- College of Medicine and Pharmacy, Umm Al-Qura University, Makkah, Saudi Arabia
Introduction: Despite advancements in stroke care, challenges persist in timely triage and treatment initiation to prevent the burden of stroke-related disabilities. Although nuclear medicine has shown promise, no imaging technique has yet provided a sufficiently rapid, precise, and cost-effective approach to routine stroke management. This study aims to review the clinical application of nuclear medicine in stroke diagnosis and treatment.
Methods: A systematic search of the Cochrane, EU Clinical Trials Register, ISRCTN, the International Stroke Trial, and the ClinicalTrials.gov database was conducted to find all registered trials reporting nuclear medicine’s clinical applications in stroke up to June 07, 2024.
Results: Among the 220 screened trials, 51 (36 interventional; 15 observational) met the eligibility criteria. Participants were older than 18 years old, with only six studies including pediatric under 17 years old, with a total of 11,262 stroke (9,232 ischemic; 2,030 haemorrhagic) participants. The bias risk varied across trials but remained mostly low to moderate.
Discussion: The review highlighted nuclear medicine’s significant contributions to stroke diagnosis and management, notably through mobile stroke units, pre-hospital acute stroke magnetic resonance image (MRI) based biomarkers, and MRI-based stroke mechanisms for 4D flow nuclear imaging. These advancements have generally reduced treatment delays and enhance clinical outcomes post-stroke. Specifically, radiopharmaceutical radiotracers can effectively discriminate between strokes and mimics, particularly in high-risk patients. Integrating novel positron emission tomography (PET) radiotracer 18F glycoprotein 1 and radionuclide angiography may improve sensitivity and specificity in thrombi detection for decisions regarding stenting or carotid endarterectomy, and the single-photon emission computed tomography and PET integration with ferumoxytol radiotracer-enhanced MRI enables functional imaging for evaluating cerebral perfusion, metabolic activity, and neuroinflammatory markers post-stroke. Overall, the integration of nuclear medicine into multimodal imaging equipment like computed-tomography PET and MRI-PET offers a more comprehensive picture of the brain. Nevertheless, further research is needed on novel stroke imaging techniques and standardization across stroke centers for optimal performance.
Systematic review registration: https://www.crd.york.ac.uk/prospero/display_record.php?ID=CRD42024541680, identifier PROSPERO CRD(42024541680).
1 Introduction
Stroke is a major cause of morbidity and mortality, accounting for 30% of stroke-related disabilities and 20% of all deaths worldwide (1). Despite stroke system-optimization efforts, interhospital communication for triage and transport to a thrombectomy center can be operationally challenging. Even in experienced stroke care systems, there is a frequent time delay of nearly 100 min (2), which may result in significant neuronal loss, increased mortality, morbidity, or even exclusion as a candidate for endovascular thrombectomy (EVT) intervention. It is estimated that over 1.9 million neuronal fibers are lost each minute without treatment (3). Indeed, for every 15 min reduction to recanalization, 34 per 1,000 treated stroke patients experience improvements in disability, as faster initiation of treatment leads to better clinical outcomes (4).
The management of stroke patients depends highly on the information obtained from imaging technologies. Nuclear medicine utilizes radiopharmaceuticals to diagnose and manage strokes by emitting gamma rays detected by specialized cameras, which provide crucial images of the brain’s internal structures and functions (5). Technologies such as the single-photon emission computed tomography (SPECT) and positron emission tomography (PET) (6) offer molecular insights into disease processes and cerebral blood flow, thereby enabling personalized care. Despite the low radiation doses, the benefits of accurate diagnosis and targeted therapy, which lead to improved outcomes and stroke care quality, outweigh the associated risks.
Specifically, nuclear medicine techniques (7) of high sensitivity and specificity enable earlier diagnosis and more adequate management over computed-tomography (CT) and magnetic resonance image (MRI) scans. Determining stroke etiology, which remains cryptogenic in as many as one-third of patients even after a comprehensive workup (8), is challenging. This is in part because it is challenging not only to confidently identify stroke mimics, a false-positive stroke which explain more than 40% of cases presenting with an acute neurological deficit (9), but also to distinguish between heterogeneous ischemic and haemorrhagic stroke disorders in circumstances where access to brain imaging is limited and a very rapid turnaround is needed. Stroke treatment algorithms are becoming increasingly complex in addressing various clinical and biological parameters, imaging the infarct penumbra volume size, determining the time of symptom onset, and assessing haemorrhagic risk transformation. At present, the pathophysiological processes involved in brain damage and repair in the context of human stroke remain poorly understood, and successful adjunctive therapies remain limited.
The aim of this study was to review the existing evidence that demonstrates the promising translation of nuclear medicine into the clinical practice of stroke diagnosis and management and to describe newly reported clinical studies that could facilitate routine stroke care. Ultimately, this study provides valuable insights into the clinical applications of nuclear medicine in stroke care.
2 Materials and methods
The review followed the Preferred Reporting Items for Systematic Reviews guidelines (10). The study protocol was registered at the University of York, with a regeneration number PROSPERO CRD (42024541680) (11).
2.1 Data sources and search strategy
The Cochrane library, the EU Clinical Trials Register, the ISRCTN, the International Stroke Trial, and the ClinicalTrials.gov (12) were screened on June 07, 2024 to retrieve all eligible clinical trials. These databased served as a comprehensive online database registry of clinical trials worldwide. Within the database search engine filters, the search terms included keywords and synonyms associated with stroke as a ‘condition/disease’ and were conjugated with nuclear medicine as an ‘interventional’ option to identify all relevant results.
2.2 Inclusion and exclusion criteria
Interventional and non-interventional clinical trials qualified for inclusion if they predominantly focused on the clinical application of nuclear medicine for stroke diagnosis and/or treatment. Trials not in English language or not related to nuclear medicine use in stroke were excluded.
2.3 Data extraction
The pertinent data were manually extracted and downloaded from reports on the clinical databases website, based on pre-established eligibility criteria. A summary step gathered the key characteristics of each clinical trial, including study identification number, title, type of trial, stroke outcomes, interventions, nuclear imaging technology, primary and secondary outcome measures, sample size, age of participants enrolled, trial design, phase, and where the trial was conducted. The authors meticulously extracted data from relevant studies and independently adjudicated discrepancies to ensure accuracy and reliability.
2.4 Outcomes measured
The reported stroke was the primary endpoint. The secondary endpoints included stroke reported by subtype (stroke etiological classification), either as ischemic (large-artery atherosclerosis, small-vessel occlusion, cardioembolic, stroke of other determined etiology, or stroke of undetermined etiology), haemorrhagic [intracerebral haemorrhage (ICH) or sub-arachnoid haemorrhage (SAH)], or minor stroke [transient ischemic attacks (TIAs)], and the diagnostic interventional technique applied for stroke management.
2.5 Quality assessment and risk of bias
To evaluate the methodological quality and potential bias in the included studies, each interventional trial was evaluated for risk of bias, and was assessed according to the criteria described in the Cochrane Quality Assessment Tool (13), which include selection, detection, performance, attrition, reporting, and others. Each domain was judged to be of either ‘low’, ‘high’, or ‘unclear’ risk, with the last category indicating uncertainty. Any study with a high score on the first three bias domains, which would indicate a ‘high risk’ trial, was considered to have low-quality evidence.
Each non-interventional trial was also evaluated for risk of bias, which was assessed according to the Risk of Bias In Non-randomized Studies of Interventions (ROBINS-I) Tool (14) with seven domains of bias, consisting of confounding, selection of participants, classifications of interventions, deviation from intended interventions, missing data, measurement of outcomes, and selection of reported results. Each domain was judged to be either ‘low risk’, ‘moderate risk’, ‘serious risk’, ‘critical risk’, or ‘no information’, with the last category indicating uncertainty in one or more key domains of bias. If a trial was judged to be at ‘critical risk’ of bias in at least one domain, it was considered to have low-quality evidence.
2.6 Statistical analyses
To summarize the characteristics of the included studies, descriptive statistics and frequency analysis were employed, presenting data in absolute numbers (n.) and percentages (%). These analyses were conducted using the Statistical Package for Social Sciences version 25, developed by IBM Corporation in Armonk, New York, United States (15). A narrative synthesis was utilized to amalgamate data from multiple studies, providing a comprehensive summary of each study’s data in both textual and tabular formats. To ensure consistency between different reviewers’ assessments, inter-rater reliability measures were implemented. Additionally, the risk of bias and quality analysis for interventional and non-interventional trials were conducted using Review Manager version 5.4.1 (16) and ROBINS-I (17) software, respectively, to generate graphical outputs.
3 Results
The systematic screening and filtration process initially identified 220 trials, comprising 178 (80.9%) interventional and 42 (19.1%) observational studies. Following the application of exclusion criteria, 111 (50.5%) trials were eliminated. The reasons for exclusion included: exclusion based on title and abstract (37; 16.7%), duplication (4; 1.7%), incomplete recruitment (9; 4.1%), protocol status (15; 6.7%), being conference abstracts (3; 1.2%), reviews (8; 3.5%), comparative studies (4; 1.7%), withdrawal (2; 0.8%), termination (7; 3.2%), ongoing status (1; 0.4%), unknown status (18; 8.2%), and non-English language (3; 1.3%). Out of the remaining 109 (49.7%) trials assessed for eligibility, 16 (7.5%) were excluded for not reporting stroke-related outcomes or being outside of phase IV (42; 19.3%). Ultimately, 51 (23.5%) clinical trials were deemed eligible and included in the final review, Figure 1.
