoma (ICH) evacuation surgery using a tubular retractor. The model included a skull frame and a replaceable module filled with edible gelatin and animal blood to mimic brain tissue and hematoma. Twenty neurosurgeons and five postgraduate students participated in the training, showing significant improvements in their skills over multiple sessions. The model was rated highly for its value as a training tool (3.65/4) and realism in simulating surgical procedures. The model received good comments regarding the bone texture (mean: 3.20), the brain tissue texture (mean: 3.20), and the experience in aspirating the hematoma (mean: 3.10). This study demonstrated the model’s effectiveness in enhancing surgical skills in ICH evacuation.
3.4.2 3D printing simulator for endoscopic surgery for pituitary adenomas
Zheng et al. (18) assessed the practical value of multimaterial and multicolor 3D-printed models for training in transnasal endoscopic surgery for pituitary adenomas. The multimaterial and multicolor model was used to simulate the endoscopic procedures: the removal of elastic turbinate and nasal septum; the removal of the anterior wall of the sphenoid sinus and the exposure of tumor. The study included three types of 3D-printed models evaluated by 12 residents and 6 experienced neurosurgeons. Results showed that the multimaterial and multicolor model provided superior anatomical teaching, surgical training, and preoperative planning compared to monomaterial models. The multimaterial model offered realistic tactile feedback and enhanced understanding of anatomical relationships, demonstrating its effectiveness in improving surgical skills and planning.
3.4.3 3D printing simulator for microvascular training in neurosurgery
Encarnacion Ramirez et al. (19) developed a 3D-printed brain model with vasculature for neurosurgical procedure visualization and training. Using patient-specific MRI data, the model was constructed from silicone to replicate brain tissue and blood vessels. Seven residents and eight senior neurosurgeons tested the model, finding it anatomically precise and helpful for training in neuroendoscopic 3D perception and navigation. This study concluded that the model provides an indispensable tool for young neurosurgeons to gain operative experience safely and effectively without exposing patients to risk. Byvaltsev et al. (20) explored the use of 3D-printed cranial models to simulate operative field depth for microvascular training in neurosurgery. The study involved creating 3D-printed skulls with openings for different surgical approaches, allowing trainees to practice deep field microsurgery on chicken thighs and rat aortas. Results demonstrated the models’ effectiveness in providing realistic depth perception, hand positioning, and instrument manipulation, enhancing microsurgical training. The study concluded that these models improve training conditions, leading to better surgical accuracy and efficiency while minimizing patient risk.
3.4.4 3D printing simulator for neuroendoscopic ventricular lesion removal
Licci et al. (28) developed and validated a synthetic 3D-printed simulator for training in neuroendoscopic ventricular lesion removal. Based on patient-specific CT data, the simulator included realistic ventricular structures and a tumor model made from polyvinyl alcohol to mimic soft-consistency lesions. The 3D-printed model was produced to simulate endoscopic ultrasonic resection of the ventricular lesions using the Endoscopic Neurosurgical Pen (ENP) (Söring GmbH). The simulator was evaluated by neurosurgical trainees, who rated its anatomical realism and mechanical properties highly. The 3D-printed model could not simulate all the surgical procedures of the neuroendoscopic ventricular lesion removal, just like a frontal approach for the insertion of a ventricular drain (e.g., the skin incision, burr hole placement, and so on), albeit its elementary importance in training young neurosurgeons on a resident’s level. Even so, the results of the study still could conclude that the simulator effectively aided in developing endoscopic surgical skills, providing a valuable, low-cost, reusable training tool that could enhance procedural competence in a risk-free environment for the young neurosurgeons.
