- Department of Rheumatology and Immunology, The First Affiliated Hospital of Guangxi Medical University, Nanning, China
Introduction: Immune cells are involved in the onset and progression of Sjögren’s syndrome (SS). This study explored the causal relationship between immune signature cells and SS, which has not been fully elucidated.
Methods: We conducted univariate, multivariate, and bidirectional Mendelian randomization to investigate the causal relationship between 731 immunological feature characteristic cells and SS pairs and explore the interaction of immune cells in SS.
Results: After false discovery rate correction, six immune cells were significantly associated with SS risk. Among them, four contributed to SS (CD24 on memory B cell, CD27 on IgD + CD24 + B cell, CD28 on CD39+ secreting CD4 Treg cell, and CD80 on CD62L + mDC); two appeared to reduce SS risk (CD3 on CD39 + CD8 + T cell and CD38 on IgD + CD38 + B cell). Pleiotropy and heterogeneity were not observed. Three immune cells exerted independent effects for SS (CD27 on IgD + CD24 + B cell, CD80 on CD62L + mDC, and CD38 on IgD + CD38 + B cell); two were risk factors (CD27 on IgD + CD24 + B cell and CD80 on CD62L + mDC); and one was a protective factor (CD38 on IgD + CD38 + B cell). Twenty-three immune cells showed a reverse causal relationship with SS.
Conclusion: These findings demonstrate the influence of immune cells on SS risk and the effects of SS on immune cells, providing new clues for further research on the mechanisms underlying SS.
Introduction
Sjögren’s syndrome (SS) is a heterogeneous, etiological, systemic autoimmune disease characterized by chronic inflammation and dysfunction of the exocrine glands. SS can affect different organs and tissues and is usually accompanied by sicca symptoms, such as fatigue, chronic pain, and multiple organ-related symptoms (1, 2). SS is the second most common autoimmune rheumatic disease, affecting between 0.4 and 3.1 million individuals (3). SS causes a health burden for patients and substantial social disease costs, necessitating early diagnosis and active intervention to improve the prognosis of SS.
The pathogenesis of SS is very complex and has not been fully elucidated. Increasingly more evidence has shown that immune system dysfunction plays a crucial role in the etiology of SS, and the interaction between inflammation and genetic and environmental variables influences the occurrence, development, and resulting tissue damage of SS (4). Studies have shown that disturbances of the innate immune barrier involving the interferon (IFN) pathway in the early stages of SS disease are involved in the etiology of SS (5, 6). Vogelsang et al. reported a significant reduction in myeloid dendritic cells (mDCs) and plasmacytoid dendritic cells (pDCs) in the peripheral blood as well as the presence of pDC in salivary glands of SS patients compared to healthy controls (7). Zhao et al. reported that plasma cell-type DCs were enriched in the minor salivary gland of SS, inducing CXCR5(+)CD19(+) B cells to accumulate by secreting type I IFN (8). In addition, the adaptive immune system plays an integral role in the development of SS, and polyclonal overactivation of B cells and proliferation of Th1 and Th17 cells contribute to the progression of the disease (9). Epithelial function is also involved in the complex etiology of SS (10). However, the results of studies on the causal relationship between SS and immune cells have been inconsistent to date, which may be due to insufficient sample sizes, confounding factors, and biases.
Recent advances in large-scale genome-wide association studies (GWASs) and Mendelian randomization (MR) methods have made it possible to assess causal relationships between immune cells and disease outcomes. Compared to other statistical methods, MR can reduce the bias caused by confounding and reverse causation (11, 12). In this study, our research applied univariate, multivariate, and bidirectional MR analyses to investigate the impact of multiple variables and the causal relationship between immune cells and SS risk to clarify the association between immune cell characteristics and SS.
Materials and methods
Study design
Our study investigated the causal associations between 731 immune cells and SS based on MR analysis. In the process of our research, MR analysis was used to observe three core assumptions to ensure unbiased causal effects: (1) Genetic variants are closely related to the exposure, (2) genetic variants are not associated with potential confounders, and (3) genetic variants affect outcomes only by the exposure pathway (13). Data summaries about immune cells and SS were acquired from publicly accessible GWAS. Therefore, there was no need to obtain ethical approval. The workflow of our research is shown in Figure 1.
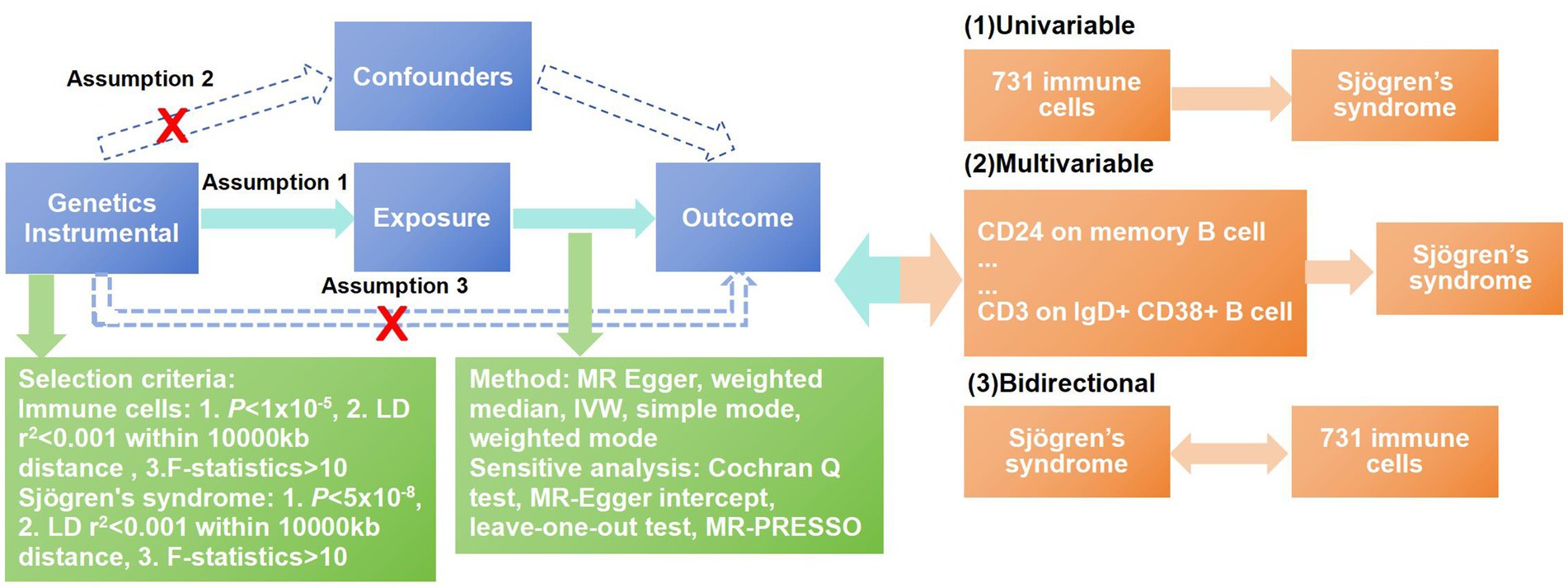
Figure 1. Workflow of the MR analysis. In this study, univariate Mendelian randomization (MR) was conducted to investigate the causal associations between immune cells and SS, and five methods were adopted to ensure the reliability of the result, including MR-Egger, weighted median, inverse-variance weighting (IVW), weighted mode and simple mode. The MR-PRESSO test, Cochran’s Q-test, MR-Egger intercept analysis, and leave-one-out test were used to observe the pleiotropy and heterogeneity of the results. To overcome the interference between immune cells, we used multivariable MR analysis to further correct the independent effects of these associated immune cells for SS. At last, a bidirectional MR analysis was conducted to explore the reverse causal relationships between immune cells and SS.