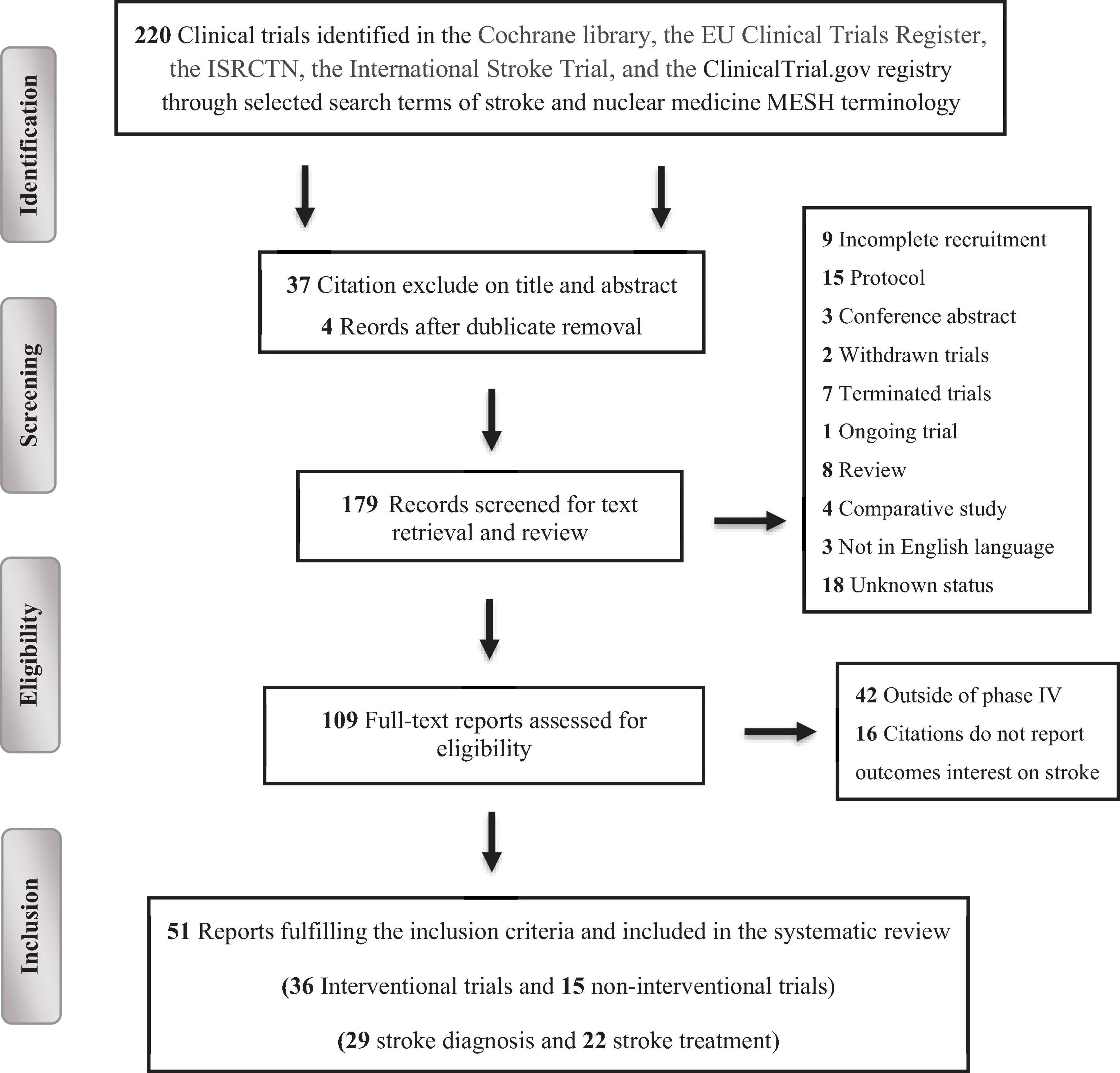
Figure 1. PRISMA flow chart depicting the selection process of the clinical trials included in the systematic review.
3.1 Clinical trials characteristics
Out of the 51 included trials, 36 (70.6%) adopted an interventional clinical approach, with the remaining 15 (29.4%) trials adopting an observational clinical design. Of the observational trials, eight (53.3%) were cohort based and seven (46.7%) were case controlled (three (20%) were retrospective and 12 (80%) were time prospective), thereby shedding light on the clinical long-term impacts of nuclear medicine in association with the stroke population. For the interventional trials, two were phase I (5.6%), three were phase II (8.3%), one was phase III (2.8%), two were phase IV (5.6%), and 28 (77.7%) were completed, with the latter offering a more thorough understanding of the clinical applications of nuclear medicine for stroke diagnosis and management.
In terms of geographical distribution, one (1.9%) was enrolled in Egypt, 14 (27.5%) were registered in Korea and China, 14 (27.5%) were conducted in the United States countries, and 22 (43.1%) were carried out in European countries. For nuclear diagnostic trials, the median follow-up duration ranged from eight hours to 18 months; for nuclear management trials, the duration ranged from 12 hours to three months.
Detailed summary of the clinical trials design and baseline characteristics were compiled and presented (Tables 1, 2 for interventional; Tables 3, 4 for non-interventional) trials.
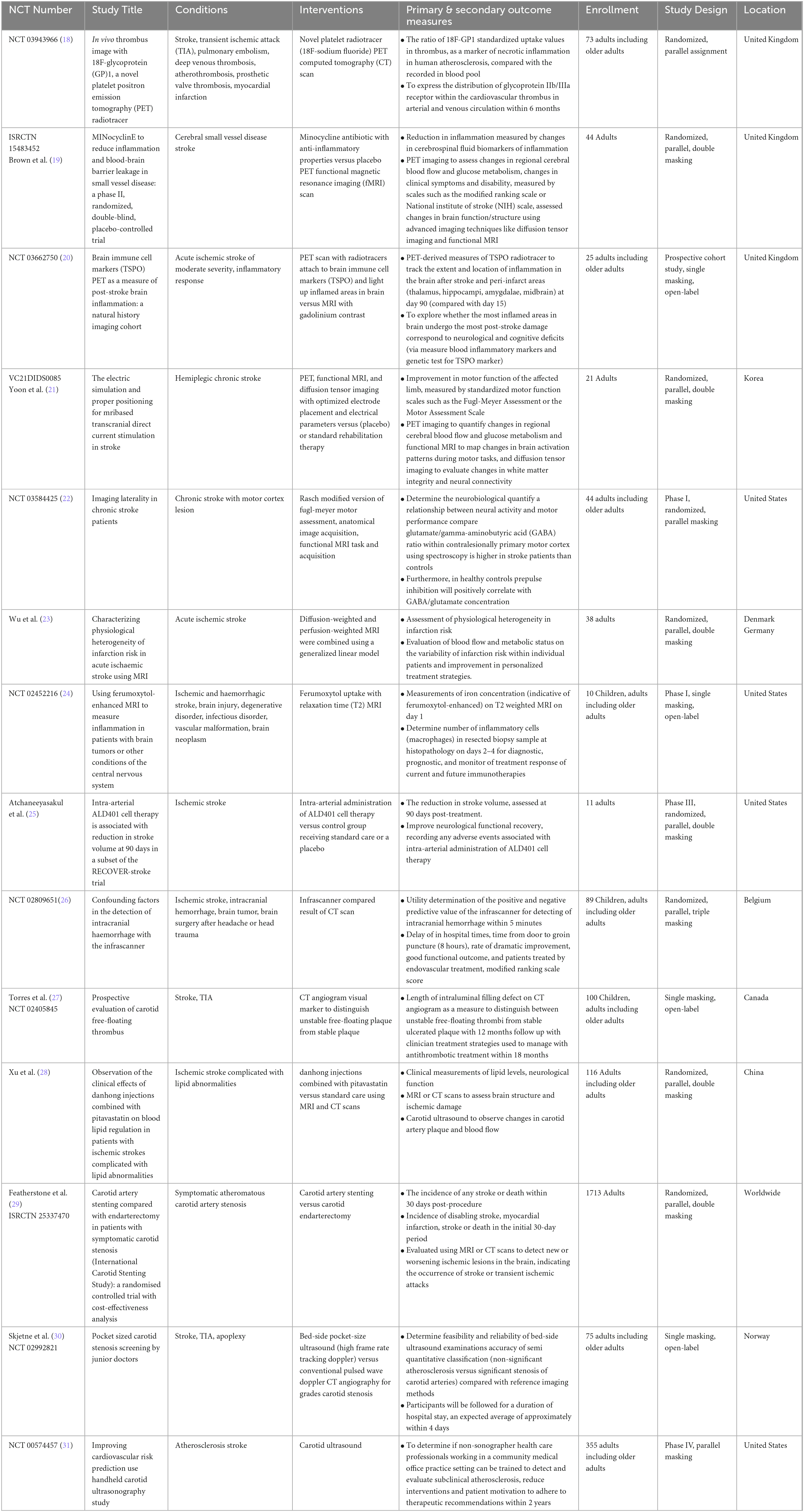
Table 1. Interventional studies for nuclear medicine applications in ischemic and haemorrhagic stroke diagnosis.
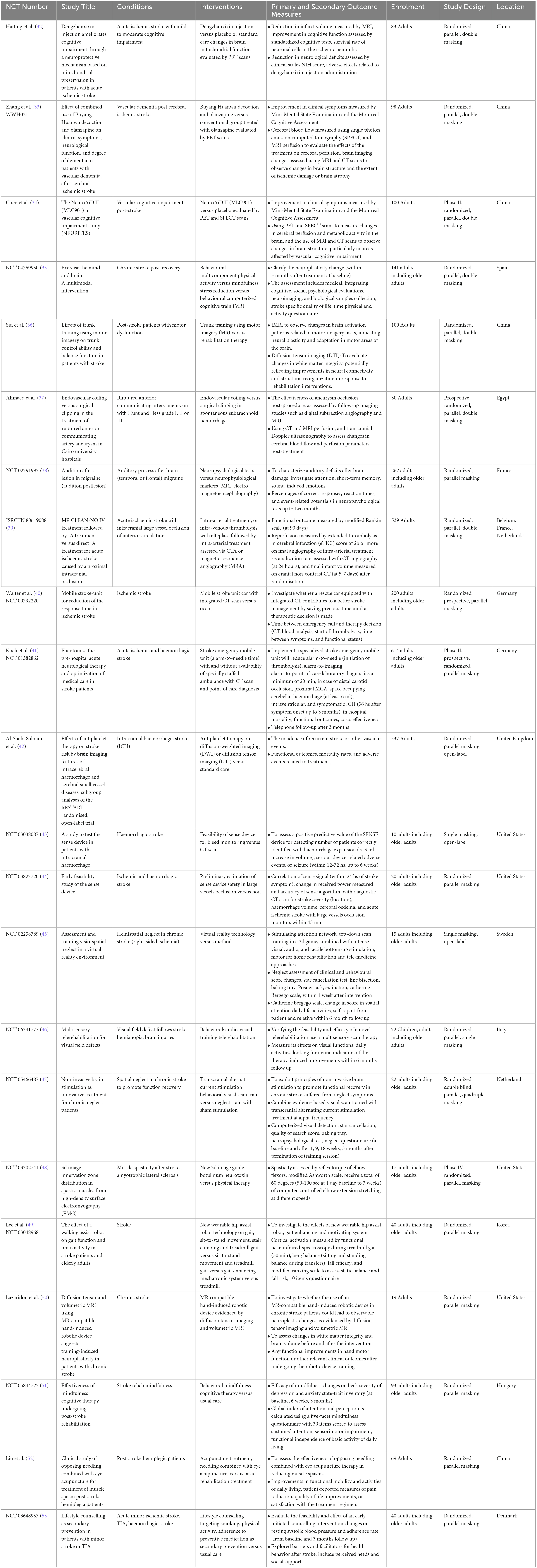
Table 2. Interventional studies for nuclear medicine applications in ischemic and haemorrhagic stroke treatment.
