- 1Department of Orthopedic Surgery, Peking Union Medical College Hospital, Peking Union Medical College and Chinese Academy of Medical Sciences, Beijing, China
- 2Fundamental Industry Training Center, Tsinghua University, Beijing, China
Background: Augmented reality (AR) technology is gradually being applied in surgical teaching as an innovative teaching method. Developing innovative teaching methods to replicate clinical theory and practical teaching scenarios, simulate preoperative planning and training for bone tumor surgery, and offer enhanced training opportunities for young physicians to acquire and apply clinical knowledge is a crucial concern that impacts the advancement of the discipline and the educational standards for young orthopedic physicians.
Objective: This study explores the application effect of augmented reality technology in anatomy teaching and surgical clinical teaching for spinal tumor.
Methods: The method utilizes virtual reality and augmented reality technology to present a spinal tumor model and the surgical process of percutaneous vertebroplasty. We conducted a random selection of 12 students forming into the augmented reality teaching group and 13 students forming into the traditional teaching group among the 8-year medical students from Peking Union Medical College and Tsinghua University, ensuring that the age and learning stage of the students in both groups were similar. Two groups of students were taught using traditional teaching methods and augmented reality technology-assisted teaching methods, respectively. A questionnaire survey was conducted after class to assess the quality of course instruction, student motivation in learning, their proficiency in anatomical structures, their comprehension of spinal tumor growth and metastasis, and their understanding and proficiency in percutaneous vertebroplasty.
Results: This study was the first to apply augmented reality technology in teaching, using spinal tumors and percutaneous vertebroplasty as examples, a head-mounted augmented reality device was used to create learning scenarios, presenting the complex three-dimensional spatial structure intuitively. The two groups of students differ significantly in their rating of teaching quality, enthusiasm for learning, knowledge of anatomical features, understanding of spinal trabecular structure, and understanding of steps in percutaneous vertebroplasty. The augmented reality technology-assisted teaching system demonstrates outstanding advantages.
Conclusion: Augmented reality technology has great potential and broad prospects in teaching bone tumors, which can help improve the visualization, interactivity, and three-dimensional spatial sense of medical teaching in spinal tumor. The application and development prospects of using augmented reality technology for anatomy instruction, surgical teaching, and simulation training are extensive.
Background
In the past decade, extended reality (XR) technology has developed exponentially, overlaying virtual reality (VR) onto the real-world environment to display information and data at various levels of the reality-virtuality continuum (1, 2). Augmented reality (AR) technology combines computer-generated three-dimensional (3D) virtual models with real-world scenes to achieve real-time interaction among users, the physical environment, and digital content. It has gradually been implemented in medical clinical teaching and practice (3, 4). Multiple studies have demonstrated that augmented reality technology, as a cutting-edge instructional approach, can be applied in orthopedic teaching, encompassing sub-specialties like trauma, joint surgery, spinal surgery, and sports medicine (5–10). This application can potentially enhance the caliber and efficacy of course instruction.
The knowledge and theory related to bone and soft tissue tumor surgery are complex, the skill learning curve is long, and students have limited practical opportunities. Conventional instructional approaches pose challenges in resolving numerous real-world issues, while AR technology integrates virtual and real environments. Providing students with an immersive learning experience in bone tumor surgery can yield several benefits, including improved teaching efficiency, increased student engagement, and enhanced course satisfaction (11, 12). Furthermore, some studies have confirmed that AR technology can simulate clinical theory and practical teaching scenarios, simulate preoperative planning and training for bone tumor surgery, and provide valuable opportunities for young physicians to learn and practice clinical knowledge, which is conducive to promoting the reform and development of traditional bone tumor surgery teaching (13, 14). This study aims to elucidate the practical significance of utilizing augmented reality technology in instructing spinal tumor anatomy and surgical clinical teaching for the first time. Simultaneously, the advantages and disadvantages of this technology application in teaching practice are summarized, and the future development trend of AR technology is thoroughly discussed.
Materials and methods
Basic materials
During the initial preparation process of this study, the orthopedic bone and soft tissue tumor team at Peking Union Medical College Hospital provided relevant data. The medical team exemplified surgical instruments used for spinal tumors and percutaneous vertebroplasty, elucidated the surgical steps, and demonstrated the operative process. Based on this premise, the team from the Fundamental Industry Training Center of Tsinghua University utilized simulation technique to create interactive anatomical program of spinal tumor and surgical operation procedure for percutaneous vertebroplasty (PVP). This primarily involved script development for AR-assisted spinal tumor and percutaneous vertebroplasty surgery, calibrating the position of anatomical structures, measuring and reconstructing surgical instruments, and establishing multi-person collaboration and position sharing (Figure 1). Construction of spinal tumor model: Firstly, with informed consent from the patient and approval from the hospital’s ethics committee, Digital Imaging and Communications in Medicine (DICOM) files were obtained. Since the DICOM files were 2D image files, the creation of the three-dimensional mesh was performed using “3D Slicer” software, which can be considered as reverse engineering. Subsequently, the model was refined and reconstructed using “3Ds Max” software; Secondly, we have taken the following steps to optimize the mesh: (1) Retopology: The mesh topology was optimized; (2) Manual simplification: Unnecessary meshes were removed, and structures with poor display quality were optimized; (3) Structural adjustment: Manually drawn models can have deviations, similar to mechanical assembly errors. Using commands like lattice deformation, details were smoothly adjusted to ensure complete integration of the lumbar vertebrae; (4) Boolean operations: Overlapping parts of two models were removed. For example, independently drawn spinal tumor and vertebral body are separate parts and will overlap when combined. Using Boolean algorithms, appropriate spaces were cut from the vertebral body to accommodate the spinal tumor. Subsequently, we used Unity program to create the AR environment and implement the communication functionality through code, then finally deployed it to HoloLens 2 for each student to experience after multiple debugging. We reconstructed surgical instruments for percutaneous vertebroplasty surgery, includeing drapes, anesthesia syringe, needle, puncture cannula, drill, sampler, bone cement, push rod, gauze, etc. The tasks of the teaching groups were to help students master the anatomical structure of the spine, spinal tumor, and learn the PVP surgical procedure. The above models all have natural interaction functions such as finger dragging, zooming, and tapping, for students to simulate and operate. In addition, the program has a visual projection function, which allows for viewing of spinal tumor lesions and surrounding structures under the guidance of teachers, as well as demonstrating the surgical process. The perspective mode is akin to viewing a real human body, where internal organs and bone structures are not visible beneath the skin. When inserting medical instruments into the human model, students rely on experience and medical imaging references. However, the perspective mode is different. It assists students by providing a three-dimensional perspective view, allowing them to clearly and intuitively see through the skin to observe the vertebral structure and spinal tumor. Through close collaboration, researchers presented spinal tumor and percutaneous vertebroplasty by using augmented reality (Figures 2, 3).
