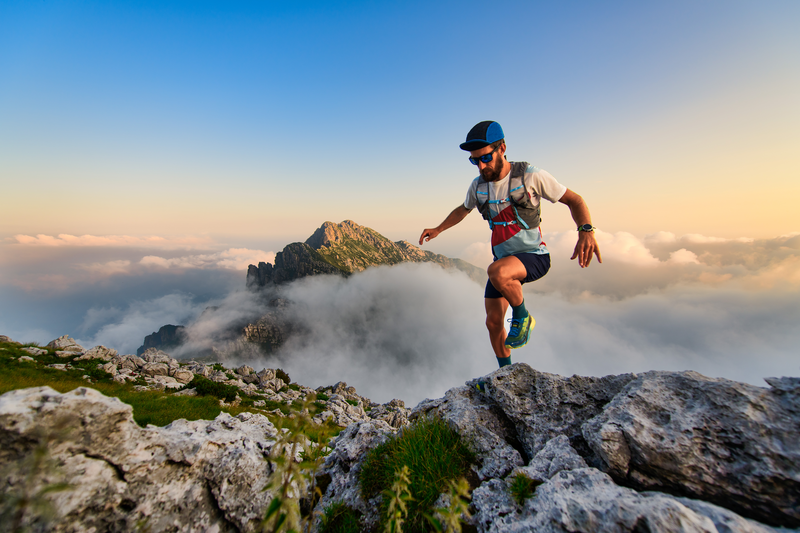
94% of researchers rate our articles as excellent or good
Learn more about the work of our research integrity team to safeguard the quality of each article we publish.
Find out more
ORIGINAL RESEARCH article
Front. Med. , 04 September 2024
Sec. Obstetrics and Gynecology
Volume 11 - 2024 | https://doi.org/10.3389/fmed.2024.1439181
Objectives: Pregnant women are at increased risk for severe SARS-CoV-2 infection and adverse neonatal outcome, primarily preterm birth and stillbirth. Our study aimed to investigate to which extent SARS-CoV-2 affects placental tissue and if viral replication within the placenta is evident, thus if there is a correlation between placental damage and adverse pregnancy outcome such as stillbirth.
Methods: We prospectively collected placentas from 61 SARS-CoV-2 infected pregnant women and 10 controls. Histopathological, immunohistochemical, and in situ hybridization studies were performed on all placentas with antibodies for SARS-CoV-2 proteins, ACE2, various immune cells, and inflammatory markers or probes for SARS-CoV-2 genes and an antisense strand.
Results: The measured scores of SARS-CoV-2 glycoprotein, nucleocapsid, and antisense strand indicating replication correlated with both the severity of maternal symptoms and presence of stillbirth. Specifically, 15/61 placentas exhibited replication, while the three cases with stillbirth had high or maximal replication scores. ACE2-H-score was significantly higher in COVID-19 patients, while the expression of various immune cells did not differ statistically. In multivariate analysis, presence of maternal comorbidities correlated with presence of severe COVID-19 infection.
Conclusion: We report evidence of active in vivo SARS-CoV-2 replication in the placenta after maternal infection in pregnancy in a case–control setting in a large population. Intensity of placental viral replication as well as viral levels were higher in women with severe or critical COVID-19 disease, supporting the rationale that severity of maternal SARS-CoV-2 infection could correlate with the severity of placentitis. Replication was maximal in cases of stillbirth, which suggests direct placental involvement in the pathophysiology of this dramatic outcome. Continuing to advocate for preventive measures against COVID-19 during pregnancy, including (re)vaccination, as well as appropriately counseling women with diagnosed infection, are of utter importance.
Pregnancy increases the risk for a severe course of COVID-19 disease (1). Whereas the pathophysiology of maternal complications is well described, the mechanisms leading to poor fetal outcome remain poorly understood (2, 3).
Several publications noted evidence of the triad chronic histiocytic intervillositis (CHI), massive perivillous fibrin deposition (MPFD), and trophoblast necrosis (TN) in association with COVID-19 miscarriage and stillbirth, in some cases being present in completely asymptomatic women (4–13). These lesions, especially if occurring together (CHI, MPFD, and TN), appear to be typical for SARS-CoV-2 and can represent the underlying cause for acute placental insufficiency and fetal demise, yet as separate entities they can also be part of an alloimmune maternal response of different etiology (5–13).
Given the inconsistency of histopathological data, a variety of investigations have been previously carried out by various research groups, leading to a paucity of publications, generally small case series or dispersed case reports, with often conflicting results. For example, whereas one study reported 100% presence of SARS-CoV-2 spike glycoprotein in the analyzed placentas, others report either no evidence of viral RNA in their cohorts, or in a significant smaller number of cases (14–17).
