- 1Centre for Advanced Medical Research and Training, Usmanu Danfodiyo University Sokoto, Sokoto, Nigeria
- 2Department of Animal Health and Production Technology, Federal Polytechnic Bali, Taraba State, Nigeria
- 3Department of Veterinary Microbiology, Usmanu Danfodiyo University Sokoto, Sokoto, Nigeria
- 4Goethe University Frankfurt, Institute of Medical Microbiology and Infection Control, University Hospital of Frankfurt, Frankfurt, Germany
- 5Department of Veterinary Public and Preventive Medicine, Usmanu Danfodiyo University Sokoto, Sokoto, Nigeria
- 6Department of Medical Biochemistry, Usmanu Danfodiyo University Sokoto, Sokoto, Nigeria
- 7Infectious Disease Research Department, King Abdullah International Medical Research Center, Riyadh, Saudi Arabia
Tick-borne febrile illnesses caused by pathogens like Anaplasma spp., Bartonella spp., Borrelia spp., Ehrlichia spp., Coxiella burnetii, Francisella tularensis, and Rickettsia spp., are significant health concerns in Africa. The epidemiological occurrence of these pathogens is closely linked to the habitats of their vectors, prevalent in rural and semi-urban areas where humans and livestock coexist. The overlapping clinical presentations, non-specific symptoms, and limited access to commercially available in vitro diagnostics in resource-limited settings exacerbate the complexity of accurate diagnoses. This review aimed to systematically extract and analyze existing literature on tick-borne febrile illnesses in Africa, highlighting the diagnostic challenges and presenting an up-to-date overview of the most relevant pathogens affecting human populations. A comprehensive literature search from January 1990 to June 2024 using databases like PubMed, Cochrane Library, Science Direct, EMBASE, and Google Scholar yielded 13,420 articles, of which 70 met the inclusion criteria. Anaplasma spp. were reported in Morocco, Egypt, and South Africa; Francisella spp. in Kenya and Ethiopia; Ehrlichia spp. in Cameroon; Bartonella spp. in Senegal, Namibia, South Africa, and Ethiopia; Borrelia spp. in Senegal, Gabon, Tanzania, and Ethiopia; Coxiella burnetii in 10 countries including Senegal, Mali, and South Africa; and Rickettsia spp. in 14 countries including Senegal, Algeria, and Uganda. Data were analyzed using a fixed-effect model in R version 4.0.1 and visualized on an African map using Tableau version 2022.2. This review highlights the urgent need for improved diagnostics to better manage and control tick-borne febrile illnesses in Africa.
1 Introduction
Ticks are parasitic arachnids that feed on the blood of different hosts including reptiles, birds, and mammals (1). They can be categorized as hard ticks belonging to the suborder Ixodidae or soft ticks within the suborder Argasidae (2). Soft ticks are usually distinguished by their distinctive fast feeding, which lasts approximately 20–30 min. In contrast to ixodid ticks, soft ticks can undergo multiple nymphal stages (Figure 1A) (3). The prerequisite for molting typically involves the consumption of a blood meal from a vertebrate host (4). However, certain tick species might undergo multiple feedings before progressing to the next developmental stage (1, 2). Soft ticks exhibit remarkable longevity as exemplified by Ornithodoros turicata (3). Worldwide, more than 800 tick species have been identified, which are known to transmit a variety of diverse viruses, bacteria, and protozoans (5).
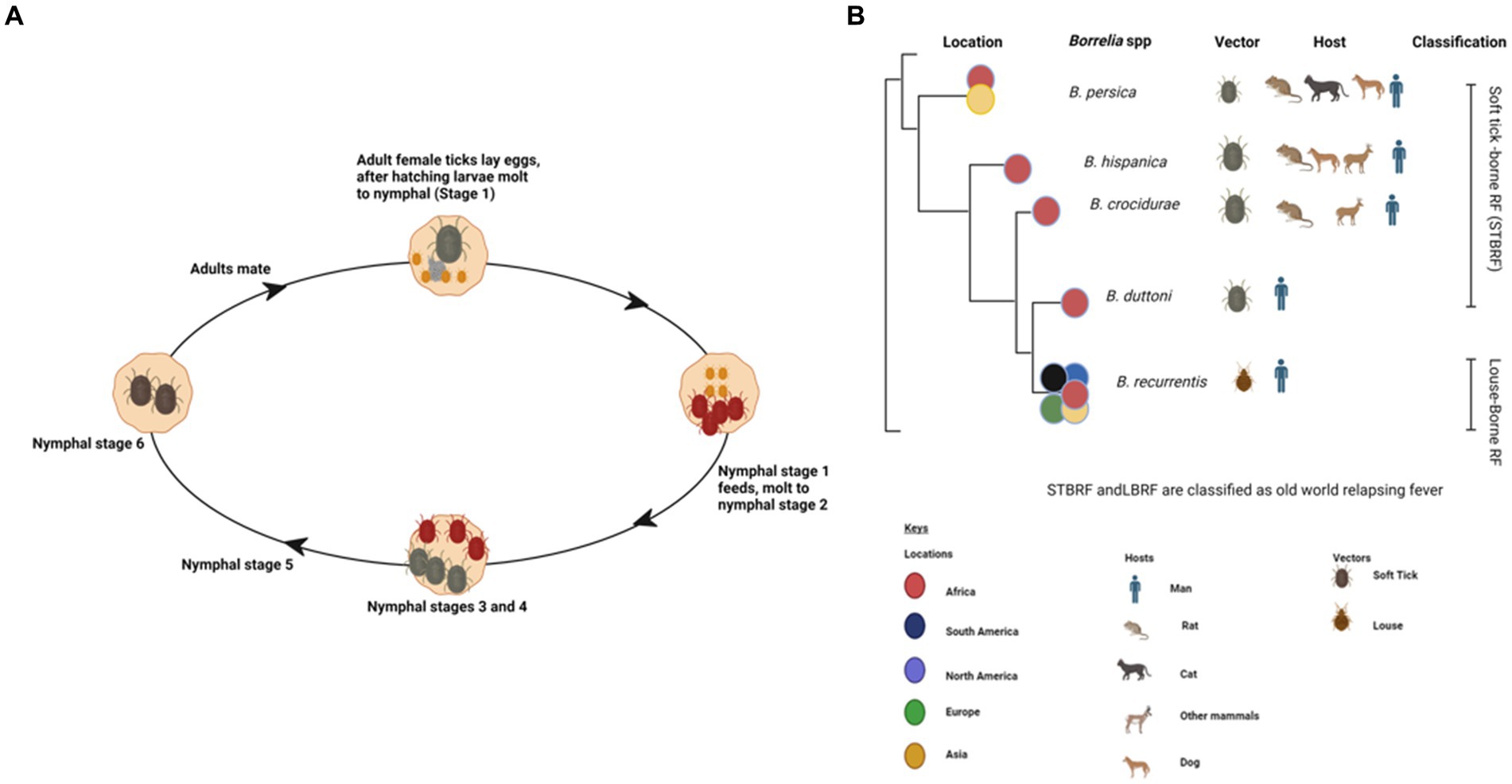
Figure 1. Various life stages of a soft tick (Ornithodoros spp.) created with BioRender (A). Phylodendrogram of average nucleotide identity values among RF borreliae genomes copied and modified from (16) with BioRender, licensed under CC BY. (B).
Ticks are the most significant transmitting vectors of human pathogens after mosquitoes, posing a substantial threat to both human and veterinary health (6). Globally, both vector-borne diseases make substantial contributions to Acute Febrile Illness (AFI) (7). However, Africa is considered to be a hotspot for a multitude of tick-borne pathogens. Recently, the significance of tick-borne diseases has been emphasized, particularly concerning their impact on the well-being of economically disadvantaged farming communities in developing countries (8, 9). In sub-Saharan Africa, there is a significant lack of access to reliable in vitro diagnostics, leading to frequent misdiagnoses, even though an accurate diagnosis is crucial for disease prevention and treatment (10). Currently, the gold standard for diagnosing tick-borne diseases still involves microscopic visualization of Giemsa-stained thick blood smears, known to lack sensitivity and specificity for discriminating the causative pathogen (11). A novel approach for the serodiagnosis of louse-borne relapsing fever with high sensitivity and specificity for both IgM and IgG has recently been developed (12). Alternatively, for the identification of tick species and transmitted pathogens more sensitive methods like DNA amplification techniques are being developed (13). However, the application of molecular techniques is constrained by the necessity for specialized and cost-intensive equipment and well-trained technicians. This review explores the aetiologies of tick-borne febrile illnesses in Africa, providing insight into the diverse causative agents, their epidemiological patterns, geographical distribution, and the challenges faced in distinguishing between these etiological agents.
2 Methodology
A comprehensive literature search was conducted using the following databases: PubMed, Cochrane Library, Science Direct, EMBASE, and the search engine Google Scholar. The search spanned from January 1990 to June 2024 and utilized keywords such as “Anaplasmosis,” “Borreliosis,” “Bartonellosis,” “Q-Fever,” “Coxiella burnetii,” “Borrelia,” “Bartonella,” “Ehrlichia,” “Ehrlichiosis,” “Francisella tularensis,” “Rickettsiosis,” “Tick-borne relapsing fever,” combined with “Africa,” “African countries,” and individual country names within Africa (Figure 2).
2.1 Inclusion criteria
Only studies involving humans infected with Anaplasma phagocytophilum, Bartonella spp., Borrelia spp., Coxiella burnetii (Q-fever), Ehrlichia spp., Francisella tularensis, and Rickettsia spp. Observational studies, including cross-sectional, prevalence, case–control, and cohort studies were included Studies published in English.
2.2 Exclusion criteria
Studies involving animals and ticks without a focus on human infections. Similarly, short reports, incomplete articles, and review articles were excluded.
3 Ticks as vectors of emerging and re-emerging human diseases
Ticks, belonging to the arachnid class, are ectoparasites that feed on blood from reptiles, birds, and mammals. They include three well-established families: Ixodidae or “hard ticks” (694 species), Argasidae or “soft ticks” (177 species), and Nuttalliellidae represented by a single species confined to southern Africa (1, 2, 14). The Ixodidae family is characterized by a three-host life cycle and features four distinct stages: egg, larva, nymph, and adult. Each of these stages typically parasitizes a different host (Figure 3). Prior to feeding, ixodid ticks attach to their hosts, which are often small mammals, for several hours. During the feeding process, ticks can acquire pathogens from the infected hosts (4, 15). In contrast to soft ticks, hard ticks feed only once on a mammalian host, most often small rodents and birds but also humans, before progressing to the next developmental stage, as depicted in Figure 3. Soft ticks have a multi-host life cycle (Figure 1A) (16) that can involve multiple feedings at each stage, but they do not spend extended periods attached to their hosts (Figure 1B). Instead, they feed quickly, often within a matter of minutes to an hour. One remarkable feature of soft ticks is their longevity; for example, Ornithodoros turicata can survive for up to 7 years without feeding and nearly 10 years with periodic blood meals (3). Soft ticks tend to live in close proximity to their hosts, such as in burrows or nests, allowing them to feed repeatedly on the same hosts over time. The transmission of pathogens from one generation of ticks to the next through transovarial means introduces a significant level of complexity to the factors influencing disease maintenance and spread (Figure 1A) (17). This transovarial transmission is observed in both hard and soft ticks, although it is more common and well-studied in hard ticks. Soft ticks, however, can also transmit pathogens through their brief yet repeated feeding sessions, increasing the risk of pathogen spread (17).
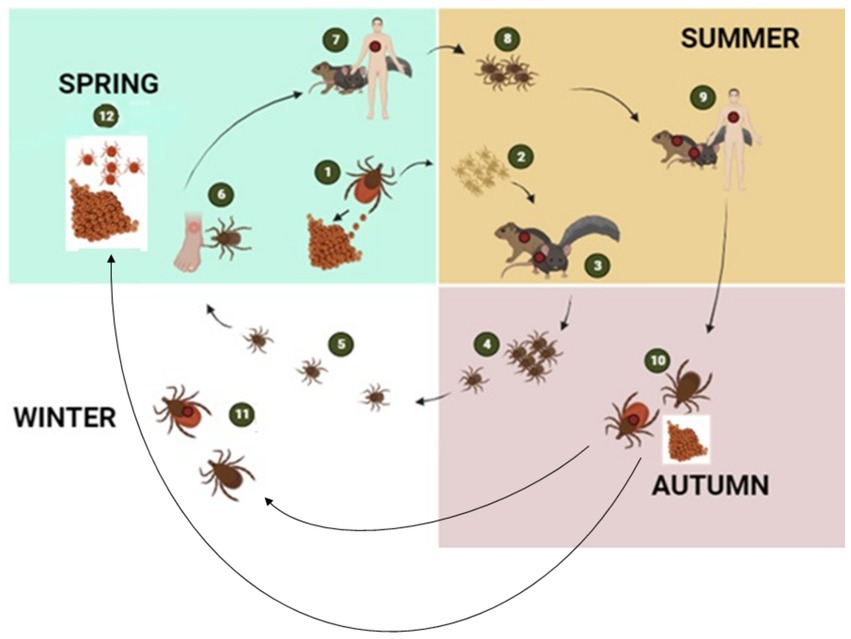
Figure 3. The figure illustrates the transmission cycle of hard ticks through different seasons of the year: The tick life cycle begins with female ticks laying eggs in the spring (1), which hatch in spring or early summer giving rise to larvae (2). During late summer, larvae become infected with bacterial pathogens when they feed on infected mice or other small rodent hosts (3). The larvae then moult into nymphs (4) and overwinter in the environment (5). By the following spring, questing nymphs again feed either on humans (6) or on wild animals (7). After feeding, nymphs drop off the host to molt into adults (8). Adult ticks then continue to feed on larger mammalian hosts including humans and diverse mammalian hosts (9). Engorged female ticks drop off the host to lay eggs and then died (10). Adult ticks remain dormant in the environment during winter when they did not feed on a host (11). With the onset of spring, the cycle repeats by the next generation of larvae (12) (2, 5) created with BioRender.com.