Student grouping
To further confirm the application effect and feasibility of AR technology in teaching spinal tumor’s anatomy and surgery, 8-year program medical students were selected from Peking Union Medical College and Tsinghua University who met the inclusion criteria in the 8-year clinical medicine program as research subjects. The inclusion criteria for this study are as follows: students who have studied for a minimum of 5 years, and have not previously undergone any spinal tumor surgery or virtual reality/augmented reality-assisted surgery. Exclusion criteria: students who could not complete the 2-h teaching plan on time, could not participate in post-teaching evaluations, were in a grade of fewer than 5 years, had previously participated in spinal tumor surgery, or received surgical simulation training.
Theoretical teaching
The teacher employs a uniform teaching curriculum to instruct involved students on fundamental theories and anatomical structures of spinal tumor. This encompasses topics such as spinal anatomy, spinal tumor anatomy, prevalent disorders associated with spinal tumors, spinal tumor metastasis, standard surgical procedures, surgical indications and contraindications, details for percutaneous vertebroplasty, and other specialized knowledge. Following the completion of the instruction, a group simulation lesson will be carried out for all students in two groups.
Group simulation operation teaching
The students included in this study will be randomly divided into a traditional teaching group (Group A, using traditional teaching methods in the simulation lesson) and a AR-assisted teaching group (Group B, using head-worn HoloLens 2 holographic glasses for AR teaching in the simulation lesson). The same senior physician will teach the two groups of students, and the teaching staff will have the same class hours, courseware, basic knowledge explanation, and model teaching aids application, except for AR-related equipment. The teacher in the traditional teaching group (Group A) explained all same procedures using 3D-printed lumbar spine anatomy models, PVP surgical tools, and solid human anatomy models. The students in group B experienced the spinal tumor instance model and PVP surgical operation process using augmented reality technology and head-worn HoloLens holographic glasses. As they go through a spinal tumor instance model, students can change the view from perspective to non-perspective, remove, enlarge, and decrease the vertebrae and attachments of each segment. The layout and structure of the spinal trabeculae, as well as the location and invasive features of spinal tumors, can be intuitively felt by students when they switch to perspective mode. Based on their learning objectives, students can control their learning process, rewatch crucial steps, and switch to non-perspective or perspective modes during the PVP surgical simulation demonstration.
Investigation and evaluation after teaching
When the regular academic offerings is over, students complete a survey comparing their levels of initiative, interaction, course outcomes, satisfaction, and information retention to see how the two groups fared. Using a step-by-step score evaluation approach, the questionnaire options are all quantified using scores with a range of 1–10 points or 1–5 points. At the same time, students in both groups were asked to fill out subjective questionnaires outlining their ideas for how the teaching techniques could be improved. After the completion of the regular academic offerings and the completion of the first survey questionnaire, all students in group A received augmented reality teaching (Group C). After completing all learning content, students in group C received the second survey. Two orthopedic experts reviewed the data and findings from the survey and used statistical methods to conclude. The flowchart of this study is shown in Figure 4.
Statistical analysis
Original data were analyzed using MedCalc 15.2.2 software (MedCalc, Ostend, Belgium). To check if the data follows a normal distribution, the Shapiro–Wilk test was applied. However, considering the particularity of questionnaire surveys, this study used non-parametric tests. The Mann–Whitney independent sample test was used to verify the correlation between the traditional teaching group (Group A) and augmented reality-assisted teaching group (Group B), and the data are described as the median and 25 and 75th interquartile ranges (Q25, Q75). U value, p value, and r values have been reported. The data are described as the median and 25 and 75th interquartile ranges (Q25, Q75); The effect size (ES) was calculated by dividing the Z-score by the square root of n: 0.20–0.49 is considered a small effect; 0.50–0.79 is considered a medium effect; and 0.80 and above are considered a large effect (15, 16). The Wilcoxon rank-sum test was used to verify the correlation between the traditional teaching group (Group A) and continuing AR teaching in the traditional group (Group C), and the data are described as the median and 25 and 75th interquartile ranges (Q25, Q75). Z-value and p values have been reported. p < 0.05 was identified as statistically significant. The Wilcoxon rank-sum test were created using SPSS 23.0 (IBM, Armonk, NY, United States). Figures were created using GraphPad Prism 10.0 (GraphPad Software, San Diego, CA, United States).
Results
Basic information between groups
The traditional teaching group included 13 students, four males and nine females, who were all eighth-year clinical medical students in fifth grade or sixth grade. The average age of the participants in the traditional teaching group is 23.5 ± 0.9 years old. Twelve medical students (seven males and five females) from fifth grade or sixth grade in the augmented reality technology teaching group served as comparisons. The average age of the students in the augmented reality assisted teaching group is 23.7 ± 1.3 years old. In terms of age, stage of cultivation, etc., no statistically significant difference was found between the two groups. According to the research requirements, all participants fulfilled the inclusion criteria and finished the course, questionnaires, and post-class feedback assessments.
Differences in teaching effectiveness in different groups
During this study, all students were taught theoretical concepts using slides and models of the human body. The augmented reality group then had percutaneous vertebroplasty surgical operation simulations and instruction using augmented reality models after receiving traditional instruction (Figures 5, 6). Teaching quality, students’ learning interest, anatomical structure mastery, and knowledge of percutaneous vertebroplasty were all significantly different between group A and group B, according to the results of the post-class evaluations. There were notable benefits (p < 0.05) for the group that was taught using augmented reality technology (Table 1). In addition, after finishing the regular academic offerings and the first teaching scenario survey, every participant in the traditional teaching group was also allowed to learn by AR simulation technology. A second survey with questions was administered following the completion of all training in the traditional teaching group (Group C). After implementing AR into the traditional teaching group, students demonstrated greater engagement in learning, enhanced comprehension of anatomical structures, and higher ratings of the overall quality of instruction (Table 2). Along with their proficiency in percutaneous vertebroplasty, students demonstrated considerable enhancements in their comprehension of the patterns of growth and spread of spinal malignancies (Table 2). The box plots were used to display the distribution among three groups (Figure 7).