Furthermore, there is a great difference in severity of placental infection and pregnancy outcome related to the various serotypes. For example, the ancestral and the Omicron serotypes caused less placentitis and less intrauterine deaths, as opposed to delta, as shown in a recent registry study (18). This could partially explain the inconsistency of results originating from small cohorts. In December 2021, a panel of experts released the first standardized definition of placental infection with SARS-CoV-2, in an attempt to minimize misclassification. According to these, confirmation of definitive placental infection requires evidence of active viral replication in placental tissue, whereas the sole evidence of viral RNA or protein in placental tissue can only provide evidence of probable transmission (19). Furthermore, according to consensus, evidence of replication should always include appropriate negative controls (3).
In situ hybridization (ISH) and/or placental RT-PCR are recommended for diagnosis of transplacental transmission, as these methods appear to be superior to placental swab PCR testing, immunohistochemistry (IHC), and electronic microscopy (EM) for this purpose (19, 20).
These insights underline the necessity for large, well-conducted case–control studies to address the extent of placental SARS-CoV-2 infection and its impact on pregnancy outcomes, by following current standards of investigation and scientific reporting.
We hypothesized that SARS-CoV-2 replicates in the placenta of infected mothers. We aimed to conduct a prospective study in a SARS-CoV-2 infected pregnant population and to perform histopathological analysis, PCR-testing, IHC and ISH at placental level, and assess the extent of damage, as well as vertical transmission and the immune response at the maternal-fetal interface, compared to corresponding controls. Furthermore, we intended to investigate if SARS-CoV-2 viral replication within the placenta is evident, and if there is a correlation between placental damage and adverse pregnancy outcome such as stillbirth.
We performed a nested case–control study, including 61 cases and 10 controls in our analysis. Cases included all women diagnosed with SARS-CoV-2 infection during pregnancy, irrespective of disease severity or gestational age at infection, who were managed at our tertiary referral hospital between May 2020 to October 2022, and who provided written participation consent.
Controls were selected from a population without SARS-CoV-2 infection during pregnancy in the predefined study period. Selection occurred based on gestational age at delivery and comorbidities, which could have influenced outcomes in a similar manner to SARS-CoV-2. We chose a nested case–control design in order to reduce costs and efforts of data collection and analysis.
Several cases were highlighted. For a simplified presentation, these were annotated with the symbol “i” for cases (i = infected) and “c” for controls (c = control).
Data were collected prospectively. Diagnosis of infection was made by SARS-CoV-2-PCR in a nasopharyngeal swab.
Beta B.1.351 variant was dominant in Switzerland until the beginning of May 2021, followed by Gamma P.1 for a period of 2 weeks, when Delta B.1.617.2 took over and continued to be dominant until mid-December 2021. Ever since, Omicron B.1.1.529 was the dominant variant in our country.
Serotype analysis was only conducted in one single placenta, more specific in Case 2i, where stillbirth occurred at 30 weeks of gestation. This case occurred at the very beginning of the Omicron outbreak, and serotype was analyzed as an exception in this case, because little was known about the new variant (i.e., Omicron), and the treating clinicians suspected high virulence. However, the affected case was ultimately infected with Delta.
The Cantonal Ethics Committee in Bern has approved conduction the study (registration-number BASEC 2020-02610). No external funding was received for the study.
A piece of tissue collected from the maternal side of the placenta was fixed with 4% paraformaldehyde for 30–36 h (h) at 4°Celsius (C) immediately after birth. It was then embedded in paraffin, and sections were cut into 6 μM pieces using the HM340E-microtome.
Placental sections were deparaffinized and rehydrated, then blocked with 10% goat serum, and 1% bovine serum albumin (BSA) in TBS for 1 h at room temperature. Subsequently, sections were incubated overnight at 4°C with following primary antibodies: CD3/CD8/CD68/CD86,/CD11b,/TNFa/ACE2,/SARS-CoV-2-nucleocapsid and SARS-CoV-2-spike-glycoprotein. After washing, sections were blocked with peroxidase for 15 min and subsequently incubated with peroxidase-labeled secondary antibody followed by diaminobenzamidine.
RNAscope® fluorescent in situ hybridization was conducted on formalin-fixed, paraffin-embedded placentas using the RNAscope® 2-Plex Detection Kit (Chromogenic) according to the manufacturer’s protocol for SARS-CoV-2 Antisense strand of the orf1ab gene, SARS-CoV-2 S gene encoding the spike protein and IL6.
ACE2 staining was quantified for immunoreactivity using Qupath. Histological (H) score calculated by multiplying the percentage of stained placental tissue (decidua and/or villi) by the average staining intensity (between 0 and 3). The program outputs the total number of detected cells, which is used to calculate the H-score. H-scores can vary from 0 (no expression) to 300 (intensity of staining 3 in 100% of the cells analyzed).