4 Pathogens responsible for human tick-borne bacterial febrile illnesses in Africa
4.1 Anaplasmosis
Human Anaplasmosis is primarily caused by Anaplasma phagocytophilum and A. platys, both are obligate intracellular Gram-negative bacteria (18). Various possibilities of transmission have been documented, including nosocomial infection by direct contact with blood and respiratory secretions, as well as transmission through blood transfusions (19, 20). Anaplasma phagocytophilum is primarily transmitted by various hard tick species within the Ixodes genus (21, 22). While Amblyomma, Dermacentor, Hyalomma, and other ixodid ticks could potentially contribute to the transmission cycle of this bacterium in Africa (23, 24). The prevalence and distribution of these ticks vary, and there are regional differences in the tick species associated with A. phagocytophilum transmission. However, their role as vectors remains uncertain (23).Human anaplasmosis manifests as a febrile illness and is characterized by symptoms such as fever, headache, muscle aches, and fatigue. The disease primarily affects white blood cells, leading to a reduction in their numbers and impacting the immune response (25).
In the African context, A. phagocytophilum has only been identified in soft ticks collected in temperate North Africa (26). In Egypt, infections with A. phagocytophilum have been reported in five individuals, accounting for 7.5% of the studied population (see Table 1; Figure 4), highlighting the significance of understanding the prevalence of tick-borne pathogens in the context of the One Health concept (27).
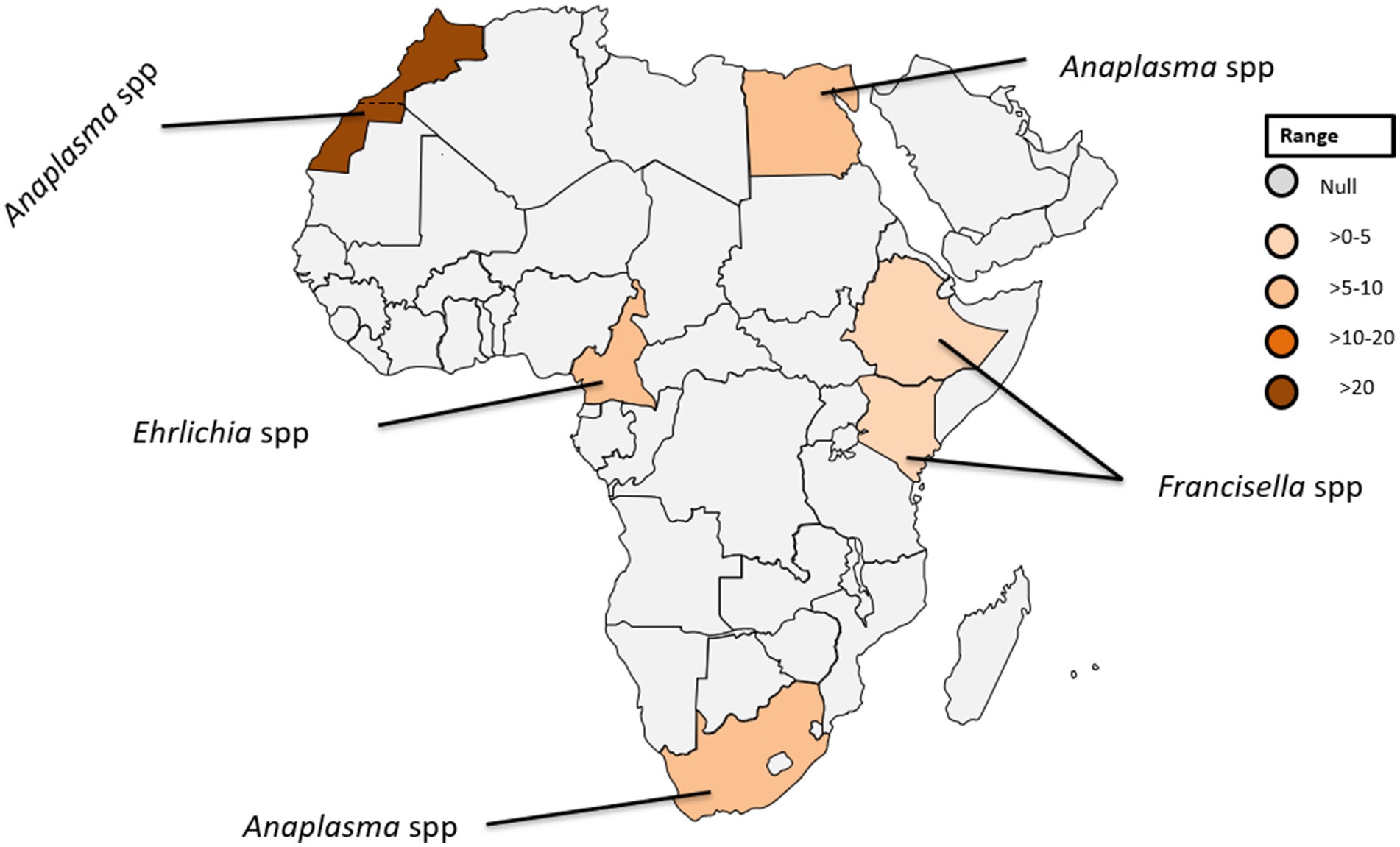
Figure 4. Pooled data using fixed effect model with R version 4.0.1 showing reported Prevalence of Anaplasma spp. Ehrlichia spp. and Francisella tularensis in different African countries designed using Tableau, 2022.2 software.
An investigation dealing with the prevalence and risk factors of A. phagocytophilum exposure in Morocco, including the analysis of seropositivity rates among dog handlers and blood donors, disclosed rates of 37 and 27% among dog handlers and 36 and 22% among blood donors, respectively. This revealed no statistically significant differences between the two groups, underscoring the overall frequent incidence of A. phagocytophilum exposure in both, high-risk populations and blood donors in Morocco (28).
The diagnosis of human anaplasmosis in various African countries has not received sufficient attention in recent years, as no reported studies are found in the selected databases since 2000. This obvious gap of knowledge highlights the need for further investigations aiming to collect data on the prevalence and impact of Anaplasma infections in different countries of Africa. Notably, North Africa has emerged as a focus of research on Anaplasma infections where A. phagocytophilum was initially identified in human body lice obtained from homeless individuals in three distinct cities in northern Algeria. The prevalence of A. phagocytophilum in these arthropods was recorded at 0.76% (29), highlighting the role of body lice as potential vectors for Anaplasma transmission and raising awareness among public health authorities.
Furthermore, intriguingly, a potentially novel species of Anaplasma spp. was identified in head lice collected from patients in Mali, with a prevalence of 0.3%, as described by Amanzougaghene et al. (30). Emphasizing the diverse nature of Anaplasma species and the importance of exploring the vector competence of different arthropods will increase the understanding of the transmission dynamics of these particular pathogens.
4.2 Ehrlichiosis
Ehrlichiae are obligate intracellular Gram-negative bacteria belonging to the same family as Anaplasma, exhibiting a predilection for monocytes and granulocytes similar to Anaplasma spp. (31). The prevalence of these human pathogenic microorganisms is widespread worldwide, and their presence significantly impacts the occurrence of ehrlichiosis in both humans and animals (29).
Within the genus Ehrlichia, Ehrlichia (E.) chaffeensis, and E. ewingii stand out as causative agents of ehrlichiosis in humans (30, 32). E. chaffeensis, responsible for human monocytic ehrlichiosis was initially identified in the United States (33). Although there has been a paucity of reported human cases in Africa (34). Remarkably, a singular case was documented in Cameroon where blood samples from 10% of the 118 investigated patients were tested positive for gene-specific E. chaffeensis DNA (35). This finding is noteworthy because the sequence obtained exhibit 100% genetic identity among the positive cases to a strain of E. chaffeensis isolated in Arkansas, USA (36). The detection of E. chaffeensis in Cameroon underscores the global distribution of these pathogens and raises questions about the potential factors influencing their epidemiology in diverse geographic regions. Furthermore, the identification of E. chaffeensis in Cameroon serves as a crucial piece of evidence, shedding light on the presence of tick-borne transmitted human pathogens in sub-Saharan Africa (see Table 1; Figure 4).
Due to the scarcity of reports since 2000, additional research is necessary to thoroughly comprehend the prevalence of potential vectors and the biological factors that influence the epidemiology of Ehrlichiae in Africa. While the genus Ehrlichia includes several species known to cause diseases in animals and humans, research and literature on E. ewingii within the African continent are notably scarce (6). The majority of studies and reports related to Ehrlichia species in Africa have primarily focused on E. chaffeensis and E. canis (6, 35, 37). E. ewingii is recognized for its ability to infect both animals and humans, causing a febrile illness known as human granulocytic ehrlichiosis (HGE) (38). The primary vector responsible for the transmission of E. ewingii is Amblyomma americanum (39). However, there is currently no conclusive evidence confirming the presence of A. americanum, and consequently E. ewingii, in Africa.
4.3 Francisella infection
Francisella, a genus of gram-negative bacteria, has emerged as a significant pathogen with Francisella tularensis, known for causing tularaemia (40). This zoonotic pathogen is highly infectious and primarily transmitted to humans through contact with infected animals, inhalation of contaminated aerosols, ingestion of contaminated water or food, and bites from infected arthropods (41, 42). Tularemia manifests in various types, including the ulceroglandular, glandular, oculoglandular, oropharyngeal, pneumonic, and typhoidal form (43). The clinical presentation can range from mild flu-like symptoms to severe systemic manifestations posing a diagnostic challenge due to its diverse symptoms (43). While historically associated with temperate regions, the prevalence of tularemia in tropical countries is an emerging concern (44). The epidemiology is influenced by factors such as climate, ecosystem dynamics, and interactions between humans and animals (45, 46). Francisella spp. exhibits a broad global distribution, with F. tularensis subspecies distributed across North America, Europe, Asia, and Africa (40–42). In tropical countries, the prevalence may be influenced by ecological factors including the presence of suitable reservoir hosts and vectors (40). In a recent study conducted in Ethiopia, three out of 394 febrile patients were diagnosed with Francisella infection (Figure 4) (14). Diagnosing this infection poses challenges due to the diverse clinical manifestations and the need for specialized laboratory techniques. Serological tests, polymerase chain reaction (PCR), and culture-based methods are commonly employed (15, 47). However, the availability and accessibility of these diagnostic tools in tropical settings may be limited, hindering timely and accurate identification of Francisella infections.
4.4 Bartonellosis
Bartonella spp. are fastidious Gram-negative bacteria responsible for a variety of clinical symptoms summarized as bartonellosis (48). Various Bartonella species have been associated with emerging and re-emerging human diseases (49). Throughout history, Bartonella spp., including B. bacilliformis, B. quintana, and B. henselae, have been recognized as significant contributors to human disease. While these bacteria are known to cause a range of infections, from mild symptoms such as fever, headache, and malaise to more severe conditions like endocarditis and hallucinations, it’s important to note that they are not the sole causative agents of these diseases (48, 49). Bartonellosis implicated as the cause of blood culture-negative endocarditis (BCNE) is discussed in a previous study conducted in South Africa (50). Similarly, Tasher et al. (51) reported B. quintana endocarditis as a rare occurrence in children. The study described five patients from Ethiopia with heart defects and endocarditis caused by either B. quintana or an undetermined Bartonella species. All patients were afebrile and oligosymptomatic, with three experiencing heart failure. The diagnosis was confirmed by echocardiography, high Bartonella IgG titers, and identification of B. quintana DNA in the blood sample. The data suggested that B. quintana is not uncommon in children with heart defects in Ethiopia and should be considered in cases of culture-negative endocarditis (51).
A study by Noden et al. (52) investigated factors related to exposure to B. henselae, revealing a correlation between residing in villages and close association with dogs and cats. Among the 105 samples analyzed for suspected infection with B. henselae, three samples (2.9%) tested positive (see Table 1; Figure 5). Additionally, an individual positive for B. henselae antibodies also exhibited positivity for C. burnetii Phase II antibodies. This finding raises the possibility of coinfections with certain human pathogens circulating in the same area or cross-reactivity, as previously noted (53). The complex interplay between Bartonella and other pathogens warrants further investigation to elucidate potential synergies or interactions in environments where the same vectors circulate.