3.2 Trials population
The majority of the included trials were conducted with stroke participants ranging from ≥ 18 and 65 years of age, and only six (16.7%) trials included pediatric patients ≤ 17 years old. The majority of participants were male (6,836; 60.7%). In total, these trials included 11,262 participants who were exclusively diagnosed with either ischemic (9,232; 82.0%) or haemorrhagic (2,030; 18.0%) strokes.
3.3 Risk of bias assessment
Among the 36 interventional trials, 29 (80.6%) were randomly allocated with a blinded treatment allocation strategy and scored with a low risk of selection bias. However, seven (19.4%) trials employed an open-label design and scored with a high risk of performance bias. In terms of masking, the primary intervention model used was double blind parallel assignment (27; 75.0%), followed by single (seven; 19.4%), triple (one; 2.8%), and quadruple (one; 2.8%) masking techniques. The risk of attrition and reporting biases was unclear in seven single masking trials (19.4%), Figure 2.
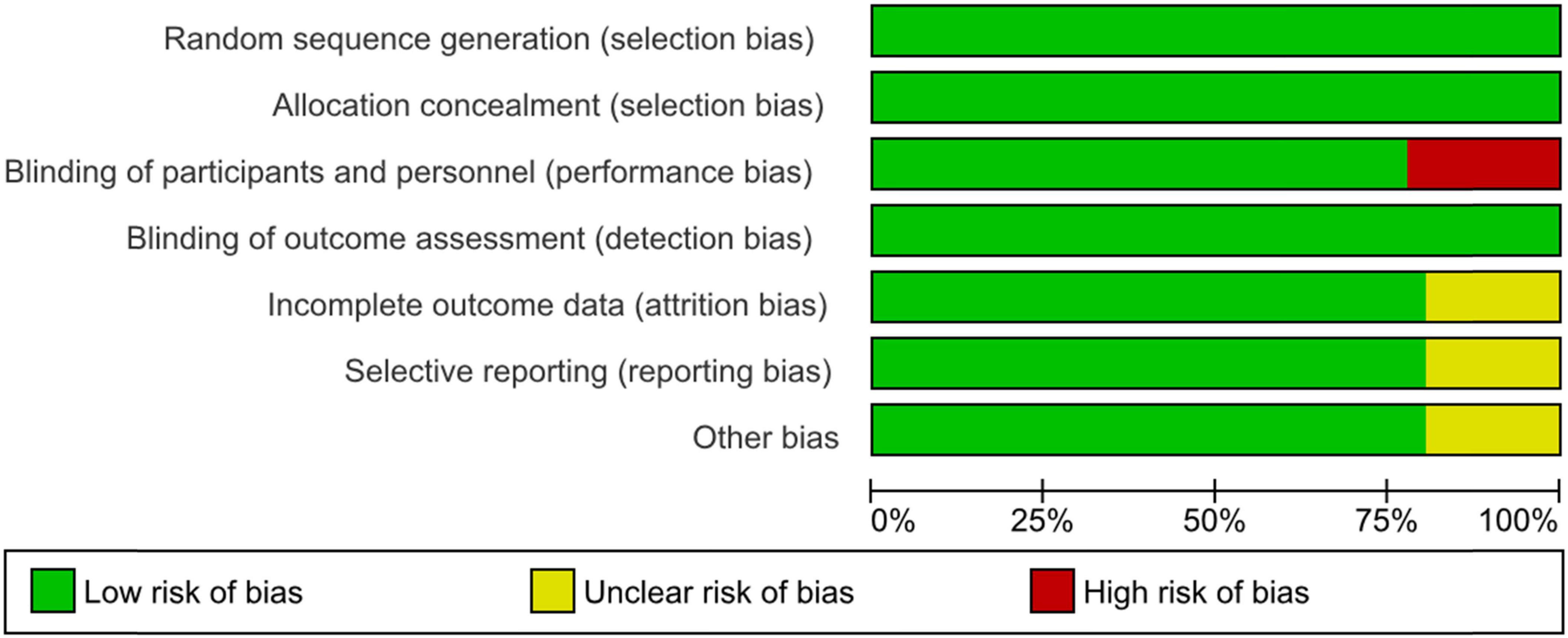
Figure 2. Risk of bias graph: review authors’ judgments about each risk of bias item presented as percentages across all included interventional trials in the systematic review.
For the observational trials, 15 (41.7%) were measured by confounding variables and scored with a serious risk of bias. Generally, the trials were pro- or retrospectively recruited with a consecutive series of participant selections. They did not deviate from the intended intervention of interest, were well defined, and were based solely on what was collected at the time of intervention. In terms of missing outcomes, participants were selected and chosen based on their outcome data. It is unlikely that the outcome measures were influenced by knowledge of the intervention on the part of the trial’s participants; methods of assessment were comparable across groups, and any error in outcome measurement was only minimally related to intervention status. Selection of the reported results was based on clinical knowledge that appeared not to be based on the intervention, outcome, or multiple analyses. Therefore, the overall risk of bias was moderate, without adjustment for confounding (Figure 3).
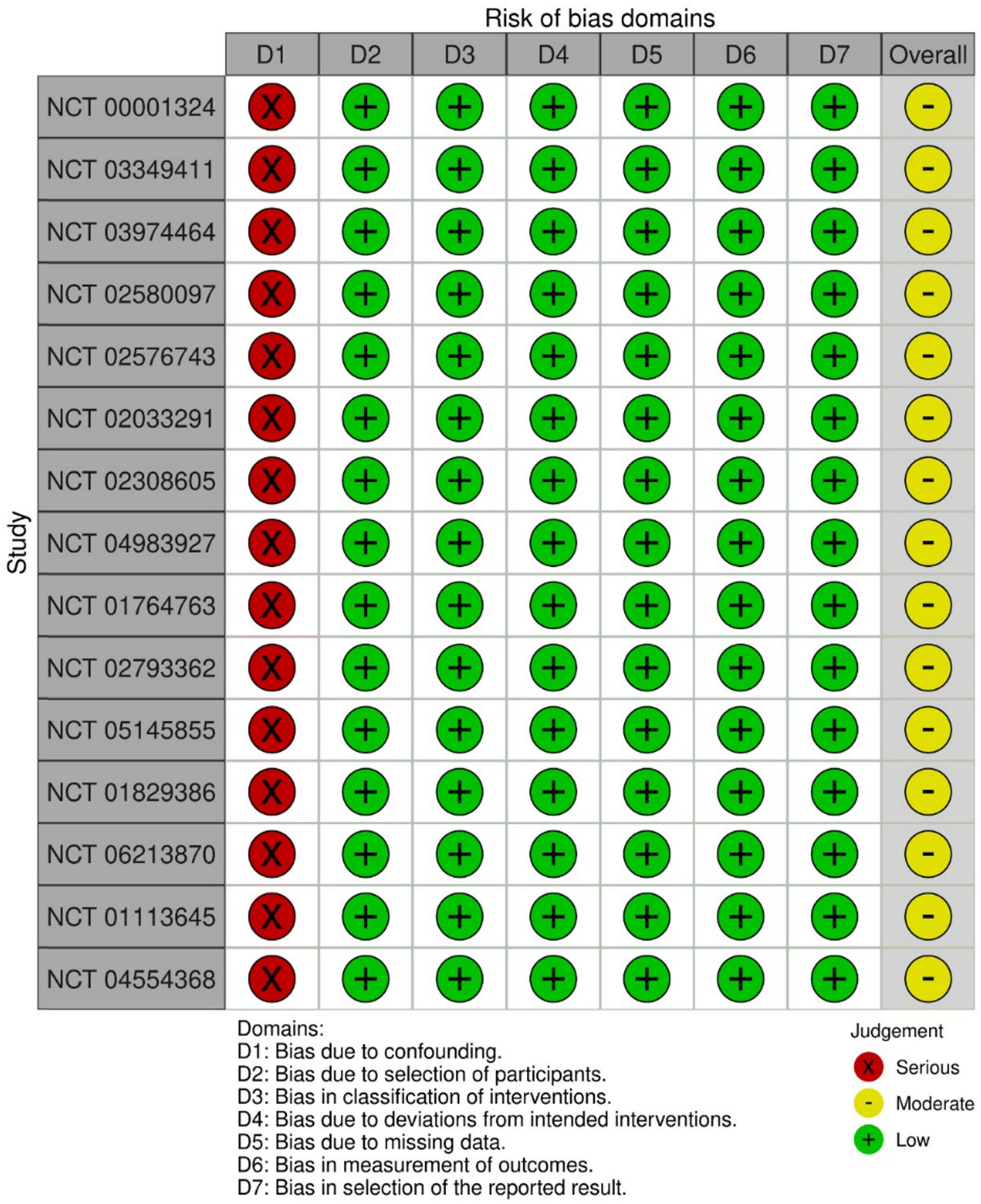
Figure 3. Risk of bias graph: review authors’ judgments about each risk of bias item presented as percentages across all included non-interventional trials in the systematic review.