For CD3/CD8/CD68/CD86/TNFa/CD11b-staining, hotspot areas were identified.
The method of the immunoreactive score (IRS; 0–1 = negative, 2–3 = mild, 3–8 = moderate, 9–12 = strongly positive) was used for all SARS-CoV-2 and IL6 staining by multiplying the percentage of positive cells (0 = no positive cells, 1 = <10%, 2 = 10–50%, 3 = 51–80%, 4 = >80% positive cells, respectively) and the staining intensity (0 = no color reaction, 1 = mild, 2 = moderate, 3 = intense reaction, respectively).
The researchers analyzing the placentas were blinded to the group. Data were analyzed by at least two persons in every case.
Mean values and standard deviation (SD) were calculated for continuous variables and percentages for qualitative variables. A student t-test and Fisher’s exact test were used for continuous parametric variables and binary variables, respectively. Multiple linear regression was performed to analyze risk factors for presence of severe or critical COVID-19 illness, respectively risk factors for presence of viral replication in the placenta. Logistic regression analysis was performed to identify if the time point of infection was associated with presence of severe COVID-19 disease. Significance was set at a p value of <0·05. Statistical analysis was carried out with RStudio for Windows.
Altogether, 41/71 (57.74%) cases were recruited in the pre-Delta period, 18/71 (25.35%) during Delta and 11/71 (15.49%) during Omicron dominance. As mentioned before, serotype analysis was only conducted in one single placenta, more specific in Case 2i.
88.5% (54/61) of the cases suffered from asymptomatic, mild/moderate SARS-CoV-2 infection, according to the National Institutes of Health (NIH) criteria for severity of the disease (20). 11·47% (7/61) presented with severe or critical illness and were managed as inpatient. 9.83% (6/61) required intensive care unit (ICU) admission and ventilation and consequently underwent emergency delivery.
4/41 (9.75%) cases recruited in the pre-Delta period presented with severe or critical illness, versus 3/18 (16.66%) cases during Delta dominance, versus no case during the Omicron phase. The difference between the rate of severe or critical cases between the pre-Delta and Delta periods was not statistically significant (p = 0.44, chi-squared test).
No maternal deaths were recorded. In two women with severe/critical illness (28.57%, 2/7), infection occurred in the second trimester of pregnancy (median [range] gestational age = 18 [17–19]). In the remaining 5/7 (71.42%), infection occurred in the third trimester of pregnancy (median [range] gestational age = 30 [29–32]). Further patient characteristics and outcomes are depicted in Table 1.
Time point of infection is depicted in Figure 1. Logistic regression showed no correlation between time point of infection and presence of severe/critical illness (p = 0.4497).
ACE2 H-score was significantly higher in cases versus controls (Figure 2B). If analyzed separately in the decidua, mean ACE2 H-score was 60.23 (±SD 44.02) in cases versus 25.06 (±SD 11.84) in controls (p = 0.0149) (Figure 2). Reversely, ACE2 H-Score in the villi was not significantly different between cases and controls (p ≥ 0.05). ACE2 H-score was significantly higher if infection occurred in the first half of pregnancy (p = 0.0052).
Figure 2. Quantification of SARS-CoV-2 specific proreins and virus replication in mild/moderate and severe/critical COVID-19 cases. IRS score for (A) the spike glycoprotein (p = 0.0013) and (B) the nucleocapsid protein (p = 0.03) is significantly higher in severe or critical cases (pink) compared to mild or moderate cases (blue). (C) A significantly higher IRS score (p = 0.034) for the virus replication was detected in severe or critical cases. Data are represented as mean ± SD.
Expression of CD3/CD8/CD11b/CD68/CD86 was not statistically different between cases and controls (Table 2; Figures 3A–H). Furthermore, expression of TNFa and IRS for IL6 were not significantly different between the two groups (p = 0.22 and p = 0.62, respectively) (Figures 3I,J).
Figure 3. ACE2 in SARS-CoV-2 infected versus not infected placentas, with distribution between villi and decidua. (A) Case 1i images show COVID-19 miscarriage at 18 weeks of gestation. Case 2i images represent COVID-19 stillbirth at 30 weeks of gestation. Case 4i are images of a COVID-19 patient with severe course of the disease (pneumonia with decompensation) who was infected at 32 weeks of gestation and delivered at 33 1/7. The control cases images are placentas from COVID-19 negative women. Case 1c (control) are images of a placenta after stillbirth at 20 weeks, case 2c (control) images are from a term birth, and case 3c (control) was an IUGR case. Scale bar: 100 μm. (B) ACE2 H-Score quantification in the entire placenta. ACE2 H-score was significantly increased in COVID-19 cases (violet) compared to control cases (blue) (p = 0.0134). (C) ACE2 H-score was significantly increased in COVID-19 cases (violet) in the decidua compared to controls (blue) (p = 0.0149). No significant difference was observed in villi between COVID-19 (violet) and control cases (blue) (p = 0.07). Data are represented as mean ± SD.