GWAS data source for 731 immune cells
The GWAS data for the 731 immune cells, which were acquired from a public catalog (GCST90001391-GCST90002121), were based on a study of the genetic characteristics of immune cells. The study aimed to examine the effects of 22 million genetic variations on 731 immune cell cells among 3,757 Sardinians and further verify the relationships among autoimmune illnesses and immunological characteristics. According to this study, we could understand the types of GWAS data, which include 389 median fluorescence intensity measurements representing surface antigen levels, 192 relative cell counts, 118 absolute cell counts, and 32 morphological characteristics (14).
GWAS data source for Sjögren’s syndrome
We chose the GWAS data for SS from the FinnGen dataset1 for MR analysis (dataset finn-b-M13_SJOGREN), including 16,380,454 single-nucleotide polymorphisms (SNPs), and the GWAS was performed on 214,435 Europeans (nCase = 1,290, nControl = 213,145).
Instrumental variable selection
Recent research indicated that when selecting important SNPs for various immune trait cells, we should choose a loose cutoff value of p < 1 × 10−5 (15). According to the 1,000 Genomes Projects reference panel, the linkage disequilibrium r2 threshold was set to be <0.001 within a 10,000-kb distance to remove the genetic linkage imbalance effect. In the reverse MR analysis, we chose a stricter standard value of p < 5 × 10−8 and r2 < 0.001 within a 10,000-kb distance. Finally, SNPs with low F statistics (<10) were removed to avoid weak instrumental bias, and the rest were used as instrumental variables for MR analysis.
Statistical analysis
Our study mainly adopted the inverse-variance weighting (IVW) method to observe the causal relationship between the 731 immune cell types and SS, while four additional supplemental methods were used, including MR-Egger, weighted median, weighted mode, and simple mode. We comprehensively analyzed the results of five methods to ensure the reliability of the results. At the same time, multiple methods were used to observe for possible pleiotropy and heterogeneity among the results. Cochran’s Q-test was utilized to assess for heterogeneity, the MR-Egger intercept was utilized to address and account for pleiotropy, the leave-one-out analysis was utilized to assess robustness, and the MR Pleiotropy RESidual Sum and Outlier (MR-PRESSO) test was used to assess for horizontal pleiotropy and detect value bias.
In our study, R statistical software (version 4.3.2) was used to complete all statistical analyses using “Two Sample MR” (version 0.5.8) packages and the MR-PRESSO package (version 1.0).
Results
The causal effect of immune cells on Sjögren’s syndrome
Through univariable MR analysis, 22 immune cells were found to be causally related to SS risk. After correcting for the false discovery rate (FDR), we identified six immune cells that have a causal relationship with SS, and they were, respectively, distributed in three B cells, two regulatory T cells (Tregs), and one classical DC (cDC). The result of applying the IVW method indicated that CD24 on memory B cell (odds ratio [OR] = 1.094, 95% confidence interval (CI) 1.026–1.165, p = 0.06, positive FDR [PFDR] = 0.031) increased the risk of SS, and the results of the other four methods were similar: MR-Egger (OR = 1.138, 95%CI 1.048–1.236, p = 0.005), weighted median (OR = 1.105, 95%CI 1.000–1.220, p = 0.049), simple mode (OR = 1.051, 95%CI 0.881–1.254, p = 0.584), and weighted mode (OR = 1.122, 95%CI 1.032–1.219, p = 0.011). CD27 on IgD+ CD24+ B cell (OR = 1.115, 95%CI 1.044–1.191, p = 0.001, PFDR = 0.027) also increased the risk of SS, and the results of the other four methods were similar: MR-Egger (OR = 1.153, 95%CI 1.053–1.262, p = 0.004), weighted median (OR = 1.151, 95%CI 1.041–1.271, p = 0.006), simple mode (OR = 1.005,95%CI 0.839–1.204, p = 0.958), and weighted mode (OR = 1.167,95%CI 1.064–1.280, p = 0.003). CD28 on CD39+ secreting CD4 regulatory T cell (OR = 1.096, 95%CI 1.006–1.195, p = 0.036, PFDR = 0.050) was a risk factor for SS, and the results of the other four methods were similar: MR-Egger (OR = 1.070, 95%CI 0.946–1.210, p = 0.295), weighted median (OR = 1.049, 95%CI 0.929–1.183, p = 0.440), simple mode (OR = 1.156, 95%CI 0.953–1.403, p = 0.155), and weighted mode (OR = 1.046, 95%CI 0.933–1.172, p = 0.451). CD80 on CD62L+ mDC (OR = 1.102, 95%CI 1.033–1.176, p = 0.003, PFDR = 0.023) was also a risk factor, and the results of the other four methods were similar: MR-Egger (OR = 1.090, 95%CI 0.998–1.189, p = 0.067), weighted median (OR = 1.078, 95%CI 0.987–1.178, p = 0.097), simple mode (OR = 1.066, 95%CI 0.916–1.241, p = 0.414), and weighted mode (OR = 1.086, 95%CI 1.002–1.177, p = 0.055). In addition, CD3 on CD39+ CD8+ T cell (OR = 0.884, 95%CI 0.782–1.000, p = 0.049, PFDR = 0.049) reduced the risk of SS, and the results of the other four methods were similar: MR-Egger (OR = 0.874, 95%CI 0.645–1.185, p = 0.402), weighted median (OR = 0.894, 95%CI 0.750–1.065, p = 0.208), simple mode (OR = 0.953, 95%CI 0.752–1.208, p = 0.699), and weighted mode (OR = 0.886, 95%CI 0.736–1.066, p = 0.220). CD38 on IgD+ CD38+ B cell (OR = 0.861, 95%CI 0.781–0.949, p = 0.003, PFDR = 0.028) reduced the risk of SS, and the results of the other four methods were similar: MR-Egger (OR = 0.833, 95%CI 0.707–0.981, p = 0.045), weighted median (OR = 0.867, 95%CI 0.754–0.998, p = 0.046), simple mode (OR = 0.789, 95%CI 0.633–0.983, p = 0.051), and weighted mode (OR = 0.859, 95%CI 0.748–0.987, p = 0.048) (Table 1). Furthermore, we used Cochran’s Q-test to assess heterogeneity and the MR-Egger intercept to account for pleiotropy; and the p-values of all results were greater than 0.05 (Table 2). The leave-one-out analysis showed that these data were stable. Finally, we did not observe any horizontal pleiotropy nor biased values through the MR-PRESSO test (Table 2) (Supplementary material S1).