3.5 3D printing simulator for orthopedics surgery
Papavasiliou et al. (21) developed a standardized simulation training module using a 3D-printed flexor tendon model to improve surgical training for plastic surgery residents. The model was used to train a two-strand modified Kessler technique, four-strand cruciate technique, four-strand adelaide technique with an epitendinous running suture and Silfverskiöld epitendinous suture. Twenty-eight Senior House Officers participated in the study, which involved a step-ladder approach with increasing levels of difficulty. Because the tendon of the 3D printed simulation model have already been exposed and therefore the trainees could not practice on dissecting the overlying tissue (raising Brunner flaps, protecting neurovascular bundle, tendon retrieval and pulley exposure). Even so, the training was still considered with significantly improve of participants’ knowledge and practical skills. All trainees confirmed that the training module improved their confidence with flexor tendon repair. The study concluded that the 3D-printed model was an effective tool for enhancing surgical skills in tendon repair, providing a cost-effective, ethical, and reproducible training method. Neijhoft et al. (22) evaluated the use of 3D-printed models for teaching and surgery of transitional fractures of the ankle. Utilizing CT data, the models were printed and used in hands-on teaching courses. The study involved pediatric traumatology courses where fracture anatomy, surgical approaches, and screw placement were demonstrated and practiced. Participants found the models effective for their understanding of complicated fractures, allowing them to visualize and practice surgical techniques more accurately. The study concluded that 3D-printed models significantly enhanced the educational process in traumatology, offering a valuable tool for both teaching and surgical planning. Ferràs-Tarragó et al. (29) introduced a novel arthroscopy training program utilizing a 3D-printed simulator, aiming to make arthroscopy training more accessible and cost-effective. The 3D-printed model was produced to simulate the repair surgical procedure of tendon injuries. The study involved twenty first-year orthopedic residents from nine hospitals, who performed fourteen progressively challenging exercises designed to develop fundamental arthroscopic skills. The exercises were evaluated using the Arthroscopic Surgical Skill Evaluation Tool (ASSET), and results showed a mean ASSET score of 22 points. The program proved to be effective, affordable, and easy to implement, with participants reporting significant skill improvement and high satisfaction.
3.6 3D printing simulator for gastrointestinal surgery
3.6.1 3D printing simulator for conventional gastrointestinal surgery
Habti et al. (30) developed and assessed a customized 3D-printed small bowel simulator for hand-sewn anastomosis (HSBA) training. This simulator, made from silicone and a 3D-printed clamp system, was tested by 16 junior residents. The majority rated it as a good or very good tool for learning HSBA, despite some suggestions for improving the silicone’s texture. The simulator provides a cost-effective and realistic alternative for acquiring essential surgical skills.
3.6.2 3D printing simulator for laparoscopic surgery
Yang et al. (31) conducted a prospective cohort study to evaluate a 3D-printed model for laparoscopic pancreaticojejunostomy training. The surgical procedures can be stimulated in the study including the classic biliary anastomosis and Cattell-Warren pancreaticointestinal anastomosis. Sixteen surgeons with varying experience levels participated, showing that those with higher seniority performed better initially. Although the stimulation of the pancreatic duct was considered as not realistic enough, the stepwise training program, starting with laparoscopic biliary-enteric anastomosis, indeed improved residents’ performance. The study demonstrated that repeated training with this model enhances operative skills, providing a valuable tool for mastering complex procedures in a controlled environment. Xia et al. (32) investigated a 3D-printed model for training in laparoscopic intracorporeal intestinal anastomosis (LIIA). The study aimed to design a cost-effective, reusable model and evaluate its authenticity and validity. Fifteen surgeons were divided into expert, intermediate, and novice groups to perform LIIA using the model. The results showed significant differences in performance scores and operation times across the groups, with repeated training improving the skills of novices to the level of untrained intermediates. The five experts rated the face and content validity of the model very high, with the median being four out of five. Although the intestine used in the experiment was single-layered, and it failed to simulate the actual structure of the human intestine, which is multilayered, the model still demonstrated excellent face, content, and construct validity, suggesting its potential as an effective training tool for LIIA. Xia et al. (33) designed and evaluated a portable 3D-printed model for laparoscopic choledochojejunostomy (LCJ) training. The study assessed the model’s face, content, and construct validity using the objective structured assessment of technical skills (OSATS). The operating space score of the model was 4.83 ± 0.41 points. The impression score of bile duct was 4.33 ± 0.52. The tactile sensation score of bile duct suture was 4.00 ± 0.63 and 3.83 ± 0.41points, respectively. Fifteen surgeons of varying experience levels participated, and results indicated significant differences in OSATS scores and operation times among groups. Repeated training improved residents’ performance, indicating the model’s potential for enhancing surgical skills and reducing patient risk. The current model could not contain blood vessels or simulation of intraoperative bleeding, which will more perfectly mimic the real surgery situation. Even so, this 3D-printed LCJ model offered a cost-effective, realistic training tool for biliary surgery. Holt et al. (34) developed and evaluated a 3D-printed endoscopic ampullectomy training model to improve the technical skills, knowledge, and confidence of endoscopists. The model included a reusable silicone stomach-duodenum, an ampullary mount, and a base to stabilize the duodenum. The 3D-printed endoscopic ampullectomy training model could stimulate the core procedural steps of endoscopic ampullectomy, including passing an ERCP endoscope through the pylorus to the ampulla, identification of the ampulla, positioning the snare over the ampulla, and performing snare resection, specimen retrieval, pancreatic duct cannulation, and pancreatic stent insertion. Sixteen endoscopists tested the prototype, performing core procedural steps such as ampulla identification and pancreatic duct cannulation. The model received mean technical and visual realism scores of 3.1 and 3.2, respectively, on a 1–5 scale. Although the developers identified a limitation with using a rigid base and ampulla mount in combination with a flexible stomach-duodenum because there was potential for the 2 to separate during endoscope maneuvering, the participants reported increased confidence and knowledge after using the model, indicating its potential effectiveness for training in this high-risk procedure.
