- 1Department of Nuclear Medicine, Candiolo Cancer Institute, FPO – IRCCS, Turin, Italy
- 2Division of Nuclear Medicine, Università degli Studi di Brescia and ASST Spedali Civili di Brescia, Brescia, Italy
- 3Nuclear Medicine Unit, Department of Medical Sciences, AOU Città della Salute e della Scienza, University of Turin, Turin, Italy
- 4Clinic of Medical Oncology, Oncology Institute of Southern Switzerland, Ente Ospedaliero Cantonale, Bellinzona, Switzerland
- 5Unità di Medicina Nucleare, GSTeP Radiopharmacy - TracerGLab, Dipartimento di Diagnostica per Immagini, Radioterapia Oncologica ed Ematologia, Fondazione Policlinico Universitario A. Gemelli, IRCCS, Rome, Italy
- 6Department of Nuclear Medicine, E.O. “Ospedali Galliera,” Genoa, Italy
- 7Clinic of Nuclear Medicine, Imaging Institute of Southern Switzerland, Ente Ospedaliero Cantonale, Bellinzona, Switzerland
- 8Faculty of Biology and Medicine, University of Lausanne, Lausanne, Switzerland
- 9Faculty of Biomedical Sciences, Università della Svizzera Italiana, Lugano, Switzerland
Background: Several recent studies have proposed the possible application of positron emission tomography/computed tomography (PET/CT) administering radiolabelled fibroblast-activation protein (FAP) inhibitors for various forms of thyroid cancer (TC), including differentiated TC (DTC), and medullary TC (MTC).
Methods: The authors conducted an extensive literature search of original studies examining the effectiveness of FAP-guided PET/CT in patients with TC. The papers included were original publications exploring the use of FAP-targeted molecular imaging in restaging metastatic DTC and MTC patients.
Results: A total of 6 studies concerning the diagnostic yield of FAP-targeted PET/CT in TC (274 patients, of which 247 DTC and 27 MTC) were included in this systematic review. The included articles reported high values of FAP-targeted PET/CT detection rates in TC, ranging from 81 to 100% in different anatomical sites and overall superior to the comparative imaging method.
Conclusion: Although there are promising results, the existing literature on the diagnostic accuracy of FAP-guided PET in this context is still quite limited. To thoroughly evaluate its potential significance in TC patients, it is needed to conduct prospective randomized multicentric trials.
1 Introduction
Differentiated thyroid cancer (DTC) is the leading malignant tumour affecting the endocrine system, and its global occurrence continues to increase annually due to the implementation of enhanced screening methods, such as neck ultrasonography and fine needle aspiration biopsy in everyday clinical practice, for detecting and characterising small thyroid nodules (1). Most of these TCs encompass small and asymptomatic papillary TCs (PTCs) belonging to a significant subclinical group of slow-growing tumours (2) and high-risk DTCs in a lower percentage (3). Adjuvant post-surgical Radioiodine (RAI) therapy and relative post-therapeutic whole-body scan performed after RAI administration have traditionally been crucial in assessing the extent of tumour burden in high-risk DTC and the ability of residual or recurrent illness to concentrate RAI (3). Regrettably, only around two-thirds of patients with metastatic DTC exhibit uptake of RAI in their lesions. Conversely, the remainder of patients either develop metastases that do not exhibit significant RAI uptake on post-therapeutic whole-body scan (or lose the ability to concentrate it) or experience disease progression after RAI treatment (3, 4). As a result of this statement, the concept of RAI-refractoriness (RAI-R) was introduced in the literature. According to the latest American and European Thyroid and Nuclear Medicine Societies, RAI-R is defined as follows: patients with abnormal thyroglobulin (Tg) levels or evidence of disease in other diagnostic examinations without RAI concentration on a diagnostic or post-therapeutic RI scan; tumour foci that show RAI uptake while others do not; progressive disease despite evidence of RAI uptake in DTC lesions (3).
Concerning the diagnostic instrumental investigations currently employed to manage RAI-R DTC patients, whole-body contrast-enhanced computed tomography (CT) is the most diffused examination due to its ability to assess the development of locally recurrent invasive disease, lymph node and distant metastases as well as its worldwide availability and cost-effectiveness (5). However, several authors have observed how fluorine-18 fluorodeoxyglucose ([18F]FDG) positron emission tomography (PET)/CT may play a role in the management of these patients, particularly as a prognosis predictor, despite its variable accuracy, which is usually affected by different pathology features such as tumour dedifferentiation and burden (3, 6, 7).
Among the variety of tumours which can onset in the context of thyroid gland, it does worth mention the medullary thyroid cancer (MTC), which is a rare neuroendocrine neoplasm originating from the para-follicular C-cells of the thyroid and encompasses for 3 to 10% of all TCs (8–10). Calcitonin is the most commonly used serum marker for screening and monitoring patients diagnosed with MTC (11). When MTC is detected, the metastatic spread to the cervical lymph nodes is a frequent condition (12). Regarding MTC, CT is the primary cross-sectional imaging technique used to evaluate the severity of the disease in individuals with elevated calcitonin levels, particularly for lymph nodes and liver metastases (although magnetic resonance imaging is more effective in detecting bone lesions) (13, 14). Regarding the molecular imaging of MTC, PET/CT is a highly beneficial nuclear medicine technology in comparison to conventional scintigraphic techniques. The PET radiopharmaceuticals most typically utilized in MTC restaging (when blood tumour marker levels rise) are [18F] dihydroxyphenylalanine ([18F]DOPA), [18F]FDG and [68Ga]Ga-labelled somatostatin analogs and, among them, [18F]DOPA has the best detection rate (15, 16).
Overall, there is an urgent need of more accurate molecular imaging methods focused on new targets for evaluating RAI-R DTC and MTC.