3.4 Outcome measures
All 51 appraised trials were specifically designed to assess strokes as primary and secondary outcomes, and there was an adequate description of the outcome data. Specifically, 14 (38.9%) interventional trials examined the impacts of nuclear medicine on ischemic stroke diagnosis (of which two examined haemorrhagic stroke as well), whereas an additional 22 (61.1%) examined the impacts on ischemic stroke treatment (of which six also examined haemorrhagic stroke). Six trials focused on acute stroke conditions, yet the predominant focus was on chronic stroke, examined in 30 trials. This substantial emphasis on chronic stroke encompassed 83.3% of patients with large-artery atherosclerosis and cardioembolic conditions. The remaining 16.7% comprised patients with cSVD (one trial) and TIAs (four trials). For the observational trials, 11 (73.3%) examined the impacts of nuclear medicine on ischemic stroke diagnosis (of which one examined haemorrhagic stroke), and an additional four trials (26.7%) examined the impacts on haemorrhagic stroke diagnosis. The most frequently examined stroke condition was acute hemorrhage, accounting for a significant group with symptomatic ICH (five; 33.3%), but a minority with SAH (one; 6.7%).
Regarding the diagnostic interventional techniques, the trials investigating the impact of nuclear medicine on stroke diagnosis employed various approaches: four utilized PET CT/MRI radiotracer scans, four employed diffusion- and perfusion-weighted MRI, three used CT angiography, one used Infrascanner, and two utilized carotid angiography ultrasounds. For stroke treatment, three trials utilized PET and SPECT scans, five employed functional MRI angiography, five used CT angiography, one implemented a novel 3D image guide, two used sensory devices, three incorporated audiovisual reality technology scans, two utilized robotic technology, and one trial explored the use of acupuncture. However, the observational trials focused solely on the impacts of stroke diagnosis, employing various techniques: one PET radiotracer scan, seven MRIs, three CTs, one epiaortic ultrasound, one new Smartcap device, and two multimodal rehabilitation approaches. The trials compared these diagnostic interventions against other methods used in stroke management.
3.5 Outcome results
Trial outcome results are detailed in Tables 1, 2 for interventional and Tables 3, 4 for non-interventional trials. The description of the stroke outcome data is organized here according to their applications in clinical practice: (1) diagnosis, (2) treatment decisions, (3) predictive biomarkers, outcomes, and prognosis, (4) secondary prevention, and (5) rehabilitation.
3.5.1 Nuclear medicine clinical applications for ischemic stroke diagnosis
3.5.1.1 Implementation of pre-hospital acute stroke care notification and therapy protocol
The implementation of a mobile stroke unit (MSU) with an integrated CT scan led to a significant reduction in the time from symptom onset to the initiation of appropriate treatment, such as thrombolysis or EVT, within 35 min (40). This reduction in response time was associated with improved clinical outcomes, including reduced disability and mortality rates among ischemic stroke patients. Although MSUs facilitate on-site stroke diagnosis and the initiation of time-critical interventions, they also contribute to more efficient and streamlined stroke care delivery. Furthermore, the implementation of pre-hospital acute neurological therapy protocols with integrated CT scans led to significant improvements in various aspects of stroke care, with mean time differences in alarm-to-treatment time of 34.7 minutes compared with conventional care (41). These improvements included reduced time to treatment initiation, increased rates of thrombolysis administration, and enhanced adherence to established stroke treatment guidelines. In addition, pre-hospital acute neurological therapy interventions were associated with improved clinical outcomes, including reduced disability and mortality rates among stroke patients. Together, these findings underscore the importance of innovative approaches, such as MSUs, in optimizing acute stroke care, minimizing treatment delays, as well as highlighting the potential benefits of implementing structured protocols for pre-hospital stroke management.
3.5.1.2 Distinction between stroke mimics, healthy controls, and acute stroke
An MRI-based method for identifying and quantifying non-heme iron levels deposited in brain tissue, particularly in areas associated with ischemic injury and neurodegeneration, following haemorrhagic stroke, has been validated (65). The degree of non-heme iron accumulation was found to be correlated with the severity of stroke-related neurological deficits and functional impairment. These findings suggest that non-heme iron accumulation may contribute to the pathophysiology of stroke and its associated complications, potentially serving as a biomarker for assessing disease severity and prognosis in stroke patients. The quantitative assessment of non-heme iron load using MRI techniques holds promise for elucidating the underlying mechanisms of stroke-related brain damage and may inform the development of novel therapeutic interventions targeting iron dysregulation in stroke.
3.5.1.3 Distinction between intracerebral hemorrhage and ischemic stroke
Potential confounding factors influencing the accurate detection of ICH can be identified using the Infrascanner. Several factors significantly impact the reliability of ICH detection, including patient age, Glasgow Coma Scale (GCS) score, and the presence of scalp hematoma (26). Specifically, older age and lower GCS scores were associated with decreased sensitivity of the Infrascanner in detecting ICH, while the presence of scalp hematoma posed challenges in distinguishing between superficial bleeding and ICH. A promising outcome was that the Smartcap blood test showed high accuracy in diagnosing stroke. The blood test utilized a rapid and portable device capable of detecting specific biomarkers and measuring purine levels in freshly drawn blood associated with stroke, enabling swift diagnosis and initiation of appropriate medical interventions in pre-hospital and emergency care settings (60). Specifically, the Smartcap blood test showed potential for distinguishing between ischemic and haemorrhagic strokes after 24 hours. Overall, these findings emphasize the importance of considering various clinical factors when interpreting Infrascanner results to improve their diagnostic accuracy and reliability in emergency settings for timely and appropriate patient management. They also suggest that the Smartcap blood test holds promise as a valuable tool for improving stroke diagnosis and triage, ultimately leading to better patient outcomes and a reduced disability burden associated with stroke.
3.5.1.4 Identify the etiology of stroke
Incorporating MRI-based stroke mechanisms such as large artery atherosclerosis, cerebral small vessel disease (cSVD), and cardioembolism into a comprehensive risk score significantly enhanced the accuracy of predicting future stroke events (57). This advanced MRI-based risk score, powered by machine learning, identified individuals at high risk of recurrent stroke with a collateral-core ratio, achieving a mean net reclassification index of 52.7% ± 32.7%, thereby facilitating targeted preventive interventions and personalized management strategies. Furthermore, ultra-high-field MRI significantly improved the spatiotemporal resolution of 4D flow nuclear imaging and dynamic magnetic resonance angiography (MRA), allowing for more detailed visualization of cerebral blood flow dynamics and vascular anatomy (58). This higher resolution enabled the detection of smaller vessels and subtle flow abnormalities, enhancing diagnostic capabilities for evaluating cerebrovascular pathologies. In another vein, the novel 18F glycoprotein (GP) 1 PET radiotracer, which binds with high affinity to the GP IIb/IIIa receptor involved in platelet aggregation, demonstrated effective in vivo thrombus detection across various preclinical models (18), offering superior resolution compared to traditional MRI and CT modalities. Lastly, minocycline’s efficacy, antibiotic with known anti-inflammatory properties, in reducing inflammation was assessed using PET (26) and MRI dynamic contrast-enhanced brain imaging (59), revealing significant blood-brain barrier (BBB) permeability alterations in patients with cSVD compared to healthy controls, indicating increased leakage of contrast agents into the brain parenchyma.
These findings underscore the potential of MRI-based risk stratification tools to optimize secondary prevention efforts and reduce recurrent strokes, the promise of ultra-high-field MRI for advancing cerebrovascular diagnostics, the utility of 18F-GP1 PET imaging for non-invasive thrombus detection, and the critical role of BBB dysfunction in the pathophysiology of cSVD, contributing to the progression of white matter hyperintensities, lacunar infarcts, and other neurovascular abnormalities.
3.5.2 Nuclear medicine clinical applications for ischemic stroke treatment
A novel scoring system was created for an enhanced flat detector CT, which effectively stratified patients based on their risk of developing symptomatic ICH following reperfusion therapy (68). The scoring system incorporated various clinical and imaging factors, such as age, stroke severity, baseline neuroimaging findings, and comorbidities, to accurately estimate individualized risk profiles for symptomatic ICH. It showed good discrimination and calibration in both the derivation and validation cohorts, indicating its reliability and generalizability for clinical use. This system may serve as a valuable tool for clinicians in risk-stratifying patients undergoing reperfusion therapy for acute ischemic stroke, facilitating informed decision making, and optimizing patient outcomes by mitigating the risk of symptomatic ICH.
3.5.2.1 Estimate the time onset of stroke and the volume of ischemic penumbra and the core
The integration of a clinical decision support system into stroke care has been shown to enhance the accuracy and consistency of interpreting the Alberta Stroke Program Early CT Score on CT images (61), leading to improved interobserver agreement and reduced variability among clinicians. Additionally, advanced MRI techniques like perfusion-weighted imaging and diffusion-weighted imaging (DWI) MRI (30) can identify varying levels at high risk of infarction in stroke patients by revealing significant within-patient heterogeneity influenced by factors such as blood flow and metabolic status, thus facilitating detailed, patient-specific assessments that improve personalized treatment strategies for acute ischemic stroke.
3.5.2.2 Predict recanalization following intravenous thrombolysis
Vascular hyperintensity-diffusion-weighted MRI (66) or CT angiography (54) can be used as a screening tool to identify eligible candidates for EVT among patients with acute ischemic stroke (39) by accurately detecting large vessel occlusions and assessing the extent of ischemic brain injury. Overall, incorporating MRI or CT angiography screening into the acute stroke workflow may enhance the efficiency and accuracy of patient selection for EVT.
3.5.2.3 Predict haemorrhagic transformation in ischemic stroke
The effect of antiplatelet therapy on stroke risk identified by brain imaging features like ICH and cSVD employs DWI or diffusion tensor imaging (DTI) (42) to monitor alterations in white matter integrity and structural damage progression. These results underscore the significance of personalized treatment strategies aligned with brain imaging characteristics to enhance stroke risk management effectively.
The sense device for bleed monitoring effectively allowed for the early detection of seizures activity, cerebral edema, and monitoring hemorrhage expansion in patients with acute ICH (43). The continuous monitoring provided by the sense device allowed for the early detection of changes in brain activity within six hours of symptom onset, providing findings similar to CT scans, facilitating prompt medical attention, and potentially improving stroke patient outcomes (44). These findings suggest that the sense device holds promise as an innovative solution, with high sensitivity and specificity, for monitoring patients with ICH and preventing seizure-related complications.