3.7 3D printing simulator for urinary surgery
Campi et al. (23) developed and tested the RAKT Box, the first entirely 3D-printed, perfused simulator for vascular anastomoses during robot-assisted kidney transplantation (RAKT). The trainees were able to perform both the arterial and venous anastomosis on the 3D-printed simulator, which were the key steps of the kidney transplantation surgery. This study involved a multidisciplinary team and a three-year iterative development process. The simulator includes a fixed model of the human pelvis, disposable iliac vessels, and a kidney model with a hydraulic circuit to simulate arterial and venous blood flow. Although, the 3D-printed simulator could not allow simulation of ureterovesical anastomosis, while ureterovesical anastomosis is a key step in kidney transplantation, the great evaluation was still given by the users. It was tested by an expert RAKT surgeon and four trainees, demonstrating reliability and effectiveness in training key surgical steps. The study concluded that the RAKT Box was a valuable educational tool for developing RAKT-specific skills and could serve as the foundation for a structured RAKT surgical curriculum. Wong et al. (24) detailed the evolution and use of a 3D-printed bladder model for teaching urethrovesical anastomosis (UVA) during minimally invasive radical prostatectomy. Early versions were made of latex and silicone, but the current model is produced using computer-aided design and 3D printing with a polymer that simulated human bladder tissue. The model was used within a realistic pelvic silicone torso to emulate surgical conditions. It has been shown to effectively transfer skills from virtual reality robotic simulators to actual robotic surgical systems, making it a valuable tool for training residents in a controlled, risk-free environment. Reilly Scott et al. (25) investigated the use of individualized 3D-printed models for trainee and patient education, and surgical planning in robotic partial nephrectomies (RAPN). All RAPN were performed by one of six attending surgeons using a Da Vinci surgical system® (Intuitive Surgical, Sunnyvale, CA) via a transperitoneal or retroperitoneal approach. The study included 40 patients and surveyed patients and surgical teams before and after exposure to the 3D models. Results indicated significant improvements in patient understanding of kidney anatomy, their disease, the surgical procedure, and associated risks after viewing the models. Surgical team members, including residents and fellows, reported increased confidence in their surgical plans. Additionally, using the models resulted in better operative outcomes, including reduced warm ischemia time and shorter hospital stays. However, the authors pointed that because computer algorithms nowadays have not yet advanced enough to control the 3D printer based on simply identifying the target lesion on both CT and MRI imaging, the interpretation of the imaging and subsequent segmentation required the cooperation of radiologist and trained printing technician, which indicated high cost of human labor and would be an essential limitation of the 3D-printed model. Hermans et al. (26) validated a 3D-printed silicone renal tumor model for RAPN training. Surgical systems used were the Da Vinci Si, X and Xi (Intuitive Surgery) with training instruments and a 30° camera, using only three arms. The study involved 36 participants with varying experience levels who performed robotic tumor excisions on the models. The results demonstrated that experts performed significantly better than novices in terms of surgical margins, excision time, preserved parenchyma, and Global Evaluative Assessment of Robotic Skills (GEARS) scores. Expert group survey results showed an average score of 6.3/10 on realism of the model, 8.2/10 on the usefulness as training model and 6.9/10 score on the usefulness as an evaluation tool. The model was rated high for realism and training utility, confirming its face and content validity. The study concluded that the 3D-printed model was a valuable tool for training and assessing RAPN skills.
3.8. 3D printing simulator for vascular surgery
Alshomer et al. (27) explored the use of desktop 3D printing to create an affordable training tool for microvascular anastomosis. The model featured multiaxial and multiangular vessel orientations, designed with CAD software and printed using a thermoplastic polyurethane filament. Trials showed that the model provided realistic training scenarios, significantly aiding in the development of microsurgical skills. This approach represented a cost-effective and versatile method for enhancing microsurgical education.