An overview of the histopathological changes in the placentas is depicted in Table 3.
In the three cases with stillbirth/miscarriage, ISH showed intense viral replication at placental level. IRS was maximal (IRS = 12) for the antisense strand of the orf1ab gene in two cases (Case1i and Case2i, miscarriage at 18 and stillbirth at 30 weeks of gestation, respectively), and strongly positive (IRS = 9) for a Case3i (miscarriage at 18 weeks of gestation). Furthermore, IRS’s for SARS-CoV-2 glycoprotein (IHC), nucleocapsid (IHC), and RNA (S gene encoding for spike protein) (ISH) were also maximal in Case1i and Case2i (IRS = 12), and strongly positive (IRS = 9) in Case3i, demonstrating detection of the virus at both protein and RNA levels (Figures 4, 5). In a control where stillbirth occurred, Case1c (stillbirth at 21 weeks of gestation, no SARS-CoV-2 infection), IRS was 0 for all examinations. Mild to moderate IRS’s (IRS = 2–6) for the antisense strand of the orf1ab gene, demonstrating replication of lower intensity, were noted in further 12 cases, leading to a total of 15 placentas with evidence of viral replication (15/61, 24.59%). On a deeper analysis, IRS for glycoprotein (IHC), nucleocapsid (IHC), and for the antisense strand of the orf1ab gene (ISH) was significantly higher in placentas originating from patients with severe/critical disease (p = 0.0013, p = 0.03, and p = 0.034, respectively) (Figure 4). In all cases with evidence of viral replication, IHC was positive for viral proteins (Figure 5).
Figure 4. Evidence of SARS-CoV-2 nucleocapsid and glycoprotein in SARS-CoV-2 infected placentas versus controls, as well as evidence of viral replication with RNAscope®. Cases are identical to Figure 3. (A–D) Representative images for the spike glycoprotein and (E–H) the nucleocapsid protein staining of cases and a control (negative). The red circles in case 3 point to a positive signal for spike glycoprotein (C), nucleocapsid protein (G). (I–P) Representative images of the RNAscope with magenta for SARS-CoV-2 S gene encoding the spike protein and cyan for SARS-CoV-2 Antisense strand of the orf1ab gene (virus replication). (K) Red circles point to a positive signal for virus replication. (M) Representative overview and (N) representative high magnification image after RNAscope staining of case 1i show high viral replication (cyan). (O) Representative overview and (P) representative high magnification image after RNAscope staining of case 2i show high viral replication (cyan).
Figure 5. Quantification of immune cells in COVID-19 and control placentas. Cases are identical to Figure 3. Representative images of COVID-19 cases and images of control cases with (A) CD8+ staining in the decidua, with (B) CD3+ staining in the decidua and with (C) CD68+ staining in the villous stroma. (A,B) Arrowheads point to some positive CD3 and CD8 cells. (C) Red circles indicate hotspots of CD68 positive cells. (D–F) Quantification of CD3-, CD8-, and CD11b positive cell number in hotspots in the decidua and villi. (G,H) Quantification of positive area of CD68 and CD86 staining in hotspots in the decidua and villi. (I) IRS-score of IL6 and (J) positive area of TNFa were additionally analyzed. In all cases, no significant difference was detected between COVID-19 and control cases (see Table 2, data represented as mean ± SD).
All cases with presence of intense replication (3/61, 4.91%) occurred during the Delta dominance.
Mean duration of time (weeks) from diagnosis of infection to delivery (thus placenta staining) was significantly lower in patients with versus without evidence of replication [8.31 versus 15.58 (weeks), p = 0.0189].
In all controls, IRS for the antisense strand of the orf1ab gene was 0.
We performed multiple linear regression to analyze the risk factors for presence of severe/critical illness, by considering maternal age, presence of comorbidities (as described in Table 1), gestational diabetes and adiposity (body mass index >25 kg/m2). Only presence of comorbidities was significantly associated with severe/critical illness (p = 0.0193). Further, we performed multiple linear regression to analyze if any of the above were risk factors for viral replication in the placenta, and found no association (p ≥ 0.05 for all). Further obstetrical outcomes are depicted in Table 4.