Multivariable MR analysis
To further assess the independent causal effect of immune cells on SS, we conducted a multivariate MR analysis (Figure 2). We found that only three immune cells exerted independent effects for SS: CD27 on IgD+ CD24+ B cell (OR = 1.111, 95%CI 1.038–1.189, p = 0.002); CD80 on CD62L+ mDC (OR = 1.105, 95%CI 1.034–1.181, p = 0.003) increased the risk of SS; and CD38 on IgD+ CD38+ B cell (OR = 0.893, 95%CI 0.818–0.975, p = 0.011) reduced the risk of SS. Remarkably, our results of multivariate MR analysis were broadly consistent with previous analyses, indicating that our results were highly reliable.
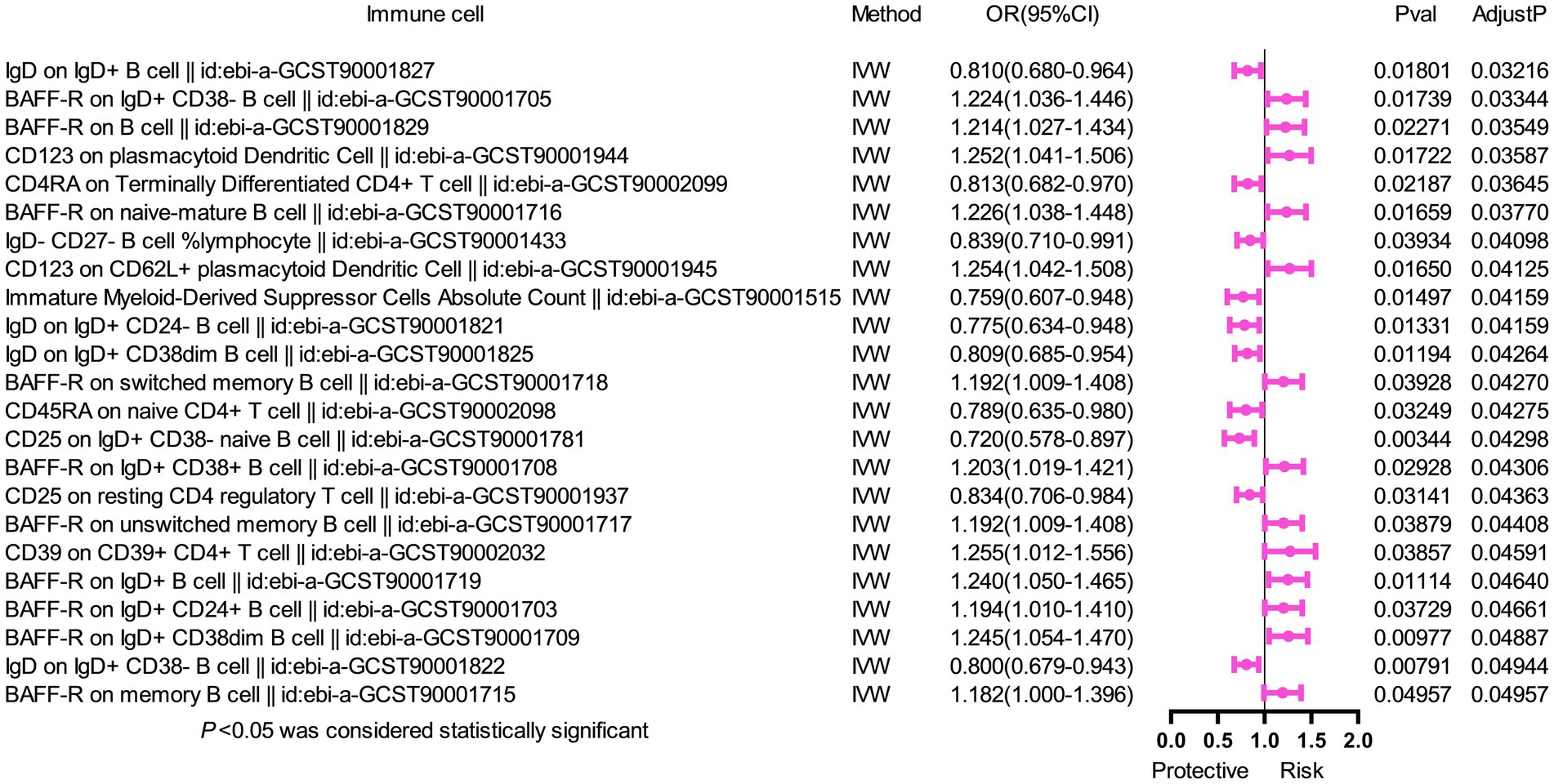
Figure 2. Forest plot of multivariable MR analysis between six identified immune cells and Sjögren’s syndrome. Multivariate MR analysis showed that three immunophenotypes remained statistically significant, while the other phenotypes lost significance. Two immunophenotypes (CD27 on IgD+ CD24+ B cell and CD80 on CD62L+ myeloid dendritic cell) were risk factors, and one immunophenotype (CD38 on IgD+ CD38+ B cell) was a protective factor.
