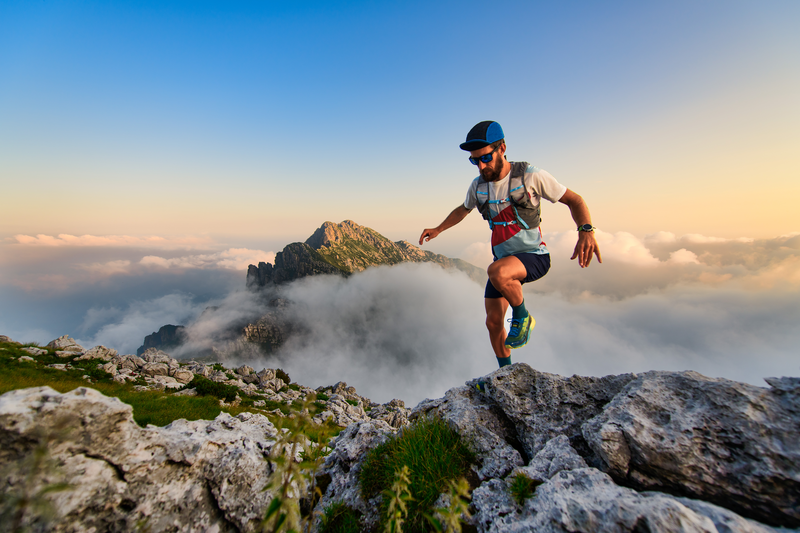
95% of researchers rate our articles as excellent or good
Learn more about the work of our research integrity team to safeguard the quality of each article we publish.
Find out more
ORIGINAL RESEARCH article
Front. Med. , 13 November 2024
Sec. Pathology
Volume 11 - 2024 | https://doi.org/10.3389/fmed.2024.1421727
This article is part of the Research Topic Understanding and Improving Hemostatic Resuscitation Through the Lens of a Bioengineer: Why Surfaces and Materials Matter View all 6 articles
Red blood cells are critical participants in normal hemostasis, but in vitro experiments have shown that the thromboelastogram (TEG) maximum amplitude has a paradoxical inverse relationship with hematocrit. This study reviewed all samples at a single academic institution where any mechanical (TEG 5000) or acoustic (TEG 6s) TEG was drawn within 5 min of hematocrit measurement. A total of 7,176 samples were identified using complete TEG and conventional coagulation test data (6,384 mechanical, 744 acoustic, and 48 both). In the primary analysis, hematocrit was negatively associated with the maximum amplitude on both mechanical and acoustic platforms after adjusting for relevant confounders. This suggests that the thromboelastogram may misrepresent the contribution of red blood cells in normal hemostasis.
Viscoelastic tests (VETs) are a family of coagulation assays that measure clot shear modulus over time and provide a view of several phases of hemostasis ex vivo (1, 2). In typical VETs such as the thromboelastogram (TEG), whole blood is exposed to an activator and then subjected to repeated stress with sound waves or mechanical torsion (3). As the clot evolves, clot initiation, propagation, stabilization, and lysis can be assessed from a single tracing (4). Although they are popular (5), fast (2), and usable at point-of-care, VETs such as the TEG do not offer a complete or reproducible picture of coagulation (6). Many acquired and inborn defects, including Von Willebrand diseases, platelet function disorders, antiplatelet therapies, and antithrombin activity are not assessed by VETs (7, 8). VETs also appear to be insensitive to the hemostatic effects of erythrocytosis and anemia (9).
Anemia is associated with an increased risk of bleeding (10–12). Red blood cells (RBCs) contribute to hemostasis through active and passive mechanisms including increased blood viscosity, mechanical margination of platelets against the vessel wall (13), ADP-dependent enhancement of platelet aggregation (14), improved fibrin network organization (15), and nitric oxide scavenging that increases vascular tone and disinhibits platelets (16). All of these mechanisms suggest that higher hematocrits should contribute to hemostasis and ideally be represented by VETs. However, previously published in vitro studies have demonstrated that the clot maximum amplitude measured on a mechanical TEG (TEG 5000) has a paradoxical inverse relationship with the hematocrit (17). Small studies in clinical patients have also found increased apparent fibrinogen function in VETs in patients with anemia (18, 19). The study hypothesis was that this paradoxical relationship would be preserved in a large clinical sample, and that both mechanical and acoustic (TEG 6s) platforms would demonstrate the same confounding.