In Case2i (stillbirth at 30 weeks of gestation), placental qPCR was positive for SARS-CoV-2. Pathology showed marked CHI (Table 3; Figure 6), whereas variant sequencing revealed the B.1.617 (Delta) variant. Alltogether, two of the stillbirth cases in the infection group were recruited during Delta, whereas Case3i was recruited during Omicron dominance.
Figure 6. Evidence of marked chronic histiocytic intervillositis. Representative images of case 2i with stillbirth at 30 weeks of gestation.
qPCR from fetal membranes, placenta, and vaginal fluid was negative in all remaining cases and controls.
Placental histopathology for Case1i (miscarriage at 18 weeks of gestation) revealed fulminant villitis and chorioamnionitis with extensive placental infarction and for Case2i massive proliferation of the intervillous fibrin with single intercalated lymphocytes and macrophages (Figures 6, 7).
The main result of our study is evidence of intense SARS-CoV-2 replication in 4.91%, and low to moderate replication in further 19.67% of the placentas originating from women infected with SARS-CoV-2 at any time point during pregnancy. The three patients, for whom intense replication was documented, all presented at our service with miscarriage/stillbirth, which could suggest a direct causality between infection and the intrauterine fetal demise (IUFD). Meanwhile, placental histopathological alterations in these cases reinforce this hypothesis.
Globally, intensity of placental viral replication was higher in women with severe/critical symptoms, supporting the rationale that severity of SARS-CoV-2 infection could correlate with the severity of placentitis. Furthermore, viral presence was confirmed in an important number of placentas by additional markers at protein (glycoprotein and nucleocapsid) and RNA (S gene) levels, reinforcing the results. Analog to the intensity of the replication, virus levels (IRS for glycoprotein and nucleocapsid) both correlated with the severity of maternal disease. No viral evidence could be found in the control placentas by any method, which underlines the accuracy of the results.
The susceptibility of the human placenta to viral replication was demonstrated in an advanced ex vivo model, with authors providing evidence that SARS-CoV-2 can undergo a full replication cycle in the placenta, which offers an equivalent for our findings (21). Our study does not only provide important data regarding the potential of SARS-CoV-2 to induce placental damage, but also on its dimensions, by quantifying this in the context of a larger pregnant population, in respect to international recommendations (3).
Concerning the timing of infection, we noted higher scores for viral replication when delivery occurred shortly after infection. Out of a clinical perspective, this suggests the existence of a `critical phase` in the first weeks after maternal SARS-CoV-2 infection. Of course, larger studies with clinical correlation are needed to support this hypothesis.
All cases with presence of intense replication occurred during the Delta dominance, underlying once again that this variant of SARS-CoV-2 was the most severe and virulent strain, characterized by its significantly higher transmissibility and increased risk of severe disease compared to earlier variants (22).
ACE2 levels were higher in SARS-CoV-2 infected placentas in our cohort, particularly in the decidua. This is in line with a recent publication describing decreasing ACE2 levels in healthy placentas thorough the pregnancy versus a persistence in case of COVID-19 infection (23). Contrastingly, Verma et al. reported a decrease in ACE2 expression in SARS-CoV-2 infected placentas, compared to controls, supporting the hypothesis of ACE2 viral colonization and thus SARS-CoV-2 associated reduction of ACE2 receptors (24). Interestingly, Roberts et al. reported a varying ACE2 expression thorough pregnancy, suggesting that early pregnancies could be more susceptible to SARS-CoV-2 infection (25). Data regarding ACE2 expression in the placenta throughout pregnancy, with or without SARS-CoV-2, remain conflicting (21). Since vertical transmission of SARS-CoV-2 only reaches 1.1–3%, a well-functioning protective mechanism seems to exist at placental level, yet this is not completely elucidated (26–28).
SARS-CoV-2 infection did not lead to SGA (small for gestational age) fetuses in our cohort. In a previous study, we could show that SARS-CoV-2 infection during pregnancy can lead to low weight placentas, also without association with IUGR (29).
Further obstetrical outcomes of our population were in line with available publications (30, 1–2).
At this point, we need to mention that controls were only matched for gestational age and obstetrical pathology, but not for maternal morbidity. This remains a limitation of our study. A further limitation is the small number of cases with IUFD, which makes it difficult to obtain robust statistics. Another important limitation is the lack of serotype analysis, which was not standardly performed in each case, in line with internal hospital practices of the respective period.
Meanwhile, major strengths of our report are its prospective nature and its case–control design. Furthermore, all staining and examinations, including IHC and ISH, have been conducted in all 71 cases, which makes our study, to our knowledge, one the most extensive of its kind. IHC and ISH were concordant in all cases, which is another strength of the study.