For several decades, carcinomas were thought to be made up of altered cells with cell-autonomous hyper-proliferative and invasive survival features. Nevertheless, the tumour microenvironment (TME), which includes tumour-associated stromal cells and the extracellular matrix, is also crucial in tumour invasion and metastases onset, promoting cell migration (17–19); for example, cancer-associated fibroblasts (CAFs) are a significant component of the tumour stroma. CAFs, also known as reactive fibroblasts or myofibroblasts, are found in a variety of malignant tumours, including head and neck malignancies other than TC (20, 21). FAP expression may also variate according to the solid tumours’ grading, such as in prostate cancer (22, 23). Despite CAFs being stromal cells, which are part and parcel of neoplastic lesions, they do not express epithelial, endothelial, or leukocyte markers and are notably devoid of oncogene mutations (24). Finally, CAFs have been found to express a variety of receptors on their cell membrane, including alpha-smooth muscle actin and fibroblast-activating protein (FAP) (25). FAP expression is typically slight in physiologic adult tissues but significantly higher in sites undergoing tissue remodelling, such as malignancies (26). According to these findings, FAP became an attractive target for molecular imaging of various cancers and non-oncological disorders. Subsequently, different radiolabelled FAP inhibitors (FAPi) have been synthesised in order to assess the in vivo expression of FAP by PET imaging in various malignancies, including TC (27). The purpose of this systematic is to thoroughly evaluate the diagnostic performance of FAP-guided PET imaging in detecting TC lesions in different clinical scenarios.
2 Materials and methods
2.1 Protocol and review question
A preconceived protocol guided the development of the current systematic review (28). Namely, it was based on the “Preferred Reporting Items for a Systematic Review and Meta-Analysis” (PRISMA 2020 statement) for its put in writing (29). Supplementary Table 1 reports the thorough PRISMA checklist. The present systematic review was not registered in any comprehensive listing of systematic review protocols (e.g., PROSPERO).
The first step was defining a review question according to the PICO (Population, Intervention, Comparator, Outcomes) framework: which is the diagnostic yield (outcome) of FAP-guided PET imaging (intervention) in patients with TC (patient) compared with other imaging methods (comparator)? This predefined review question has guided the choice of eligibility criteria for the inclusion of pertinent studies in the systematic review.
The comprehensive literature search, study selection, quality assessment and data extraction were all performed by two reviewers (AR and GT) independently. Any disagreements among the reviewers were resolved through a discussion with a third reviewer.
2.2 Literature search strategy and information sources
As stated, the authors searched for articles concerning the employment of FAP guided PET in the management of TC using two distinct electronic bibliographic databases (Cochrane Library and PubMed/MEDLINE).
Taking into account the predefined review question, a search algorithm was created based on a combination of the following terms: (A) “FAP” OR “FAPi” AND (B) “thyroid.” Terms such as “PET,” “positron,” and “cancer” were deliberately excluded from the search algorithm since the authors agreed on opting for a more sensitive research string than for a specific one, trying to collect all the articles concerning the preconceived topic.
No restrictions were applied regarding the articles’ language or publication year. Moreover, reviewers screened included studies’ references, searching for additional eligible articles considering the review question. The literature search was last updated on 06 December 2023.
2.3 Eligibility criteria
This systematic review deemed clinical trials reporting data on the diagnostic yield of FAP-guided PET imaging in TC patients appropriate for inclusion. Editorials, letters, reviews, comments, case reports, minor case series, and original investigations on different topics than the preconceived one (including pre-clinical studies) were excluded. Moreover, since the present review had the aim to assess the potential role of FAP-guided PET in TC diagnostics, studies concerning the role of FAP-targeting radiopharmaceuticals as radioligand therapy agents were not considered as papers in the field of interest.
2.4 Selection process
At least two review authors (AR and GT) separately examined the titles and abstracts from the list of records generated using the search string in the selected bibliographic databases. They chose the studies eligible for the systematic review based on the stated inclusion and exclusion criteria, explaining their reasoning for each selection.
2.5 Data collection process and data extraction
The reviewers (AR and GT) gathered data from all of the included studies, taking advantage of full-text, tables, and figures regarding general study information (authors, publication year, country, study design, funding sources); patients’ characteristics (sample size, age, sex ratio, clinical setting, histological TC subtypes, serum markers levels); and index text characteristics (employed radiopharmaceuticals, hybrid imaging protocol, administered radiopharmaceutical activity, uptake time).
2.6 Quality assessment (risk of bias assessment)
QUADAS-2, a methodology for evaluating quality in diagnostic test accuracy studies, was chosen to analyse the risk of bias in individual studies and their applicability to the review question (28). Two authors (AR and GT) graded the research in the systematic review based on the potential for bias in four areas (patient selection, index test, reference standard, and flow and timing) and applicability in three categories (patient selection, index test, and reference standard).
3 Results
3.1 Literature search and study selection
The literature search was updated on 06 December 2023 and yielded 244 records. Subsequently, 238 publications were excluded based on the previously stated selection criteria. Of these, 217 were deemed irrelevant to the field of interest, five were reviews, editorials, book chapters, or letters related to the analysed topic, and 16 were case reports within the field of interest. At the end, six publications were judged as eligible by the review authors and they were included in the qualitative synthesis based on the preconceived inclusion criteria (30–35). Reviewers were unable to locate any further appropriate publications by examining the references of these articles. Figure 1 provides a concise overview of the approach used to select the studies for this analysis.
3.2 Study characteristics
The present systematic review includes six studies satisfying the inclusion criteria. These studies involve 247 TC patients and are comprehensively analysed in Tables 1–3 (30–35). The chosen studies were published by Chinese (3/6), Indian (2/6), and Turkish (1/6) groups during the years 2022 and 2023. Half of the studies reported in the analysis utilized a prospective design, while the remaining half conducted a retrospective analysis of their case studies. Each trial included in the review was performed in a single Institution. Additionally, two studies mentioned the sources of funding in their text.
Table 2 provides details regarding the TC patients included across the different studies. The number of individuals involved ranged from 24 to 117, with an average age of 42.4 to 53.2 years. The proportion of male participants varied from 24 to 51%. The index test was only employed for restaging RAI-R DTC patients in two papers (29, 32). In three research, it was utilized for restaging DTC patients regardless of their refractoriness to RAI (31–33). The last study focused on employing the index test for both staging and restaging MTC patients (35). Regarding histologic subtypes, five studies included individuals with DTC, with the most common variant being papillary thyroid cancer (PTC) with 184 patients (30–34). The remaining research specifically targeted patients who had been diagnosed with MTC (33). Regarding the five investigations dealing with DTC, the average value of Tg varied from 60 to 1552 ng/mL (30–34). Conversely, the only study including patients diagnosed with MTC revealed a central value of calcitonin at 666.5 pg/mL (35). Ultimately, tree research studies compared the results obtained from the index test using only [18F]FDG PET/CT (31, 33, 34); another publication compared the index test to [18F]FDG PET/CT and post-therapeutic RAI whole-body scan (32); one paper utilised contrast-enhanced CT as a comparator (30), while the last paper employed [68Ga]Ga-DOTANOC PET/CT (35).