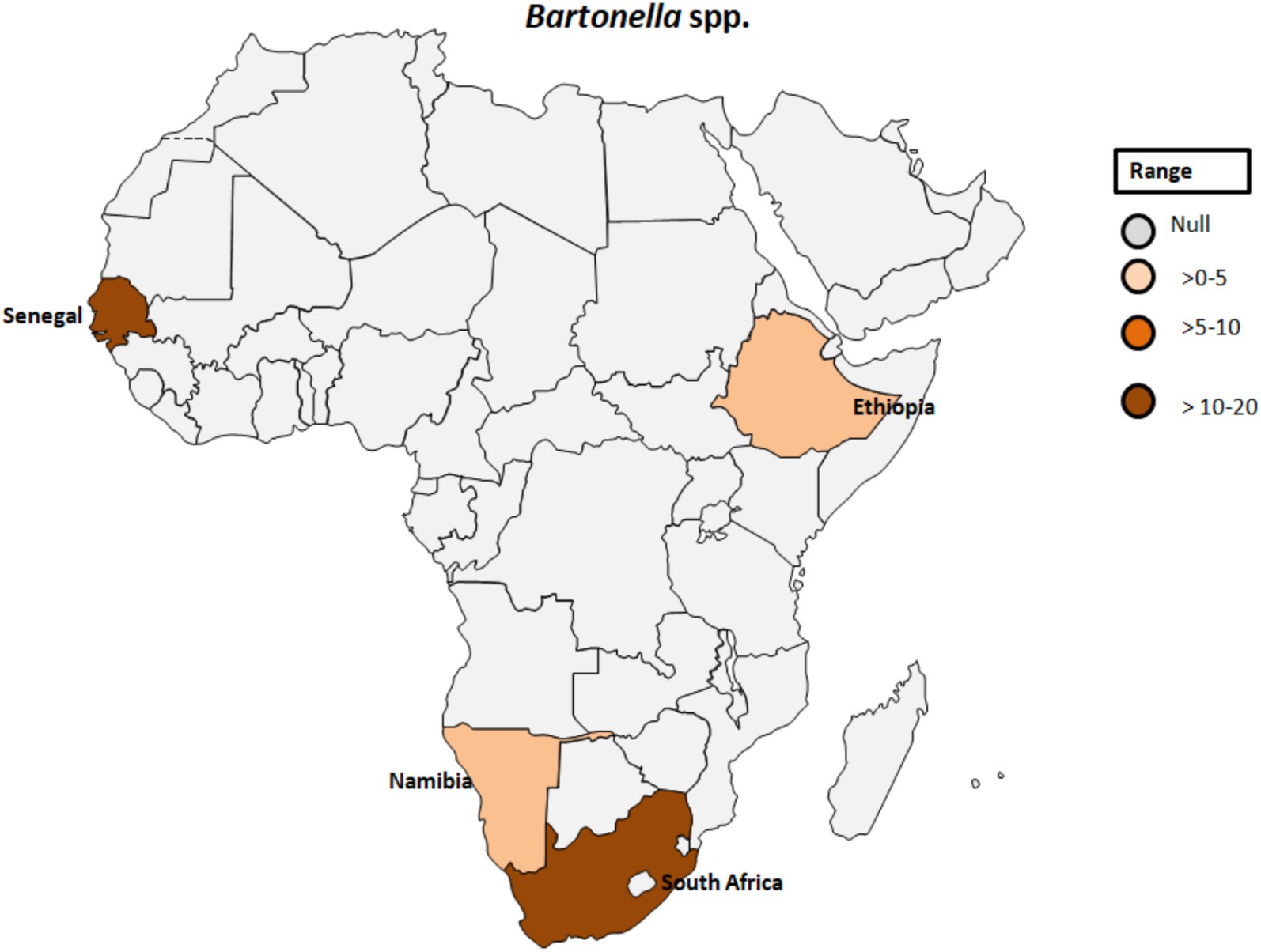
Figure 5. Pooled data using fixed effect model with R version 4.0.1 showing reported Prevalence of Bartonella spp. in different African countries designed using Tableau, 2022.2 software.
Typically, each Bartonella species establishes a specific association with its host, leading to sustained intraerythrocytic bacteremia in the reservoir host, often without detectable harm to the respective host (6, 54). While numerous hematophagous ectoparasites are known to be vectors of Bartonella, ixodid ticks have also been controversially discussed as potential vectors for Bartonella spp. (35).
Arthropods are primary vectors in the spread of Bartonella species among mammals including humans, while fleas play a significant role for their distribution (55). Additionally, ticks have been identified as vectors for certain Bartonella strains, highlighting their involvement in the transmission cycle (56, 57). Moreover, previous studies suggest that lice may also serve as vectors, emphasizing the adaptability of Bartonella to different ectoparasites (58). Notably, the potential for direct transmission from animals to humans through scratches or bites poses another avenue for the spread of Bartonella (49, 59). Overall, arthropodsare crucial vectors for unravelling the epidemiology of Bartonella-related illnesses and devising effective prevention and control strategies.
4.5 Tick-borne relapsing fever
Tick-borne relapsing fever (TBRF) is a vector-borne disease caused by spirochetes belonging to the genus Borrelia (2, 60) including B. duttonii, B. crocidurae, B. hispanica, B. persica, and Candidatus Borrelia kalaharica in Africa and Near East. It is typically transmitted to humans through a bite of infected ticks (Figure 1B) (61–63). In contrast, B. recurrentis, the causative agent of louse-borne relapsing fever (LBRF), is the only Borrelia spp. vectored by lice (Figure 1B) (60–62, 64). TBRF and LBRF are characterized by recurrent episodes of fever, chills, headache, muscle and joint pain as well as other flu-like symptoms (1, 64).
Since the inception of molecular methods, the detection of these pathogens has been verified in different samples obtained from arthropods and animals, with less frequent occurrence in humans from West Africa (9). While TBRF is endemic in different African countries, outbreaks of LBRF are frequently reported from Ethiopia, Eritrea, Somalia, and South Sudan.
Regarding the occurrence of TBRF, 115 out of 1,566 samples were tested positive (7.3%) for B. crocidurae DNA by Mediannikov et al. (65) in Senegal (see Table 1; Figure 6). In addition, clinical cases of TBRF have been documented, particularly in rural areas in Senegal where individuals may have close contact with ticks (66). In this study, B. crocidurae DNA was detected in 7.22% (159/2,202) with a higher prevalence observed during the summer months of July and August.
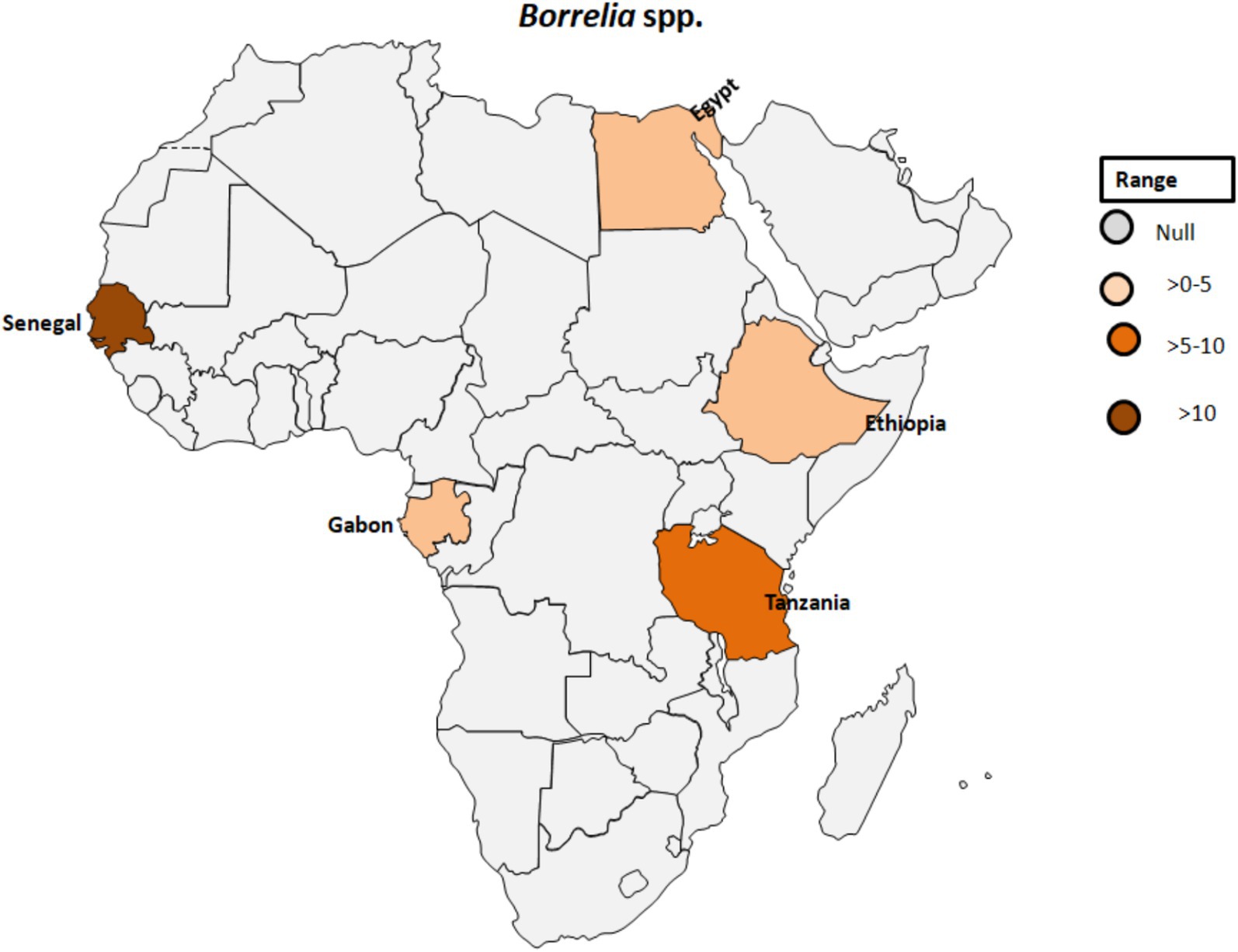
Figure 6. Pooled data using fixed effect model with R version 4.0.1 showing reported Prevalence of Borrelia spp. in different African countries designed using Tableau, 2022.2 software.
Grecchi et al. (67) reported a case of relapsing fever caused by B. recurrentis in a refugee traveling to Europe from Mali. Two out of five incidences of recurrent fever were reported from refugees originated from Somalia in Turin (Italy) (68). Febrile diseases in rural areas of Africa could be caused by bacteria transmitted by arthropods (14). Thirteen patients tested positive for DNA from these pathogens, including Borrelia spp., Francisella group, Rickettsia bellii, Rickettsia felis, and Bartonella rochalimae. This finding suggests that previously ignored organisms like Rickettsia, Bartonella, Francisella, and Borrelia should be considered in empiric therapies, leading to more informed decision-making on the accurate anti-microbial treatment.
A novel Borrelia species closely related to B. crocidurae or B. hispanica was identified in a 24-year-old man with a travel history to Senegal. He presented symptoms including fever, chills, headache, myalgia, arthralgia, and mild diarrhea (69).
In Dielmo, Senegal, a study by Vial et al. (70) reported an average TBRF incidence of 11 cases per 100 person-years over 14 years with variations over time. Another study conducted in Algeria between May 2012 and October 2015 found that 5 out of 48 (10.4%) ticks collected from Larus michahellis nests were positive for Borrelia spp. Further analysis of flaB gene sequences revealed 100% identity with North American genotypes of B. turicatae and a 99.77% identity with another B. turicatae genotype (71). In a study assessing the prevalence of bacteria and Plasmodium spp. in febrile and afebrile children in Franceville, Gabon, Borrelia spp. was detected in two controls, while Rickettsia felis was found in 10 children (8 febrile, 2 afebrile). No DNA of other microorganisms could be detected in this investigation (72).
In Zambia, Ornithodoros faini ticks and bats, particularly Rousettus aegyptiacus, were suggested as potential vector and reservoir hosts, respectively, for a Borrelia species closely related to New World relapsing fever borreliae, raising questions about the evolutionary history and distribution of Borrelia species in Southern Africa (73).
Egyptian farmers living in Nile Delta villages exhibited instances of anaplasmosis and TBRF, with evidence of A. phagocytophilum infection found in five individuals (7.5%) and DNA of B. burgdorferi identified in two samples (3%; Table 1; Figure 6) (27). The relevance of this finding seems to be questionable as Lyme disease Borrelia species are exclusively vectored by ixodid ticks known to be absent in Egypt.
4.6 Q fever
Coxiella burnetii is an obligate intracellular, Gram-negative bacterium and the causative agent of Q fever. This pathogen is transmitted by various ticks such as Ornithodorus sonrai, Amblyomma variegatum, Hyalomma spp., and Rhipicephalus spp. across different countries in Africa (Figure 7) (74). The clinical manifestations of Q fever include a febrile illness, pneumonia, and hepatitis, typically appearing 2 to 3 weeks after infection (75). C. burnetii naturally infects a range of livestock animals, including cattle, goats, and sheep. The bacteria can be found in various organs of infected animals, such as the placenta, as well as in body fluids like amniotic fluid, urine, and milk (76, 77). Humans can become infected through inhalation of C. burnetii-contaminated dust or by consuming unpasteurized milk (78). In Gambia, seropositivity for C. burnetii was reported in 3.8 to 9.7% of adult humans and 24.9% of small ruminants in Kiang West, underscoring the interconnectedness of human and animal exposure to C. burnetii (Figure 7) (79). Seroprevalence studies have also shown the presence of IgG antibodies to various human pathogenic agents, including C. burnetii but also Rickettsia, Leptospira, West Nile fever virus, and dengue virus, among peacekeepers deployed to Southern Sudan (80).