We report evidence of active in vivo SARS-CoV-2 replication in the placenta after maternal infection in pregnancy in a case–control setting in a larger population. Replication was maximal in cases of stillbirth, which suggests direct placental involvement in the pathophysiology of this dramatic outcome. In our opinion, evidence of viral replication at the maternal-fetal interface reinforces the necessity of systematically examining the placenta for SARS-CoV-2 in all stillbirth cases, even in absence of maternal COVID-19 diagnosis or infection signs. Understanding the causes of stillbirth remains essential for counseling in regards to future pregnancies.
Continuing to advocate for preventive measures against COVID-19 during pregnancy, including (re)vaccination, as well as appropriately counseling women with diagnosed infection, are of utter importance.
The datasets presented in this study can be found in online repositories. The names of the repository/repositories and accession number(s) can be found in the article/Supplementary material.
The studies involving humans were approved by the Cantonal Ethics Committee in Bern (registration-number BASEC 2020-02610). The studies were conducted in accordance with the local legislation and institutional requirements. The participants provided their written informed consent to participate in this study.
A-PR: Conceptualization, Data curation, Formal analysis, Investigation, Methodology, Project administration, Supervision, Validation, Visualization, Writing – original draft. PR: Conceptualization, Data curation, Formal analysis, Investigation, Methodology, Validation, Writing – review & editing. LR: Methodology, Supervision, Validation, Visualization, Writing – review & editing. A-SV: Data curation, Methodology, Software, Validation, Visualization, Writing – review & editing. VH: Data curation, Investigation, Methodology, Visualization, Writing – review & editing. MT: Data curation, Formal analysis, Investigation, Methodology, Software, Visualization, Writing – review & editing. DS: Conceptualization, Formal analysis, Funding acquisition, Investigation, Methodology, Project administration, Resources, Supervision, Validation, Visualization, Writing – review & editing.
The author(s) declare that financial support was received for the research, authorship, and/or publication of this article. Open access funding by the University of Bern.
The authors declare that the research was conducted in the absence of any commercial or financial relationships that could be construed as a potential conflict of interest.
All claims expressed in this article are solely those of the authors and do not necessarily represent those of their affiliated organizations, or those of the publisher, the editors and the reviewers. Any product that may be evaluated in this article, or claim that may be made by its manufacturer, is not guaranteed or endorsed by the publisher.
The Supplementary material for this article can be found online at: https://www.frontiersin.org/articles/10.3389/fmed.2024.1439181/full#supplementary-material
1. Di Mascio, D, Buca, D, Berghella, V, Khalil, A, Rizzo, G, Odibo, A, et al. Counseling in maternal-fetal medicine: SARS-CoV-2 infection in pregnancy. Ultrasound Obstet Gynecol. (2021) 57:687–97. doi: 10.1002/uog.23628
2. Gurol-Urganci, I, Jardine, JE, Carroll, F, Draycott, T, Dunn, G, Fremeaux, A, et al. Maternal and perinatal outcomes of pregnant women with SARS-CoV-2 infection at the time of birth in England: national cohort study. Am J Obstet Gynecol. (2021) 225:522.e1–522.e11. doi: 10.1016/j.ajog.2021.05.016
3. Roberts, DJ, Edlow, AG, Romero, RJ, Coyne, CB, Ting, DT, Hornick, JL, et al. A standardized definition of placental infection by SARS-CoV-2, a consensus statement from the National Institutes of Health/Eunice Kennedy Shriver National Institute of Child Health and Human Development SARS-CoV-2 placental infection workshop. Am J Obstet Gynecol. (2021) 225:593.e1–9. doi: 10.1016/j.ajog.2021.07.029
4. Stenton, S, McPartland, J, Shukla, R, Turner, K, Marton, T, Hargitai, B, et al. SARS-COV2 placentitis and pregnancy outcome: a multicentre experience during the alpha and early Delta waves of coronavirus pandemic in England. EClinicalMedicine. (2022) 47:101389. doi: 10.1016/j.eclinm.2022.101389