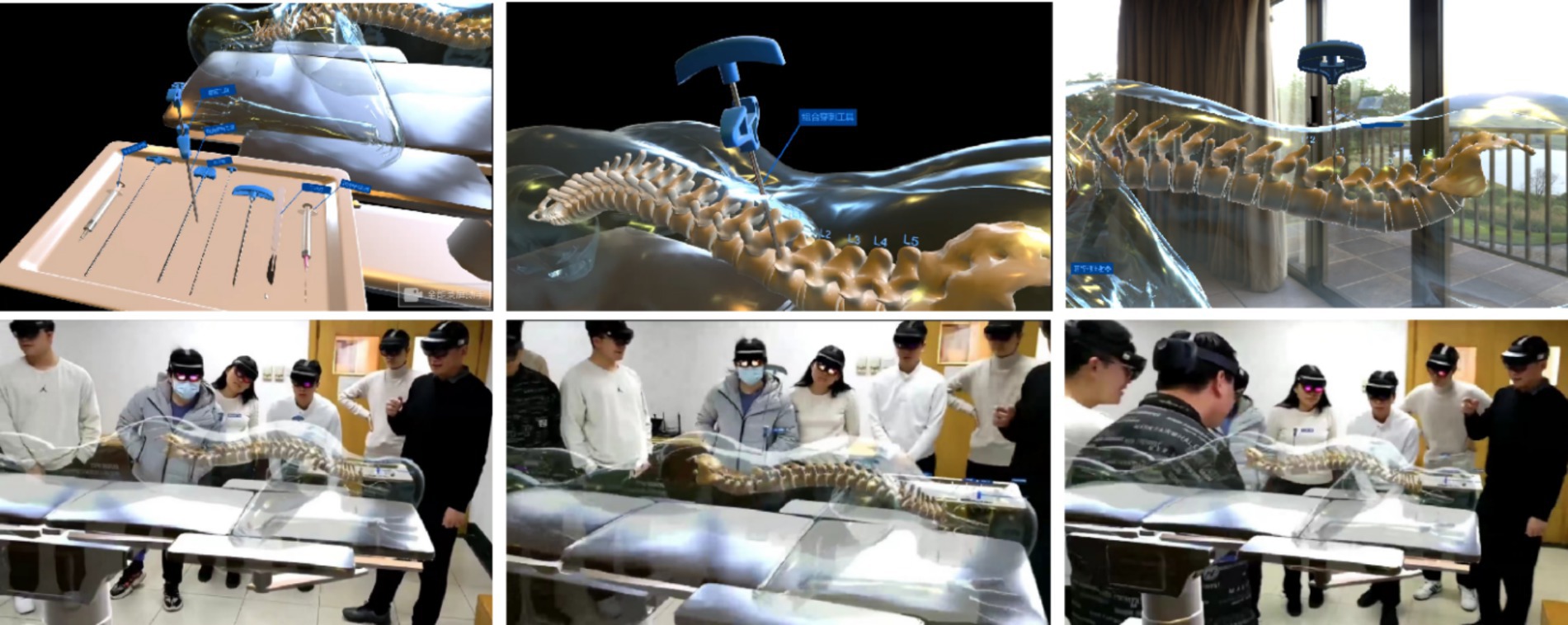
Figure 6. Augmented reality technology displaying the surgical process of percutaneous vertebroplasty (PVP).
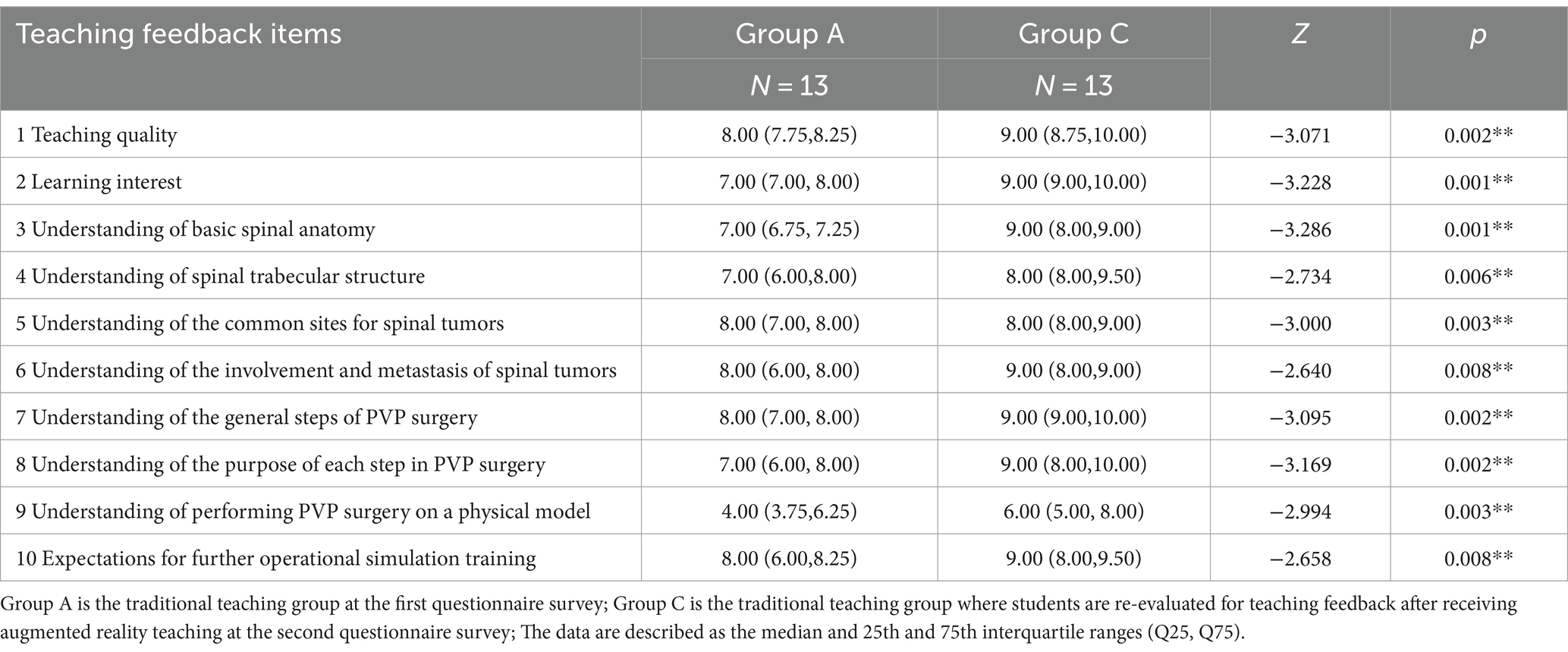
Table 2. Comparison of course evaluations of students in the traditional teaching group before and after receiving augmented reality technology teaching.