The index test features exhibited substantial variation among the studies taken into account, as seen in Table 3 of the present review. Three experiments utilised [68Ga]Ga-DOTA-FAPi-04 (30, 31, 33), two studies employed [68Ga]Ga-DOTA-SA-FAPi (34, 35), and one utilised [18F]FAPi-42 (32). The administered activity varied between 180 and 222 MBq when measured using absolute values and between 1.8 and 2.22 MBq/Kg when measured using relative values. Furthermore, there was a time interval of 30 to 60 min between the administration of the experimented radiopharmaceutical and the PET imaging procedure. All the experiments utilised PET/CT as a hybrid imaging technique. PET scans in all the included studies were analysed using qualitative and semiquantitative techniques (30–35). The PET metrics assessed in the included publications were the target-to-background uptake ratio (TBR), as well as the maximal and mean standardised uptake values (SUVmax and SUVmean) of the pathological findings under examination. Regarding the PET metrics documented in the research, two articles authored by the same group presented the standardised uptake value adjusted for the lean body mass of the analysed lesions (SULpeak) (34, 35).
3.3 Risk of bias and applicability
The authors assessed the risk of bias and the relevance of the included publications using the QUADAS-2 tool, extracting the information presented in each investigation. Figure 2 presents the findings concerning the quality evaluation as well as the concerns regarding the applicability of the included research.
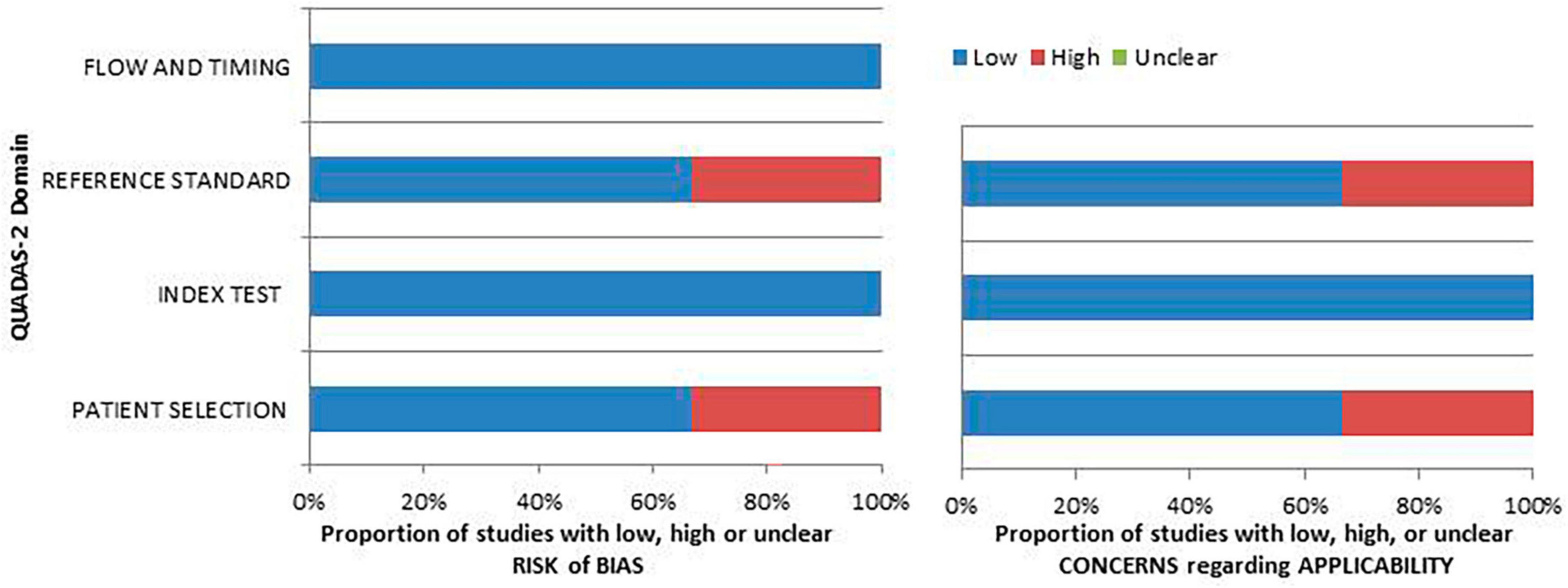
Figure 2. An iconographic summary of the quality assessment carried out with the QUADAS-2 tool. The researchers classified the papers included in the qualitative synthesis according to their degree of bias or applicability concerns for specific areas indicated on the ordinate axis. By contrast, the abscissa axis displays the percentage of studies. Based on the graph, about the 40% of the assessed studies demonstrate a notable risk of bias in the “patient selection” and “reference standard” domains.
3.4 Results of individual studies (qualitative synthesis)
None of the reports included in the analysis revealed any negative effects following the injection of FAP-targeting radiopharmaceuticals (30). None of the included papers evaluated the inter-reader agreement of FAP-targeted PET images in assessing thyroid malignancies. All the studies dealing with DTC evaluated the uptake of FAP-targeting radiopharmaceuticals in both regional and metastatic sites: in the majority of reports, the tumour uptake was reported to be higher than the surrounding background. Concerning the semiquantitative metrics, average SUVmax reported values ranged from 4.2 and 12.6 for local recurrences and varied between 4.1 and 9.1 for metastatic lesions, including neck lymph nodes and distant metastases in lung, bone, liver and pleura. The high heterogeneity observed among the included studies may be explained by differences in the administered FAP-targeting radiopharmaceutical forms, radiolabelled with different positron-emitters radionuclides, in the PET devices employed and in the uptake times (30–35).