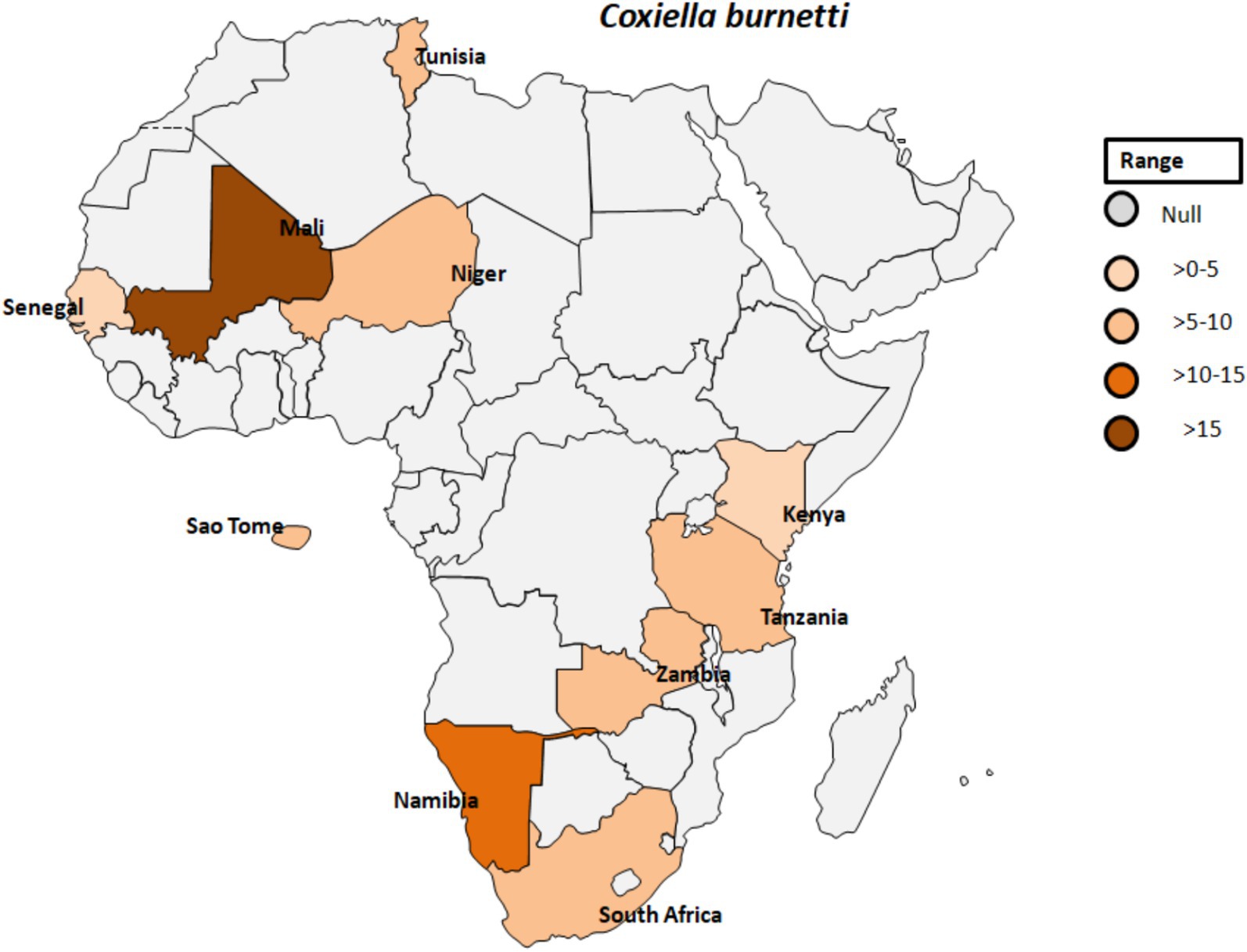
Figure 7. Pooled data using fixed effect model with R version 4.0.1 showing reported Prevalence of Coxiella burnetti in different African countries designed using Tableau, 2022.2 software.
4.7 Rickettsial infection
The Spotted fever group (SFG) rickettsiae comprises a collection of closely related bacteria transmitted to humans primarily through the bite of infected ticks (81, 82). These almost neglected bacteria belong to the genus Rickettsia and are associated with a range of febrile illnesses known as spotted fever (83). The distinctive feature of SFG rickettsioses (SFGR) is the development of a characteristic eschar, a necrotic skin lesion at the site of tick attachment (83). While the SFG includes various species, each with unique clinical presentations, they collectively pose significant public health concerns, particularly in regions where the vectors thrive (84, 85). Understanding the diverse species within this group of human pathogenic bacteria is crucial for effective diagnosis, treatment, and control strategies.
Rickettsia africae, the causative agent of African tick-bite fever, is transmitted by Amblyomma ticks and is endemic in various regions of sub-Saharan Africa (Figure 8). Prior to 2010, Africa reported only one case of Rickettsiosis (86); however, serological evidence of contact with Rickettsiae in Dielmo village, Senegal, revealed that 21.4% of 238 individuals tested positive and 51% of 241 ticks from Ndiop village, Senegal, carried Rickettsia spp. by molecular identification (87). Additionally, a study in Senegal showed that Rickettsia species had a higher prevalence (4.0%) than other bacterial agents in patients with fever of unknown origin, with co-infections of Plasmodium spp., Dengue virus, and Salmonella typhi (88). In Cameroon, 26.9% of the 903 participants tested positive for antibodies reactive to R. africae. However, the observed seroprevalences varied across different regions. Specifically, in Njikwa, the seroprevalence was 51.8%, while in Lomie, it was 38%. In Sobia and Nyabisan, which represent gallery highland and most lowland village sites, the seroprevalences were 37 and 28.7%, respectively (89). This suggests a correlation between the landscapes of the collection sites and the seroprevalence rates among individuals in those regions. In Uganda, rickettsioses and malaria, including Typhus Group Rickettsiosis and SFG Rickettsia, were identified as major causes of acute febrile illness in selected clinics (90). Tick collections from domestic and wild animals in Guinea and Liberia, two neighboring countries in tropical West Africa, revealed Rickettsiae in nine different tick species. R. africae was found in Amblyomma variegatum along with other species such as Rhipicephalus geigyi, Rh. annulatus, Rh. decoloratus, and Amblyomma compressum. A new Rickettsia species, provisionally named Candidatus Rickettsia liberiensis, was identified in Ixodes muniensis collected from a dog in Liberia (91). Diagnosis of human rickettsiosis typically relies on serological methods (7). However, identification of the causative agents by swabbing eschars in patients with skin lesions after traveling to South Africa (92). Molecular tools targeting distinct genes revealed the presence of R. africae in patients who had returned from South Africa, even in cases where serology yielded negative results. A study conducted on 141 adult patients in rural South Africa to determine the prevalence and risk factors of two endemic zoonoses, Q fever and SFGR revealed that 27% of patients were exposed to Coxiella burnetii with a higher prevalence among individuals attending cattle inspection facilities (see Table 1; Figure 8) (93). Additionally, 21% of patients had evidence of acute SFGR with higher odds of seropositivity among females and those attending cattle inspection facilities. A study dealing with the prevalence of different zoonoses and the determination of certain risk factors at human-wildlife-livestock interface at Mpumalanga region of South Africa showed that 77% of febrile individuals and 98% of dip-tanksters had at least one positive test for zoonotic pathogens (94). Detection of two fastidious bacteria, Rickettsia felis and Borrelia spp., in two control samples emphasizes the importance of including controls in the study.
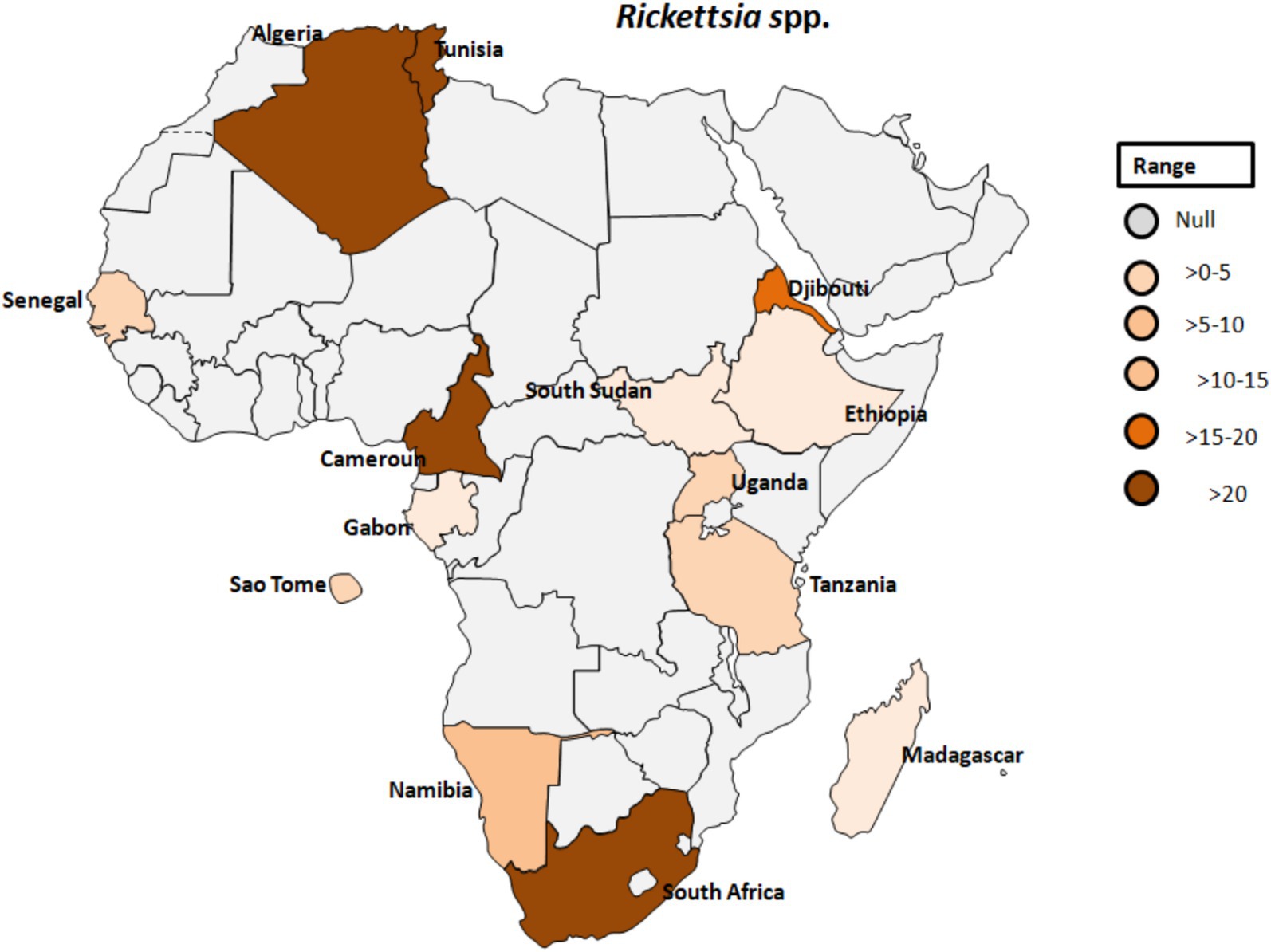
Figure 8. Pooled data using fixed effect model with R version 4.0.1 showing reported Prevalence of Rickettsia spp. in different African countries designed using Tableau, 2022.2 software.
Furthermore, Rickettsia felis was identified in 10 children, comprising eight with febrile conditions and two without fever (72). This signifies the importance of including controls for better understanding of the causative agent of fever in sub-Saharan Africa. Unlike Plasmodium spp., there has been a lack of investigation into the presence of rickettsial agents in human feces (95). In Ethiopia, 23 out of 102 (22.5%) children with fever were tested positive for one or more zoonotic-transmitted pathogens using real-time PCR. Rickettsia spp. were detected in three children and Borrelia spp. in two children, of which one child (0.9%) was tested positive for both Plasmodium spp. and Rickettsia spp. (96). Similarly, SFG Rickettsiae were identified in 11.9% of samples tested in Namibia, with male gender as the only significant risk factor (52). There may be a regional trend in exposure, with higher rates in northern regions and the lowest prevalence in the southern region of Hardap in Namibia, with male gender and the 20–29 year age group as the only significant risk factors.
Other studies found regional trends in exposure to spotted fever and typhus group Rickettsiae, with sub-acute neuropathy associated with African tick bite fever (ATBF) in Namibia (97). However, this study only represents a selected group of individuals and does not provide a comprehensive prevalence rate for the general population. Therefore, the study by Keller et al. (97) lacks sufficient data to establish the comprehensive prevalence of ATBF and the involvement of neuropathy. Importantly, detailed descriptions of clinical symptoms are lacking. Treatment primarily involved doxycycline for the majority of patients (77%), with other cases receiving thiamphenicol, fluoroquinolones, or macrolide antibiotics. The outcomes were favorable for 8.6% of patients, with the resolution of fever occurring, on average, after 2.92 days. Similarly, rickettsioses were most commonly diagnosed during the summer months of June, July, and August in Namibia (52). In the same study area, severe forms of the disease were observed in 14.7% of patients suffering with neurological manifestations and multi-visceral involvement. However, the study focused on clinical and epidemiological aspects and did not investigate the underlying mechanisms of the disease.