Exploration of the causal effect of SS on immune cells
We conducted the MR analysis to explore the causal effect of SS on immune cells. We found that 25 immune cells had important relationships with SS. After correcting for FDR, 23 immune cells (Figure 3) were identified to have reverse causal associations with SS, including B cell (16 cells), Treg (2 cells), cDC (2 cells), the maturation stage of T cells (2 cells), and mDC (1 cell). However, we did not observe a bidirectional causal relationship between immune cells and SS. SS was a risk factor for 13 types of immune cells, including BAFF-R on IgD+ CD38- B cell (OR = 1.224, 95%CI 1.036–1.446, p = 0.017, PFDR = 0.033), BAFF-R on B cell (OR = 1.214, 95%CI 1.027–1.434, p = 0.023, PFDR = 0.035), CD123 on pDC (OR = 1.252, 95%CI 1.041–1.506, p = 0.017, PFDR = 0.036), BAFF-R on naive-mature B cell (OR = 1.226, 95%CI 1.038–1.448, p = 0.017, PFDR = 0.038), CD123 on CD62L+ pDC (OR = 1.254, 95%CI 1.042–1.508, p = 0.017, PFDR = 0.041), BAFF-R on switched memory B cell (OR = 1.192, 95%CI 1.009–1.408, p = 0.039, PFDR = 0.043), BAFF-R on IgD+ CD38+ B cell (OR = 1.203, 95%CI 1.019–1.421, p = 0.029, PFDR = 0.043), BAFF-R on unswitched memory B cell (OR = 1.192,95%CI 1.009–1.408, p = 0.039, PFDR = 0.044), CD39 on CD39+ CD4+ T cell (OR = 1.255,95%CI 1.012–1.556, p = 0.039, PFDR = 0.046), BAFF-R on IgD+ B cell (OR = 1.240,95%CI 1.050–1.465, p = 0.011, PFDR = 0.046), BAFF-R on IgD+ CD24+ B cell (OR = 1.194,95%CI 1.010–1.410, p = 0.037, PFDR = 0.047), BAFF-R on IgD+ CD38dim B cell (OR = 1.245,95%CI 1.054–1.470, p = 0.010, PFDR = 0.049), and BAFF-R on memory B cell (OR = 1.182,95%CI 1.000–1.396, p = 0.050, PFDR = 0.050). In addition, SS was a protective factor for 10 types of immune cells, including IgD on IgD+ B cell (OR = 0.810, 95%CI 0.680–0.964, p = 0.018, PFDR = 0.032), CD4RA on terminally differentiated CD4+ T cell (OR = 0.813, 95%CI 0.682–0.970, p = 0.022, PFDR = 0.036), IgD- CD27- B cell %lymphocyte (OR = 0.839,95%CI 0.710–0.991, p = 0.039, PFDR = 0.041), immature myeloid-derived suppressor cells absolute count (OR = 0.759,95%CI 0.607–0.948, p = 0.015, PFDR = 0.042), IgD on IgD+ CD24- B cell (OR = 0.775,95%CI 0.634–0.948, p = 0.013, PFDR = 0.042), IgD on IgD+ CD38dim B cell (OR = 0.809,95%CI 0.685–0.954, p = 0.012, PFDR = 0.043), CD45RA on naive CD4+ T cell (OR = 0.789,95%CI 0.635–0.980, p = 0.032, PFDR = 0.043), CD25 on IgD+ CD38- naive B cell (OR = 0.720,95%CI 0.578–0.897, p = 0.003, PFDR = 0.043), CD25 on resting CD4 regulatory T cell (OR = 0.834,95%CI 0.706–0.984, p = 0.031, PFDR = 0.044), and IgD on IgD+ CD38-B cell (OR = 0.800,95%CI 0.679–0.943, p = 0.008, PFDR = 0.049). In the same way, the results of the other four methods were similar to those of the IVW method (Supplementary material S2). Furthermore, we found no evidence of heterogeneity and pleiotropy via Cochran’s Q-test and MR-Egger intercept analysis (Table 3), so we could assume that there was no horizontal pleiotropy. Finally, the leave-one-out test suggested that the result of reverse MR analysis was also stable (Supplementary material S2).
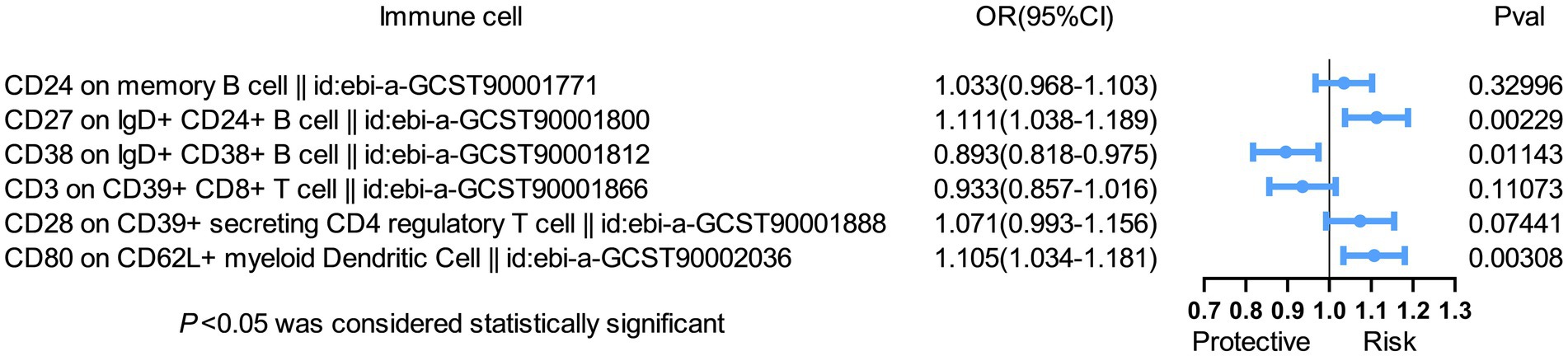
Figure 3. Forest plot with MR analysis showed that SS affected 25 immune cells. MR analysis showed that SS affected 25 immune cells. After correcting for FDR, 23 immune cells, belonging to B cell (16 cells), Treg (2 cells), cDC (2 cells), the maturation stage of T cells (2 cells), and myeloid cells (1 cell) were identified to have a reverse causal relationship with SS.