Among the studies exploring the role of FAP-targeted PET in DTC patients, one expressed the uptake of the lesions using standardized uptake value corrected for lean body mass (SULpeak) as the unit of measurement; subsequently, it was not feasible to compare its results to the other included studies. Among the included papers, four assessed the FAP-guided PET diagnostic yield in the detection of local recurrences as well as of distant metastases in DTC based on a per-patient analysis: two of them reported an overall value of detection rate (DR) of approximately 85%, one reported an overall sensitivity and specificity of 96 and 50%, respectively, and one assessed a DR for lymph node metastases of 86%, for lung lesions of 81.7% and bone secondaries of 100%. Conversely, only two of the included studies performed a per lesion analysis, with one paper reporting a sensitivity and specificity for neck lesions of 83 and 42%, respectively, and a DR for distant metastases of 79%, and the other reporting a DR for lymph node metastases of 95.4% (30–34).
When compared to [18F]FDG PET, the index test overall showed a superior diagnostic performance; FAP-guided PET was indeed able to reveal a higher number of lesions both in lymph nodes as well as in lung, bone, and liver; however, its superiority was not statistically relevant in most of the cases. The results of the included papers dealing with DTC, including semiquantitative metrics, sites of the lesions, and diagnostic yield of the index test, are reported in Table 4 (30–34).
Only one of the included papers focused on patients diagnosed with MTC, enrolling subjects both in a staging and restaging setting. A comprehensive overview of the FAP-guided PET metrics and diagnostic accuracy reported in the paper are presented in Table 4. Concerning the diagnostic performance of the index test when compared to [68Ga]Ga-DOTANOC PET/CT, it showed higher accuracy in detecting both local recurrences and distant metastases (35).
With regard to FAP-guided PET imaging accuracy in different TC histopathologic subtypes, none of the included studies made a statistical analysis to assess differences in uptake values among the explored histopathologic variants. Moreover, it is worth noting that none of the included studies incorporated an immunohistochemistry (IHC) analysis in its design to assess the immunostaining of FAP in biopsy samples; in this setting, only one of the included papers performed an IHC analysis to evaluate the expression of FAP in the TME only on one pleural lesion.
Since each study explored the diagnostic yield of FAP-guided PET using a single radiopharmaceutical form, no reports are available concerning the differences in the accuracy of the different FAP-targeting radiopharmaceuticals available in this clinical setting.
4 Discussion
The upregulation of FAP on the cell membrane of stromal cells in the TME offers a promising opportunity for molecular imaging and potentially radioligand therapy (35). In the past few years, there has been a consistent increase in clinical research investigating the application of PET imaging using radiolabelled FAPi in several contexts, mainly in oncology. This emerging research provides crucial insights into the potential applications of this innovative diagnostic approach. Moreover, recent investigations have demonstrated that FAP-guided PET has displayed remarkable results in identifying various malignancies, including tumours frequently associated with limited or insignificant [18F]FDG uptake (36–38). FAP-targeted PET imaging offers several advantages, since it exhibits relatively lower levels of background activity in muscle and blood pool compared to other tracers (39).
Over the past 2 years, various clinical studies have attempted to assess the diagnostic yield of PET imaging using radiolabelled FAPi in TCs. The research aimed to determine the accuracy of FAPi in various clinical scenarios and to identify its conceivable applications for individuals diagnosed with DTC and MTC (30–35). These research papers have included newly diagnosed individuals and those who previously had surgical procedures or systemic treatments, including RAI therapy. The objective of this systematic review was to collect the existing data, analyse variations among the included studies, and advocate for future research perspectives to ultimately establish a more accurate assessment of the effectiveness of FAP-guided PET.
One of the main issues concerning the regular employment of FAP-targeting radiopharmaceuticals, both as a diagnostic probe or as a theragnostic compound, is the radiopharmaceutical retention in the target cells, especially in monomeric pharmaceutical forms, irrespectively of the radionuclide it is radiolabelled with (36). This statement opens a debate on the best uptake time to acquire emissive images. In the studies gathered in this systematic review, the uptake time ranged between 30 and 60 min, and none of them included a dynamic acquisition in their design. Theoretically, using a shorter uptake time should forestall the radiopharmaceutical excretion; however, dynamic studies of biodistribution in different neoplasms are warranted to assess the best uptake time and advocate for univocal procedure guidelines.
Concerning the diagnostic performance of FAP-targeted PET in DTC, despite most of the studies gathered in this systematic review where proof of concept trials enrolling a constrained number of DTC patients, the evidence is relatively homogeneous (30–34). Indeed, the four studies assessing the DR of this novel instrumental examination observed optimal values in neck lesions (expressed as local recurrence and lymph node metastases) as well as distant metastases in typical and atypical anatomic sites, including lung, liver, bone, and pleura. Interestingly, when compared to [18F]FDG PET, FAP-targeted PET imaging showed more true positive findings and fewer false negative results (30, 31, 33, 34). Despite these differences in terms of number of lesions were not statistically significant in any of the included papers, more studies are needed to assess if the increased DR of this novel imaging technique might be able to change DTC patients’ stage and, subsequently, management. The difference in terms of DR of these two instrumental examinations might underlie the variable diagnostic accuracy of [18F]FDG PET in DTC, which is affected by several different clinical and histological features (40–42); however, since none of the included studies assessed the differences in diagnostic accuracy of both examinations based on histologic variants and clinical features; it is not feasible to validate this hypothesis. In this setting, it is noteworthy that [18F]FDG has a valuable diagnostic performance in DTC patients with hematogenous metastases, as stated in a recent meta-analysis of literature (43); unfortunately, it is currently not feasible to make an indirect comparison of these two imaging techniques since the data gathered in this systematic review does not allow to pool the diagnostic accuracies reported in the included studies. More studies are needed in this setting to assess which patients might benefit from FAP-targeted imaging rather than [18F]FDG PET. Furthermore, given the recent technologic developments in PET devices, which allow dual-tracer acquisition protocols, future research should explore the potential impact of an imaging technique assessing two metabolic pathways (related on glucose metabolism and fibroblastic tissue remodelling, respectively) in DTC patients (44, 45).
Regarding the diagnostics of DTC, recent IHC and molecular imaging studies explored the in vitro and in vivo expression of prostate-specific membrane antigen (PSMA, also known as carboxypeptidase type II), reporting a variable expression of this transmembrane protein on the surface of neo endothelial cells within tumour’s neo angiogenesis and conflicting results concerning the potential employment of PSMA-targeted PET in DTC patients (46–48). Given the lack of existing literature comparing these two tracers in DTC patients and the recent development of bispecific radiopharmaceuticals designed to target both FAP and PSMA, it is necessary to conduct prospective studies encompassing both diagnostic methods in order to assess which instrumental examination is more dependable for this type of malignancy (49).