This study screened all clinical TEGs from a single academic hospital for samples in which hematocrit was drawn at the same time. Specifically, samples were included if they had any mechanical (TEG 5000TM; Haemonetics, Boston, MA, USA) or acoustic (TEG 6sTM) (19) TEG drawn within 5 min of a hematocrit or hemoglobin, and the results were available in the electronic health record. All times were based on the time of sample draw. All TEGs were performed in the core laboratory. All mechanical TEGs were activated with calcium and kaolin. Acoustic TEGs were run using either the “Trauma” (Global Hemostasis with Lysis®) cartridge or the Global Hemostasis (with Heparinase)® cartridges. In either case, we recorded the MA from the “rapid” TEG channel, which is activated with calcium, kaolin, and tissue factor.
Hemoglobin was most often measured using a commercially available blood gas analyzer (ABL800 FLEX; Radiometer, Copenhagen). Approximately 36% of all hemoglobin measurements had an accompanying hematocrit measured on a cell counter in the core laboratory; of those paired measurements, the mean hematocrit: hemoglobin ratio was 3 ± 0.1. If hemoglobin was drawn without a hematocrit, it was converted using the following formula: hematocrit (%) = hemoglobin (g/dL) × 3. Platelet counts (Sysmex XN10s; Sysmex America, Kobe, Japan), activated partial thromboplastin time (aPTT; ACL-700 Series, Werfen, Bedford, MA, USA), international normalized ratio (INR) (19), and Clauss fibrinogen level (19) drawn within 5 min of the index TEG were also recorded, as were age and sex assigned at birth. All the study procedures were approved by the Institutional Review Board of the University of Wisconsin (approval number 2022-1330).
The primary analysis consisted of two multivariate linear models of maximum amplitude: one for the mechanical TEGs and one for the acoustic TEGs. A mixed-model structure was used to account for more than one sample drawn from the same patient. In both models, the platelet count and Clauss fibrinogen levels were included a priori because they were expected to be the primary predictors of the maximum amplitude. All variables were reviewed for normality; if a significant skew was present, the variables were log-transformed and centered prior to inclusion in the model. Other significant variables in the univariate analyses were also included in the preliminary models. These variables were added using both forward and backward selections, and any discrepancies in the final model composition were resolved using the Akaike Information Criterion. Secondary analyses separately modeled the effect of hematocrit on TEG R-time, K-time, and alpha angle for both the mechanical and acoustic platforms. TEG R-time was available for all TEG samples; K-time and alpha angle were available for all mechanical samples and those acoustic samples run using the Global Hemostasis (with heparinase) cartridge.
To verify the results of the multivariate model, a subgroup analysis was planned for patients with normal values on conventional coagulation tests. The intent of this subgroup analysis was to ensure that any relationship between the maximum amplitude and hematocrit observed in the primary analysis was not the result of confounding factors due to the association of anemia with coagulopathy in the setting of bleeding. A simple linear model of the maximum amplitude as a function of the hematocrit was planned without any adjustment for conventional coagulation test values. Normal ranges for conventional coagulation tests were defined as a platelet count ≥150 × 103 cells/μL, an INR ≤ 1.3, and a Clauss fibrinogen ≥200 mg/dL. It was anticipated that at least a fraction of the study samples would meet these criteria, as it is routine practice at the study hospital to draw simultaneous TEGs and conventional coagulation tests at the beginning of all major cardiac, vascular, and liver surgeries to establish a baseline.
All results were analyzed and reported using conventional statistical methods. Continuous variables were reported as means with standard deviations and categorical results as counts with percentages. Univariate comparisons between continuous variables were made with Student's t-test or simple linear regression, and between categorical variables with Pearson's χ2 test. Statistical significance was set at p < 0.05. Any variables significant at p < 0.1 level were considered for inclusion in multivariate models.
There were 2,507,486 available health records during the study period. After applying the inclusion and exclusion criteria, there were 7,176 samples with complete TEG and conventional coagulation test data (6,384 mechanical, 744 acoustic, 48 both) from a total of 2,849 unique patients between November 2006 and July 2023. Among these, there were 793 samples (660 mechanical, 130 acoustic, and 6 both), and the accompanying platelet count, INR, and Clauss fibrinogen were all within their normal ranges. The sample and patient characteristics are summarized in Table 1.
In unadjusted analyses, hematocrit was positively associated with maximum amplitude on both the mechanical (β = 0.35 mm/%, 95% confidence interval [CI] 0.30, 0.40; p > 0.001) and acoustic platforms (β = 0.29 mm/%, 95% CI 0.20, 0.38; p > 0.001). Platelet count, Clauss fibrinogen, INR, and aPTT were all significantly associated with the maximum amplitude on both platforms, and all tests were significantly associated with hematocrit. Among the patient characteristics, age was significantly associated with the maximum amplitude on both platforms, and sex was significantly associated with the maximum amplitude on the acoustic platform but not on the mechanical platform.