Discussion
In our study, we conducted MR to investigate the causal relationship between 731 immune cells and SS. In this study, we found a strong causal relationship between six immune cells for SS (PFDR < 0.05) and 23 immune cells for SS (PFDR < 0.05). Our results provide further insight into the causal relationship between immune cells and SS. As far as we know, this is the first univariate, bivariate, and bidirectional MR analysis performed to investigate the link between immune cells and SS.
We observed that two types of T cells were significantly associated with the risk of SS, with elevated CD28 on CD39+ secreting CD4 regulatory T cells increasing the risk of SS, and elevated CD3 on CD39+ CD8+ T cells decreasing the risk of SS. CD39 plays an important role in the immune system. CD39+ CD8+ T cells inhibit the production of IFN-γ by CD39- CD8+ T cells through paracrine secretion of adenosine, which operates through the A2A receptor (16). Early disturbance of the innate immune barrier involving the IFN pathway in SS disease is associated with the etiology of SS (6). IFN-γ induces salivary gland epithelial cell ferroptosis in SS (17). An increase in the number of CD39+ CD8+ T cells found in Crohn’s patients is correlated with enhanced signal transduction of reactive oxygen species (ROS) (16). The ROS/pSTAT4/important protein aquaporin 5 axis affects salivary dysfunction in SS (18). Although some studies have focused on Tregs and SS, the role of Tregs in the occurrence and development of SS remains elusive. Sarigul et al. reported that the increase of Foxp3+ Treg cells in the peripheral blood of SS patients was positively correlated with a higher grade of infiltration at the salivary glands (19). Alunno et al. reported an expansion of CD4+ CD25- GITR+ regulatory T cell subsets in the peripheral blood of patients with primary SS (pSS)’, which was correlated with the degree of disease activity (20). The correlation between CD28 on CD39+ secreting CD4 regulatory T cell and SS has not been clarified at present. In other autoimmune diseases, an increase in peripheral CD39-expressing T regulatory cells has been associated with relapsing–remitting multiple sclerosis (21). In addition, in patients with type 2 diabetes, elevated CD39+ Treg cells are associated with hyperglycemia, overweight, and obesity (22). The collaboration of CD39 and CD73 results in the conversion of ATP to ADP and adenosine 5′-monophosphate (cAMP), ultimately generating adenosine (23, 24). Adenosine plays an immunosuppressive role when interacting with A2A and A2B receptors but stimulates an immune response when interacting with A1 and A3 receptors (25–27). These findings may be the reason why CD28 on CD39+ secreting CD4 regulatory T cell is a risk factor for SS, whereas CD3 on CD39+ CD8+ T cell plays a protective role in SS. However, further experiments are needed to clarify the relevant mechanisms. cAMP can activate the mitogen-activated protein kinase (MAPK) pathway, thereby inhibiting nicotinamide adenine dinucleotide phosphate oxidase and alleviating TGF-B1-induced salivary gland fibrosis. CD28 on CD39+ secreting CD4 regulatory T cell and CD3 on CD39+ CD8+ T cell may affect the pathogenesis and development of SS through adenosine (and its derivatives) or IFN, which needs to be clarified by validation and functional evaluation experiments.
In addition, our results showed that three phenotypes of B cells were associated with SS risk. Elevations of CD24 on memory B cells and CD27 on IgD+ CD24+ B cells increased the risk of SS, while CD38 on IgD+ CD38+ B cells decreased the risk of SS. Polyclonal over-proliferation of B cells is one of the immunological features of SS (9). CD24 expression on pro-B cells plays a role in the selection and development of B cells in bone marrow. In memory B cells, there was a strong positive correlation between CD24 expression and phosphorylation flow (phosphorylation of AMPK-pAMPK), especially in IgD+ IgM+ memory B cells (28). MAPKs are downstream of many immune and cytokine receptors, such as toll-like receptors, interleukin (IL)-1R, tumor necrosis factor receptor, colony-stimulating factor 1 receptor, IL-17R, epidermal cell growth factor, fibroblast growth factor, and vascular endothelial growth factor (29). These immune and cellular factors are involved in the occurrence or development of SS and its complications, to varying degrees (30–34).
Peripheral blood IgD+ CD38+ B cells, also known as naïve B cells, were significantly higher in SS patients than in healthy donors and higher in women than men (35, 36). An increased proportion of CD38 high IgD+ B cells in pSS is involved in IgG overproduction, including autoantibodies, and correlates with disease progression (37). In patients with pSS treated with iguratimod, CD38+ IgD+ B cells and BAFF-R were significantly reduced, while disease activity scores were decreased (38). This is not consistent with our findings, suggesting that IgD+ CD38+ B cells reduce the risk of SS, but the results of MR analysis could not fully reveal the relationship between immune cells and SS and need to be confirmed in combination with experiments. It is also possible that interference between immune cells causes their true role in SS to be obscured.
Furthermore, our results showed that the elevation of CD80 on CD62L+ mDCs was positively correlated with the risk of SS and remained significantly correlated after adjusting for multivariate MR. mDCs undergo many activities that contribute to the initiation of immunity. There are two main subtypes of human DCs: mDCs and pDCs. pDCs primarily drive innate inflammatory responses to pathogens by secreting large amounts of IFN-alpha (IFNα), whereas mDCs are specifically used for antigen presentation to guide adaptive responses (39, 40). CD80 on CD62L+ is the more mature mDCs phenotype, and mature DCs are stimulators of T cell immune response, whereas immature DCs support T cell tolerance (41). Compared to healthy controls, pDC and mDC2 in the peripheral blood of pSS patients were significantly reduced (40). Compared to non-S’S dry eye and healthy volunteers, SS’ dry eye showed significantly higher DC density, larger DC size, and more DC dendrites with a larger DC field. Moreover, DC density and morphological parameters were significantly correlated with the degree of salivary gland pathology, serum antibody titer, and ocular surface damage (42). However, there is no literature on the change in CD80 on CD62L+ mDC expression level in SS, which is worthy of further exploration.