In southwestern Tanzania, the seroprevalence of SFG rickettsiosis was calculated to be 67.9% (Table 1; Figure 8) (98). This study also found that seropositivity was strongly associated with age, gender, higher temperatures during the day, and elevation, with a significant decline above 1,578 meters. Similarly, another study from Algeria revealed increased antibody titres against spotted fever rickettsial antigen in 77% of patients. Although human exposure to infected Ornithodoros ticks was observed, evidence of rickettsial DNA in blood samples from villagers was lacking (99). There is a potential link between SPG Rickettsiae and unexplained febrile illnesses despite malaria control in Sao Tome (100). The study provided serological evidence in humans for SFG Rickettsiea and C. burnetii, along with molecular evidence in ticks for SFG Rickettsiae in Sao Tome and Principe. In a similar study that focused on the frequency of febrile illnesses caused by vector-borne bacterial pathogens (52), 319 serum samples were collected, with the majority of individuals tested residing in urban settings (81.4%) and regularly interacting with animals (97.3%), including domestic and companion animals. Interaction with cattle, donkeys, and/or horses significantly increased the risk of exposure to C. burnetii (52).
Furthermore, in Gabon, zoonotic pathogens previously believed to exclusively infect humans have been found to originate in apes (55). In Djibouti, abattoir workers exhibited seropositivity for SFG Rickettsiae, typhus group Rickettsiae, and Orientia spp. This marks the first evidence of exposure to this Rickettsia spp. in the Horn of Africa (101). Orientia species are responsible for scrub typhus, which is closely related to the clinical symptoms of rickettsioses (102), but mainly found in Asia and Australia (57). Similarly, serological evidence of rickettsial infection was confirmed in 57.5%, and Q-fever diagnosed in 8.5% in Tunisia (103). Despite the significant annual cases of rickettsial infection recorded in Tunisia, the causative agent(s) have, regrettably, not been conclusively identified (104). However, a study employing quantitative real-time PCR and a Reverse Line Blot test did identify the presence of rickettsial DNA in skin biopsies and swabs, with Rickettsia conorii being the most prevalent bacterial species (104).
5 Challenges in distinguishing between different etiological agents of fever of unknown origin
Access to reliable diagnostic testing in most African countries is limited, and traditional diagnostic methods face challenges (11), prompting the establishment of a Point-of-Care (POC) laboratory (105). The POC laboratory was designed to address specific challenges in rural settings. Molecular-based POC testing utilizing real-time PCR was implemented for diagnosing various infectious diseases (105). Recently, mass spectrometry was established as an alternative method for species identification, but this diagnostic tool is highly cost-intensive and requires well-trained personnel (95). Distinguishing between different etiological agents of tick-borne febrile illnesses poses significant challenges due to overlapping clinical presentations and diagnostic limitations:
i. Non-specific Symptoms: Many tick-borne diseases share common clinical symptoms, such as fever, fatigue, myalgia, and arthralgia, making it difficult to clearly differentiate between each disease solely on clinical parameters. Common clinical manifestations among patients with positive PCR results for Rickettsiae included headaches (100%), chills (93.8%), muscle aches (68.8%), joint pains (68.8%), and rash (4.4%) (106).
ii. Limited access to diagnostics: In many resource-limited settings in Africa, access to advanced diagnostic tests is restricted, leading to reliance on clinical diagnosis or basic laboratory tests that may lack specificity. The disease was initially screened at the dispensary, followed by a second screening in Dakar conducted by highly trained personnel. Among the samples that tested positive, only 4 (15%) were identified as positive by thick smears at the dispensary, while 15 (56%) were confirmed positive during the second screening (107). In Senegal, MALDI-TOF MS was shown to be a valuable tool for tick species identification in the study of ticks, enabling species identification, detection of tick-associated microorganisms, and comparison of preservation methods (13). It provides researchers with important insights into tick biology and tick-borne diseases. There is the emergence of new approaches, like swabbing eschars for PCR testing (77). Rickettsial diagnosis is challenging in routine laboratories, and serology provides only a retrospective diagnosis (28). Thus, molecular methods, particularly qPCR, are proposed for routine diagnosis to overcome the obvious limitations of antibody testing (63). However, despite the advantage of the implementation of PCR-based methodologies in routine diagnosis, a cost-effective evaluation, and cost-intensive equipment, as well as well-trained technicians are required, which is often nearly impossible to realize in rural settings (63). Recently, Röttgerding et al. [12] developed two novel immunoassays (line immunoblot and ELISA) for IgM and IgG, employing complement-inhibiting protein (CihC) and glycerophosphodiester phosphodiesterase (GlpQ) of B. recurrentis as promising candidates for the diagnosis of louse-borne relapsing fever.
iii. Co-infections: Co-infections with multiple tick-borne pathogens can further complicate diagnosis and treatment decisions, as symptoms may be masked or exacerbated. Liu et al. (106) suggested the inclusion of rickettsial infections in the comprehensive diagnostic evaluation of febrile cases within region in western Kenya. Furthermore, there is a distinct recommendation for the establishment of diagnostic capabilities tailored specifically to rickettsial infections, particularly in locales characterized by a high prevalence of malaria.
iv. Cross-reactivity: Cross-reactivity refers to the ability of antibodies produced in response to the infection of a specific pathogen to react with similar antigens from other pathogens (108). In the context of tick-borne febrile illnesses, this phenomenon can complicate diagnostic efforts, thus leading to potential misidentifications and, consequently, misguided treatment strategies (109). The cross-reactivity issue is particularly pronounced in serological tests, where antibodies may not distinguish between different pathogens, causing false-positive or false-negative results (110). Several factors contribute to cross-reactivity in tick-borne febrile illnesses (111). Furthermore, the coexistence of multiple pathogens within the same geographic area, coupled with overlapping tick vectors, enhances the likelihood of cross-reactivity (112).
Cross-reactivity in tick-borne febrile illnesses has profound implications, affecting the accuracy of in vitro diagnostic and subsequent treatment decisions (Table 2) (113). The challenges posed by cross-reactivity highlight the pressing need for more specific and targeted diagnostic tools to differentiate closely related pathogens (76). False-positive results may lead to unnecessary treatments, while false negatives can result in delayed or inadequate interventions.
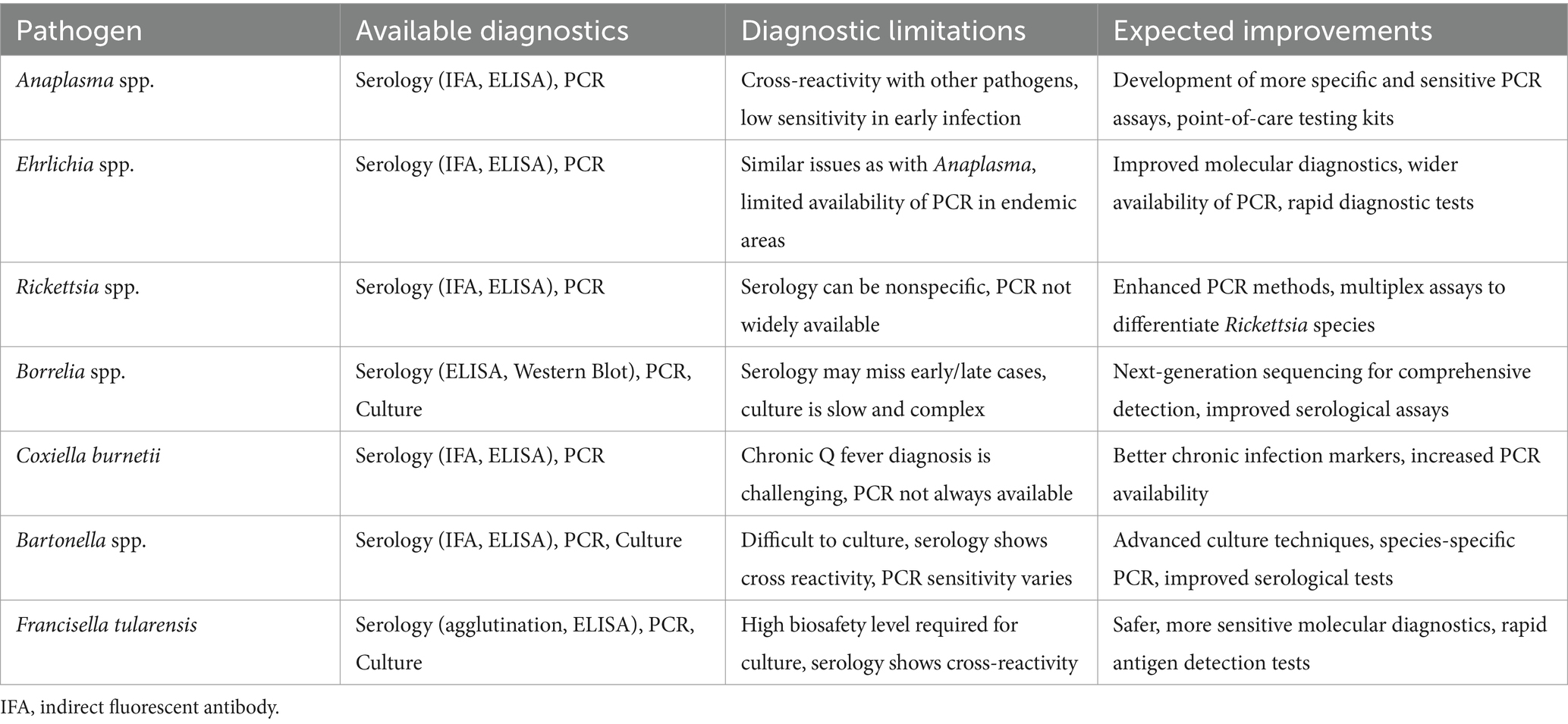
Table 2. Available diagnostics, diagnostic limitations, and expected improvements for bacterial tick-borne infections in Africa.
Currently, in vitro diagnostics employing immunological and molecular methods, such as ELISA, line blots, or various PCR-based assays, may encounter challenges in mitigating cross-reactivity. Although mass spectrometry and point-of-care testing show promise, obstacles persist in their widespread adoption, particularly in resource-limited settings where many tick-borne diseases are prevalent (77). In conclusion, to address the challenges known in the diagnosis of tick-borne diseases, research efforts should focus on developing more specific and reliable diagnostic tools. Targeting unique antigens associated with each pathogen, exploring the potential of next-generation sequencing technologies, and implementing advanced techniques like mass spectrometry offer new opportunities for improving accuracy in species identification and differentiation (77).
6 Conclusion
In conclusion, this review has delved into the complicated nature of tick-borne febrile illnesses in humans in Africa. Tick-borne febrile illnesses are undeniably significant health concerns in Africa, given the multiple human pathogens transmitted by ticks, including Anaplasma spp., Bartonella spp., Borrelia spp., Coxiella burnetii, Ehrlichia spp., Francisella tularensis, and Rickettsia africae (1, 60, 61, 63, 114). The epidemiological pattern of these diseases is linked with tick habitats, prevalent in rural and semi-urban areas where humans and livestock closely interact with tick habitats (8, 52). Despite the significant impact of tick-borne diseases on the well-being of economically disadvantaged farming communities in developing countries, diagnostic limitations pose substantial challenges (63). The overlapping clinical presentations, non-specific symptoms, and limited access to current diagnostic tests in resource-limited settings contribute to the complexity of achieving accurate diagnoses.
The exploration of aetiologies, through a thorough literature search from January 1990 to June 2024, has revealed the diversity of tick-borne pathogens affecting human populations in Africa. By acknowledging the diagnostic challenges and emphasizing the urgency for improvement, this review contributes to the broader understanding of tick-borne febrile illnesses, paving the way for advancements in diagnostics that are crucial for disease prevention and treatment in the African context.
The exploration of the unique features of hard ticks (Ixodidae) and soft ticks (Argasidae) further enriches the understanding of the complex life cycles and transmission dynamics of these vectors highlighting the importance of addressing transovarial transmission for effective disease control. In essence, this review serves as a valuable resource for health professionals, researchers, and policymakers urging collaborative efforts to enhance diagnostic capabilities, promote awareness, develop novel in vitro diagnostics (simple but specific POC tests for low-income countries, preferred created as a multiplex test), and strategies for mitigating the impact of tick-borne febrile illnesses on public health in Africa.
Author contributions
AA: Conceptualization, Writing – original draft, Writing – review & editing, Data curation, Investigation. FR: Data curation, Validation, Writing – review & editing, Visualization. NL: Data curation, Validation, Visualization, Writing – review & editing, Supervision, Investigation. AH: Data curation, Supervision, Validation, Writing – review & editing, Formal analysis. MI: Supervision, Validation, Writing – review & editing, Conceptualization, Project administration. MB: Conceptualization, Project administration, Supervision, Validation, Writing – review & editing, Data curation, Formal analysis. PK: Conceptualization, Data curation, Funding acquisition, Project administration, Supervision, Validation, Writing – review & editing.
Funding
The author(s) declare that financial support was received for the research, authorship, and/or publication of this article. This work was supported by the LOEWE Center DRUID (Novel Drug Targets against Poverty-Related and Neglected Tropical Infectious Diseases), LOEWE/1/10/519/03/03.001(0016)/53, project C3 (PK).
Acknowledgments
The authors gratefully acknowledge the contribution and support of research associates and scholars at the Centre for Advanced Medical Research and training, Usmanu Danfodiyo University Sokoto. This work forms part of the doctoral thesis of FR and AA.
Conflict of interest
The authors declare that the research was conducted in the absence of any commercial or financial relationships that could be construed as a potential conflict of interest.
The author(s) declared that they were an editorial board member of Frontiers, at the time of submission. This had no impact on the peer review process and the final decision.
Publisher’s note
All claims expressed in this article are solely those of the authors and do not necessarily represent those of their affiliated organizations, or those of the publisher, the editors and the reviewers. Any product that may be evaluated in this article, or claim that may be made by its manufacturer, is not guaranteed or endorsed by the publisher.