Only one of the included studies explored the diagnostic accuracy of FAP-targeted PET in MTC, reporting an optimal DR in the detection of neck lesions as well as distant metastases, superior to [68Ga]Ga-DOTA-NOC PET (35). In recent literature, it is reported that FAP is highly expressed in peritumoral and intratumoral stromal compartments of MTC and that the expression of AP positively correlates with the level of desmoplasia determined in the histological analysis (50–52). Moreover, it has been reported that desmoplasia is associated with a higher incidence of lymph node metastases in both PTC and MTC. The high uptake of radiolabelled FAPi in MTC lesions is likely explained by the stromal development and the abundant stromal components found even at the early stages of MTC onset (52). As well known, neuroendocrine neoplasms accumulate amine precursors and amino acids, including dihydroxyphenylalanine (DOPA). This molecule, labelled with [18F]F, has been successfully used as an imaging compound for PET/CT investigations in neuroendocrine tumours and is currently considered the best choice for imaging MTC patients in various clinical settings (53, 54). In order to assess the actual advantage of using FAP-targeting radiopharmaceuticals rather than currently available tracers in MTC and advocate for their regular employment in clinical practice, it is necessary to develop clinical trials comparing FAP-targeted imaging to [18F]DOPA PET in patients diagnosed with this malignancy.
So far, RAI has been the most often used additional treatment for patients with intermediate- or high-risk of disease recurrence or metastatic DTC (55). However, patients with advanced DTC either have inherent resistance or develop resistance to this treatment (56). Additionally, MTC and anaplastic TC do not exhibit a valuable response to RAI therapy due to their inability to metabolize iodine. Despite significant advancements in Tyrosine Kinase inhibitor treatments for RAI-R TC in recent years, the presence of drug resistance continues to pose a significant challenge in enhancing prognosis (57). To date, there are no alternative therapies accessible to individuals with progressive tumours who have either completed or declined traditional treatment choices (58). Based on these statements, FAP-targeted therapy with radiolabelled FAPI might provide a potential therapeutic option for RAI-R TC patients. In this setting, several [177Lu]Lu-radiolabelled FAP-targeting compounds were tested to assess their safety; as a result, these pharmaceuticals were well tolerated by RAI-R TC patients. Moreover, the preliminary data on the efficacy of these novel radiopharmaceuticals encourage further research to assess if they can significantly prolong survival in this clinical scenario (59–63). Table 5 synthesizes the main results of these trials.
This systematic review represents the first thorough literature examination concerning the use of FAP-targeting PET radiopharmaceuticals in patients with thyroid malignancies. However, it is worth noting that it accounts for significant limitations, including the limited number of studies in the field of interest with constrained sample sizes and their heterogeneity, which hampered the possibility of drawing up a quantitative analysis of the retrieved data. Furthermore, potential sources of bias about patient selection and comparative imaging domains were found in the included studies.
5 Conclusion
The presented systematic review has furnished qualitative data underscoring the potential role of FAP-targeted radiopharmaceuticals in the diagnostics of different forms of TC, and potentially in its employment as theragnostic agent.
Data availability statement
The original contributions presented in the study are included in the article/Supplementary material, further inquiries can be directed to the corresponding author.
Author contributions
AR: Writing – review and editing, Writing – original draft. DA: Writing – review and editing. FD: Writing – review and editing. MC: Writing – review and editing. BM: Writing – review and editing. SA: Writing – review and editing. MR: Writing – review and editing. FB: Writing – review and editing. AP: Writing – review and editing. GT: Writing – review and editing, Writing – original draft.
Funding
The author(s) declare that no financial support was received for the research, authorship, and/or publication of this article.
Conflict of interest
The authors declare that the research was conducted in the absence of any commercial or financial relationships that could be construed as a potential conflict of interest.
The author(s) declared that they were an editorial board member of Frontiers, at the time of submission. This had no impact on the peer review process and the final decision.
Publisher’s note
All claims expressed in this article are solely those of the authors and do not necessarily represent those of their affiliated organizations, or those of the publisher, the editors and the reviewers. Any product that may be evaluated in this article, or claim that may be made by its manufacturer, is not guaranteed or endorsed by the publisher.