The two primary analytical models are listed in Table 2. In the primary analysis of TEG samples run on the mechanical platform, hematocrit was negatively associated with the maximum amplitude after adjusting for platelet count, Clauss fibrinogen, and aPTT, and accounting for repeated measures from the same patient. In the primary analysis of samples run on the acoustic platform, hematocrit was also negatively associated with the maximum amplitude after adjusting for platelet count, Clauss fibrinogen, and age, and accounting for repeated measures from the same patient. Patient sex and INR were not significantly associated with the maximum amplitude in either model.
Table 2. Primary analyses using mixed multivariable models of mechanical thromboelastogram (kaolin) and acoustic thromboelastogram (kaolin & tissue factor) maximum amplitude as a function of hematocrit and other factors among all eligible samples.
In the subgroup analysis of patients with only normal conventional test values, hematocrit was negatively associated with the maximum amplitude as measured on both the mechanical (β = −0.29 mm/%, 95% CI −0.21, −0.36; p < 0.001, Figure 1A) and acoustic platforms (β = −0.22 mm/%, 95% CI −0.10, −0.33; p < 0.001, Figure 1B). No adjustments were made for conventional coagulation test values in this subgroup.
Figure 1. Subgroup analysis using multivariable models of mechanical [(A); n = 666] and acoustic [(B); n = 137] thromboelastogram maximum amplitude among samples with only normal conventional coagulation values. Plots represent actual values of maximum amplitude vs. hematocrit, overlaid with a simple linear regression model of the two variables. The band around the line represents the 95% confidence interval for the model. Hematocrit was inversely associated with maximum amplitude after adjusting for patient age on both mechanical (β = −0.29 mm/%, 95% CI −0.21, −0.36; r2 = 0.08, p < 0.001) and acoustic (β = −0.22 mm/%, 95% CI −0.10, −0.33; r2 = 0.09 p < 0.001) platforms. No adjustment was made for any other factors. Normal ranges for conventional coagulation tests were defined as a platelet count ≥150 × 103 cells/μL, an international normalized ratio ≤ 1.3, and a Clauss fibrinogen ≥200 mg/dL.
In secondary analyses, hematocrit was associated with other fibrinogen-associated TEG parameters. On the mechanical platform, there was an inverse association with the alpha angle (β = −0.17 mm/°, 95% CI −0.20, −0.14; p < 0.001) and a positive association with the K time (β = 0.04 mm/min, 95% CI 0.03, 0.05; p < 0.001) after controlling for Clauss fibrinogen levels and multiple samples from the same patient. No association with R time was observed after controlling for aPTT. The effects were of similar direction and magnitude for the acoustic platform; however, none were statistically significant (data not shown).
Among acoustic samples, we noted that 619 (78%) were run using the Global Hemostasis with Lysis® cartridge, 105 (13%) were run using the Global Hemostasis (with Heparinase)® cartridge, and 68 (9%) were run using both cartridges. When the multivariable model of acoustic MA was stratified by cartridge type, we found no meaningful difference in the magnitude or the direction of the relationship with hematocrit compared to the primary, pooled analysis (Lysis β = −0.21 mm/°, 95% CI −0.26, −0.16; Heparinase β = −0.26 mm/°, 95% CI −0.42, −0.11).
In a large retrospective clinical sample, the TEG maximum amplitude had an inverse relationship with hematocrit after controlling for fibrinogen and platelet concentrations. In general, there was a reduction in maximum amplitude of approximately 0.25 mm for each percentage point increase in hematocrit, and this was consistent across a wide range of hematocrits and coagulation test values and on both mechanical and acoustic testing platforms. Although the magnitude of the effect is not large, it is still paradoxical because higher hematocrit values are associated with less bleeding and increased coagulation potential, which would ideally be reflected in a higher clot shear modulus, as measured by TEG.