The current 3D printing simulators for surgical training are listed in the following Tables 1–4.
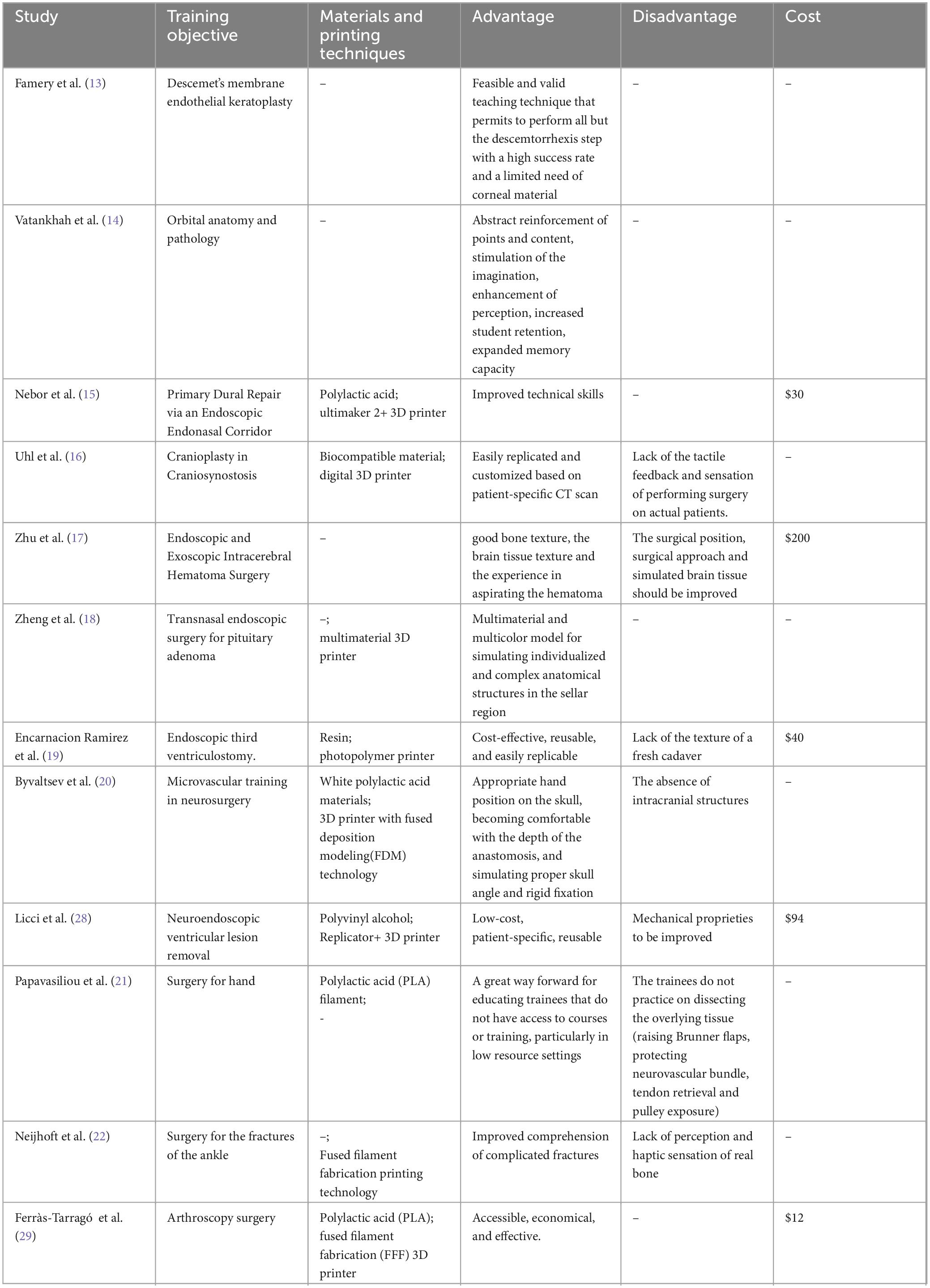
Table 2. The list of the current 3D printing simulator for ophthalmology, neurosurgery and orthopedics surgery.