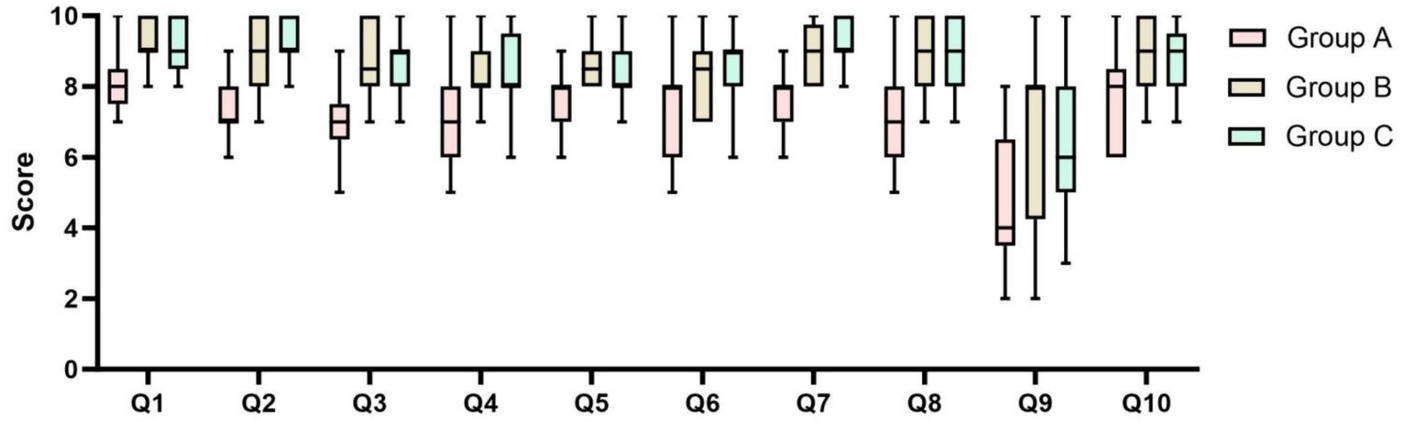
Figure 7. The box plots displaying the distribution differences among three groups. Plot: min to max; Q: Question; Q1: Teaching quality; Q2: Learning interest; Q3: Understanding of spinal anatomy; Q4: Understanding of spinal trabecular structure; Q5: Understanding of the common sites for spinal tumors; Q6: Understanding of the involvement and metastasis of spinal tumors; Q7: Understanding of the general steps of PVP surgery; Q8: Understanding of the purpose of each step in PVP surgery; Q9: Capability of performing PVP surgery on a physical model; Q10: Expectation for further hands-on simulation training.
Opinions and feasible improvement measures proposed by students
According to the study above, the researchers collected the identified issues, methods for enhancement, and the teaching pros and cons for each set of test students by utilizing subjective feedback during the teaching process (Table 3). The survey questionnaires for each group are listed as attachment forms (Supplementary materials 1, 2). The findings indicated that using AR holographic glasses might lead to a higher incidence of discomfort among students, such as dizziness, while initially using them, primarily due to the weight of the helmet and the three-dimensional image. However, after making the required adjustments, discomfort can be quickly relieved.
Discussion
Application of AR in medical education
Virtual reality (VR) technology has been instrumental in enhancing psychological skill training in high-performance professions, especially those that require a high level of safety awareness and complex skills (17). VR can efficiently train and enhance psychological skills by creating a safe, repeatable, and fully controllable training environment. In high-pressure and high-performance environments, the VR technology offers various innovative solutions for the training and learning of complex skills, which are often expensive or dangerous to practice in reality (18, 19). Extended reality (XR) is an advanced version and expansion of VR technology, and the utilization of XR in orthopedics and medical education is gaining popularity. AR technology emerges from integrating human visual exploration and interaction with computer-generated 3D worlds, which are then overlaid onto the real-world environment, incorporating virtual circumstances (20). In medical education, AR tools allow for anatomical simulation, evaluation, diagnosis, and surgical teaching and training through realistic surgical simulations (21). In recent years, the teaching attempts and applications of AR technology in undergraduate, graduate, and junior resident physicians have received immense attention (22).
Through the VR/AR technology, realistic operating environments can be simulated, enabling trainees to improve key skills in virtual contexts, such as attention, decision-making ability, situational awareness, adaptability, and stress management (18). Such signals can be monitored and evaluated by analyzing physiological parameters such as heart rate, respiratory rate, and electroencephalogram (EEG) (19, 23). By wearing AR glasses, students and teachers can learn about the anatomy, imaging knowledge, and surgical approach selection of relevant cases in space of virtual and real fusion. Applying this teaching method allows for a more intuitive and clear display of the anatomical structure of limbs, joints, and spine. Küçük et al. (24) found that the use of AR technology to learn anatomical knowledge has an impact on the academic performance and cognitive aspects of medical students. Lopes et al. (25) divided 20 medical students without any experience in basic surgical skills into an AR telestration teaching group and a traditional teaching group, and then evaluated the students’ learning experience, self-confidence, and self-assessment through questionnaires. Through result analysis, it was found that using helmet devices with AR functions can replace traditional teaching methods in terms of the quality of the surgical gesture, tension of the suture, self-evaluation or confidence. In addition, Nagayo et al. (26) established a self-training system that provides 3D information through AR technology. There was no significant difference in the improvement of test scores between the AR group and the video group, however, the actions provided by the AR system were more helpful in manipulating surgical instruments than videos (p = 0.02). Students can quickly grasp and apply knowledge points such as complex fracture, joint disorders, and spinal diseases. Meanwhile, it also frees medical education from the limitations of traditional teaching venues (22).
Application of AR in teaching spinal tumor
Surgery for tumor in bone and soft tissue is a specialized area that combines the expertise of orthopedics and oncology. Subspecialty teaching encounters numerous hurdles, including intricate anatomical underpinnings, a wide range of disease classifications, and lengthy training periods for young physicians. Although the application of VR/AR/MR technology in orthopedic specialties such as spine, joints, and trauma has been on the rise (27, 28), few studies have explored the application of VR/AR/MR technology in bone and soft tissue tumors (Table 4). The management of bone tumors requires long-term and continuous clinical learning and practical operation. Therefore, the VR/AR/MR technology may provide simulated clinical teaching incorporating hearing, vision, and touch elements, enabling students to participate in clinical practice under various scenarios. It also facilitates real-time guidance from teachers, increases teacher-student interaction, and improves teaching efficiency.