3.5.3 Nuclear medicine clinical applications for ischemic stroke prognosis
The dynamic changes in PET scans with radiotracers of TSPO, which are putative markers of microglia activation and neuroinflammation in stress-related brain inflammation, can indicate a fluctuation in the inflammatory response following stroke over a period of 90 days (20). Specifically, TSPO PET imaging showed elevated binding in the acute phase of stroke distant from the infarct area (e.g., thalamus, hippocampi, amygdalae, and midbrain), which gradually decreased over time, suggesting resolution of inflammation when compared with day 15 (mean SD g/mL 0.89; P = 0.04). However, a subset of patients exhibited persistent or even increased TSPO binding, indicating ongoing or exacerbated inflammation that corresponded to the cognitive and neurological deficits experienced by stroke patients and thus poorer functional outcomes. These findings highlight the potential of TSPO PET imaging as a valuable tool for assessing post-stroke inflammation dynamics and its implications for stroke treatment strategies and patient prognosis.
Additionally, the impact of intra-arterial ALD401, a stem cell therapy product derived from autologous bone marrow mononuclear cells, on stroke volume reduction has had a significant reduction in stroke volume at 90 days post-treatment 22.9 ± 15.5 ml (P = 0.046) in ischemic stroke patients (25). These results suggest that ALD401 cell therapy may offer a beneficial intervention for reducing stroke-related brain damage in certain stroke patients.
3.5.3.1 Predict the early peri- and post-operative stroke complications
Endovascular coiling demonstrated a lower complication rate and shorter recovery time compared to surgical clipping, though both techniques were effective in achieving aneurysm occlusion in ruptured anterior communicating artery aneurysms, suggesting the minimally invasive nature of coiling offers superior patient outcomes (37). Similarly, the use of intraoperative epiaortic scanning significantly reduced early stroke complications in off-pump coronary artery bypass surgery by allowing real-time visualization of aortic atherosclerosis and embolic sources, thus enhancing perioperative safety and outcomes (62). Additionally, cranioplasty performed within 6 to 28 hours post-head injury markedly improved cerebral perfusion parameters, such as cerebral blood flow and cerebrovascular reactivity, with younger patients and those treated earlier showing near-normal perfusion levels, as evidenced by cerebral CT perfusion and transcranial Doppler evaluations (67). These findings underscore the critical role of advanced intraoperative imaging techniques and timely surgical interventions in optimizing patient recovery and improving neurological outcomes in various cerebrovascular and cardiovascular conditions.
3.5.4 Risk stratification for secondary prevention
3.5.4.1 Transient ischemic attacks
A lifestyle counseling intervention was found to significantly reduce modifiable risk factors for stroke recurrence, such as hypertension, diabetes, smoking, and physical inactivity, among patients with minor stroke and TIA (42). Participants who received counseling demonstrated greater adherence to healthy lifestyle behaviors, including regular physical activity, balanced diet, and medication compliance, which contributed to a reduced risk of recurrent stroke and improved overall prognosis. These findings underscore the importance of lifestyle modifications as key components of secondary prevention strategies for mitigating stroke risk and optimizing long-term outcomes in individuals with minor stroke and TIA.
3.5.4.2 Large artery atherosclerotic stroke
Pocket-sized ultrasound scanners have demonstrated high feasibility and reliability for assessing carotid artery intima-media thickness and the presence of plaques, showing sensitivity of 97%, specificity of 63%, and positive and negative predictive values of 93% and 87%, respectively, even when used by inexperienced operators (30). This suggests that such portable devices could be valuable tools for junior doctors, enabling early detection of carotid artery stenosis and subclinical atherosclerosis, thereby potentially reducing the need for more advanced and costly imaging procedures (31). Integrating these devices into routine clinical practice, especially in settings with limited access to specialized equipment, could enhance the efficiency and accessibility of carotid artery screening for cardiovascular risk prediction.
Meanwhile, in a study comparing carotid artery stenting and endarterectomy in patients with symptomatic carotid stenosis, both procedures were effective in stroke prevention, though carotid stenting was associated with a higher incidence of procedural complications (29). The combined use of MRI and CT angiography and portable ultrasound devices may provide comprehensive strategies for optimizing carotid artery disease management to assess restenosis rates and cerebral ischemic lesions, contributing to a cost-effectiveness analysis of the two treatments.
3.5.4.3 Embolic stroke
Carotid free-floating thrombus (FFT) was primarily detected in stroke patients with atherosclerosis and significant carotid stenosis. Symptomatic carotid FFT patients face a higher risk of embolic events than those with asymptomatic thrombi. CT angiogram identified morphological characteristics, such as thrombus size and mobility, as significant embolic risk predictors. A cranial-caudal length threshold of 3.8 mm distinguished FFT from plaque with 88% sensitivity and 83% specificity (27). Additionally, the combination of danhong injections and pitavastatin significantly improved lipid profiles and reduced ischemic lesions in ischemic stroke patients, as evidenced by MRI and CT angiogram scans. Carotid ultrasound showed decreased artery plaque and enhanced cerebral blood flow, suggesting improved recovery and reduced stroke risk in patients receiving this combined therapy (28). Early detection and risk stratification are crucial for guiding management strategies and preventing embolic complications.
3.5.4.4 Predict the stroke severity and outcomes
The levels of copeptin and the calcium-binding protein B (S-100B protein), which function as biomarkers for assessing stroke severity and predicting clinical outcomes, were significantly elevated in stroke patients compared to healthy controls (56). Higher levels of copeptin and S-100B on MRI were associated with increased stroke severity, as evidenced by higher National Institutes of Health Stroke Scale (NIHSS) scores, and were predictive of poorer clinical outcomes, including an increased risk of mortality and disability following a stroke. Furthermore, ferumoxytol-enhanced MRI successfully detected and quantified inflammatory activity within the central nervous system (CNS), which provided valuable insights into disease progression and treatment response (24). Specifically, the imaging technique demonstrated enhanced contrast between inflamed and non-inflamed tissues. Ferumoxytol-enhanced MRI has shown potential as a non-invasive biomarker for monitoring therapeutic interventions and assessing treatment efficacy in patients with brain tumors and other CNS disorders, thereby facilitating more personalized and timely clinical management strategies.
3.5.5 Predict clinical improvements post-stroke rehabilitation
3.5.5.1 Predict the post-stroke cognitive recovery
Dengzhanxixin injection, a traditional Chinese medicinal plant extensively utilized for ischemic cardio-cerebral vascular diseases, has demonstrated notable efficacy in reducing infarct volume, promoting neuronal cell survival in the ischemic penumbra, and preserving mitochondrial function in brain tissue. These effects, as evidenced through MRI and PET scans, suggest their significant potential to improve neurological and cognitive outcomes in acute ischemic stroke patients (32). Similarly, the combination of Buyang Huanwu Decoction, known for enhancing blood circulation, with olanzapine (33), or NeuroAiD II (MLC901), herbal components known for their neuroprotective and anti-inflammatory properties (34), has shown significant improvements in clinical symptoms, neurological function, and dementia severity in vascular dementia patients’ post-stroke, as evaluated through PET and SPECT scans. This integrative approach indicates superior therapeutic benefits compared to conventional therapy alone, offering promising avenues for managing vascular dementia following cerebral ischemic events.
Meanwhile, mindfulness cognitive therapy has demonstrated significant improvements in cognitive function and emotional well-being among stroke survivors undergoing rehabilitation (53), as evidenced by social-psychological, biochemical, and functional MRI (fMRI) measurements (35). Integration of neuroimaging techniques like fMRI and DTI into rehabilitation programs, such as trunk training using motor imagery, offers valuable insights into neural changes associated with motor recovery and balance function, highlighting neural plasticity and structural reorganization in stroke rehabilitation (36).
3.5.5.2 Predict the post-stroke delirium, neglect, and functional recovery
A significant association exists between spatial neglect and the incidence of delirium in stroke patients. Specifically, decreased connectivity of the basal ganglia and right basal forebrain to the brain stem has been shown to predict severe spatial neglect, which increases the risk of delirium during the acute stroke recovery phase (55). Patients with right hemisphere strokes who exhibit offline anosognosia, or a lack of awareness of their spatial neglect deficits, respond less favorably to rehabilitation targeting spatial awareness and attentional deficits compared to those without anosognosia (64).
Virtual reality (VR) technology has provided a reliable and accurate means of evaluating and training visuospatial neglect, offering a more comprehensive and ecologically valid assessment than traditional paper-and-pencil tests (45). VR-based training interventions have proven effective in enhancing visuospatial awareness and reducing neglect symptoms in patients with stroke and neurological deficits. The immersive and interactive nature of VR environments allows for personalized and engaging training experiences, leading to better rehabilitation outcomes.
Furthermore, non-invasive brain stimulation techniques, such as transcranial magnetic stimulation and transcranial direct current stimulation (tDCS), have shown significant improvements in visuospatial neglect symptoms among chronic neglect stroke patients (47). These interventions target specific neural circuits involved in neglect, promoting neural plasticity and facilitating the restoration of spatial awareness and attentional deficits. The effects of non-invasive brain stimulation have been found to be durable, persisting beyond the intervention period, indicating potential long-term benefits for patients with chronic neglect. Collectively, these findings underscore the potential of non-invasive brain stimulation as an innovative and effective treatment approach for alleviating the debilitating symptoms of chronic neglect and improving stroke patient outcomes.
3.5.5.3 Predict the post-stroke audiovisual defects
Individuals experiencing brain damage (in the temporal and/or frontal areas) or migraine attacks exhibited alterations in auditory processing characterized by increased sensitivity to sound stimuli and heightened perception of loudness (38). The neuropsychological assessments were combined with neurophysiological markers, such as electro-encephalography, magnetoencephalography, and MRI, and the auditory thresholds were found to be significantly lower during migraine attacks compared to the interictal period, indicating enhanced auditory sensitivity during brain damage or migraine episodes. These findings suggest a potential link between temporal or frontal brain damage and migraine pathophysiology and alterations in auditory processing, which points to the need for further research on auditory symptoms in these patients. In this regard, the effectiveness of novel remote rehabilitation interventions, incorporating multiple sensory modalities, in improving visual field impairments in patients with post-stroke brain lesions has been reported (46). In this particular study, participants underwent a tailored telerehabilitation programme involving various sensory stimuli, such as auditory and tactile cues, to stimulate residual vision and facilitate visual field expansion. The results indicate promising outcomes, suggesting that multisensory telerehabilitation holds potential as an effective approach for enhancing visual perception, functional abilities, and the search for neural indicators of improvement in stroke individuals with visual field defects.