4. Evaluation of the 3D printing simulator
4.1 Cost-effectiveness of the 3D printing simulator
In the studies, the cost of the 3D printing simulators seemed generally inexpensive. As mentioned by the studies, the lowest-cost 3D printing simulator was 3D-printed teeth with the cost of $0.60 and the most expensive simulator was 3D-Printed Training Model for Robot-assisted Kidney Transplantation with the cost of € 5,000. The average cost of the 3D printing simulators was between $30–300. However, as the studies described, there were extremely high potential labor cost during the 3D printing that hadn’t been calculated into. Frithioff et al. (8) mentioned that it took approximately 16 h to print a single temporal bone model, excluding time for maintenance of the printer. Iannella et al. (9) mentioned that printing and post-printing processes took 3 h and 1 h, respectively, for a total of 4 h required to produce each single model. Encarnacion Ramirez et al. (19) mentioned that for the time of segmentation, modeling in several iterations, and preparation of the prototype model for printing, they estimated approximately 12–25 h of working time. Reilly Scott et al. (25) mentioned that the average time their model took was 8 h to print (range 3–10 h), which was usually accomplished overnight. Licci et al. (28) mentioned that approximately 10–15 h of working time were estimated for the printing of the simulator. Therefore, although the direct cost of the 3D printing simulators was inexpensive, the overall indirect cost was hardly estimable which we should notice.
4.2 Limitations of the 3D printing simulator
The advantages of the 3D printing model were commonly acknowledged, including that customized, low-cost, patient-specific, reusable (16, 19, 25, 26, 28–30). However, in the current stage, the 3D printing simulator still have specific limitations. The most mentioned limitation was poor haptic feedback of the simulators (1, 9, 10, 16, 19, 22, 30), which was very important during the surgical training, since it is the key element for junior doctors to master practical procedures. Additionally, some simulators didn’t possess the integrated and elaborate structure as the human tissue, hence not the whole surgical procedures can be practiced by the simulators, and further improvement should be made (6, 8, 11, 17, 20, 21).
4.3 Surgical training curriculum with the use of 3D printing simulators
According to the review, we concluded some principles of 3D stimulator training curriculum. First, generalized simulators were usually used for medical students, aiming to give pre-clinical education and train their surgical skill. The customized simulators were usually used by surgeons for specific procedure training or pre-operative training. Second, subjective questionnaires were most commonly used to evaluate the generalized simulators by the medical students (1, 3, 7, 9, 10). However, there was no scale tool for the medical students to evaluate their understandings and surgical skills by the use of 3D stimulators. But, for the advanced and customized simulators, specific designed scale tools were usually used to evaluate the surgical capability of the surgeons, which can help them understand their weaknesses and improve their surgical skills better. Suzuki et al. (5) used the objective structured assessment of technical skills (OSATS) to evaluate the trainees’ performance in the functional endoscopic sinus surgery training curriculum by the use of 3D printing simulator. Licci et al. (28) used the global evaluative assessment of robotic skills (GEARS) and assessment of robotic console skills (ARCS) tools to evaluate the trainees’ performance in the robot-assisted kidney transplantation training curriculum by the use of 3D printing simulator. Campi et al. (23) used the modified anastomosis objective structured assessment of technical skills (MAOSATS) to evaluate the trainees’ performance in the laparoscopic intracorporeal intestinal anastomosis training curriculum by the use of 3D printing simulator. Hermans et al. (26) used the arthroscopic surgical skill evaluation tool (ASSET) to evaluate the trainees’ performance in the arthroscopy training curriculum by the use of 3D printing simulator. Besides, operative time was the most commonly used objective evaluation tool to evaluate the surgical capability of the surgeons (4, 6, 13, 15, 28, 32). In conclusion, generalized simulators can be used as teaching tools for medical students to have pre-clinical education and customized simulators can be used by surgeons for specific procedure training or pre-operative training. In the matter of assessments, specific scale tools and operative time are good methods to evaluate the surgical capability of the trainees in the 3D printing simulator curriculum, especially for the advanced and customized 3D printing surgical simulators.
5 Discussion
The application of 3D printing simulator in surgical training has been transformative, offering numerous benefits and opportunities for improving medical education and patient outcomes. 3D printing simulator can replicate the exact anatomical structures of patients, providing highly realistic models for surgical training. They offer a tangible and accurate representation of complex anatomies, which is crucial for understanding spatial relationships and practicing surgical techniques. Practicing on 3D printing simulator could reduce the risks associated with the first-time performance of new or intricate surgical procedures on actual patients.
Also, according to the cost-effectiveness, traditional surgical training methods, such as cadaveric dissection or live animal models, can be expensive and logistically challenging. 3D printing offers a more cost-effective and accessible alternative, with reusable and reproducible models.