Our results showed that SS was a risk factor or protective factor for 23 immunophenotypes, including 16 B cells, 4 T cells, 2 cDC cells, and 1 mDC. Our results confirm that SS is a B cell-associated disease at the genetic level. BAFF is a member of the TNF superfamily by binding to the transmembrane activator, calcium modulator, and cyclophilin ligand interactor or BAFF-R on B cells. BAFF supports B cell development, differentiation, and survival, especially for plasma and plasma cells, and plays a key role in the pathogenesis of B cell-associated autoimmune diseases. BAFF therapy targeting B cells in SS’ helps reduce disease activity in SS and restores normalization of B cell frequency, phenotype, and function (43, 44). The results of this study provide potential indicators and clues for the early diagnosis and activity assessment of SS, which can be further explored and validated in future clinical cases.
Considered together, our findings demonstrate that immune cells play a potential causal role in SS, providing an important auxiliary role for clarifying diagnosis and therapeutic strategies, as well as providing directions for the development of new drugs. Our MR analysis offered several advantages. First, we used univariate, multivariate, and bidirectional MR to mitigate confounding factors and reverse causation. Second, our study adopted five methods to ensure the reliability of the result, including MR-Egger, weighted median, IVW, weighted mode, and simple mode. At the same time, multiple methods were used to observe pleiotropy and heterogeneity of the results, such as the MR-PRESSO test, Cochran’s Q-test, MR-Egger intercept analysis, and leave-one-out test, to ensure that our MR results were robust and reliable, with no apparent bias from other sources of pleiotropy. However, our study has limitations. First, in this study, multifactor, bidirectional MR analysis was performed, and the results showed that six types of immune cells may be associated with the risk of SS. However, MR analysis could not reveal the causal relationship between immune cells and SS, but it provides new avenues for studying the mechanisms of SS. Further experiments are needed to evaluate and validate the function of the selected immune cells by analyzing patient clinical data and biological samples. Second, the GWAS data used in this study were derived from European populations, so may not be directly applicable to other populations. Third, GWAS data were currently unable to distinguish between pSS and secondary SS, which made it impossible to perform subgroup stratification analyses of the SS population. Fourth, the limited sample size could introduce bias, so a larger sample is needed to obtain reliable results.
Conclusion
This study used univariate, multivariate, and bidirectional MR analysis to investigate the causal relationship between several immune cells and SS and to clarify that immune cells affect the progression of SS in a complex pattern. These findings improve our understanding of the interaction between immune cells and SS risk, providing new avenues for studying the prevention, diagnosis, and treatment of SS. Nevertheless, further experiments are needed to elucidate the underlying mechanisms.
Data availability statement
The original contributions presented in the study are included in the article/Supplementary material, further inquiries can be directed to the corresponding author.
Ethics statement
Ethical approval was not required for the study involving humans in accordance with the local legislation and institutional requirements. Written informed consent to participate in this study was not required from the participants or the participants’ legal guardians/next of kin in accordance with the national legislation and the institutional requirements.
Author contributions
WZ: Conceptualization, Data curation, Formal analysis, Funding acquisition, Investigation, Methodology, Project administration, Resources, Software, Supervision, Validation, Visualization, Writing – original draft, Writing – review & editing. MH: Writing – original draft, Conceptualization, Data curation, Formal analysis, Funding acquisition, Investigation, Methodology, Project administration, Resources, Software, Supervision, Validation, Visualization. YZ: Conceptualization, Data curation, Formal analysis, Funding acquisition, Investigation, Methodology, Project administration, Resources, Software, Supervision, Validation, Visualization, Writing – review & editing. JP: Conceptualization, Software, Writing – original draft. FQ: Methodology, Project administration, Writing – original draft. XL: Conceptualization, Investigation, Writing – review & editing. LZ: Data curation, Formal analysis, Writing – original draft. LL: Conceptualization, Software, Writing – original draft, Writing – review & editing.
Funding
The author(s) declare financial support was received for the research, authorship, and/or publication of this article. This study was supported in part by grants from The National Natural Science Foundation of China (Regional Science Foundation Project, #82060300), the Guangxi Natural Science Foundation (#2023GXNSFDA026061), and the Guangxi Medical and Health Technology Development and Promotion Project (#S2022075).
Acknowledgments
The authors are grateful for the FinnGen database, the public catalog of GWAS data provided, and the relevant participants and researchers.
Conflict of interest
The authors declare that the research was conducted in the absence of any commercial or financial relationships that could be construed as a potential conflict of interest.
Publisher’s note
All claims expressed in this article are solely those of the authors and do not necessarily represent those of their affiliated organizations, or those of the publisher, the editors and the reviewers. Any product that may be evaluated in this article, or claim that may be made by its manufacturer, is not guaranteed or endorsed by the publisher.