Supplementary material
The Supplementary Material for this article can be found online at: https://www.frontiersin.org/articles/10.3389/fmed.2024.1381863/full#supplementary-material
References
1. McLeod D, Zhang L, Durante C, Cooper D. Contemporary debates in adult papillary thyroid cancer management. Endocr Rev. (2019) 40:1481–99. doi: 10.1210/er.2019-00085
2. Enewold L, Zhu K, Ron E, Marrogi A, Stojadinovic A, Peoples G, et al. Rising thyroid cancer incidence in the United States by demographic and tumor characteristics, 1980–2005. Cancer Epidemiol Biomark Prev. (2009) 18:784–91. doi: 10.1158/1055-9965.EPI-08-0960
3. Haugen B, Alexander E, Bible K, Doherty G, Mandel S, Nikiforov Y, et al. 2015 American thyroid association management guidelines for adult patients with thyroid nodules and differentiated thyroid cancer: The American thyroid association guidelines task force on thyroid nodules and differentiated thyroid cancer. Thyroid. (2016) 26:1–133. doi: 10.1089/thy.2015.0020
4. Fugazzola L, Elisei R, Fuhrer D, Jarzab B, Leboulleux S, Newbold K, et al. 2019 European thyroid association guidelines for the treatment and follow-up of advanced radioiodine-refractory thyroid cancer. Eur Thyroid J. (2019) 8:227–45. doi: 10.1159/000502229
5. Yoon D, Hwang H, Chang S, Rho Y, Ahn H, Kim J, et al. CT, MR, U,18F-FDG PET/CT, and their combined use for the assessment of cervical lymph node metastases in squamous cell carcinoma of the head and neck. Eur Radiol. (2009) 19:634–42. doi: 10.1007/s00330-008-1192-6
6. Rizzo A, Perotti G, Zagaria L, Lanni V, Racca M, Palestini N, et al. 18F-FDG PET/CT concurrent with first radioiodine post-therapeutic scan in high risk differentiated thyroid cancer: A useful tool or just an expensive diversion? Q J Nucl Med Mol Imaging. (2023) 67:158–66. doi: 10.23736/S1824-4785.22.03364-7
7. Leboulleux S, Schroeder P, Busaidy N, Auperin A, Corone C, Jacene H, et al. Assessment of the incremental value of recombinant thyrotropin stimulation before 2-[18F]-Fluoro-2-deoxy-D-glucose positron emission tomography/computed tomography imaging to localize residual differentiated thyroid cancer. J Clin Endocrinol Metab. (2009) 94:1310–6. doi: 10.1210/jc.2008-1747
8. Imperiale A, Berti V, Burgy M, Cazzato R, Piccardo A, Treglia G. Molecular imaging and related therapeutic options for medullary thyroid carcinoma: State of the art and future opportunities. Rev Endocr Metab Disord. (2023) 25:187–202. doi: 10.1007/s11154-023-09836-y
9. Costante G, Meringolo D, Durante C, Bianchi D, Nocera M, Tumino S, et al. Predictive value of serum calcitonin levels for preoperative diagnosis of medullary thyroid carcinoma in a cohort of 5817 consecutive patients with thyroid nodules. J Clin Endocrinol Metab. (2007) 92:450–5. doi: 10.1210/jc.2006-1590
10. Essig G, Porter K, Schneider D, Arpaia D, Lindsey S, Busonero G, et al. Multifocality in sporadic medullary thyroid carcinoma: An international multicenter study. Thyroid. (2016) 26:1563–72. doi: 10.1089/thy.2016.0255
11. Machens A, Dralle H. Biological relevance of medullary thyroid microcarcinoma. J Clin Endocrinol Metab. (2012) 97:1547–53. doi: 10.1210/jc.2011-2534
12. Moley J. Medullary thyroid carcinoma: Management of lymph node metastases. J Natl Compr Canc Netw. (2010) 8:549–56. doi: 10.6004/jnccn.2010.0042
13. Machens A, Dralle H. Biomarker-based risk stratification for previously untreated medullary thyroid cancer. J Clin Endocrinol Metab. (2010) 95:2655–63. doi: 10.1210/jc.2009-2368
14. Klain M, Hadoux J, Nappi C, Finessi M, Ambrosio R, Schlumberger M, et al. Imaging medullary thyroid cancer patients with detectable serum markers: State of the art and future perspectives. Endocrine. (2022) 75:330–7. doi: 10.1007/s12020-021-02930-8
15. Castinetti F, Taïeb D. Positron emission tomography imaging in medullary thyroid carcinoma: Time for reappraisal? Thyroid. (2021) 31:151–5. doi: 10.1089/thy.2020.0674
16. Treglia G, Sadeghi R, Giovinazzo F, Galiandro F, Annunziata S, Muoio B, et al. PET with different radiopharmaceuticals in neuroendocrine neoplasms: An umbrella review of published meta-analyses. Cancers (Basel). (2021) 13:5172. doi: 10.3390/cancers13205172
17. Yang X, Lin Y, Shi Y, Li B, Liu W, Yin W, et al. FAP promotes immunosuppression by cancer-associated fibroblasts in the tumor microenvironment via STAT3-CCL2 signaling. Cancer Res. (2016) 76:4124–35. doi: 10.1158/0008-5472.CAN-15-2973
18. Jiang K, Xu L, Ning J, Cheng F. FAP promotes clear cell renal cell carcinoma progression via activating the PI3K/AKT/mTOR signaling pathway. Cancer Cell Int. (2023) 23:217. doi: 10.1186/s12935-023-03073-8
19. Cho J, Byeon H, Oh K, Baek S, Kwon S, Jung K, et al. Clinicopathological significance of cancer-associated fibroblasts in papillary thyroid carcinoma: A predictive marker of cervical lymph node metastasis. Eur Arch Otorhinolaryngol. (2018) 275:2355–61. doi: 10.1007/s00405-018-5061-x
20. Rizzo A, Miceli A, Racca M, Bauckneht M, Morbelli S, Albano D, et al. Diagnostic accuracy of [68Ga]Ga labeled fibroblast-activation protein inhibitors in detecting head and neck cancer lesions using positron emission tomography: A systematic review and a meta-analysis. Pharmaceuticals. (2023) 16:1664. doi: 10.3390/ph16121664
21. Treglia G, Muoio B, Roustaei H, Kiamanesh Z, Aryana K, Sadeghi R. Head-to-head comparison of fibroblast activation protein inhibitors (FAPI) radiotracers versus [18F]F-FDG in oncology: A systematic review. Int J Mol Sci. (2021) 22:11192. doi: 10.3390/ijms222011192
22. Laudicella R, Spataro A, Crocè L, Giacoppo G, Romano D, Davì V, et al. Preliminary findings of the role of FAPi in prostate cancer theranostics. Diagnostics (Basel). (2023) 13:1175. doi: 10.3390/diagnostics13061175
23. Giesel F, Kratochwil C, Lindner T, Marschalek M, Loktev A, Lehnert W, et al. 68Ga-FAPI PET/CT: Biodistribution and preliminary dosimetry estimate of 2 DOTA-containing FAP-targeting agents in patients with various cancers. J Nucl Med. (2019) 60:386–92.