References
1. Vannier, E, and Krause, PJ. Human babesiosis. N Engl J Med. (2012) 366:2397–407. doi: 10.1056/NEJMra1202018
2. Oliver, JH. Biology and Systematics of Ticks (Acari:Ixodida). Ann Rev Eco System. (1989) 20:397–430. Available at: http://www.jstor.org/stable/2097098
3. Cutler, SJ. Relapsing fever – a forgotten disease revealed. J Appl Microbiol. (2010) 108:1115–22. doi: 10.1111/j.1365-2672.2009.04598.x
4. Davis, GE. Ornithodoros turicata:the males; feeding and copulation habits, fertility, span of life, and the transmission of relapsing fever spirochetes. Public Health Rep. (1941) 56:1799–802.
5. Jongejan, F, and Uilenberg, G. The global importance of ticks. Parasitology. (2004) 129:S3–14. doi: 10.1017/s0031182004005967
6. Diarra, AZ, Kelly, P, Davoust, B, and Parola, P. Tick-borne diseases of humans and animals in west Africa. Pathogens. (2023) 12:1276. doi: 10.3390/pathogens12111276
7. Parola, P, Paddock, CD, and Raoult, D. Tick-borne rickettsioses around the world: emerging diseases challenging old concepts. Clin Microbiol Rev. (2005) 18:719–56. doi: 10.1128/CMR.18.4.719-756.2005
8. Wu, X, Lu, Y, Zhou, S, Chen, L, and Xu, B. Impact of climate change on human infectious diseases: empirical evidence and human adaptation. Environ Int. (2016) 86:14–23. doi: 10.1016/j.envint.2015.09.007
9. Ehounoud, CB, Fenollar, F, Dahmani, M, N’Guessan, JD, Raoult, D, and Mediannikov, O. Bacterial arthropod-borne diseases in West Africa. Acta Trop. (2017) 171:124–37. doi: 10.1016/j.actatropica.2017.03.029
10. Petti, CA, Polage, CR, Quinn, TC, Ronald, AR, and Sande, MA. Laboratory medicine in Africa: a barrier to effective health care. Clin Infect Dis. (2006) 42:377–82. doi: 10.1086/499363
11. Cutler, SJ, Rudenko, N, Golovchenko, M, Cramaro, WJ, Kirpach, J, Savic, S, et al. Diagnosing Borreliosis. Vector Borne Zoonotic Dis. (2017) 17:2–11. doi: 10.1089/vbz.2016.1962
12. Röttgerding, F, Njeru, J, Schlüfter, E, Latz, A, Mahdavi, R, Steinhoff, U, et al. Novel approaches for the serodiagnosis of louse-borne relapsing fever. Front Cell Infect Microbiol. (2022) 12:983770. doi: 10.3389/fcimb.2022.983770
13. Hadji, E, Ndiaye, I, Diarra, AZ, Diouf, FS, Bouganali, C, Almeras, L, et al. Ornithodoros sonrai soft ticks and associated bacteria in Senegal. Pathogens. (2023) 12:1078. doi: 10.3390/pathogens12091078
14. Ramos, JM, Pérez-Tanoira, R, Martín-Martín, I, Prieto-Pérez, L, Tefasmariam, A, Tiziano, G, et al. Arthropod-borne bacteria cause Nonmalarial fever in rural Ethiopia: a cross-sectional study in 394 patients. Vector Borne Zoonotic Dis. (2019) 19:815–20. doi: 10.1089/vbz.2018.2396
15. Hepburn, MJ, and Simpson, AJH. Tularemia: current diagnosis and treatment options. Expert Rev Anti-Infect Ther. (2008) 6:231–40. doi: 10.1586/14787210.6.2.231
16. Talagrand-Reboul, E, Boyer, PH, Bergström, S, Vial, L, and Boulanger, N. Relapsing fevers: neglected tick-borne diseases. Front Cell Infect Microbiol. (2018) 8:98. doi: 10.3389/fcimb.2018.00098
17. Hauck, D, Jordan, D, Springer, A, Schunack, B, Pachnicke, S, Fingerle, V, et al. Transovarial transmission of Borrelia spp., Rickettsia spp. and Anaplasma phagocytophilum in Ixodes ricinus under field conditions extrapolated from DNA detection in questing larvae. Parasit Vectors. (2020) 13:176. doi: 10.1186/s13071-020-04049-7
18. Dumler, JS, Choi, KS, Garcia-Garcia, JC, Barat, NS, Scorpio, DG, Garyu, JW, et al. Human granulocytic anaplasmosis and Anaplasma phagocytophilum. Emerg Infect Dis. (2005) 11:1828–34. doi: 10.3201/eid1112.050898
19. Annen, K, Friedman, K, Eshoa, C, Horowitz, M, Gottschall, J, and Straus, T. Two cases of transfusion-transmitted Anaplasma phagocytophilum. Am J Clin Pathol. (2012) 137:562–5. doi: 10.1309/AJCP4E4VQQQOZIAQ
20. Zhang, L, Liu, H, Xu, B, Zhang, Z, Jin, Y, Li, W, et al. Rural residents in China are at increased risk of exposure to tick-borne pathogens Anaplasma phagocytophilum and Ehrlichia chaffeensis. Biomed Res Int. (2014) 2014:313867. doi: 10.1155/2014/645056
21. Stuen, S, Granquist, EG, and Silaghi, C. Anaplasma phagocytophilum--a widespread multi-host pathogen with highly adaptive strategies. Front Cell Infect Microbiol. (2013) 3:31. doi: 10.3389/fcimb.2013.00031
22. Woldehiwet, Z. The natural history of Anaplasma phagocytophilum. Vet Parasitol. (2010) 167:108–22. doi: 10.1016/j.vetpar.2009.09.013
23. Kolo, A. Anaplasma species in Africa-a century of discovery: a review on molecular epidemiology, genetic diversity, and control. Pathogens. (2023) 12:702. doi: 10.3390/pathogens12050702
24. Fourie, JJ, Evans, A, Labuschagne, M, Crafford, D, Madder, M, Pollmeier, M, et al. Transmission of Anaplasma phagocytophilum (Foggie, 1949) by Ixodes ricinus (Linnaeus, 1758) ticks feeding on dogs and artificial membranes. Parasit Vectors. (2019) 12:136. doi: 10.1186/s13071-019-3396-9
25. Brown, WC. Adaptive immunity to Anaplasma pathogens and immune dysregulation: implications for bacterial persistence. Comp Immunol Microbiol Infect Dis. (2012) 35:241–52. doi: 10.1016/j.cimid.2011.12.002
26. Ehounoud, CB, Yao, KP, Dahmani, M, Achi, YL, Amanzougaghene, N, Kacou N’Douba, A, et al. Multiple pathogens including potential new species in tick vectors in Côte d’Ivoire. PLoS Negl Trop Dis. (2016) 10:e0004367. doi: 10.1371/journal.pntd.0004367
27. Ghafar, MW, and Eltablawy, NA. Molecular survey of five tick-borne pathogens (Ehrlichia chaffeensis, sensu lato and Babesia microti) in Egyptian Farmers. Glob Veterinaria. (2011) 7:249–55.
28. Elhamiani Khatat, S, Sahibi, H, Hing, M, Alaoui Moustain, I, El Amri, H, Benajiba, M, et al. Human exposure to Anaplasma phagocytophilum in two cities of northwestern Morocco. PLoS One. (2016) 11:e0160880. doi: 10.1371/journal.pone.0160880
29. Louni, M, Mana, N, Bitam, I, Dahmani, M, Parola, P, Fenollar, F, et al. Body lice of homeless people reveal the presence of several emerging bacterial pathogens in northern Algeria. PLoS Negl Trop Dis. (2018) 12:e0006397. doi: 10.1371/journal.pntd.0006397
30. Amanzougaghene, N, Fenollar, F, Sangaré, AK, Sissoko, MS, Doumbo, OK, Raoult, D, et al. Detection of bacterial pathogens including potential new species in human head lice from Mali. PLoS One. (2017) 12:e0184621. doi: 10.1371/journal.pone.0184621
31. Ben Said, M, Belkahia, H, and Messadi, L. Anaplasma spp. in North Africa: a review on molecular epidemiology, associated risk factors and genetic characteristics. Ticks Tick-Borne Dis. (2018) 9:543–55. doi: 10.1016/j.ttbdis.2018.01.003
32. Dumler, JS, Barbet, AF, Bekker, CP, Dasch, GA, Palmer, GH, Ray, SC, et al. Reorganization of genera in the families Rickettsiaceae and Anaplasmataceae in the order Rickettsiales: unification of some species of Ehrlichia with Anaplasma, Cowdria with Ehrlichia and Ehrlichia with Neorickettsia, descriptions of six new species comb. Int J Syst Evol Microbiol. (2001) 51:2145–65. doi: 10.1099/00207713-51-6-2145
33. Ismail, N, Bloch, KC, and McBride, JW. Human ehrlichiosis and anaplasmosis. Clin Lab Med. (2010) 30:261–92. doi: 10.1016/j.cll.2009.10.004
34. da Vieira, RFC, Biondo, AW, AMS, G, Dos Santos, AP, Dos Santos, RP, Dutra, LH, et al. Ehrlichiosis in Brazil. Rev Brasileira Parasitologia Veterinaria. (2011) 20:01–12. doi: 10.1590/S1984-29612011000100002
35. Ndip, LM, Labruna, M, Ndip, RN, Walker, DH, and McBride, JW. Molecular and clinical evidence of Ehrlichia chaffeensis infection in Cameroonian patients with undifferentiated febrile illness. Ann Trop Med Parasitol. (2009) 103:719–25. doi: 10.1179/000349809X12554106963753
36. Muraro, LS, de Souza, AO, TNS, L, Cândido, SL, ALT, M, Toma, HS, et al. First evidence of Ehrlichia minasensis infection in horses from Brazil. Pathogens. (2021) 10:265. doi: 10.3390/pathogens10030265
37. Ndip, LM, Ndip, RN, Esemu, SN, Walker, DH, and McBride, JW. Predominance of Ehrlichia chaffeensis in Rhipicephalus sanguineus ticks from kennel-confined dogs in Limbe, Cameroon. Exp Appl Acarol. (2010) 50:163–8. doi: 10.1007/s10493-009-9293-8
38. Paddock, CD, and Childs, JE. Ehrlichia chaffeensis: a prototypical emerging pathogen. Clin Microbiol Rev. (2003) 16:37–64. doi: 10.1128/CMR.16.1.37-64.2003
39. Beall, MJ, Alleman, AR, Breitschwerdt, EB, Cohn, LA, Couto, CG, Dryden, MW, et al. Seroprevalence of Ehrlichia canis, Ehrlichia chaffeensis and Ehrlichia ewingii in dogs in North America. Parasit Vectors. (2012) 5:29. doi: 10.1186/1756-3305-5-29
40. McLendon, MK, Apicella, MA, and Allen, LAH. Francisella tularensis: taxonomy, genetics, and Immunopathogenesis of a potential agent of biowarfare. Ann Rev Microbiol. (2006) 60:167–85. doi: 10.1146/annurev.micro.60.080805.142126
41. Stidham, RA, Freeman, DB, von Tersch, RL, Sullivan, PJ, and Tostenson, SD. Epidemiological review of francisella tularensis: a case study in the complications of dual diagnoses. PLoS Currents. (2018) 10. doi: 10.1371/ecurrents.outbreaks.8eb0b55f377abc2d250314bbb8fc9d6d
42. Petersen, JM, Mead, PS, and Schriefer, ME. Francisella tularensis: an arthropod-borne pathogen. Vet Res. (2009) 40:7. doi: 10.1051/vetres:2008045
43. Ellis, J, Oyston, PCF, Green, M, and Titball, RW. Tularemia. Clin Microbiol Rev. (2002) 15:631–46. doi: 10.1128/CMR.15.4.631-646.2002
44. Abdellahoum, Z, Maurin, M, and Bitam, I. Tularemia as a mosquito-borne disease. Microorganisms. (2020) 9:26. doi: 10.3390/microorganisms9010026
45. Akimana, C, and Kwaik, YA. Francisella-arthropod vector interaction and its role in patho-adaptation to infect mammals. Front Microbiol. (2011) 2:34. doi: 10.3389/fmicb.2011.00034
46. Troha, K, Božanić Urbančič, N, Korva, M, Avšič-Županc, T, Battelino, S, and Vozel, D. Vector-borne tularemia: a re-emerging cause of cervical lymphadenopathy. Trop Med Infect Dis. (2022) 7:189. doi: 10.3390/tropicalmed7080189
47. Maurin, M. Francisella tularensis, Tularemia and serological diagnosis. Front Cell Infect Microbiol. (2020) 10:512090. doi: 10.3389/fcimb.2020.512090
48. Diddi, K, Chaudhry, R, Sharma, N, and Dhawan, B. Strategy for identification & characterization of Bartonella henselae with conventional & molecular methods. Indian J Med Res. (2013) 137:380–7.