Supplementary material
The Supplementary material for this article can be found online at: https://www.frontiersin.org/articles/10.3389/fmed.2024.1408562/full#supplementary-material
Footnotes
References
1. Shiboski, CH, Shiboski, SC, Seror, R, Criswell, LA, Labetoulle, M, Lietman, TM, et al. 2016 American College of Rheumatology/European league against rheumatism classification criteria for primary Sjögren's syndrome: a consensus and data-driven methodology involving three international patient cohorts. Arthritis Rheumatol. (2017) 69:35–45. doi: 10.1002/art.39859
2. Vivino, FB. Sjogren's syndrome: clinical aspects. Clin Immunol. (2017) 182:48–54. doi: 10.1016/j.clim.2017.04.005
3. Helmick, CG, Felson, DT, Lawrence, RC, Gabriel, S, Hirsch, R, Kwoh, CK, et al. Estimates of the prevalence of arthritis and other rheumatic conditions in the United States. Part I. Arthritis Rheum. (2008) 58:15–25. doi: 10.1002/art.23177
4. Negrini, S, Emmi, G, Greco, M, Borro, M, Sardanelli, F, Murdaca, G, et al. Sjögren's syndrome: a systemic autoimmune disease. Clin Exp Med. (2022) 22:9–25. doi: 10.1007/s10238-021-00728-6
5. Brito-Zerón, P, Baldini, C, Bootsma, H, Bowman, SJ, Jonsson, R, Mariette, X, et al. Sjögren syndrome. Nat Rev Dis Primers. (2016) 2:16047. doi: 10.1038/nrdp.2016.47
6. Shimizu, T, Nakamura, H, and Kawakami, A. Role of the innate immunity signaling pathway in the pathogenesis of Sjögren's syndrome. Int J Mol Sci. (2021) 22:90. doi: 10.3390/ijms22063090
7. Vogelsang, P, Brun, JG, Oijordsbakken, G, Skarstein, K, Jonsson, R, and Appel, S. Levels of plasmacytoid dendritic cells and type-2 myeloid dendritic cells are reduced in peripheral blood of patients with primary Sjogren's syndrome. Ann Rheum Dis. (2010) 69:1235–8. doi: 10.1136/ard.2009.118158
8. Zhao, J, Kubo, S, Nakayamada, S, Shimajiri, S, Zhang, X, Yamaoka, K, et al. Association of plasmacytoid dendritic cells with B cell infiltration in minor salivary glands in patients with Sjögren's syndrome. Mod Rheumatol. (2016) 26:716–24. doi: 10.3109/14397595.2015.1129694
9. Chivasso, C, Sarrand, J, Perret, J, Delporte, C, and Soyfoo, MS. The involvement of innate and adaptive immunity in the initiation and perpetuation of Sjögren's syndrome. Int J Mol Sci. (2021) 22:658. doi: 10.3390/ijms22020658
10. Goules, AV, Kapsogeorgou, EK, and Tzioufas, AG. Insight into pathogenesis of Sjögren's syndrome: dissection on autoimmune infiltrates and epithelial cells. Clin Immunol. (2017) 182:30–40. doi: 10.1016/j.clim.2017.03.007
11. Bowden, J, and Holmes, MV. Meta-analysis and Mendelian randomization: a review. Res Synth Methods. (2019) 10:486–96. doi: 10.1002/jrsm.1346
12. Davey Smith, G, and Hemani, G. Mendelian randomization: genetic anchors for causal inference in epidemiological studies. Hum Mol Genet. (2014) 23:R89–98. doi: 10.1093/hmg/ddu328
13. Davies, NM, Holmes, MV, and Davey, SG. Reading Mendelian randomisation studies: a guide, glossary, and checklist for clinicians. BMJ. (2018) 362:k601. doi: 10.1136/bmj.k601
14. Orrù, V, Steri, M, Sidore, C, Marongiu, M, Serra, V, Olla, S, et al. Complex genetic signatures in immune cells underlie autoimmunity and inform therapy. Nat Genet. (2020) 52:1036–45. doi: 10.1038/s41588-020-0684-4
15. Wang, C, Zhu, D, Zhang, D, Zuo, X, Yao, L, Liu, T, et al. Causal role of immune cells in schizophrenia: Mendelian randomization (MR) study. BMC Psychiatry. (2023) 23:590. doi: 10.1186/s12888-023-05081-4
16. Bai, A, Moss, A, Rothweiler, S, Serena Longhi, M, Wu, Y, Junger, WG, et al. NADH oxidase-dependent CD39 expression by CD8(+) T cells modulates interferon gamma responses via generation of adenosine. Nat Commun. (2015) 6:8819. doi: 10.1038/ncomms9819
17. Cao, T, Zhou, J, Liu, Q, Mao, T, Chen, B, Wu, Q, et al. Interferon-γ induces salivary gland epithelial cell ferroptosis in Sjogren's syndrome via JAK/STAT1-mediated inhibition of system xc. Free Radic. Bio Med. (2023) 205:116–28. doi: 10.1016/j.freeradbiomed.2023.05.027
18. Zhou, J, Pathak, JL, Wu, L, Chen, B, Cao, T, Wei, W, et al. Downregulated GPX4 in salivary gland epithelial cells contributes to salivary secretion dysfunction in Sjogren's syndrome via lipid ROS/pSTAT4/AQP5 axis. Free Radic. Bio Med. (2024) 218:1–15. doi: 10.1016/j.freeradbiomed.2024.04.003
19. Sarigul, M, Yazisiz, V, Başsorgun, CI, Ulker, M, Avci, AB, Erbasan, F, et al. The numbers of Foxp3 + Treg cells are positively correlated with higher grade of infiltration at the salivary glands in primary Sjogren's syndrome. Lupus. (2010) 19:138–45. doi: 10.1177/0961203309348234
20. Liu, C, Guan, Z, Zhao, L, Song, Y, and Wang, H. Elevated level of circulating CD4+Helios+FoxP3+ cells in primary Sjogren's syndrome patients. Mod Rheumatol. (2017) 27:630–7. doi: 10.1080/14397595.2016.1226470
21. Álvarez-Sánchez, N, Cruz-Chamorro, I, Díaz-Sánchez, M, Lardone, PJ, Guerrero, JM, and Carrillo-Vico, A. Peripheral CD39-expressing T regulatory cells are increased and associated with relapsing-remitting multiple sclerosis in relapsing patients. Sci Rep. (2019) 9:2302. doi: 10.1038/s41598-019-38897-w
22. Cortez-Espinosa, N, Cortés-Garcia, JD, Martínez-Leija, E, Rodríguez-Rivera, JG, Barajas-López, C, González-Amaro, R, et al. CD39 expression on Treg and Th17 cells is associated with metabolic factors in patients with type 2 diabetes. Hum Immunol. (2015) 76:622–30. doi: 10.1016/j.humimm.2015.09.007
23. Ohta, A, and Sitkovsky, M. Role of G-protein-coupled adenosine receptors in downregulation of inflammation and protection from tissue damage. Nature. (2001) 414:916–20. doi: 10.1038/414916a
24. Lima, CF, Tamegnon, A, Rodriguez, S, Maru, D, Martin, PL, Cooper, ZA, et al. Exploring the expression of adenosine pathway-related markers CD73 and CD39 in colorectal and pancreatic carcinomas characterized by multiplex immunofluorescence: a pilot study. Pathobiology. (2023) 91:205–18. doi: 10.1159/000534677