Surgeons can use 3D printing simulator to plan and rehearse surgeries, leading to more precise and efficient procedures. Medical students and residents can practice basic and advanced surgical skills on 3D printing simulator, allowing for a safer and more effective learning curve.
However, there are specific barriers hindering the widespread adoption of 3D printed models in certain surgical fields. Some of the key barriers include: (1) Complexity of Anatomy: In certain surgical fields where the anatomy is highly complex or variable, creating accurate and detailed 3D printed models can be challenging. The intricate structures and variations in anatomy may require more advanced imaging techniques and expertise to generate precise models. (2) Cost and Resources: The cost associated with acquiring and maintaining 3D printing technology, as well as the resources required for creating high-quality models, can be a significant barrier. Not all medical institutions may have the budget or infrastructure to invest in 3D printing capabilities. (3) Training and Expertise: Utilizing 3D printed models effectively in surgical training requires training and expertise in both the creation of the models and their integration into educational curricula. Lack of training programs and expertise in this area can limit the adoption of 3D printed models in certain fields. (4) Standardization and Validation: The lack of standardized protocols for creating and validating 3D printed models for surgical training purposes can also be a barrier. Without established guidelines and quality control measures, there may be concerns about the accuracy and reliability of the models.
Addressing these barriers through increased investment in technology, training programs, and standardization efforts can help overcome the challenges and facilitate the wider integration of 3D printed models in all fields of surgical training.
6 Conclusion
Overall, 3D printing simulator have revolutionized surgical training, offering a realistic, customizable, and cost-effective method for preparing surgeons. As technology advances, their application will continue to expand, further enhancing the quality of surgical education and patient care.
Author contributions
YJ: Writing – original draft. HJ: Writing−review and editing. ZY: Writing – review and editing. YL: Writing – review and editing, Writing – original draft.
Funding
The author(s) declare no financial support was received for the research, authorship, and/or publication of the article.
Conflict of interest
The authors declare that the research was conducted in the absence of any commercial or financial relationships that could be construed as a potential conflict of interest.
Publisher’s note
All claims expressed in this article are solely those of the authors and do not necessarily represent those of their affiliated organizations, or those of the publisher, the editors and the reviewers. Any product that may be evaluated in this article, or claim that may be made by its manufacturer, is not guaranteed or endorsed by the publisher.
References
1. Seifert LB, Schnurr B, Herrera-Vizcaino C, Begic A, Thieringer F, Schwarz F. 3D-printed patient individualised models vs cadaveric models in an undergraduate oral and maxillofacial surgery curriculum: Comparison of student’s perceptions. Eur J Dent Educ. (2020) 24:799–806.
2. Nica DF, Gabor AG, Duma V-F, Tudericiu VG, Tudor A, Sinescu C. Sinus lift and implant insertion on 3d-printed polymeric maxillary models: Ex vivo training for in vivo surgical procedures. J Clin Med. (2021) 10:1–15. doi: 10.3390/jcm10204718
3. Sonkaya E, Bek Kürklü ZG. Comparisons of student comprehension of 3D-printed, standard model, and extracted teeth in hands-on sessions. Eur J Dent Educ. (2023) 28:452–60.
4. Alrasheed AS, Nguyen LHP, Mongeau L, Funnell WRJ, Tewfik MA. Development and validation of a 3D-printed model of the ostiomeatal complex and frontal sinus for endoscopic sinus surgery training. Int Forum Allergy Rhinol. (2017) 7:837–41.