49. Cheslock, MA, and Embers, ME. Human Bartonellosis: an Underappreciated Public Health Problem? Trop Med Infect Dis. (2019) 4:69. doi: 10.3390/tropicalmed4020069
50. Pecoraro, A, Herbst, P, Pienaar, C, Taljaard, J, Prozesky, H, Janson, J, et al. Bartonella species as a cause of culture-negative endocarditis in South Africa. Eur J Clin Microbiol Infect Dis. (2021) 40:1873–9. doi: 10.1007/s10096-021-04239-w
51. Tasher, D, Raucher-Sternfeld, A, Tamir, A, Giladi, M, and Somekh, E. Bartonella quintana, an unrecognized cause of infective endocarditis in children in Ethiopia. Emerg Infect Dis. (2017) 23:1246–52. doi: 10.3201/eid2308.161037
52. Noden, BH, Tshavuka, FI, Van Der, CBE, Chipare, I, and Wilkinson, R. Exposure and risk factors to Coxiella burnetii, spotted fever group and typhus group rickettsiae, and Bartonella henselae among volunteer blood donors in Namibia. PLoS One. (2014) 9:3–10. doi: 10.1371/journal.pone.0108674
53. Pantchev, N, Pluta, S, Huisinga, E, Nather, S, Scheufelen, M, Vrhovec, MG, et al. Tick-borne diseases (Borreliosis, Anaplasmosis, Babesiosis) in German and Austrian dogs: status quo and review of distribution, transmission, clinical findings, diagnostics and prophylaxis. Parasitol Res. (2015) 114:19–54. doi: 10.1007/s00436-015-4513-0
54. Esemu, SN, Ndip, LM, and Ndip, RN. Ehrlichia species, probable emerging human pathogens in sub-Saharan Africa: environmental exacerbation. Rev Environ Health. (2011) 26:269–79. doi: 10.1515/reveh.2011.034
55. Gutiérrez, R, Krasnov, B, Morick, D, Gottlieb, Y, Khokhlova, IS, and Harrus, S. Bartonella infection in rodents and their flea ectoparasites: an overview. Vector Borne Zoonotic Dis. (2015) 15:27–39. doi: 10.1089/vbz.2014.1606
56. Ereqat, S, Nasereddin, A, Vayssier-Taussat, M, Abdelkader, A, Al-Jawabreh, A, Zaid, T, et al. Molecular evidence of Bartonella species in Ixodid ticks and domestic animals in Palestine. Front Microbiol. (2016) 7:1217. doi: 10.3389/fmicb.2016.01217
57. Harms, A, and Dehio, C. Intruders below the radar: molecular pathogenesis of Bartonella spp. Clin Microbiol Rev. (2012) 25:42–78. doi: 10.1128/CMR.05009-11
58. Tsai, YL, Chang, CC, Chuang, ST, and Chomel, BB. Bartonella species and their ectoparasites: selective host adaptation or strain selection between the vector and the mammalian host? Comp Immunol Microbiol Infect Dis. (2011) 34:299–314. doi: 10.1016/j.cimid.2011.04.005
59. Mosbacher, ME, Klotz, S, Klotz, J, and Pinnas, JL. Bartonella henselae and the potential for arthropod vector-borne transmission. Vector Borne Zoonotic Dis. (2011) 11:471–7. doi: 10.1089/vbz.2010.0106
60. Binetruy, F, Garnier, S, Boulanger, N, Talagrand-Reboul, É, Loire, E, Faivre, B, et al. A novel Borrelia species, intermediate between Lyme disease and relapsing fever groups, in neotropical passerine-associated ticks. Sci Rep. (2020) 10:10596. doi: 10.1038/s41598-020-66828-7
61. Fingerle, V, Pritsch, M, Wächtler, M, Margos, G, Ruske, S, Jung, J, et al. Candidatus “Borrelia kalaharica” detected from a febrile traveller returning to Germany from vacation in Southern Africa. PLoS Negl Trop Dis. (2016) 10:e0004559. doi: 10.1371/journal.pntd.0004559
62. Raoult, D, and Roux, V. The body louse as a vector of reemerging human diseases. Clin Infect Dis. (1999) 29:888–911. doi: 10.1086/520454
63. Eweda, A, Kwak, ML, Nonaka, N, and Nakao, R. Human-biting ticks and zoonotic tick-borne pathogens in north Africa: diversity, distribution, and trans-mediterranean public health challenges. One Health. (2023) 16:100547. doi: 10.1016/j.onehlt.2023.100547
64. Warrell, DA. Louse-borne relapsing fever (Borrelia recurrentis infection). Epidemiol Infect. (2019) 147:e106. doi: 10.1017/S0950268819000116
65. Mediannikov, O, Socolovschi, C, Bassene, H, Diatta, G, Ratmanov, P, Fenollar, F, et al. Borrelia crocidurae infection in acutely febrile patients, Senegal. Emerg Infect Dis. (2014) 20:1335–8. doi: 10.3201/eid2008.130550
66. El Hadji, N, Ibrahima, GD, Zan, DA, Bassene, H, and Cheikh Sokhna, PP. Quantitative polymerase chain reaction from malaria rapid diagnostic tests to detect Borrelia crocidurae, the agent of tick-borne relapsing fever, in febrile patients in Senegal. Am J Trop Med Hyg. (2023) 108:968–76. doi: 10.4269/ajtmh.22-0342
67. Grecchi, C, Zanotti, P, Pontarelli, A, Chiari, E, Rachele, L, Maurizio, T, et al. Louse - borne relapsing fever in a refugee from Mali. Infection. (2017) 45:373–6. doi: 10.1007/s15010-017-0987-2
68. Lucchini, A, Lipani, F, Costa, C, Scarvaglieri, M, Balbiano, R, et al. Louseborne relapsing fever among east African Refugees, Italy. Travel Med Infect Dis. (2016) 14:110–4. doi: 10.1016/j.tmaid.2016.01.004
69. Points, KEY. Relapsing fever in a traveller returning from Senegal. CMAJ. (2021) 193:E285–8. doi: 10.1503/cmaj.201644
70. Vial, L, Diatta, G, Tall, A, Ba, EH, Bouganali, H, Durand, P, et al. Incidence of tick-borne relapsing fever in west Africa: longitudinal study. Lancet. (2006) 368:37–43. doi: 10.1016/S0140-6736(06)68968-X
71. Lafri, I, El Hamzaoui, B, Bitam, I, Leulmi, H, Lalout, R, Mediannikov, O, et al. Detection of relapsing fever Borrelia spp., Bartonella spp. and Anaplasmataceae bacteria in argasid ticks in Algeria. PLoS Negl Trop Dis. (2017) 11:1–13. doi: 10.1371/journal.pntd.0006064
72. Fenollar, F, Socolovschi, C, Lemamy, GJ, Nzoughe, H, Kouna, LC, Toure-ndouo, F, et al. Molecular detection of fastidious and common bacteria as well as Plasmodium spp. in Febrile and Afebrile Children in Franceville, Gabon. Am J Trop Med Hyg. (2015) 92:926–32. doi: 10.4269/ajtmh.14-0699
73. Qiu, Y, Nakao, R, Mudenda, B, Sato, K, Kajihara, M, Kanchela, S, et al. Human borreliosis caused by a new world relapsing fever Borrelia – like organism in the old world. Clin Infect Dis. (2019) 69:107–12. doi: 10.1093/cid/ciy850
74. Dragan, AL, and Voth, DE. Coxiella burnetii: international pathogen of mystery. Microbes Infect. (2020) 22:100–10. doi: 10.1016/j.micinf.2019.09.001
75. Hartzell, JD, Wood-Morris, RN, Martinez, LJ, and Trotta, RF. Q fever: epidemiology, diagnosis, and treatment. Mayo Clin Proc. (2008) 83:574–9. doi: 10.1016/S0025-6196(11)60733-7
76. Salam, MA, Al-Amin, MY, Pawar, JS, Akhter, N, and Lucy, IB. Conventional methods and future trends in antimicrobial susceptibility testing. Saudi J Biol Sci. (2023) 30:103582. doi: 10.1016/j.sjbs.2023.103582
77. Mediannikov, O, Socolovschi, C, Million, M, Sokhna, C, Bassene, H, Diatta, G, et al. Molecular identification of pathogenic bacteria in eschars from acute febrile patients, Senegal. Am J Trop Med Hyg. (2014) 91:1015–9. doi: 10.4269/ajtmh.13-0629
78. Tigertt, WD, Benenson, AS, and Gochenour, WS. Airborne Q fever. Bacteriol Rev. (1961) 25:285–93. doi: 10.1128/br.25.3.285-293.1961
79. Bok, J, Hogerwerf, L, Germeraad, EA, Roest, HIJ, Faye-Joof, T, Jeng, M, et al. Coxiella burnetii (Q fever) prevalence in associated populations of humans and small ruminants in the Gambia. Trop Med Int Health. (2017) 22:323–31. doi: 10.1111/tmi.12827
80. Enkhtsetseg, A, Davadoorj, R, Fernandez, S, Mongkolsirichaikul, D, Altantuul, D, Elbegdorj, E, et al. Seroconversion to causes of febrile illness in Mongolian peacekeepers deployed to South Sudan. Am J Trop Med Hyg. (2016) 95:1469–71. doi: 10.4269/ajtmh.16-0174
81. Saito, TB, Bechelli, J, Smalley, C, Karim, S, and Walker, DH. Vector tick transmission model of spotted fever rickettsiosis. Am J Pathol. (2019) 189:115–23. doi: 10.1016/j.ajpath.2018.09.005
82. Tomassone, L, Portillo, A, Nováková, M, de Sousa, R, and Oteo, JA. Neglected aspects of tick-borne rickettsioses. Parasit Vectors. (2018) 11:263. doi: 10.1186/s13071-018-2856-y
83. Robinson, MT, Satjanadumrong, J, Hughes, T, Stenos, J, and Blacksell, SD. Diagnosis of spotted fever group Rickettsia infections: the Asian perspective. Epidemiol Infect. (2019) 147:e286. doi: 10.1017/S0950268819001390
84. Eremeeva, ME, and Dasch, GA. Challenges posed by tick-borne rickettsiae: eco-epidemiology and public health implications. Front Public Health. (2015) 3:55. doi: 10.3389/fpubh.2015.00055
85. Montenegro, DC, Bitencourth, K, de Oliveira, SV, Borsoi, AP, Cardoso, KM, Sousa, MSB, et al. Spotted fever: epidemiology and vector-Rickettsia-host relationship in Rio de Janeiro state. Front Microbiol. (2017) 8:505. doi: 10.3389/fmicb.2017.00505
86. Fenollar, F, and Mediannikov, O. Emerging infectious diseases in Africa in the 21st century. New Microb New Infect. (2018) 26:S10–8. doi: 10.1016/j.nmni.2018.09.004
87. Mediannikov, O, Diatta, G, Fenollar, F, Sokhna, C, Trape, JF, and Raoult, D. Tick-borne rickettsioses, neglected emerging diseases in rural Senegal. PLoS Negl Trop Dis. (2010) 4:821. doi: 10.1371/journal.pntd.0000821
88. Ali, MA, James, OC, Mohamed, AA, Joachim, A, Mubi, M, and Omodior, O. Etiologic agents of fever of unknown origin among patients attending Mnazi Mmoja Hospital, Zanzibar. J Community Health. (2020) 45:1073–80. doi: 10.1007/s10900-020-00832-w
89. Ndip, LM, Biswas, HH, Nfonsam, LE, LeBreton, M, Ndip, RN, Bissong, MA, et al. Risk factors for African tick-bite fever in rural Central Africa. Am J Trop Med Hyg. (2011) 84:608–13. doi: 10.4269/ajtmh.2011.10-0191
90. Kigozi, BK, Kharod, GA, Bukenya, H, Shadomy, SV, Haberling, DL, Stoddard, RA, et al. Investigating the etiology of acute febrile illness: a prospective clinic - based study in Uganda. BMC Infect Dis. (2023) 23:411. doi: 10.1186/s12879-023-08335-4
91. Mediannikov, O, Diatta, G, Zolia, Y, Balde, MC, Kohar, H, Trape, JF, et al. Tick-borne rickettsiae in Guinea and Liberia. Ticks Tick Borne Dis. (2012) 3:43–8. doi: 10.1016/j.ttbdis.2011.08.002
92. Socolovschi, C, Renvoisé, A, Brouqui, P, Parola, P, and Raoult, D. Ticks and tick-borne diseases the use of Eschar swabs for the diagnosis of African tick-bite fever. Ticks Tick Borne Dis. (2012) 3:355–60. doi: 10.1016/j.ttbdis.2012.10.019
93. Berrian, AM, Martínez-López, B, Quan, V, Conrad, PA, van Rooyen, J, Simpson, GJG, et al. Risk factors for bacterial zoonotic pathogens in acutely febrile patients in Mpumalanga Province, South Africa. Zoonoses Public Health. (2019) 66:458–69. doi: 10.1111/zph.12577
94. Simpson, GJG, Quan, V, Frean, J, Knobel, DL, Rossouw, J, Weyer, J, et al. Prevalence of selected zoonotic diseases and risk factors at a human-wildlife-livestock interface in Mpumalanga Province, South Africa. Vector Borne Zoonotic Dis. (2018) 18:303–10. doi: 10.1089/vbz.2017.2158
95. Keita, AK, Socolovschi, C, Ahuka-mundeke, S, Ratmanov, P, Butel, C, Ayouba, A, et al. Molecular evidence for the presence of rickettsia felis in the feces of wild-living African Apes. PLoS One. (2013) 8:e54679. doi: 10.1371/journal.pone.0054679
96. Aarsland, SJ, Castellanos-gonzalez, A, Lockamy, KP, Mulu-droppers, R, Mulu, M, White, AC, et al. Treatable bacterial infections are underrecognized causes of fever in ethiopian children. Am J Trop Med Hyg. (2012) 87:128–33. doi: 10.4269/ajtmh.2012.12-0171
97. Keller, C, Krüger, A, Schwarz, NG, Rakotozandrindrainy, R, Rakotondrainiarivelo, JP, Razafindrabe, T, et al. High detection rate of Rickettsia africae in Amblyomma variegatum but low prevalence of anti-rickettsial antibodies in healthy pregnant women in Madagascar. Ticks Tick-Borne Dise. (2016) 7:60–5. doi: 10.1016/j.ttbdis.2015.08.005
98. Heinrich, N, Dill, T, Dobler, G, Clowes, P, and Kroidl, I. High seroprevalence for spotted fever group Rickettsiae, is associated with higher temperatures and rural environment in Mbeya Region, Southwestern Tanzania. PLoS Negl Trop Dis. (2015) 9:e0003626. doi: 10.1371/journal.pntd.0003626
99. Mouffok, N, Parola, P, Lepidi, H, and Raoult, D. Mediterranean spotted fever in Algeria - new trends. Int J Infect Dis. (2009) 13:227–35. doi: 10.1016/j.ijid.2008.06.035
100. En, T, Sui, H, Hsiao, W, Minahan, NT, Ying, T, Vicente, A, et al. Seroepidemiological and molecular investigation of spotted fever group rickettsiae and Coxiella burnetii in Sao Tome Island: a one health approach. Transbound Emerg Dis. (2019) 67:36–43. doi: 10.1111/tbed.13191
101. Horton, KC, Jiang, J, Maina, A, Dueger, E, Zayed, A, Ahmed, AA, et al. Evidence of rickettsia and orientia infections among abattoir workers in Djibouti. Am J Trop Med Hyg. (2016) 95:462–5. doi: 10.4269/ajtmh.15-0775
102. Tamura, A, Ohashi, N, Urakami, H, and Miyamura, S. Classification of Rickettsia tsutsugamushi in a new genus, Orientia gen. nov., as Orientia tsutsugamushi comb. nov. Int J Syst Bacteriol. (1995) 45:589–91. doi: 10.1099/00207713-45-3-589
103. Kaabia, N, Rolain, JM, Khalifa, M, Ben Jazia, E, Bahri, F, Raoult, D, et al. Serologic study of rickettsioses among acute febrile patients in Central Tunisia. Ann N Y Acad Sci. (2006) 1078:176–9.