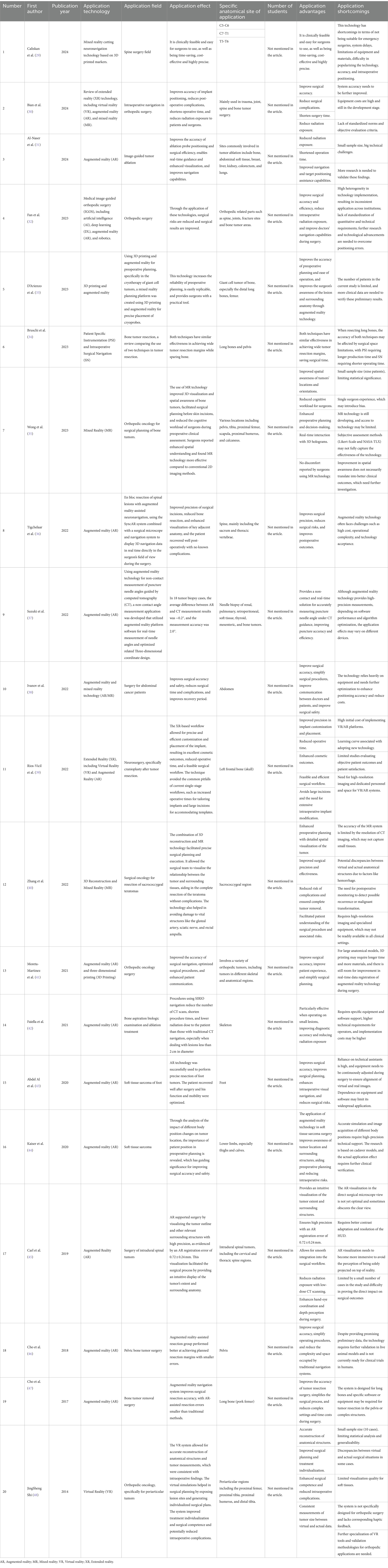
Table 4. Summary of previous literature on the application of AR/MR/VR/XR technology in bone and soft tissue tumors.
Spinal tumor surgery poses significant challenges and risks, particularly in cases of malignant tumors, when determining the tumor boundaries is typically challenging. The surgical procedure is challenging and demands exceptional surgical expertise from the operator. In this context, spinal tumors, as one of the most difficult bone tumor diseases in orthopedics, have the characteristics of difficult-to-grasp anatomical structures, difficult-to-understand tumor invasion characteristics, and long surgical learning curves. As an innovative teaching method, AR technology has rapidly developed with unique advantages such as immersion, interactivity, and accuracy, helping to promote the transformation and progress of teaching models in bone and soft tissue tumor surgery. To assure surgical safety, AR technology can visualize crucial anatomical structures, including main blood arteries, nerves, spinal cord, and other vital tissues. The time needed for surgical procedures can be significantly decreased by projecting these rebuilt anatomical structures onto the patient (47). Under such circumstances, this study explores the training effectiveness of applying augmented reality technology in simulated teaching of spinal tumors.
Cho et al. was the first to report the application of AR-assisted navigation technology in surgical resection of pig femoral and pelvic tumors, laying the foundation for the application of AR technology in the field of bone and soft tissue tumors (46, 47). Subsequently, Carl et al. (45) and Tigchelaar et al. (36) applied AR technology to the surgical treatment of spinal intradural tumor and spinal epidural tumor, respectively. However, although there are many literature reports, the expansion and attempts of AR-assisted teaching methods for medical education are especially lacking. In this context, our team developed an AR-assisted spinal tumor-teaching model and surgical teaching system for medical students.
The main contribution of this study
This study was the first reported attempt to apply AR technology in medical teaching, using spinal tumors and percutaneous vertebroplasty as examples, head-mounted augmented reality devices were used by students to create specific learning scenarios, presenting the complex three-dimensional spatial structure intuitively. After further data analysis, we found that the AR-assisted teaching group had better teaching quality in learning interest, understanding of spinal analysis, understanding of spinal trabecular structure, understanding of the common sites for spinal tubers, and understanding of the general steps of PVP surgery, compared to the traditional teaching group. In addition, after receiving AR-assisted teaching in traditional teaching group, significant improvements had been found in teaching quality, learning interest, understanding of basic spinal anatomy, understanding of spinal trabecular structure, understanding of the common sites for spinal tumors, understanding of the involvement and metathesis of spinal tumors, understanding of the general steps of PVP surgery, and expectations for further operational simulation training. The above research results confirmed the teaching significance of the spinal tumor model constructed in this study and the demonstration model of percutaneous vertebroplasty surgery, as well as the important value of augmented reality technology in assisting spinal tumor teaching. The research results of this study will lay a theoretical and practical foundation for the application of AR-assisted spinal tumor anatomy teaching and preclinical teaching, as well as the improvement of medical education level.
Limitations and future plans
Spinal tumors are a difficult point for medical students or young physicians during learning, most of them require prolonged learning period to master. The use of AR technology to simulate spinal tumor models and interactive surgical scenarios needs to be further verified in the medical preparatory stage or young physicians. For this purpose, our research team independently designed a spinal tumor model and a percutaneous vertebroplasty surgery procedure that can be used to achieve interactive teaching. Although the number of medical students included in the two groups was not high, statistically significant differences were observed between multiple indicators among groups. Future research will focus on expanding the participant pool, exploring diverse teaching methods and scenarios, and incorporating objective skill assessments (e.g., surgical operation simulations) with scoring systems. As the number of students increases, more teaching data will be accumulated for more in-depth analysis of multiple factors such as gender, age, student type, and major. In terms of device improvement, students will have more opportunities for interactive operations to enhance their clinical operational skills. These advancements will further validate the feasibility of AR as a valuable tool for modern orthopedic and surgical oncology education.
Machine learning and artificial intelligence technology can be applied in psychological skill assessment and training, and the combination of the two is expected to further improve the quality of medical education (18, 23). For example, using machine learning algorithms, patterns can be extracted from a large amount of physiological and behavioral data to evaluate individual performance and progress at different training stages (23). The technologies can also be used to establish adaptive learning systems that dynamically adjust training content and difficulty based on real-time feedback from trainees, thereby improving training effectiveness. In addition, artificial intelligence can create virtual coaches and intelligent decision support systems, which may improve the experience of trainees in virtual reality learning environments (49, 50). Thus, the promotion and improvement of AR technology in medical teaching need to accumulate more practical data and still face many challenges (51, 52).