Normal clots are formed by the interplay of clotting enzymes and cofactors, platelets, RBCs, the physics of shear flow, blood pressure, vascular tone, temperature, pH, and the extent of endothelial injury. RBCs promote and support hemostasis in vivo (10–16), and some of the prothrombotic effects of RBCs will still function in a TEG chamber. However, because the testing volume of whole blood in TEG is fixed, any increase in the hematocrit must be accompanied by a decrease in the total mass of available fibrinogen. Fibrinogen loss may also be the result of differences in handling between whole blood TEG samples and conventional coags tests, which are performed in plasma. Fibrinogen is a more potent contributor to the clot shear modulus than platelet quantity or RBCs (20); thus, a decrease in the total fibrinogen mass would result in a decreased maximum amplitude. In this situation, the increase in the hematocrit and decrease in total fibrinogen mass would also result in a lower alpha angle and increased K time, which was observed in this study (1). Although the current study can only identify statistical associations, displacement of fibrinogen mass appears to be the most likely hypothesis to explain how hematocrit would be negatively associated with maximum amplitude, regardless of the true hemostatic potential of the whole blood.
A study of 7,176 clinical TEG and hematocrit samples suggests that TEG misrepresents the contribution of RBCs to normal hemostasis. Given the dominant contributions of fibrinogen and platelets to clot shear modulus, this effect is likely to be minor in most patients with bleeding. Further research is required to quantify the clinical implications of this phenomenon.
The raw data supporting the conclusions of this article will be made available by the authors, without undue reservation.
The studies involving humans were approved by University of Wisconsin Institutional Review Board. The studies were conducted in accordance with the local legislation and institutional requirements. The ethics committee/institutional review board waived the requirement of written informed consent for participation from the participants or the participants' legal guardians/next of kin because minimal risk of harm from retrospective, limited data.
AH: Conceptualization, Data curation, Formal analysis, Funding acquisition, Investigation, Methodology, Project administration, Resources, Software, Supervision, Validation, Visualization, Writing – original draft, Writing – review & editing.
The author(s) declare that no financial support was received for the research, authorship, and/or publication of this article.
The author declares that the research was conducted in the absence of any commercial or financial relationships that could be construed as a potential conflict of interest.
All claims expressed in this article are solely those of the authors and do not necessarily represent those of their affiliated organizations, or those of the publisher, the editors and the reviewers. Any product that may be evaluated in this article, or claim that may be made by its manufacturer, is not guaranteed or endorsed by the publisher.
1. Carll T, Wool GD. Basic principles of viscoelastic testing. Transfusion. (2020) 60:S1–S9. doi: 10.1111/trf.16071
2. Gonzalez E, Moore EE, Moore HB, Chapman MP, Chin TL, Ghasabyan A, et al. Goal-directed hemostatic resuscitation of trauma-induced coagulopathy: a pragmatic randomized clinical trial comparing a viscoelastic assay to conventional coagulation assays. Ann Surg. (2016) 263:1051–9. doi: 10.1097/sla.0000000000001608
3. Wool GD, Carll T. Viscoelastic testing: critical appraisal of new methodologies and current literature. Int J Lab Hematol. (2023) 45:643–58. doi: 10.1111/ijlh.14144
4. Wegner J, Popovsky MA. Clinical utility of thromboelastography: one size does not fit all. Semin Thromb Hemost. (2010) 36:699–706. doi: 10.1055/s-0030-1265286
5. DeSimone RA, Hess AS, Rajendran P, Tanaka KA, Cushing MM, Eichbaum Q. Blood utilization in liver transplantation (BUILT): a multidisciplinary survey of transfusion practices. Transfusion. (2023) 63:83–91. doi: 10.1111/trf.17180
6. Pham HP, Azad A, Gounlong J, Gutierrez J, Mikrut K, Miller JL, et al. Comparison of viscoelastic testing by rotational torsion and harmonic resonance methods. Am J Clin Pathol. (2021) 156:818–28. doi: 10.1093/ajcp/aqab028
7. Ranucci M, Baryshnikova E. Sensitivity of viscoelastic tests to platelet function. J Clin Med. (2020) 9:189. doi: 10.3390/jcm9010189
8. Regling K, Kakulavarapu S, Thomas R, Hollon W, Chitlur MB. Utility of thromboelastography for the diagnosis of von Willebrand disease. Pediatr Blood Cancer. (2019) 66:e27714. doi: 10.1002/pbc.27714