5. Marton, T, Hargitai, B, Hunter, K, Pugh, M, and Murray, P. Massive Perivillous fibrin deposition and chronic histiocytic Intervillositis a complication of SARS-CoV-2 infection. Pediatr Dev Pathol. (2021) 24:450–4. doi: 10.1177/10935266211020723
6. Schwartz, DA, and Dhaliwal, A. Coronavirus diseases in pregnant women, the placenta, fetus, and neonate. Adv Exp Med Biol. (2021) 1318:223–41. doi: 10.1007/978-3-030-63761-3_14
7. Schwartz, DA, Baldewijns, M, Benachi, A, Bugatti, M, Collins, RRJ, de Luca, D, et al. Chronic histiocytic intervillositis with trophoblast necrosis is a risk factor associated with placental infection from coronavirus disease 2019 (COVID-19) and intrauterine maternal-fetal severe acute respiratory syndrome coronavirus 2 (SARS-CoV-2) transmission in live-born and stillborn infants. Arch Pathol Lab Med. (2021) 145:517–28. doi: 10.5858/arpa.2020-0771-SA
8. Argueta, LB, Lacko, LA, Bram, Y, Tada, T, Carrau, L, Zhang, T, et al. SARS-CoV-2 infects syncytiotrophoblast and activates inflammatory responses in the placenta. bioRxiv [Preprint]. (2022) 25:104223. doi: 10.1016/j.isci.2022.104223
9. Schwartz, DA, and Morotti, D. Placental pathology of COVID-19 with and without fetal and neonatal infection: trophoblast necrosis and chronic histiocytic intervillositis as risk factors for transplacental transmission of SARS-CoV-2. Viruses. (2020) 12:1308. doi: 10.3390/v12111308
10. Pulinx, B, Kieffer, D, Michiels, I, Petermans, S, Strybol, D, Delvaux, S, et al. Vertical transmission of SARS-CoV-2 infection and preterm birth. Eur J Clin Microbiol Infect Dis. (2020) 39:2441–5. doi: 10.1007/s10096-020-03964-y
11. Debelenko, L, Katsyv, I, Chong, AM, Peruyero, L, Szabolcs, M, and Uhlemann, AC. Trophoblast damage with acute and chronic intervillositis: disruption of the placental barrier by severe acute respiratory syndrome coronavirus. Hum Pathol. (2021) 109:69–79. doi: 10.1016/j.humpath.2020.12.004
12. Mongula, JE, Frenken, MWE, van Lijnschoten, G, Arents, NLA, de Wit-Zuurendonk, LD, Schimmel-de Kok, APA, et al. COVID-19 during pregnancy: non-reassuring fetal heart rate, placental pathology and coagulopathy. Ultrasound Obstet Gynecol. (2020) 56:773–6. doi: 10.1002/uog.22189
13. Vivanti, AJ, Vauloup-Fellous, C, Prevot, S, Zupan, V, Suffee, C, do Cao, J, et al. Transplacental transmission of SARS-CoV-2 infection. Nat Commun. (2020) 11:3572. doi: 10.1038/s41467-020-17436-6
14. Taglauer, E, Benarroch, Y, Rop, K, Barnett, E, Sabharwal, V, Yarrington, C, et al. Consistent localization of SARS-CoV-2 spike glycoprotein and ACE2 over TMPRSS2 predominance in placental villi of 15 COVID-19 positive maternal-fetal dyads. Placenta. (2020) 100:69–74. doi: 10.1016/j.placenta.2020.08.015
15. Hecht, JL, Quade, B, Deshpande, V, Mino-Kenudson, M, Ting, DT, Desai, N, et al. SARS-CoV-2 can infect the placenta and is not associated with specific placental histopathology: a series of 19 placentas from COVID-19-positive mothers. Mod Pathol. (2020) 33:2092–103. doi: 10.1038/s41379-020-0639-4
16. Edlow, AG, Li, JZ, Collier, AY, Atyeo, C, James, KE, Boatin, AA, et al. Assessment of maternal and neonatal SARS-CoV-2 viral load, Transplacental antibody transfer, and placental pathology in pregnancies during the COVID-19 pandemic. JAMA Netw Open. (2020) 3:e2030455. doi: 10.1001/jamanetworkopen.2020.30455
17. Algarroba, GN, Rekawek, P, Vahanian, SA, Khullar, P, Palaia, T, Peltier, MR, et al. Visualization of severe acute respiratory syndrome coronavirus 2 invading the human placenta using electron microscopy. Am J Obstet Gynecol. (2020) 223:275–8. doi: 10.1016/j.ajog.2020.05.023
18. Favre, G, Maisonneuve, E, Pomar, L, Daire, C, Poncelet, C, Quibel, T, et al. Maternal and perinatal outcomes following pre-Delta, Delta, and omicron SARS-CoV-2 variants infection among unvaccinated pregnant women in France and Switzerland: a prospective cohort study using the COVI-PREG registry. Lancet Reg Health Eur. (2023) 26:100569. doi: 10.1016/j.lanepe.2022.100569
19. World Health Organization (2021). Definition and categorization of the timing of mother-to-child transmission of SARS-CoV-2. Available online at: https://www.who.int/publications/i/item/WHO-2019-nCoV-mother-to-child-transmission-2021.1 (Accessed March 01, 2021).
20. National Institutes of Health (NIH) (2023). Clinical Spectrum of SARS-CoV-2 Infection. Available online at: https://www.covid19treatmentguidelines.nih.gov/overview/clinical-spectrum/ (Accessed March 10, 2022).