24. Sahai E, Astsaturov I, Cukierman E, DeNardo D, Egeblad M, Evans R, et al. A framework for advancing our understanding of cancer-associated fibroblasts. Nat Rev Cancer. (2020) 20:174–86. doi: 10.1038/s41568-019-0238-1
25. Peltier A, Seban R, Buvat I, Bidard F, Mechta-Grigoriou F. Fibroblast heterogeneity in solid tumors: From single cell analysis to whole-body imaging. Semin Cancer Biol. (2022) 86:262–72. doi: 10.1016/j.semcancer.2022.04.008
26. Mousavi M, Farhadi E, Vodjgani M, Karami J, Tahmasebi M, Sharafat Vaziri A, et al. Role of fibroblast activation protein alpha in fibroblast-like synoviocytes of rheumatoid arthritis. Iran J Allergy Asthma Immunol. (2021) 20:338–49. doi: 10.18502/ijaai.v20i3.6335
27. Mona C, Benz M, Hikmat F, Grogan T, Lueckerath K, Razmaria A, et al. Correlation of 68Ga-FAPi-46 PET biodistribution with FAP expression by immunohistochemistry in patients with solid cancers: Interim analysis of a prospective translational exploratory study. J Nucl Med. (2022) 63:1021–6. doi: 10.2967/jnumed.121.262426
28. Sadeghi R, Treglia G. Systematic reviews and meta-analyses of diagnostic studies: A practical guideline. Clin Transl Imaging. (2017) 5:83–7. doi: 10.1007/s40336-016-0219-2
29. Page M, McKenzie J, Bossuyt P, Boutron I, Hoffmann T, Mulrow C, et al. The PRISMA 2020 statement: An updated guideline for reporting systematic reviews. BMJ. (2021) 372:n71. doi: 10.1136/bmj.n71
30. Chen Y, Zheng S, Zhang J, Yao S, Miao W. 68Ga-DOTA-FAPI-04 PET/CT imaging in radioiodine-refractory differentiated thyroid cancer (RR-DTC) patients. Ann Nucl Med. (2022) 36:610–22. doi: 10.1007/s12149-022-01742-8
31. Fu H, Wu J, Huang J, Sun L, Wu H, Guo W, et al. 68Ga fibroblast activation protein inhibitor PET/CT in the detection of metastatic thyroid cancer: Comparison with 18F-FDG PET/CT. Radiology. (2022) 304:397–405. doi: 10.1148/radiol.212430
32. Mu X, Huang X, Jiang Z, Li M, Jia L, Lv Z, et al. [18F]FAPI-42 PET/CT in differentiated thyroid cancer: Diagnostic performance, uptake values, and comparison with 2-[18F]FDG PET/CT. Eur J Nucl Med Mol Imaging. (2023) 50:1205–15. doi: 10.1007/s00259-022-06067-2
33. Sayiner Z, Elboğa U, Sahin E, Ozturk S, Cayirli Y, Celen Y, et al. Comparison of 68Ga-FAPI-04 and 18F-FDG PET/CT for diagnosis of metastatic lesions in patients with recurrent papillary thyroid carcinoma. Hell J Nucl Med. (2023) 26:41–6. doi: 10.1967/s002449912560
34. Ballal S, Yadav M, Roesch F, Satapathy S, Moon E, Martin M, et al. Head-to-head comparison of [68Ga]Ga-DOTA.SA.FAPi with [18F]F-FDG PET/CT in radioiodine-resistant follicular-cell derived thyroid cancers. Eur J Nucl Med Mol Imaging. (2023) 51:233–44. doi: 10.1007/s00259-023-06404-z
35. Ballal S, Yadav M, Roesch F, Raju S, Satapathy S, Sheokand P, et al. Head-to-head comparison of [68Ga]Ga-DOTA.SA.FAPi and [68Ga]Ga-DOTANOC positron emission tomography/computed tomography imaging for the follow-up surveillance of patients with medullary thyroid cancer. Thyroid. (2023) 33:974–82. doi: 10.1089/thy.2023.0008
36. Gilardi L, Airò Farulla L, Demirci E, Clerici I, Omodeo Salè E, Ceci F. Imaging cancer-associated fibroblasts (CAFs) with FAPi PET. Biomedicines. (2022) 10:523. doi: 10.3390/biomedicines10030523
37. Kratochwil C, Flechsig P, Lindner T, Abderrahim L, Altmann A, Mier W, et al. 68Ga-FAPI PET/CT: Tracer uptake in 28 different kinds of cancer. J Nucl Med. (2019) 60:801–5. doi: 10.2967/jnumed.119.227967
38. Chen H, Zhao L, Ruan D, Pang Y, Hao B, Dai Y, et al. Usefulness of [68Ga]Ga-DOTA-FAPI-04 PET/CT in patients presenting with inconclusive [18F]FDG PET/CT findings. Eur J Nucl Med Mol Imaging. (2021) 48:73–86. doi: 10.1007/s00259-020-04940-6
39. Meyer C, Dahlbom M, Lindner T, Vauclin S, Mona C, Slavik R, et al. Radiation dosimetry and biodistribution of 68Ga-FAPI-46 PET imaging in cancer patients. J Nucl Med. (2020) 61:1171–7. doi: 10.2967/jnumed.119.236786
40. Salvatori M, Biondi B, Rufini V. Imaging in endocrinology: 2-[18F]-fluoro-2-deoxy-D-glucose positron emission tomography/computed tomography in differentiated thyroid carcinoma: Clinical indications and controversies in diagnosis and follow-up. Eur J Endocrinol. (2015) 173:R115–30. doi: 10.1530/EJE-15-0066
41. Santhanam P, Khthir R, Solnes L, Ladenson P. THE RELATIONSHIP OF BRAFV600E MUTATION STATUS TO FDG PET/CT AVIDITY IN THYROID CANCER: A REVIEW AND META-ANALYSIS. Endocr Pract. (2018) 24:21–6. doi: 10.4158/EP-2017-0080
42. Sonavane S, Basu S. Role of PET/computed tomography in elderly thyroid cancer: Tumor biology and clinical management. PET Clin. (2023) 18:81–101. doi: 10.1016/j.cpet.2022.09.005
43. Quartuccio N, Rubello D. Role of 18F-FDG PET/CT in detection of hematogenous metastases of advanced differentiated thyroid carcinoma: A systematic review and meta-analysis. J Cancer Metastasis Treat. (2021) 7:14. doi: 10.20517/2394-4722.2020.118
44. Filippi L, Dimitrakopoulou-Strauss A, Evangelista L, Schillaci O. Long axial field-of-view PET/CT devices: Are we ready for the technological revolution? Expert Rev Med Devices. (2022) 19:739–43. doi: 10.1080/17434440.2022.2141111
45. Roth K, Voltin C, van Heek L, Wegen S, Schomäcker K, Fischer T, et al. Dual-tracer PET/CT protocol with [18F]-FDG and [68Ga]Ga-FAPI-46 for cancer imaging: A proof of concept. J Nucl Med. (2022) 63:1683–6. doi: 10.2967/jnumed.122.263835
46. Rizzo A, Racca M, Dall’Armellina S, Delgado Bolton R, Albano D, Dondi F, et al. Potential role of PSMA-targeted PET in thyroid malignant disease: A systematic review. Diagnostics (Basel). (2023) 13:564. doi: 10.3390/diagnostics13030564
47. Sollini M, di Tommaso L, Kirienko M, Piombo C, Erreni M, Lania A, et al. PSMA expression level predicts differentiated thyroid cancer aggressiveness and patient outcome. EJNMMI Res. (2019) 9:93. doi: 10.1186/s13550-019-0559-9
48. Feng, Y, Shi Y, Xia Z, Xu L, Li W, Pang H, et al. The clinical signification and application value of [68Ga]Ga-PSMA imaging in thyroid malignancy. Endocrine. (2023). doi: 10.1007/s12020-023-03599-x [Epub ahead of print].