25. Moesta, AK, Li, XY, and Smyth, MJ. Targeting CD39 in cancer. Nat Rev Immunol. (2020) 20:739–55. doi: 10.1038/s41577-020-0376-4
26. Mittal, D, Sinha, D, Barkauskas, D, Young, A, Kalimutho, M, Stannard, K, et al. Adenosine 2B receptor expression on Cancer cells promotes metastasis. Cancer Res. (2016) 76:4372–82. doi: 10.1158/0008-5472.CAN-16-0544
27. Huang, S, Apasov, S, Koshiba, M, and Sitkovsky, M. Role of A2a extracellular adenosine receptor-mediated signaling in adenosine-mediated inhibition of T-cell activation and expansion. Blood. (1997) 90:1600–10. doi: 10.1182/blood.V90.4.1600
28. Mensah, FFK, Armstrong, CW, Reddy, V, Bansal, AS, Berkovitz, S, Leandro, MJ, et al. CD24 expression and B cell maturation shows a novel link with energy metabolism: potential implications for patients with Myalgic encephalomyelitis/chronic fatigue syndrome. Front Immunol. (2018) 9:2421. doi: 10.3389/fimmu.2018.02421
29. Zarrin, AA, Bao, K, Lupardus, P, and Vucic, D. Kinase inhibition in autoimmunity and inflammation. Nat Rev Drug Discov. (2021) 20:39–63. doi: 10.1038/s41573-020-0082-8
30. Zhang, K, Luo, Z, Zhu, X, Yao, X, Lu, D, Chen, L, et al. Identification of key genes for Pyroptosis-induced salivary gland inflammation in Sjogren's syndrome based on microarray data and immunohistochemistry analysis. J Inflamm Res. (2023) 16:5865–79. doi: 10.2147/JIR.S435008
31. Routsias, JG, and Tzioufas, AG. Autoimmune response and target autoantigens in Sjogren's syndrome. Eur J Clin Investig. (2010) 40:1026–36. doi: 10.1111/j.1365-2362.2010.02342.x
32. Mavragani, CP, and Crow, MK. Activation of the type I interferon pathway in primary Sjogren's syndrome. J Autoimmun. (2010) 35:225–31. doi: 10.1016/j.jaut.2010.06.012
33. Manoussakis, MN, Spachidou, MP, and Maratheftis, CI. Salivary epithelial cells from Sjogren's syndrome patients are highly sensitive to anoikis induced by TLR-3 ligation. J Autoimmun. (2010) 35:212–8. doi: 10.1016/j.jaut.2010.06.010
34. Gilboa-Geffen, A, Wolf, Y, Hanin, G, Melamed-Book, N, Pick, M, Bennett, ER, et al. Activation of the alternative NFκB pathway improves disease symptoms in a model of Sjogren's syndrome. PLoS One. (2011) 6:e28727. doi: 10.1371/journal.pone.0028727
35. Bohnhorst, J, Bjørgan, MB, Thoen, JE, Natvig, JB, and Thompson, KM. Bm1-Bm5 classification of peripheral blood B cells reveals circulating germinal center founder cells in healthy individuals and disturbance in the B cell subpopulations in patients with primary Sjögren's syndrome. J Immunol. (2001) 167:3610–8. doi: 10.4049/jimmunol.167.7.3610
36. Feng, R, Zhao, J, Sun, F, Miao, M, Sun, X, He, J, et al. Comparison of the deep immune profiling of B cell subsets between healthy adults and Sjögren's syndrome. Ann Med. (2022) 54:472–83. doi: 10.1080/07853890.2022.2031272
37. Ishioka-Takei, E, Yoshimoto, K, Suzuki, K, Nishikawa, A, Yasuoka, H, Yamaoka, K, et al. Increased proportion of a CD38highIgD+ B cell subset in peripheral blood is associated with clinical and immunological features in patients with primary Sjögren's syndrome. Clin Immunol. (2018) 187:85–91. doi: 10.1016/j.clim.2017.10.008
38. Jiang, W, Zhang, L, Zhao, Y, He, X, Hu, C, and Liu, Y. The efficacy and mechanism for action of iguratimod in primary Sjögren's syndrome patients. Int Ophthalmol. (2020) 40:3059–65. doi: 10.1007/s10792-020-01490-6
39. Derby, N, Martinelli, E, and Robbiani, M. Myeloid dendritic cells in HIV-1 infection. Curr Opin HIV AIDS. (2011) 6:379–84. doi: 10.1097/COH.0b013e3283499d63
40. Coccia, EM. IFN regulation and functions in myeloid dendritic cells. Cytokine Growth. (2008) 19:21–32. doi: 10.1016/j.cytogfr.2007.10.005
41. Morva, A, Lemoine, S, Achour, A, Pers, JO, Youinou, P, and Jamin, C. Maturation and function of human dendritic cells are regulated by B lymphocytes. Blood. (2012) 119:106–14. doi: 10.1182/blood-2011-06-360768
42. Hao, R, Ding, Y, and Li, X. Alterations in corneal epithelial dendritic cell in Sjogren's syndrome dry eye and clinical correlations. Sci Rep. (2022) 12:11167. doi: 10.1038/s41598-022-15537-4
43. Pontarini, E, Fabris, M, Quartuccio, L, Cappeletti, M, Calcaterra, F, Roberto, A, et al. Treatment with belimumab restores B cell subsets and their expression of B cell activating factor receptor in patients with primary Sjogren's syndrome. Rheumatology. (2015) 54:1429–34. doi: 10.1093/rheumatology/kev005
44. Evans, LS, Lewis, KE, DeMonte, D, Bhandari, JG, Garrett, LB, Kuijper, JL, et al. Povetacicept, an enhanced dual APRIL/BAFF antagonist that modulates B lymphocytes and pathogenic autoantibodies for the treatment of lupus and other B cell-related autoimmune diseases. Arthritis Rheumatol. (2023) 75:1187–202. doi: 10.1002/art.42462
Keywords: Mendelian randomization, immune cells, Sjögren’s syndrome, causal relationship, B cell
Citation: Zeng W, Huang M, Zeng Y, Pan J, Qin F, Liao X, Zheng L and Lei L (2024) The causal relationship between immune cells and Sjögren’s syndrome: a univariate, multivariate, bidirectional Mendelian randomized study. Front. Med. 11:1408562. doi: 10.3389/fmed.2024.1408562
Edited by:
Zhiming Lin, Third Affiliated Hospital of Sun Yat-sen University, ChinaReviewed by:
Chaozheng Li, Sun Yat-sen University, ChinaJiuliang Zhao, Peking Union Medical College Hospital (CAMS), China
Xinxiang Huang, People’s Hospital of Guangxi Zhuang Autonomous Region, China
Copyright © 2024 Zeng, Huang, Zeng, Pan, Qin, Liao, Zheng and Lei. This is an open-access article distributed under the terms of the Creative Commons Attribution License (CC BY). The use, distribution or reproduction in other forums is permitted, provided the original author(s) and the copyright owner(s) are credited and that the original publication in this journal is cited, in accordance with accepted academic practice. No use, distribution or reproduction is permitted which does not comply with these terms.
*Correspondence: Ling Lei, bGVpbGluZzE5NzJAMTYzLmNvbQ==
†These authors share first authorship