21. Fahmi, A, Brügger, M, Démoulins, T, Zumkehr, B, Oliveira Esteves, BI, Bracher, L, et al. SARS-CoV-2 can infect and propagate in human placenta explants. Cell Rep Med. (2021) 2:100456. doi: 10.1016/j.xcrm.2021.100456
22. Cocherie, T, Zafilaza, K, Leducq, V, Marot, S, Calvez, V, Marcelin, AG, et al. Epidemiology and Characteristics of SARS-CoV-2 Variants of Concern: The Impacts of the Spike Mutations. Microorganisms. (2022) 11:30. doi: 10.3390/microorganisms11010030
23. Lu-Culligan, A, Chavan, AR, Vijayakumar, P, Irshaid, L, Courchaine, EM, Milano, KM, et al. SARS-CoV-2 infection in pregnancy is associated with robust inflammatory response at the maternal-fetal interface. medRxiv [Preprint]. (2021) 2:591–610.e10. doi: 10.1016/j.medj.2021.04.016
24. Verma, S, Joshi, CS, Silverstein, RB, He, M, Carter, EB, and Mysorekar, IU. SARS-CoV-2 colonization of maternal and fetal cells of the human placenta promotes alteration of local renin-angiotensin system. Med. (2021) 2:575–590.e5. doi: 10.1016/j.medj.2021.04.009
25. Roberts, DJ, Bebell, LM, and Edlow, AG. Severe acute respiratory syndrome coronavirus 2 ACE2 and TMPRSS2 receptor protein expression patterns throughout gestation. J Infect Dis. (2021) 224:S642–6. doi: 10.1093/infdis/jiab164
26. Best Rocha, A, Stroberg, E, Barton, LM, Duval, EJ, Mukhopadhyay, S, Yarid, N, et al. Detection of SARS-CoV-2 in formalin-fixed paraffin-embedded tissue sections using commercially available reagents. Lab Investig. (2020) 100:1485–9. doi: 10.1038/s41374-020-0464-x
27. Piñana, M, Abril, JF, Andrés, C, Silgado, A, Navarro, A, Suy, A, et al. Viral populations of SARS-CoV-2 in upper respiratory tract, placenta, amniotic fluid and umbilical cord blood support viral replication in placenta. Clin Microbiol Infect. (2021) 27:1542–4. doi: 10.1016/j.cmi.2021.07.008
28. Valdespino-Vázquez, MY, Helguera-Repetto, CA, León-Juárez, M, Villavicencio-Carrisoza, O, Flores-Pliego, A, Moreno-Verduzco, ER, et al. Fetal and placental infection with SARS-CoV-2 in early pregnancy. J Med Virol. (2021) 93:4480–7. doi: 10.1002/jmv.26965
29. Radan, AP, Baud, D, Favre, G, Papadia, A, Surbek, D, Baumann, M, et al. Low placental weight and altered metabolic scaling after severe acute respiratory syndrome coronavirus type 2 infection during pregnancy: a prospective multicentric study. Clin Microbiol Infect. (2022) 28:718–22. doi: 10.1016/j.cmi.2022.02.003
Keywords: COVID-19, placenta, SARS-CoV-2, SARS-CoV-2 replication, stillbirth
Citation: Radan A-P, Renz P, Raio L, Villiger A-S, Haesler V, Trippel M and Surbek D (2024) SARS-CoV-2 replicates in the placenta after maternal infection during pregnancy. Front. Med. 11:1439181. doi: 10.3389/fmed.2024.1439181
Received: 03 June 2024; Accepted: 14 August 2024;
Published: 04 September 2024.
Edited by:
Moises Leon Juarez, Instituto Nacional de Perinatología (INPER), MexicoReviewed by:
José M. Reyes-Ruiz, Mexican Social Security Institute, MexicoCopyright © 2024 Radan, Renz, Raio, Villiger, Haesler, Trippel and Surbek. This is an open-access article distributed under the terms of the Creative Commons Attribution License (CC BY). The use, distribution or reproduction in other forums is permitted, provided the original author(s) and the copyright owner(s) are credited and that the original publication in this journal is cited, in accordance with accepted academic practice. No use, distribution or reproduction is permitted which does not comply with these terms.
*Correspondence: Daniel Surbek, ZGFuaWVsLnN1cmJla0BpbnNlbC5jaA==
†These authors have contributed equally to this work
Disclaimer: All claims expressed in this article are solely those of the authors and do not necessarily represent those of their affiliated organizations, or those of the publisher, the editors and the reviewers. Any product that may be evaluated in this article or claim that may be made by its manufacturer is not guaranteed or endorsed by the publisher.
Research integrity at Frontiers
Learn more about the work of our research integrity team to safeguard the quality of each article we publish.