49. Verena A, Zhang Z, Kuo H, Merkens H, Zeisler J, Wilson R, et al. Synthesis and preclinical evaluation of three novel 68Ga-labeled bispecific PSMA/FAP-targeting tracers for prostate cancer imaging. Molecules. (2023) 28:1088. doi: 10.3390/molecules28031088
50. Prete A, Borges de Souza P, Censi S, Muzza M, Nucci N, Sponziello M. Update on fundamental mechanisms of thyroid cancer. Front Endocrinol (Lausanne). (2020) 11:102. doi: 10.3389/fendo.2020.00102
51. Arcucci A, Ruocco M, Granato G, Sacco A, Montagnani S. Cancer: An oxidative crosstalk between solid tumor cells and cancer associated fibroblasts. Biomed Res Int. (2016) 2016:4502846. doi: 10.1155/2016/4502846
52. Koperek O, Scheuba C, Puri C, Birner P, Haslinger C, Rettig W, et al. Molecular characterization of the desmoplastic tumor stroma in medullary thyroid carcinoma. Int J Oncol. (2007) 31:59–67.
53. Treglia G, Rufini V, Piccardo A, Imperiale A. Update on management of medullary thyroid carcinoma: Focus on nuclear medicine. Semin Nucl Med. (2023) 53:481–9. doi: 10.1053/j.semnuclmed.2023.01.003
54. Araz M, Soydal Ç, Demir Ö, Gökcan M, Küçük N. The Role of 18F-FDOPA PET/CT in recurrent medullary thyroid cancer patients with elevated serum calcitonin levels. Mol Imaging Radionucl Ther. (2023) 32:1–7. doi: 10.4274/mirt.galenos.2022.81904
55. Luster M, Pfestroff A, Hänscheid H, Verburg F. Radioiodine therapy. Semin Nucl Med. (2017) 47:126–34. doi: 10.1053/j.semnuclmed.2016.10.002
56. Chan W, Chan S, Kwong D. Radioiodine refractory differentiated thyroid cancer. Methods Mol Biol. (2022) 2534:243–57. doi: 10.1007/978-1-0716-2505-7_17
57. Schlumberger M, Tahara M, Wirth L, Robinson B, Brose M, Elisei R, et al. Lenvatinib versus placebo in radioiodine-refractory thyroid cancer. N Engl J Med. (2015) 372:621–30. doi: 10.1056/NEJMoa1406470
58. Ancker O, Krüger M, Wehland M, Infanger M, Grimm D. Multikinase inhibitor treatment in thyroid cancer. Int J Mol Sci. (2019) 21:10. doi: 10.3390/ijms21010010
59. Ballal S, Yadav M, Moon E, Rösch F, ArunRaj S, Agarwal S, et al. First-in-human experience with 177Lu-DOTAGA.(SA.FAPi)2 therapy in an uncommon case of aggressive medullary thyroid carcinoma clinically mimicking as anaplastic thyroid cancer. Clin Nucl Med. (2022) 47:e444–5. doi: 10.1097/RLU.0000000000004164
60. Ballal S, Yadav M, Moon E, Roesch F, Kumari S, Agarwal S, et al. Novel fibroblast activation protein inhibitor-based targeted theranostics for radioiodine-refractory differentiated thyroid cancer patients: A pilot study. Thyroid. (2022) 32:65–77. doi: 10.1089/thy.2021.0412
61. Fu H, Huang J, Sun L, Wu H, Chen H. FAP-targeted radionuclide therapy of advanced radioiodine-refractory differentiated thyroid cancer with multiple cycles of 177 Lu-FAPI-46. Clin Nucl Med. (2022) 47:906–7. doi: 10.1097/RLU.0000000000004260
62. Fu H, Huang J, Zhao T, Wang H, Chen Y, Xu W, et al. Fibroblast activation protein-targeted radioligand therapy with 177Lu-EB-FAPI for metastatic radioiodine-refractory thyroid cancer: First-in-human, dose-escalation study. Clin Cancer Res. (2023) 29:4740–50. doi: 10.1158/1078-0432.CCR-23-1983
Keywords: thyroid cancer, FAPI, PET, nuclear medicine, oncology, systematic review
Citation: Rizzo A, Albano D, Dondi F, Cioffi M, Muoio B, Annunziata S, Racca M, Bertagna F, Piccardo A and Treglia G (2024) Diagnostic yield of FAP-guided positron emission tomography in thyroid cancer: a systematic review. Front. Med. 11:1381863. doi: 10.3389/fmed.2024.1381863
Received: 04 February 2024; Accepted: 27 February 2024;
Published: 25 March 2024.
Edited by:
Ronan Abgral, Centre Hospitalier Regional Universitaire (CHU) de Brest, FranceReviewed by:
Natale Quartuccio, Azienda Ospedaliera Ospedali Riuniti Villa Sofia Cervello, ItalyRiccardo Laudicella, Università degli Studi di Messina, Italy
Copyright © 2024 Rizzo, Albano, Dondi, Cioffi, Muoio, Annunziata, Racca, Bertagna, Piccardo and Treglia. This is an open-access article distributed under the terms of the Creative Commons Attribution License (CC BY). The use, distribution or reproduction in other forums is permitted, provided the original author(s) and the copyright owner(s) are credited and that the original publication in this journal is cited, in accordance with accepted academic practice. No use, distribution or reproduction is permitted which does not comply with these terms.
*Correspondence: Giorgio Treglia, Z2lvcmdpby50cmVnbGlhQGVvYy5jaA==