9. Bolliger D, Seeberger MD, Tanaka KA. Principles and practice of thromboelastography in clinical coagulation management and transfusion practice. Transfus Med Rev. (2012) 26:1–13. doi: 10.1016/j.tmrv.2011.07.005
10. Martí D, Carballeira D, Morales MJ, Concepción R, Del Castillo H, Marschall A, et al. Impact of anemia on the risk of bleeding following percutaneous coronary interventions in patients ≥75 years of age. Am J Cardiol. (2020) 125:1142–7. doi: 10.1016/j.amjcard.2020.01.010
11. Vicente-Ibarra N, Marín F, Pernías-Escrig V, Sandín-Rollán M, Núñez-Martínez L, Lozano T, et al. Impact of anemia as risk factor for major bleeding and mortality in patients with acute coronary syndrome. Eur J Intern Med. (2019) 61:48–53. doi: 10.1016/j.ejim.2018.12.004
12. Westenbrink BD, Alings M, Granger CB, Alexander JH, Lopes RD, Hylek EM, et al. Anemia is associated with bleeding and mortality, but not stroke, in patients with atrial fibrillation: insights from the Apixaban for Reduction in Stroke and Other Thromboembolic Events in Atrial Fibrillation (ARISTOTLE) trial. Am Heart J. (2017) 185:140–9. doi: 10.1016/j.ahj.2016.12.008
13. Fogelson AL, Neeves KB. Fluid mechanics of blood clot formation. Annu Rev Fluid Mech. (2015) 47:377–403. doi: 10.1146/annurev-fluid-010814-014513
14. Silvain J, Abtan J, Kerneis M, Martin R, Finzi J, Vignalou JB, et al. Impact of red blood cell transfusion on platelet aggregation and inflammatory response in anemic coronary and noncoronary patients: the TRANSFUSION-2 study (impact of transfusion of red blood cell on platelet activation and aggregation studied with flow cytometry use and light transmission aggregometry). J Am Coll Cardiol. (2014) 63:1289–96. doi: 10.1016/j.jacc.2013.11.029
15. Aleman MM, Byrnes JR, Wang JG, Tran R, Lam WA, Di Paola J, et al. Factor XIII activity mediates red blood cell retention in venous thrombi. J Clin Invest. (2014) 124:3590–600. doi: 10.1172/jci75386
16. Rother RP, Bell L, Hillmen P, Gladwin MT. The clinical sequelae of intravascular hemolysis and extracellular plasma hemoglobin: a novel mechanism of human disease. Jama. (2005) 293:1653–62. doi: 10.1001/jama.293.13.1653
17. Noorman F, Hess JR. The contribution of the individual blood elements to the variability of thromboelastographic measures. Transfusion. (2018) 58:2430–6. doi: 10.1111/trf.14884
18. Ogawa S, Szlam F, Bolliger D, Nishimura T, Chen EP, Tanaka KA. The impact of hematocrit on fibrin clot formation assessed by rotational thromboelastometry. Anesth Analg. (2012) 115:16–21. doi: 10.1213/ANE.0b013e31824d523b
19. Solomon C, Rahe-Meyer N, Schöchl H, Ranucci M, Görlinger K. Effect of haematocrit on fibrin-based clot firmness in the FIBTEM test. Blood Transfus. (2013) 11:412–8. doi: 10.2450/2012.0043-12
20. Tanaka KA, Egan K, Szlam F, Ogawa S, Roback JD, Sreeram G, et al. Transfusion and hematologic variables after fibrinogen or platelet transfusion in valve replacement surgery: preliminary data of purified lyophilized human fibrinogen concentrate versus conventional transfusion. Transfusion. (2014) 54:109–18. doi: 10.1111/trf.12248
Keywords: hemostasis, thromboelastogram, red blood cells, fibrinogen, hematocrit
Citation: Hess AS (2024) The thromboelastogram is confounded by hematocrit in clinical samples tested on both mechanical and acoustic platforms. Front. Med. 11:1421727. doi: 10.3389/fmed.2024.1421727
Received: 22 April 2024; Accepted: 23 September 2024;
Published: 13 November 2024.
Edited by:
Susan M. Shea, University of Pittsburgh, United StatesReviewed by:
Heidi J. Dalton, Inova Health System, United StatesCopyright © 2024 Hess. This is an open-access article distributed under the terms of the Creative Commons Attribution License (CC BY). The use, distribution or reproduction in other forums is permitted, provided the original author(s) and the copyright owner(s) are credited and that the original publication in this journal is cited, in accordance with accepted academic practice. No use, distribution or reproduction is permitted which does not comply with these terms.
*Correspondence: Aaron S. Hess, QWhlc3M1QHdpc2MuZWR1
Disclaimer: All claims expressed in this article are solely those of the authors and do not necessarily represent those of their affiliated organizations, or those of the publisher, the editors and the reviewers. Any product that may be evaluated in this article or claim that may be made by its manufacturer is not guaranteed or endorsed by the publisher.
Research integrity at Frontiers
Learn more about the work of our research integrity team to safeguard the quality of each article we publish.