3.5.5.4 Predict the post-stroke neural plasticity, aphasia, and functional mobility
PET imaging was utilized to visualize and quantify regional cerebral blood flow and glucose metabolism, mapping functional activation patterns during various motor tasks in chronic stroke patients, revealing elevated blood oxygen-level dependent signals in both lesioned and non-lesioned primary motor cortices during unimanual movements with the affected hand (22). Optimal electrode placement and electrical parameters for MRI-based tDCS have been shown to enhance motor recovery in stroke patients, significantly improving the efficacy of tDCS through precise simulation and positioning (21). Additionally, integrating new wearable technologies, such as a hip walking assist robot (49) and MR-compatible hand-induced robotic systems (50), has led to significant improvements in gait and hand function, stride length, walking speed, and overall stability. fMRI data revealed training-induced neuroplasticity associated with motor control, suggesting neural adaptations and increased cortical activation in response to these rehabilitation interventions. These findings underscore the need for personalized assessments to understand neural reorganization and develop targeted rehabilitation strategies.
3.5.5.5 Predict the post-stroke weakness and spasticity
Sarcopenia, a condition characterized by loss of muscle mass and strength, is prevalent among stroke survivors undergoing rehabilitation (63). Post-stroke individuals with sarcopenia initially showed poorer functional outcomes and slower recovery, emphasizing the need to address sarcopenia in post-stroke rehabilitation to optimize recovery and enhance quality of life. Additionally, detailed mapping of innervation zones within spastic muscles using high-density surface electromyography (EMG) and 3D imaging provided precise localization for guiding botulinum toxin injections and understanding neurodegenerative progression post-stroke (48). Furthermore, the study on opposing needling combined with eye acupuncture therapy in post-stroke hemiplegia patients suggests this approach could be a promising treatment option for reducing muscle spasms and improving outcomes in this population (52). These findings may inform the development of more targeted and effective interventions for individuals with neuromuscular disorders characterized by muscle spasticity.
4 Discussion
This review identified various promising applications of nuclear medicine in stroke diagnosis and management. For stroke diagnosis, the best discrimination between stroke and mimics has been observed when utilizing a radiopharmaceutical radiotracer to analyse patients at a high risk of recurrent stroke. For accurate detection of ICH, the use of an Infrascanner, enhanced flat detector CT, Sence device, or Smartcap blood test show high accuracy in distinguishing ischemic from haemorrhagic strokes. The efficacy of MSU with integrated CT and the use of pre-hospital acute stroke care notification helped assess factors crucial for predicting stroke severity assessment, including time of stroke onset, ischemic penumbra volume at risk, and reperfusion success, and might perform better if combined with selected specific brain blood biomarkers. For treatment precision, the incorporation of MRI-based biomarkers, quantifying non-heme iron levels, and MRI-based stroke mechanisms for 4D flow nuclear imaging into scoring systems may identify patients with clinical diffusion mismatches, thereby enabling more precise personalized risk stratification to reduce stroke burden. The integration of radionuclide angiography and a novel PET radiotracer, namely 18F glycoprotein 1, might achieve sufficient sensitivity and specificity to effectively detect thrombi in various anatomical locations across different clinical models. Generally, visualizing and quantifying blood flow speeds to determine the degree of carotid artery stenosis could refine patient selection for stenting or carotid endarterectomy. For stroke prognosis, plasma copeptin and S-100 b protein assay can be added to NIHSS to predict stroke severity and functional outcomes. Through techniques like SPECT and PET with radiotracers of TSPO, co-registration with ferumoxytol-enhanced MRI and CT for functional imaging allows for valuable insights into cerebral perfusion, metabolic activity, neuroinflammatory markers, and receptor binding. To predict post-stroke rehabilitation outcomes, the efficacy of a new wearable hip walking-assist robot and 3D imaging of the botulinum neurotoxin may optimize functional recovery and promote neural plasticity post-stroke.
In the context of the existing literature on nuclear medicine, the novel diagnostic radiotracer 99mTc-omberacetam holds promising clinical implications in the management of the hyperacute phase of ischemic stroke (69) by quantifying the α-amino-3-hydroxy-5-methyl-4-isoxazolepropionic acid receptors, which are thought to cause excitotoxicity in brain ischemia. Such quantification not only enhances the accuracy of stroke diagnosis but also provides valuable insights into disease progression and responses to therapy. As research continues to advance, the integration of nuclear medicine into routine clinical practice has the potential to revolutionize stroke management, offer clinicians enhanced diagnostic capabilities, and further improve stroke care.
The application of artificial intelligence (AI) in diagnosing acute stroke using CT and MRI imaging has shown significant advancements and potential. AI systems, particularly those leveraging machine learning and deep learning techniques, offer a level of precision and efficiency that surpasses traditional methods. These AI systems can process and analyze large volumes of imaging data swiftly, identifying subtle patterns and anomalies that may be missed by human reviewers. For instance, convolutional neural networks are particularly effective in image classification tasks, allowing for the automated detection and characterization of stroke-related changes in brain scans with high accuracy (70). Platforms like RapidAI (71) utilize sophisticated algorithms to assist clinicians in making timely and accurate decisions by quickly interpreting CT and MRI scans for suspected stroke cases. These tools not only enhance diagnostic accuracy but also streamline workflow, reduce turnaround times, and facilitate rapid treatment decisions, which are crucial in acute stroke management. The integration of AI in stroke diagnosis thus holds the promise of improving patient outcomes through more efficient and precise imaging analysis, ultimately advancing the field of stroke and neuroradiology.
5 Implications for future research
Comparing non-nuclear medicine imaging techniques such as CT and MRI to nuclear medicine imaging in stroke diagnosis reveals distinct advantages and disadvantages. CT and MRI are favored for their rapid acquisition and excellent anatomical detail, essential for quickly identifying hemorrhagic and ischemic strokes. CT excels in acute settings due to its speed, while MRI provides superior soft tissue contrast, making it sensitive to subtle ischemic changes. However, these modalities primarily offer structural insights, potentially missing early functional or metabolic alterations. Conversely, nuclear medicine techniques like PET and SPECT provide invaluable functional and metabolic data, detecting cerebral blood flow and glucose metabolism changes before structural damage becomes apparent. Despite their higher costs, longer acquisition times, and the use of radioactive tracers, nuclear imaging’s ability to highlight early functional disruptions presents a crucial complement to the anatomical clarity of CT and MRI, thereby enriching the diagnostic and management landscape of stroke (72).
The integration of nuclear medicine techniques with traditional imaging modalities represents a significant advancement in the diagnosis and management of stroke. These nuclear methods provide unparalleled insights into cerebral physiology by enabling the visualization of regional blood flow, metabolic activity, and receptor binding in the brain, which are critical for identifying viable but functionally compromised tissue. This functional imaging capability allows for the detection of ischemic penumbra—areas that are at risk but not yet infarcted—thus guiding therapeutic interventions like reperfusion therapy more accurately. When combined with the anatomical precision of CT and MRI, nuclear medicine techniques enhance the diagnostic accuracy and prognostic assessment of stroke. This multimodal approach not only improves the identification of stroke subtypes but also aids in monitoring the effectiveness of treatments, tailoring rehabilitation strategies, and ultimately enhancing patient outcomes. By marrying the anatomical and functional data, clinicians can make more informed decisions, advancing the overall care continuum for stroke patients.
There are shortcomings of nuclear medicine strategies that should be resolved through the introduction of novel radiopharmaceuticals and radiological techniques. Given the multifactorial etiological nature of stroke, the potential to apply nuclear medicine to multimodal neuroimaging paradigms may allow for sufficient sensitivity and specificity to address the heterogeneity of stroke. De facto future research should concentrate on nanotechnological technique optimization and standardization in subacute and acute phases of stroke care, together with improvements in clinical activity workflows, to further advance risk stratification, clinical decision making, and personalized diagnosis and management while continuing to consider the unique characteristics of each patient.
6 Strengths and limitations
This study provides clinicians and researchers with a detailed and systematic approach to screening and filtering a large number of clinical trials, ensuring the inclusion of high-quality and relevant data. The detailed characterization of trial designs, geographical distribution, and participant demographics enhances the study’s generalizability and relevance across different populations and settings. Furthermore, the rigorous risk of bias assessment provides confidence in the reliability of the findings, while the diverse range of outcome measures and diagnostic techniques examined in the included trials underscores the study’s thoroughness and depth in exploring the multifaceted applications of nuclear medicine in stroke care. Nevertheless, it is imperative to acknowledge that the presented analysis has some limitations, there may be bias in favor of the limited number of clinical trials with interventional measures registered in recent years compared to those conducted in earlier periods. Although the study is primarily focuses on adult populations, future reviews could benefit from a more detailed examination of pediatric stroke trials, despite their rarity, to assess the impact of nuclear medicine on this subgroup, providing insights that could refine diagnostic and therapeutic approaches tailored for younger patients.
The application of nuclear medicine techniques in stroke management is fraught with challenges. These techniques are often expensive and require specialized radiologists and equipment, which limits their widespread use. For instance, not all healthcare facilities have the resources and expertise to perform SPECT or PET scanning. The injection of radioactive materials associated with radiation exposure also poses problems, and the high cost of these imaging procedures can also hinder their routine use and availability. While nuclear medicine techniques can provide a comprehensive view of cerebral function and blood flow, some divergence from the actual pathology of stroke is undeniable. Additional imaging data from other procedures, such as CT or MRI, may be needed to complement the information obtained from nuclear medicine images. As the world grapples with these limitations, demand continues to increase for new radiopharmaceuticals and imaging technologies that are more affordable, convenient, precise, and readily available. Accordingly, a comprehensive approach utilizing multiple imaging techniques is still often required for accurate stroke diagnosis and management.