5. Suzuki M, Miyaji K, Watanabe R, Suzuki T, Matoba K, Nakazono A, et al. Repetitive simulation training with novel 3D-printed sinus models for functional endoscopic sinus surgeries. Laryngosc Invest Otolaryngol. (2022) 7:943–54. doi: 10.1002/lio2.873
6. Barber SR, Kozin ED, Dedmon M, Lin BM, Lee K, Sinha S, et al. 3D-printed pediatric endoscopic ear surgery simulator for surgical training. Int J Pediatr Otorhinolaryngol. (2016) 90:113–8. doi: 10.1016/j.ijporl.2016.08.027
7. Chien WW, da Cruz MJ, Francis HW. Validation of a 3D-printed human temporal bone model for otology surgical skill training. World J Otorhinolaryngol Head Neck Surg. (2021) 7:88–93. doi: 10.1016/j.wjorl.2020.12.004
8. Frithioff A, Frendø M, Weiss K, Foghsgaard S, Pedersen DB, Sørensen MS, et al. Effect of 3D-printed models on cadaveric dissection in temporal bone training. OTO Open. (2021) 5:1–7. doi: 10.1177/2473974X211065012
9. Iannella G, Pace A, Mucchino A, Greco A, De Virgilio A, Lechien JR, et al. A new 3D-printed temporal bone: ‘the SAPIENS’—specific anatomical printed-3D-model in education and new surgical simulations. Eur Arch Oto Rhino Laryngol. (2024) 281:405–14. doi: 10.1007/s00405-024-08645-6
10. Nguyen Y, Mamelle E, De Seta D, Sterkers O, Bernardeschi D, Torres R. Modifications to a 3D-printed temporal bone model for augmented stapes fixation surgery teaching. Eur Arch Oto Rhino Laryngol. (2017) 274:2733–9. doi: 10.1007/s00405-017-4572-1
11. Takahashi K, Morita Y, Ohshima S, Izumi S, Kubota Y, Yamamoto Y, et al. Creating an optimal 3D printed model for temporal bone dissection training. Ann Otol Rhinol Laryngol. (2017) 126:530–6. doi: 10.1177/0003489417705395
12. Narayanan V, Narayanan P, Rajagopalan R, Karuppiah R, Rahman ZAA, Wormald P-J, et al. Endoscopic skull base training using 3D printing simulator with pre-existing pathology. Eur Arch Oto Rhino Laryngol. (2015) 272:753–7. doi: 10.1007/s00405-014-3300-3
13. Famery N, Abdelmassih Y, El-Khoury S, Guindolet D, Cochereau I, Gabison EE. Artificial chamber and 3D printed iris: A new wet lab model for teaching Descemet’s membrane endothelial keratoplasty. Acta Ophthalmol. (2019) 97:e179–83. doi: 10.1111/aos.13852
14. Vatankhah R, Emadzadeh A, Nekooei S, Tafaghodi Yousefi B, Khadem Rezaiyan M, Karimi Moonaghi H, et al. 3D printing simulator for teaching orbital anatomy, anomalies and fractures. J Ophthalm Vis Res. (2021) 16:611–9. doi: 10.18502/jovr.v16i4.9751
15. Nebor I, Hussein AE, Montemagno K, Fumagalli R, Labiad I, Xu A, et al. primary dural repair via an endoscopic endonasal corridor: Preliminary development of a 3D-printed model for training. J Neurol Surg Part B Skull Base. (2022) 83:e260–5. doi: 10.1055/s-0041-1725027
16. Uhl JF, Sufianov A, Ruiz C, Iakimov Y, Mogorron HJ, Encarnacion Ramirez M, et al. The use of 3D printing simulator for surgical simulation of cranioplasty in craniosynostosis as training and education. Brain Sci. (2023) 13:894. doi: 10.3390/brainsci13060894
17. Zhu J, Wen G, Tang C, Zhong C, Yang J, Ma C. A practical 3D-printed model for training of endoscopic and exoscopic intracerebral hematoma surgery with a tubular retractor. J Neurol Surg Part A Cent Eur Neurosurg. (2020) 81:404–11. doi: 10.1055/s-0039-1697023
18. Zheng J-P, Li C-Z, Chen G-Q. Multimaterial and multicolor 3D-printed model in training of transnasal endoscopic surgery for pituitary adenoma. Neurosurg Focus. (2019) 47:E21. doi: 10.3171/2019.6.FOCUS19294
19. Encarnacion Ramirez M, Ramirez Pena I, Barrientos Castillo RE, Sufianov A, Goncharov E, Soriano Sanchez JA, et al. Development of a 3D printed brain model with vasculature for neurosurgical procedure visualisation and training. Biomedicines. (2023) 11:1–10. doi: 10.3390/biomedicines11020330
20. Byvaltsev V, Polkin R, Bereznyak D, Giers MB, Hernandez PA, Shepelev V, et al. 3D-printed cranial models simulating operative field depth for microvascular training in neurosurgery. Surg Neurol Int. (2021) 12:1–10. doi: 10.25259/SNI_849_2020
21. Papavasiliou T, Nicholas R, Cooper L, Chan JCY, Ibanez J, Bain CJ, et al. Utilisation of a 3D printed ex vivo flexor tendon model to improve surgical training. J Plast Reconstruct Aesth Surg. (2022) 75:1255–60. doi: 10.1016/j.bjps.2021.11.027