Conclusion
In summary, AR technology, which combines reality and virtual worlds, has great potential in applying medical education. For teaching spinal tumors, it can effectively compensate for the shortcomings of traditional teaching models, make medical education more precise, efficient, and intuitive, and gradually promote the reform of medical education models. In clinical teaching of spinal tumors, AR-assisted teaching is an optimization method that has better teaching effectiveness and can be further explored in the future.
Data availability statement
The original contributions presented in the study are included in the article/Supplementary material; further inquiries can be directed to the corresponding authors.
Ethics statement
The ethical approval of this study was evaluated and waived by the Ethics Committee of Peking Union Medical College Hospital (No. I-24ZM0019). The authors confirm that all methods were carried out in accordance with relevant guidelines and regulations. Written informed consent was obtained from all subjects involved in this study.
Author contributions
SLiu: Conceptualization, Formal analysis, Funding acquisition, Investigation, Methodology, Resources, Software, Writing – original draft, Writing – review & editing. JY: Conceptualization, Formal analysis, Investigation, Methodology, Project administration, Resources, Software, Writing – original draft, Writing – review & editing. HJ: Conceptualization, Formal analysis, Methodology, Resources, Software, Writing – original draft. AL: Data curation, Investigation, Software, Writing – original draft. QZ: Conceptualization, Methodology, Resources, Software, Writing – original draft. JX: Data curation, Investigation, Software, Writing – original draft. YL: Conceptualization, Formal analysis, Funding acquisition, Investigation, Methodology, Project administration, Resources, Software, Supervision, Writing – original draft, Writing – review & editing. SLi: Conceptualization, Formal analysis, Investigation, Methodology, Project administration, Resources, Software, Supervision, Writing – original draft, Writing – review & editing.
Funding
The author(s) declare that financial support was received for the research, authorship, and/or publication of this article. This study was supported by the first batch of Young Scholars Support Program of Peking Union Medical College (Project No. 2022002; Grant recipient: SLiu), and Undergraduate Education and Teaching Reform Project of Peking Union Medical College (Project No. 2021zlgc0126; Grant recipient: YL).
Acknowledgments
The authors would like to thank all the reviewers who participated in the review, as well as MJEditor (www.mjeditor.com) for providing English editing services during the preparation of this manuscript. We would like to thank our colleagues at the Departments of Orthopedic Surgery, Fundamental Industry Training Center of Tsinghua University for their support.
Conflict of interest
The authors declare that the research was conducted in the absence of any commercial or financial relationships that could be construed as a potential conflict of interest.
Publisher’s note
All claims expressed in this article are solely those of the authors and do not necessarily represent those of their affiliated organizations, or those of the publisher, the editors and the reviewers. Any product that may be evaluated in this article, or claim that may be made by its manufacturer, is not guaranteed or endorsed by the publisher.
Supplementary material
The Supplementary material for this article can be found online at: https://www.frontiersin.org/articles/10.3389/fmed.2024.1403423/full#supplementary-material
References
1. Taghian, A, Abo-Zahhad, M, Sayed, MS, and Abd El-Malek, AH. Virtual and augmented reality in biomedical engineering. Biomed Eng Online. (2023) 22:76. doi: 10.1186/s12938-023-01138-3
2. Zhang, J, Lu, V, and Khanduja, V. The impact of extended reality on surgery: a scoping review. Int Orthop. (2023) 47:611–21. doi: 10.1007/s00264-022-05663-z
3. Hey, G, Guyot, M, Carter, A, and Lucke-Wold, B. Augmented reality in neurosurgery: a new paradigm for training. Medicina. (2023) 59:1721. doi: 10.3390/medicina59101721
4. Ghenbot, Y, Ahmad, HS, Chauhan, D, Wathen, C, Arena, J, Turlip, R, et al. Effects of augmented reality on thoracolumbar pedicle screw instrumentation across different levels of surgical experience. World Neurosurg. (2023) 182:e284–91. doi: 10.1016/j.wneu.2023.11.100
5. Shaikh, HJF, Hasan, SS, Woo, JJ, Lavoie-Gagne, O, Long, WJ, and Ramkumar, PN. Exposure to extended reality and artificial intelligence-based manifestations: a primer on the future of hip and knee arthroplasty. J Arthroplast. (2023) 38:2096–104. doi: 10.1016/j.arth.2023.05.015
6. Gomindes, AR, Adeeko, ES, Khatri, C, Ahmed, I, Sehdev, S, Carlos, WJ, et al. Use of virtual reality in the education of Orthopaedic procedures: a randomised control study in early validation of a novel virtual reality simulator. Cureus. (2023) 15:e45943. doi: 10.7759/cureus.45943
7. Jung, Y, Muddaluru, V, Gandhi, P, Pahuta, M, and Guha, D. The development and applications of augmented and virtual reality technology in spine surgery training: a systematic review. Can J Neurol Sci. (2023) 51:255–64. doi: 10.1017/cjn.2023.46
8. Shahzad, H, Bhatti, NS, Phillips, FM, and Khan, SN. Applications of augmented reality in Orthopaedic spine surgery. J Am Acad Orthop Surg. (2023) 31:e601–9. doi: 10.5435/JAAOS-D-23-00023
9. Takatsume, Y, Nakase, J, Oshima, T, Kanayama, T, Imanishi, N, and Tsuchiya, H. Development of an educational three-dimensional anatomical structure replication tool and its application to medial open-wedge high tibial osteotomy. Knee. (2023) 44:150–7. doi: 10.1016/j.knee.2023.08.005
10. Stetson, WB, Polinsky, S, Dilbeck, S, and Chung, BC. The use of Telesurgery mentoring and augmented reality to teach arthroscopy. Arthrosc Tech. (2022) 11:e203–7. doi: 10.1016/j.eats.2021.10.008
11. Pierzchajlo, N, Stevenson, TC, Huynh, H, Nguyen, J, Boatright, S, Arya, P, et al. Augmented reality in minimally invasive spinal surgery: a narrative review of available technology. World Neurosurg. (2023) 176:35–42. doi: 10.1016/j.wneu.2023.04.030
12. Cannizzaro, D, Zaed, I, Safa, A, Jelmoni, AJM, Composto, A, Bisoglio, A, et al. Augmented reality in neurosurgery, state of art and future projections. Front Surg. (2022) 9:864792. doi: 10.3389/fsurg.2022.864792
13. Cofano, F, Di Perna, G, Zeppa, P, Lanotte, M, and Garbossa, D. Letter: improving surgical skills during residency: a scheduled and certified approach with virtual-augmented reality and life-like simulators: experience in a single School of Neurosurgery. Neurosurgery. (2022) 91:e71–3. doi: 10.1227/neu.0000000000002055
14. Kandasamy, G, Bettany-Saltikov, J, Cordry, J, and McSherry, R. Use of vision-based augmented reality to improve student learning of the spine and spinal deformities. An exploratory study. S Afr J Physiother. (2021) 77:1579. doi: 10.4102/sajp.v77i2.1579
15. Portney, LG, and Watkins, MP. Foundations of Clinical Research: Applications to Practice. 3rd ed. Upper Saddle River: Pearson Education, Inc. (2009).