7 Conclusion
This review provides valuable insights into the clinical trials in nuclear medicine that could advance the diagnosis and management of patients with stroke. Although some evidence suggests a potential diagnostic and therapeutic impact of nuclear medicine on defining stroke localization, calculating neurophysiological scores, documenting changes in the late phase of stroke progression, determining responses to thrombolytic therapy, and obtaining information on the presence of sufficient collateral blood supply, conflicting evidence has also highlighted its limited discrimination between stroke and stroke mimics. Rigorous studies are warranted to further integrate nuclear medicine into routine stroke care, with a focus on improving diagnostic and treatment protocols and maximizing stroke patient outcomes.
Data availability statement
The original contributions presented in the study are included in the article/supplementary material, further inquiries can be directed to the corresponding author.
Author contributions
HA: Conceptualization, Data curation, Formal analysis, Funding acquisition, Investigation, Methodology, Project administration, Resources, Software, Supervision, Validation, Visualization, Writing–original draft, Writing–review and editing.
Funding
The authors declare that no financial support was received for the research, authorship, or publication of this article.
Acknowledgments
We thank Dr. Tiaf Alharbi, Pharm.D., at the college of pharmacy, Umm Al-Qura University, Saudi Arabia, for her contribution in the trial’s searches and identification.
Conflict of interest
The author declares that the research was conducted in the absence of any commercial or financial relationships that could be construed as a potential conflict of interest.
Publisher’s note
All claims expressed in this article are solely those of the authors and do not necessarily represent those of their affiliated organizations, or those of the publisher, the editors and the reviewers. Any product that may be evaluated in this article, or claim that may be made by its manufacturer, is not guaranteed or endorsed by the publisher.
References
1. GBD 2019 Stroke Collaborators. Global, regional, and national burden of stroke and its risk factors, 1990–2019: a systematic analysis for the global burden of disease study 2019. Lancet Neurol. (2021) 20:795–820. doi: 10.1016/S1474-4422(21)00252-0
2. Giuseppe M, Bohumir P, Petr W. The importance of time: Time delays in acute stroke. Cor et Vasa. (2016) 58:e225–32. doi: 10.1016/j.crvasa.2016.03.002
3. Jeffrey LS. Time is brain—quantified. Stroke. (2006) 37:263–6. doi: 10.1161/01.STR.0000196957.55928.ab
4. Sunil AS, Reza J, Jan G, Vitor MP, Raul GN, Elad IL, et al. SWIFT-STAR Trialists. Time to endovascular reperfusion and degree of disability in acute stroke. Ann Neurol. (2015) 78:584–93. doi: 10.1002/ana.24474
5. Hughes SJ. Nuclear medicine functional imaging of the brain. Clin Med. (2012) 12:364–8. doi: 10.7861/clinmedicine.12-4-364
6. Usha J. Functional cerebral SPECT and PET Imaging. J Nucl Med. (2010) 51:1326–7. doi: 10.2967/jnumed.110.076901
7. Latchaw RE, Alberts MJ, Lev MH, Connors JJ, Harbaugh RE, Higashida RT, et al. Recommendations for imaging of acute ischemic stroke. Stroke. (2009) 40:3646–78. doi: 10.1161/STROKEAHA.108.192616
8. Yaghi S, Bernstein RA, Passman R, Okin PM, Furie KL. Cryptogenic stroke: research and practice. Circ Res. (2017) 120:527–40. doi: 10.1161/CIRCRESAHA.116.308447
9. Briard JN, Zewude RT, Kate MP, Rowe BH, Buck B, Butcher K, et al. Stroke mimics transported by emergency medical services to a comprehensive stroke center: The magnitude of the problem. J Stroke Cerebrovasc Dis. (2018) 27:2738–45. doi: 10.1016/j.jstrokecerebrovasdis.2018.05.046
10. Stewart LA, Clarke M, Rovers M, Riley RD, Simmonds M, Stewart G, et al. Preferred reporting items for a systematic review and meta-analysis of individual participant data: The PRISMA-IPD statement. JAMA. (2015) 313:1657–65. doi: 10.1001/jama.2015.3656
11. Azhari HF, Alharbi T. Advancing stroke diagnosis and management through nuclear medicine: A systematic review of ClinicalTrials.gov. PROSPERO international prospective register of systematic reviews. (2024). Available online at: https://www.crd.york.ac.uk/prospero/display_record.php?ID=CRD42024541680 Accessed February 30, 2024
12. NIH U.S National library of medicine.Metaregister of controlled trials. (2024). Available online at: https://clinicaltrials.gov/ (Accessed February 30, 2024).
13. Higgins JPT, Thompson SG, Deeks JJ, Altman DG. Measuring inconsistency in meta-analyses. BMJ. (2003) 327:557–60. doi: 10.1136/bmj.327.7414.557
14. Sterne JAC, Hernán MA, Reeves BC, Savović J, Berkman ND, Viswanathan M, et al. ROBINS-I: a tool for assessing risk of bias in non-randomized studies of interventions. BMJ. (2016) 355:i4919. doi: 10.1136/bmj.i4919
15. SPSS.The statistical package for the social sciences (SPSS) software. (2024). Available online at: https://www.jmp.com/en_gb/download-jmp-free-trial.html (Accessed February 30, 2024).
16. Cochrane Community.The RevMan 5 download | Cochrane Community. (2024). Available online at: https://training.cochrane.org/online-learning/core-software-cochrane-reviews/revman (Accessed February 30, 2024).
17. ROBINS-I.The Risk of bias in non-randomized studies - of interventions (ROBINS-I) tool | Cochrane methods. (2024). Available online at: https://mcguinlu.shinyapps.io/robvis/ (Accessed February 30, 2024).
18. NCT 03943966.In-vivo thrombus imaging with 18F-GP1, a novel platelet PET radiotracer (iThrombus). (2024). Available online at: https://clinicaltrials.gov/study/nct03943966 (Accessed February 30, 2024).
19. Brown RB, Tozer DJ, Loubiere L, Harshfield EL, Hong YT, Fryer TD, et al. Toward defining the preclinical stages of Alzheimer’s disease: recommendations from the National Institute on Aging-Alzheimer’s Association workgroups on diagnostic guidelines for Alzheimer’s disease. Alzheimer Dementia. (2024) 20:3852–63. doi: 10.1002/alz.13830
20. NCT 03662750.TSPO PET as a measure of post-stroke brain inflammation: a natural history cohort. (2024). Available online at: https://clinicaltrials.gov/study/nct03662750 (Accessed February 30, 2024).
21. Yoon MJ, Park HJ, Yoo YJ, Oh HM, Im S, Kim TW, et al. Electric field simulation and appropriate electrode positioning for optimized transcranial direct current stimulation of stroke patients: an in Silico model. Sci Rep. (2024) 14:2850. doi: 10.1038/s41598-024-52874-y
22. NCT 03584425.Imaging laterality in chronic stroke patients. (2024). Available online at: https://clinicaltrials.gov/study/nct03584425 (Accessed February 30, 2024).
23. Wu O, Christensen S, Hjort N, Dijkhuizen RM, Kucinski T, Fiehler J, et al. Characterizing physiological heterogeneity of infarction risk in acute human ischaemic stroke using MRI. Brain. (2006) 129:2384–93. doi: 10.1093/brain/awl183
24. NCT 02452216.Using ferumoxytol-enhanced MRI to measure inflammation in patients with brain tumors or other conditions of the CNS. (2024). Available online at: https://clinicaltrials.gov/study/nct02452216 (Accessed February 30, 2024).
25. Atchaneeyasakul K, Dharmadhikari S, Sidani C, Ramdas K, Guada L, Pafford R, et al. Intra-arterial ALD401 cell therapy is associated with reduction in stroke volume at 90 days in a subset of the RECOVER-stroke trial. Stroke. (2016) 47:ATM32. doi: 10.1161/str.47.suppl_1.tmp32
26. NCT 02809651.Confounding factors in the detection of intracranial hemorrhage with the Infrascanner. (2024). Available online at: https://clinicaltrials.gov/study/NCT02809651 (Accessed February 30, 2024).
27. Torres C, Lum C, Puac-Polanco P, Stotts G, Shamy MCF, Blacquiere D, et al. Differentiating carotid free-floating thrombus from atheromatous plaque using intraluminal filling defect length on CTA: A validation study. Neurology. (2021) 97:e785–93. doi: 10.1212/WNL.0000000000012368
28. Xu Y, Chen M, Xiao Q, Zhao Y, Tian J. Observation of the clinical effects of danhong injections combined with pitavastatin on blood lipid regulation in patients with ischemic strokes complicated with lipid abnormalities. Int J Clin Exp Med. (2019) 12:3364–75.
29. Featherstone RL, Dobson J, Ederle J, Doig D, Bonati LH, Morris S, et al. Carotid artery stenting compared with endarterectomy in patients with symptomatic carotid stenosis (International Carotid Stenting Study): a randomised controlled trial with cost-effectiveness analysis. Health Technol Assess. (2016) 20:1–94. doi: 10.3310/hta20200
30. Skjetne K, Graven T, Haugen BO, Salvesen O, Kleinau JO, Dalen H. Diagnostic influence of cardiovascular screening by pocket-size ultrasound in a cardiac unit. Eur J Echocardiogr. (2011) 12:737–43. doi: 10.1093/ejechocard/jer111
31. NCT 00574457.Improving cardiovascular risk prediction using handheld carotid ultrasonography study (MERC-CIMT). (2024). Available online at: https://clinicaltrials.gov/study/nct00574457 (Accessed February 30, 2024).
32. Haiting A, Wuhai T, Ying L, Peng L, Min L, Xiaxia Z, et al. Dengzhanxixin injection ameliorates cognitive impairment through a neuroprotective mechanism based on mitochondrial preservation in patients with acute ischemic stroke. Front Pharmacol. (2021) 12:712436. doi: 10.3389/fphar.2021.712436
33. Zhang L, Wu D, Chen N. Effect of combined use of Buyang Huanwu decoction and olanzapine on clinical symptoms, neurological function, and degree of dementia in patients with vascular dementia after cerebral ischemic stroke. Trop J Pharm Res. (2023) 22:1951–8. doi: 10.4314/tjpr.v22i9.25
34. Chen CL, Ikram K, Anqi Q, Yin WT, Chen A, Venketasubramanian N. The NeuroAiD II (MLC901) in vascular cognitive impairment study (NEURITES). Cerebrovasc Dis. (2013) 35:23–9. doi: 10.1159/000346234
35. NCT 04759950.Exercise the mind and brain. a multimodal intervention in stroke (Mindfit). (2024). Available online at: https://clinicaltrials.gov/study/nct04759950 (Accessed February 30, 2024).