22. Neijhoft J, Henrich D, Mörs K, Marzi I, Janko M. Visualization of complicated fractures by 3D-printed models for teaching and surgery: Hands-on transitional fractures of the ankle. Eur J Trauma Emerg Surg. (2022) 48:3923–31. doi: 10.1007/s00068-022-01879-1
23. Campi R, Pecoraro A, Vignolini G, Spatafora P, Sebastianelli A, Sessa F, et al. The first entirely 3D-printed training model for robot-assisted kidney transplantation: The RAKT box. Eur Urol Open Sci. (2023) 53:98–105. doi: 10.1016/j.euros.2023.05.012
24. Wong NC, Hoogenes J, Guo Y, Quantz MA. Techniques: Utility of a 3D printed bladder model for teaching minimally invasive urethrovesical anastomosis. Can Urol Assoc J. (2017) 11:E321–2. doi: 10.5489/cuaj.4262
25. Reilly Scott E, Singh A, Quinn AM, Morano S, Karp A, Boyd K, et al. The use of individualized 3D-printed models on trainee and patient education, and surgical planning for robotic partial nephrectomies. J Robot Surg. (2023) 17:465–72. doi: 10.1007/s11701-022-01441-6
26. Hermans T, Snoeks JM, vom Dorp F, Wiesner C, Steiner T, von Rundstedt F-C. Validation of a 3D-printed robot-assisted partial nephrectomy training model. BJUI Compass. (2023) 5:90–100. doi: 10.1002/bco2.269
27. Alshomer F, Alhazmi B, Alowais F, Aldekhayel S. A low-cost 3D-printed tool with multiaxial/angular vessel orientation for microvascular anastomosis training. Plast Reconstruct Surg Glob Open. (2020) 8:e2567. doi: 10.1097/GOX.0000000000002567
28. Licci M, Thieringer FM, Guzman R, Soleman J. Development and validation of a synthetic 3D-printed simulator for training in neuroendoscopic ventricular lesion removal. Neurosurg Focus. (2020) 48:E18. doi: 10.3171/2019.12.FOCUS19841
29. Ferràs-Tarragó J, Jover-Jorge N, Miranda-Gómez I. A novel arthroscopy training program based on a 3D printed simulator. J Orthop. (2022) 32:43–51. doi: 10.1016/j.jor.2022.04.006
30. Habti M, Bénard F, Arutiunian A, Bérubé S, Cadoret D, Meloche-Dumas L, et al. Development and learner-based assessment of a novel, customized, 3D printed small bowel simulator for hand-sewn anastomosis training. Cureus. (2021) 13:e20536. doi: 10.7759/cureus.20536
31. Yang J, Luo P, Wang Z, Shen J. Simulation training of laparoscopic pancreaticojejunostomy and stepwise training program on a 3D-printed model. Int J Surg. (2022) 107:106958. doi: 10.1016/j.ijsu.2022.106958
32. Xia J, Wu J, Chen H, Mao J, Xu X, Zhang J, et al. Assessment of laparoscopic intracorporeal intestinal anastomosis training using simulation-based 3D printing simulator: Exploring surgical performance and learning curves. Int J Surg. (2023) 109:2953–61. doi: 10.1097/JS9.0000000000000582
33. Xia J, Mao J, Chen H, Xu X, Zhang J, Yang J, et al. Development and evaluation of a portable and soft 3D-printed cast for laparoscopic choledochojejunostomy model in surgical training. BMC Med Educ. (2023) 23:77. doi: 10.1186/s12909-023-04055-0
Keywords: 3D printed, surgical training, medical education, simulator, advances
Citation: Jiang Y, Jiang H, Yang Z and Li Y (2024) The current application of 3D printing simulator in surgical training. Front. Med. 11:1443024. doi: 10.3389/fmed.2024.1443024
Received: 05 June 2024; Accepted: 05 August 2024;
Published: 29 August 2024.
Edited by:
Te-Chuan Chen, National Sun Yat-sen University, TaiwanReviewed by:
Wei-Chih Chen, Chang Gung Memorial Hospital, TaiwanGionata Fragomeni, Magna Græcia University, Italy
Copyright © 2024 Jiang, Jiang, Yang and Li. This is an open-access article distributed under the terms of the Creative Commons Attribution License (CC BY). The use, distribution or reproduction in other forums is permitted, provided the original author(s) and the copyright owner(s) are credited and that the original publication in this journal is cited, in accordance with accepted academic practice. No use, distribution or reproduction is permitted which does not comply with these terms.
*Correspondence: Zhikun Yang, yangzhikun@pumch.cn; Ying Li, liying6007@pumch.cn
†These authors have contributed equally to this work