16. Karadimitriou, SM, and Marshall, E. Mann-Whitney in SPSS. (2021). Available at: https://www.sheffield.ac.uk/polopoly_fs/1.714552!/file/stcp-marshall-MannWhitS.pdf (Accessed July 19, 2021).
17. Richlan, F, Weiß, M, Kastner, P, and Braid, J. Virtual training, real effects: a narrative review on sports performance enhancement through interventions in virtual reality. Front Psychol. (2023) 14:1240790. doi: 10.3389/fpsyg.2023.1240790
18. Kluge, MG, Maltby, S, Kuhne, C, Walker, N, Bennett, N, Aidman, E, et al. Evaluation of a virtual reality platform to train stress management skills for a defense workforce: multisite, mixed methods feasibility study. J Med Internet Res. (2023) 25:e46368. doi: 10.2196/46368
19. Aidman, E . Cognitive fitness framework: towards assessing, training and augmenting individual-difference factors underpinning high-performance cognition. Front Hum Neurosci. (2020) 13:466. doi: 10.3389/fnhum.2019.00466
20. Morimoto, T, Kobayashi, T, Hirata, H, Otani, K, Sugimoto, M, Tsukamoto, M, et al. XR (extended reality: virtual reality, augmented reality, mixed reality) Technology in Spine Medicine: status quo and quo Vadis. J Clin Med. (2022) 11:470. doi: 10.3390/jcm11020470
21. Co, M, Chiu, S, and Billy Cheung, HH. Extended reality in surgical education: a systematic review. Surgery. (2023) 174:1175–83. doi: 10.1016/j.surg.2023.07.015
22. Arjomandi Rad, A, Subbiah Ponniah, H, Shah, V, Nanchahal, S, Vardanyan, R, Miller, G, et al. Leading transformation in medical education through extended reality. Adv Exp Med Biol. (2023) 1421:161–73. doi: 10.1007/978-3-031-30379-1_7
23. Maltby, S, Garcia-Esperon, C, Jackson, K, Butcher, K, Evans, JW, O'Brien, W, et al. TACTICS VR stroke telehealth virtual reality training for health care professionals involved in stroke Management at Telestroke Spoke Hospitals: module design and implementation study. JMIR Serious Games. (2023) 11:e43416. doi: 10.2196/43416
24. Küçük, S, Kapakin, S, and Göktaş, Y. Learning anatomy via mobile augmented reality: effects on achievement and cognitive load. Anat Sci Educ. (2016) 9:411–21. doi: 10.1002/ase.1603
25. Neves Lopes, V, Dantas, I, Barbosa, JP, and Barbosa, J. Telestration in the teaching of basic surgical skills: a randomized trial. J Surg Educ. (2022) 79:1031–42. doi: 10.1016/j.jsurg.2022.02.013
26. Nagayo, Y, Saito, T, and Oyama, H. Augmented reality self-training system for suturing in open surgery: a randomized controlled trial. Int J Surg. (2022) 102:106650. doi: 10.1016/j.ijsu.2022.106650
27. Lohre, R, Wang, JC, Lewandrowski, KU, and Goel, DP. Virtual reality in spinal endoscopy: a paradigm shift in education to support spine surgeons. J Spine Surg. (2020) 6:S208–23. doi: 10.21037/jss.2019.11.16
28. Sumdani, H, Aguilar-Salinas, P, Avila, MJ, Barber, SR, and Dumont, T. Utility of augmented reality and virtual reality in spine surgery: a systematic review of the literature. World Neurosurg. (2022) 161:e8–e17. doi: 10.1016/j.wneu.2021.08.002
29. Caliskan, KE, Yavas, G, and Cagli, MS. Intraoperative mixed-reality spinal neuronavigation system: a novel navigation technique for spinal intradural pathologies. Neurosurg Focus. (2024) 56:E2. doi: 10.3171/2023.10.FOCUS23624
30. Bian, D, Lin, Z, Lu, H, Zhong, Q, Wang, K, Tang, X, et al. The application of extended reality technology-assisted intraoperative navigation in orthopedic surgery. Front Surg. (2024) 11:1336703. doi: 10.3389/fsurg.2024.1336703
31. Al-Naser, Y, Halka, F, Alshadeedi, F, Albahhar, M, and Athreya, S. The applications of augmented reality in image-guided tumor ablations: a scoping review. J Med Imag Radiat Sci. (2024) 55:125–33. doi: 10.1016/j.jmir.2023.12.006
32. Fan, X, Zhu, Q, Tu, P, Joskowicz, L, and Chen, X. A review of advances in image-guided orthopedic surgery. Phys Med Biol. (2023) 68:02TR01. doi: 10.1088/1361-6560/acaae9
33. D'Arienzo, A, Scognamiglio, B, Campo, FR, Cosseddu, F, Ruinato, DA, Ipponi, E, et al. Preliminary results of preoperative planning using 3D printing and augmented reality in cryotherapy treatment of Giant cell tumor of bone-CRIO2AR project. Healthcare (Basel). (2023) 11:2629. doi: 10.3390/healthcare11192629
34. Bruschi, A, Donati, DM, and Di Bella, C. What to choose in bone tumour resections? Patient specific instrumentation versus surgical navigation: a systematic review. J Bone Oncol. (2023) 42:100503. doi: 10.1016/j.jbo.2023.100503
35. Wong, KC, Sun, EY, Wong, IOL, and Kumta, SM. Mixed reality improves 3D visualization and spatial awareness of bone tumors for surgical planning in Orthopaedic oncology: a proof of concept study. Orthop Res Rev. (2023) 15:139–49. doi: 10.2147/ORR.S421077
36. Tigchelaar, SS, Medress, ZA, Quon, J, Dang, P, Barbery, D, Bobrow, A, et al. Augmented reality Neuronavigation for En bloc resection of spinal column lesions. World Neurosurg. (2022) 167:102–10. doi: 10.1016/j.wneu.2022.08.143
37. Suzuki, K, Morita, S, Endo, K, Yamamoto, T, and Sakai, S. Noncontact measurement of puncture needle angle using augmented reality technology in computed tomography-guided biopsy: stereotactic coordinate design and accuracy evaluation. Int J Comput Assist Radiol Surg. (2022) 17:745–50. doi: 10.1007/s11548-022-02572-9