36. Sui YF, Cui ZH, Song ZH, Fan QQ, Lin XF, Li B, et al. Effects of trunk training using motor imagery on trunk control ability and balance function in patients with stroke. BMC Sports Sci Med Rehabil. (2023) 15(142):753. doi: 10.1186/s13102-023-00753-w
37. Ahmed AZ, Zohdi AM, Zaghloul MS, Elsamman AK. Endovascular coiling versus surgical clipping in the treatment of ruptured anterior communicating artery aneurysm in Cairo University Hospitals. The Egypt J Radiol Nuclear Med. (2013) 44:523–30. doi: 10.1016/j.ejrnm.2013.06.007
38. NCT 02791997.Audition after a lesion and in migraine. (AuditionPostLesion). (2024). Available online at: https://clinicaltrials.gov/study/nct02791997 (Accessed February 30, 2024).
39. ISRCTN 80619088.MR CLEAN-NO IV: Intravenous treatment followed by intra-arterial treatment versus direct intra-arterial treatment for acute ischaemic stroke caused by a proximal intracranial occlusion. ISRCTN registry. (2024). Available online at: https://www.isrctn.com/ISRCTN80619088 (Accessed June 07, 2024).
40. Walter S, Kostopoulos P, Haass A, Keller I, Lesmeister M, Schlechtriemen T, et al. Diagnosis and treatment of patients with stroke in a mobile stroke unit versus in hospital: a randomised controlled trial. Lancet Neurol. (2012) 11:397–404. doi: 10.1016/S1474-4422(12)70057-1
41. Koch PM, Kunz A, Ebinger M, Geisler F, Rozanski M, Waldschmidt C, et al. Influence of distance to scene on time to thrombolysis in a specialized stroke ambulance. Stroke. (2016) 47:2136–40. doi: 10.1161/STROKEAHA.116.013057
42. Al-Shahi Salman R, Minks DP, Mitra D, Rodrigues MA, Bhatnagar P, du Plessis JC, et al. Effects of antiplatelet therapy on stroke risk by brain imaging features of intracerebral haemorrhage and cerebral small vessel diseases: subgroup analyses of the RESTART randomised, open-label trial. Lancet Neurol. (2019) 18:643–52. doi: 10.1016/S1474-4422(19)30184-X
43. NCT 03038087.A study to test the sense device in patients with intracranial hemorrhage. (2024). Available online at: https://clinicaltrials.gov/study/nct03038087 (Accessed February 30, 2024).
44. NCT 03827720.Early feasibility study of the sense device. (2024). Available online at: https://clinicaltrials.gov/study/nct03827720 (Accessed February 30, 2024).
45. NCT 02258789.Assessment and training visio spatial neglect in a virtual reality environment (RehAtt). (2024). Available online at: https://clinicaltrials.gov/study/nct02258789 (Accessed February 30, 2024).
46. NCT 06341777.Multisensory telerehabilitation for visual field defects (MUST). (2024). Available online at: https://clinicaltrials.gov/study/NCT06341777 (Accessed February 30, 2024).
47. NCT 05466487.Non-invasive brain stimulation as an innovative treatment for chronic neglect patients (NibsNeglect). (2024). Available online at: https://clinicaltrials.gov/study/nct05466487 (Accessed February 30, 2024).
48. NCT 03302741.Imaging of 3D innervation zone distribution in spastic muscles from high-density surface EMG recordings. (2024). Available online at: https://clinicaltrials.gov/study/nct03302741 (Accessed February 30, 2024).
49. Lee SH, Lee HJ, Shim Y, Chang WH, Choi BO, Ryu GH, et al. Wearable hip-assist robot modulates cortical activation during gait in stroke patients: a functional near-infrared spectroscopy study. J Neuroeng Rehabil. (2020) 17:145. doi: 10.1186/s12984-020-00777-0
50. Lazaridou A, Astrakas L, Mintzopoulos D, Khanicheh A, Singhal AB, Moskowitz MA, et al. Diffusion tensor and volumetric magnetic resonance imaging using an MR-compatible hand-induced robotic device suggests training-induced neuroplasticity in patients with chronic stroke. Int J Mol Med. (2013) 32:995–1000. doi: 10.3892/ijmm.2013.1476
51. NCT 05844722.Effectiveness of mindfulness-based cognitive therapy undergoing post stroke rehabilitation. (2024). Available online at: https://clinicaltrials.gov/study/nct05844722 (Accessed February 30, 2024).
52. Liu G, Li T, Zhang H, Yang H, Wang J, Zhang Z, et al. Clinical study of opposing needling combined with eye acupuncture therapy for treatment of muscle spasm in post-stroke hemiplegia patients therapy for treatment of muscle spasm in post-stroke hemiplegia patients. Acupunct Electro-Therap Res. (2023) 48:225–38. doi: 10.3727/036012923X168907527894626
53. NCT 03648957.Lifestyle counselling as secondary prevention in patients with minor stroke and transient ischemic attack. (2024). Available online at: https://clinicaltrials.gov/study/nct03648957 (Accessed February 30, 2024).
54. NCT 00001324.PET scan to study brain control of human movement. (2024). Available online at: https://clinicaltrials.gov/study/nct00001324 (Accessed February 30, 2024).
55. Boukrina O, Kowalczyk M, Koush Y, Kong Y, Barrett AM. Brain network dysfunction in poststroke delirium and spatial neglect: An fMRI study. Stroke. (2022) 53:930–8. doi: 10.1161/STROKEAHA.121.035733
56. NCT 03974464.Copeptin and the S-100b protein in stroke. (2024). Available online at: https://clinicaltrials.gov/study/NCT03974464 (Accessed February 30, 2024).
57. Lyu J, Xiao S, Meng Z, Wu X, Chen W, Wang G, et al. Collateral-core ratio as a novel predictor of clinical outcomes in acute ischemic stroke. Transl Stroke Res. (2023) 14:73–82. doi: 10.1007/s12975-022-01066-9
58. NCT 02576743.Pushing spatiotemporal limits for 4D flow MRI and dynamic MRA in the brain at ultra-high field. (2024). Available online at: https://clinicaltrials.gov/study/nct02576743 Accessed 30 February 2024.
59. NCT 02033291.Blood-brain barrier quantification in cerebral small vessel disease. (2024). Available online at: https://clinicaltrials.gov/study/nct02033291 (Accessed February 30, 2024).
60. Dale N, Tian F, Sagoo R, Phillips N, Imray C, Roffe C. Point-of-care measurements reveal release of purines into venous blood of stroke patients. Purinergic Signal. (2019) 15:237–46. doi: 10.1007/s11302-019-09647-4
61. NCT 04983927.Evaluation of clinical decision support system for scoring of ASPECT score using brain CT images. (2024). Available online at: https://clinicaltrials.gov/study/nct04983927 (Accessed February 30, 2024).
62. NCT 01764763.Intraoperative epiaortic scanning for preventing early stroke after off-pump coronary artery bypass. (2024). Available online at: https://clinicaltrials.gov/study/nct01764763 (Accessed February 30, 2024).
63. NCT 02793362.Measurement of sarcopenia at post-stroke rehabilitation outcome. (2024). Available online at: https://clinicaltrials.gov/study/nct02793362 (Accessed February 30, 2024).
64. Langer KG, Bogousslavsky J. The merging tracks of anosognosia and neglect. Eur Neurol. (2020) 83:438–46. doi: 10.1159/000510397
65. NCT 01829386.Non-heme iron load quantification in the brain MRI of patients with stroke. (2024). Available online at: https://classic.clinicaltrials.gov/ct2/show/NCT01829386?term=NCT+01829386&draw=2&rank=1 (Accessed February 30, 2024).
66. NCT 06213870.Screening of endovascular thrombectomy with MRI in acute ischemic stroke. (2024). Available online at: https://clinicaltrials.gov/study/nct06213870 (Accessed February 30, 2024).
67. Chibbaro S, Di Rocco F, Mirone G, Fricia M, Makiese O, Di Emidio P, et al. Decompressive craniectomy and early cranioplasty for the management of severe head injury: a prospective multicenter study on 147 patients. World Neurosurg. (2011) 75:558–62. doi: 10.1016/j.wneu.2010.10.020
68. NCT 04554368.A novel score to predict risk of symptomatic intracerebral hemorrhage. (2024). Available online at: https://clinicaltrials.gov/study/nct04554368 (Accessed February 30, 2024).
69. Azhari HF, Hashem AM. Quantifying AMPARs with 99mTc-omberacetam: a novel diagnostic radiotracer for ischemic stroke. J Umm Al-Qura Univ Appll Sci. (2023) 10:211–24. doi: 10.1007/s43994-023-00093-y
70. Soun JE, Chow DS, Nagamine M, Takhtawala RS, Filippi CG, Yu W, et al. Artificial intelligence and acute stroke imaging. AJNR Am J Neuroradiol. (2021) 42:2–11. doi: 10.3174/ajnr.A6883
71. RapidAI.RapidAI platform. (2024). Available online at: https://www.rapidai.com/ (Accessed June 30, 2024).
Keywords: ischemic stroke, hemorrhagic stroke, nuclear medicine, diagnosis, management, outcome prediction
Citation: Azhari HF (2024) Advancing stroke diagnosis and management through nuclear medicine: a systematic review of clinical trials. Front. Med. 11:1425965. doi: 10.3389/fmed.2024.1425965
Received: 30 April 2024; Accepted: 19 July 2024;
Published: 19 August 2024.
Edited by:
Chuen-Yen Lau, National Institutes of Health (NIH), United StatesReviewed by:
Hanfei Wang, National Institutes of Health (NIH), United StatesFeng Li, National Institutes of Health (NIH), United States
Copyright © 2024 Azhari. This is an open-access article distributed under the terms of the Creative Commons Attribution License (CC BY). The use, distribution or reproduction in other forums is permitted, provided the original author(s) and the copyright owner(s) are credited and that the original publication in this journal is cited, in accordance with accepted academic practice. No use, distribution or reproduction is permitted which does not comply with these terms.
*Correspondence: Hala F. Azhari, aGZhemhhcmlAdXF1LmVkdS5zYQ==