104. Khrouf, F, Sellami, H, Elleuch, E, Hattab, Z, Ammari, L, Khalfaoui, M, et al. Molecular diagnosis of Rickettsia infection in patients from Tunisia. Ticks Tick Borne Dis. (2016) 7:653–6. doi: 10.1016/j.ttbdis.2016.02.010
105. Sokhna, C, Mediannikov, O, Fenollar, F, Bassene, H, and Diatta, G. Point-of-care laboratory of pathogen diagnosis in rural Senegal. PLoS Negl Trop Dis. (2013) 7:e1999. doi: 10.1371/journal.pntd.0001999
106. Liu, Q, Jin, X, Cheng, J, Zhou, H, Zhang, Y, and Dai, Y. Advances in the application of molecular diagnostic techniques for the detection of infectious disease pathogens. Mol Med Rep. (2023) 27:104. doi: 10.3892/mmr.2023.12991
107. Parola, P, Diatta, G, Socolovschi, C, Mediannikov, O, Tall, A, Bassene, H, et al. Tick-borne relapsing fever Borreliosis, rural Senegal. Emerg Infect Dis. (2011) 17:883–5. doi: 10.3201/eid1705.100573
108. Fairlie-Clarke, KJ, Shuker, DM, and Graham, AL. Why do adaptive immune responses cross-react? Evol Appl. (2009) 2:122–31. doi: 10.1111/j.1752-4571.2008.00052.x
109. Grąźlewska, W, and Holec-Gąsior, L. Antibody cross-reactivity in serodiagnosis of lyme disease. Antibodies (Basel, Switzerland). (2023) 12:63. doi: 10.3390/antib12040063
110. Parola, P, and Raoult, D. Ticks and tickborne bacterial diseases in humans: an emerging infectious threat. Clin Infet Dis. (2001) 32:897–928. doi: 10.1086/319347
111. Guérin, M, Shawky, M, Zedan, A, Octave, S, Avalle, B, Maffucci, I, et al. Lyme borreliosis diagnosis: state of the art of improvements and innovations. BMC Microbiol. (2023) 23:204. doi: 10.1186/s12866-023-02935-5
112. Garcia, K, Weakley, M, Do, T, and Mir, S. Current and future molecular diagnostics of tick-borne diseases in cattle. Vet Sci. (2022) 9:241. doi: 10.3390/vetsci9050241
113. Burt, T, Button, KS, Thom, H, Noveck, RJ, and Munafò, MR. The burden of the ‘false-negatives’ in clinical development: analyses of current and alternative scenarios and corrective measures. Clin Transl Sci. (2017) 10:470–9. doi: 10.1111/cts.12478
114. Maina, AN, Knobel, DL, Jiang, J, Halliday, J, Feikin, DR, Cleaveland, S, et al. Rickettsia felis infection in febrile patients, western Kenya, 2007-2010. Emerg Infect Dis. (2012) 18:328–31. doi: 10.3201/eid1802.111372
115. Elhamiani Khatat, S, Daminet, S, Kachani, M, Leutenegger, CM, Duchateau, L, El Amri, H, et al. Anaplasma spp. in dogs and owners in North-Western Morocco. Parasit Vectors. (2017) 10:202. doi: 10.1186/s13071-017-2148-y
116. Kolo, AO, Collins, NE, Brayton, KA, Chaisi, M, Blumberg, L, Frean, J, et al. Anaplasma phagocytophilum and other Anaplasma spp. in various hosts in the mnisi community, Mpumalanga Province, South Africa. Microorganisms. (2020) 8:1812. doi: 10.3390/microorganisms8111812
117. Kisinza, WN, McCall, PJ, Mitani, H, Talbert, A, and Fukunaga, M. A newly identified tick-borne Borrelia species and relapsing fever in Tanzania. Lancet. (2003) 362:1283–4. doi: 10.1016/S0140-6736(03)14609-0
118. Trape, JF, Duplantier, JM, Bouganali, H, Godeluck, B, Legros, F, Cornet, JP, et al. Tick-borne Borreliosis in West Africa. Lancet (London, England). (1991) 337:473–5.
119. Ndiaye, EHI, Diouf, FS, Ndiaye, M, Bassene, H, Raoult, D, Sokhna, C, et al. Tick-borne relapsing fever Borreliosis, a major public health problem overlooked in Senegal. PLoS Negl Trop Dis. (2021) 15:e0009184. doi: 10.1371/journal.pntd.0009184
120. Levine, ZC, Sene, A, Mkandawire, W, Deme, AB, Ndiaye, T, Sy, M, et al. Investigating the etiologies of non-malarial febrile illness in Senegal using metagenomic sequencing. Nat Commun. (2024) 15:747. doi: 10.1038/s41467-024-44800-7
121. Yimer, M, Abera, B, Mulu, W, Bezabih, B, and Mohammed, J. Prevalence and risk factors of louse-borne relapsing fever in high risk populations in Bahir Dar city northwest, Ethiopia. BMC Res Notes. (2014) 7:615. doi: 10.1186/1756-0500-7-615
122. Abera, EG, Tukeni, KN, Didu, GH, Chala, TK, Yilma, D, and Gudina, EK. Epistaxis and thrombocytopenia as major presentations of louse borne relapsing fever: hospital-based study. PLoS One. (2022) 17:e0279721. doi: 10.1371/journal.pone.0279721
123. Prabhu, M, Nicholson, WL, Roche, AJ, Kersh, GJ, Fitzpatrick, KA, Oliver, LD, et al. Q fever, spotted fever group, and typhus group rickettsioses among hospitalized febrile patients in northern Tanzania. Clin Infect Dis. (2011) 53:e8–e15. doi: 10.1093/cid/cir411
124. van der Hoek, W, Sarge-Njie, R, Herremans, T, Chisnall, T, Okebe, J, Oriero, E, et al. Short communication: prevalence of antibodies against Coxiella burnetii (Q fever) in children in the Gambia, West Africa. Trop Med Int Health. (2013) 18:850–3. doi: 10.1111/tmi.12116
125. Okabayashi, T, Hasebe, F, Samui, KL, Mweene, AS, Pandey, SG, Yanase, T, et al. Short report: prevalence of antibodies against spotted fever, murine typhus, and Q fever rickettsiae in humans living in Zambia. Am J Trop Med Hyg. (1999) 61:70–2. doi: 10.4269/ajtmh.1999.61.70
126. Wardrop, NA, Thomas, LF, Cook, EAJ, de Glanville, WA, Atkinson, PM, Wamae, CN, et al. The sero-epidemiology of Coxiella burnetii in humans and cattle, Western Kenya: evidence from a cross-sectional study. PLoS Negl Trop Dis. (2016) 10:e0005032. doi: 10.1371/journal.pntd.0005032
127. Steinmann, P, Bonfoh, B, Péter, O, Schelling, E, Traoré, M, and Zinsstag, J. Seroprevalence of Q-fever in febrile individuals in Mali. Trop Med Infect Health. (2005) 10:612–7. doi: 10.1111/j.1365-3156.2005.01420.x
128. Julvez, J, Michault, A, and Kerdelhue, C. Serological study of rickettsia infections in Niamey, Niger. Med Trop. (1997) 57:153–6.
129. Letaief, AO, Yacoub, S, Dupont, HT, Le Cam, C, Ghachem, L, Jemni, L, et al. Seroepidemiological survey of rickettsial infections among blood donors in Central Tunisia. Trans R Soc Trop Med Hyg. (1995) 89:266–8. doi: 10.1016/0035-9203(95)90531-6
130. Njeru, J, Tomaso, H, Mertens, K, Henning, K, Wareth, G, Heller, R, et al. Serological evidence of Francisella tularensis in febrile patients seeking treatment at remote hospitals, northeastern Kenya, 2014–2015. New Microb New Infect. (2017) 19:62–6. doi: 10.1016/j.nmni.2017.05.015
131. Koyo, CSB, Oyegue-Liabagui, SL, Mediannikov, O, Cortaredona, S, Kouna, LC, Raoult, D, et al. High circulation of malaria and low prevalence of bacteremia in febrile and afebrile children in northeastern Gabon. Am J Trop Med Hyg. (2020) 102:121–9. doi: 10.4269/ajtmh.19-0368
132. Kabinet, A, Florence, K, and Cristina, F. The detection of vector-borne-disease-related DNA in human stool paves the way to large epidemiological studies. Eur J Epidemiol. (2015) 30:1021–6. doi: 10.1007/s10654-015-0022-9
133. Bouchaib, H, Eldin, C, Laroche, M, Raoult, D, and Parola, P. Tick-and fl ea-borne rickettsioses in Tizi-Ouzou, Algeria: Implications for travel medicine. Travel Med Infect Dis. (2018) 26:51–7. doi: 10.1016/j.tmaid.2018.11.005
134. Znazen, A, Sellami, H, Elleuch, E, Hattab, Z, and Ben, SL. Comparison of two quantitative real time pcr assays for rickettsia detection in patients from Tunisia. PLoS Negl Trop Dis. (2015) 9:e0003487. doi: 10.1371/journal.pntd.0003487
135. Jensenius, M, Edouard, FP, Fladby, T, Hellum, KB, and Hagen, TØ TPRI, et al. Sub-acute neuropathy in patients with African tick bite fever. Scand J Infect Dis. (2006) 38:114–8. doi: 10.1080/00365540500321579
Appendix 1
List of articles included in the review.
Anaplasma phagocytophilum: (27, 28, 115, 116), Bartonella spp.: (14, 50, 51, 94, 103, 105), Borrelia spp.: (14, 27, 70, 72, 96, 105, 107, 117–122), Coxiella burnetii: (52, 79, 80, 93, 94, 100, 103, 105, 123–129), Ehrlichia spp.: (35), Francisella spp.: (14, 130), Rickettsia spp.: (14, 52, 72, 80, 87–90, 93, 94, 96–101, 103, 104, 117, 120, 131–135).
Keywords: Africa, zoonoses, tick-borne disease, aetiologies, human health, in vitro diagnostics
Citation: Adamu A, Reyer F, Lawal N, Hassan AJ, Imam MU, Bello MB and Kraiczy P (2024) Aetiologies of bacterial tick-borne febrile illnesses in humans in Africa: diagnostic limitations and the need for improvement. Front. Med. 11:1419575. doi: 10.3389/fmed.2024.1419575
Edited by:
Renata Šmit, Goethe University, GermanyReviewed by:
Ryan Oliver Marino Rego, Centre for Biology, Academy of Sciences of the Czech Republic (ASCR), CzechiaKokouvi Kassegne, Shanghai Jiao Tong University, China
Copyright © 2024 Adamu, Reyer, Lawal, Hassan, Imam, Bello and Kraiczy. This is an open-access article distributed under the terms of the Creative Commons Attribution License (CC BY). The use, distribution or reproduction in other forums is permitted, provided the original author(s) and the copyright owner(s) are credited and that the original publication in this journal is cited, in accordance with accepted academic practice. No use, distribution or reproduction is permitted which does not comply with these terms.
*Correspondence: Peter Kraiczy, a3JhaWN6eUBlbS51bmktZnJhbmtmdXJ0LmRl; Muhammad Bashir Bello, YmVsbG9tQGthaW1yYy5lZHUuc2E=