38. Ivanov, VM, Krivtsov, AM, Strelkov, SV, Smirnov, AY, Shipov, RY, Grebenkov, VG, et al. Practical application of augmented/mixed reality technologies in surgery of abdominal cancer patients. J Imag. (2022) 8:183. doi: 10.3390/jimaging8070183
39. Rios-Vicil, CI, Barbery, D, Dang, P, and Jean, WC. Single-stage cranioplasty with customized polyetheretherketone implant after tumor resection using virtual reality and augmented reality for precise implant customization and placement: illustrative case. J Neurosurg Case Lessons. (2022) 3:CASE2255. doi: 10.3171/CASE2255
40. Zhang, H, Ji, L, Liu, J, Li, S, Chen, T, Li, J, et al. Case report: resection of a massive primary sacrococcygeal mature teratoma in an adult using 3-dimensional reconstruction and mixed reality technology. Front Surg. (2022) 9:948388. doi: 10.3389/fsurg.2022.948388
41. Moreta-Martinez, R, Pose-Díez-de-la-Lastra, A, Calvo-Haro, JA, Mediavilla-Santos, L, Pérez-Mañanes, R, and Pascau, J. Combining augmented reality and 3D printing to improve surgical workflows in orthopedic oncology: smartphone application and clinical evaluation. Sensors. (2021) 21:1370. doi: 10.3390/s21041370
42. Faiella, E, Castiello, G, Bernetti, C, Pacella, G, Altomare, C, Andresciani, F, et al. Impact of an augmented reality navigation system (SIRIO) on bone percutaneous procedures: a comparative analysis with standard CT-guided technique. Curr Oncol. (2021) 28:1751–60. doi: 10.3390/curroncol28030163
43. Abdel Al, S, Chaar, MKA, Mustafa, A, Al-Hussaini, M, Barakat, F, and Asha, W. Innovative surgical planning in resecting soft tissue sarcoma of the foot using augmented reality with a smartphone. J Foot Ankle Surg. (2020) 59:1092–7. doi: 10.1053/j.jfas.2020.03.011
44. Kaiser, D, Hoch, A, Kriechling, P, Graf, DN, Waibel, FWA, Gerber, C, et al. The influence of different patient positions on the preoperative 3D planning for surgical resection of soft tissue sarcoma in the lower limb-a cadaver pilot study. Surg Oncol. (2020) 35:478–83. doi: 10.1016/j.suronc.2020.10.008
45. Carl, B, Bopp, M, Saß, B, Pojskic, M, and Nimsky, C. Augmented reality in intradural spinal tumor surgery. Acta Neurochir. (2019) 161:2181–93. doi: 10.1007/s00701-019-04005-0
46. Cho, HS, Park, MS, Gupta, S, Han, I, Kim, HS, Choi, H, et al. Can augmented reality be helpful in pelvic bone Cancer surgery? An in vitro study. Clin Orthop Relat Res. (2018) 476:1719–25. doi: 10.1007/s11999.0000000000000233
47. Cho, HS, Park, YK, Gupta, S, Yoon, C, Han, I, Kim, HS, et al. Augmented reality in bone tumour resection: an experimental study. Bone Joint Res. (2017) 6:137–43. doi: 10.1302/2046-3758.63.BJR-2016-0289.R1
48. Shi, J, Xia, J, Wei, Y, Wang, S, Wu, J, Chen, F, et al. Three-dimensional virtual reality simulation of periarticular tumors using Dextroscope reconstruction and simulated surgery: a preliminary 10 case study. Acta Orthop Belg. (2014) 80:132–8.
49. Rossi, SMP, Mancino, F, Sangaletti, R, Perticarini, L, Lucenti, L, and Benazzo, F. Augmented reality in orthopedic surgery and its application in Total joint arthroplasty: a systematic review. Appl Sci. (2022) 12:5278. doi: 10.3390/app12105278
50. Andriollo, L, Picchi, A, Sangaletti, R, Perticarini, L, Rossi, SMP, Logroscino, G, et al. The role of artificial intelligence in anterior cruciate ligament injuries: current concepts and future perspectives. Health. (2024) 12:300. doi: 10.3390/healthcare12030300
51. Godzik, J, Farber, SH, Urakov, T, Steinberger, J, Knipscher, LJ, Ehredt, RB, et al. "disruptive technology" in spine surgery and education: virtual and augmented reality. Oper Neurosurg. (2021) 21:S85–93. doi: 10.1093/ons/opab114
Keywords: augmented reality technology, spinal tumors, anatomy teaching, surgical teaching, teaching evaluation
Citation: Liu S, Yang J, Jin H, Liang A, Zhang Q, Xing J, Liu Y and Li S (2024) Exploration of the application of augmented reality technology for teaching spinal tumor’s anatomy and surgical techniques. Front. Med. 11:1403423. doi: 10.3389/fmed.2024.1403423
Edited by:
Tor Finseth, Honeywell (United States), United StatesReviewed by:
Agnieszka Pregowska, Polish Academy of Sciences, PolandMirza Pojskic, University Hospital of Giessen and Marburg, Germany
Stefano Marco Paolo Rossi, Fondazione Poliambulanza Istituto Ospedaliero, Italy
Copyright © 2024 Liu, Yang, Jin, Liang, Zhang, Xing, Liu and Li. This is an open-access article distributed under the terms of the Creative Commons Attribution License (CC BY). The use, distribution or reproduction in other forums is permitted, provided the original author(s) and the copyright owner(s) are credited and that the original publication in this journal is cited, in accordance with accepted academic practice. No use, distribution or reproduction is permitted which does not comply with these terms.
*Correspondence: Yong Liu, bGl1eW9uZ19wdW1jaEAxNjMuY29t; Shuangshou Li, bHNzQHRzaW5naHVhLmVkdS5jbg==
†These authors have contributed equally to this work