- 1Department of Systems Medicine, University of Rome Tor Vergata, Rome, RM, Italy
- 2UOSD Nephrology and Dialysis, Policlinico Tor Vergata, Rome, RM, Italy
- 3Department of Clinical Sciences, Catholic University Our Lady of Good Counsel, Tirana, Albania
- 4Section of Clinical Nutrition and Nutrigenomics, Department of Biomedicine and Prevention, University of Rome Tor Vergata, Rome, RM, Italy
- 5Programma Clinico di Tipo A “Nutrizione Clinica”, Policlinico Tor Vergata, Rome, RM, Italy
- 6Program in Specialization in Nephrology, Catholic University Our Lady of Good Counsel, Tirana, Albania
- 7Nuova Clinica Annunziatella, Rome, RM, Italy
Introduction: Sarcopenia is a chronic pathological condition, first defined in 2010 and revised in 2018. The most recent definition of sarcopenia focuses mostly on “low muscle strength.” A secondary form of sarcopenia is represented by uremic sarcopenia (US), a condition that characterizes end-stage kidney disease (ESKD) patients. The intramuscular adipose tissue (IMAT) seems to impact negatively on muscle strength, as it would seem to replace muscle fibers with a non-contractile component. The study aims to compare body composition parameters—both standardized and innovative—related to the diagnosis of US in hemodialysis (HD) patients, stratified by sarcopenia diagnosis. Furthermore, the different indices of sarcopenia are compared in order to evaluate their predictive capacity.
Methods: We analyzed 48 ESKD patients according to the sarcopenia diagnosis, obtained using dual-energy X-ray absorptiometry (DXA). Moreover, we assessed the presence of IMAT and calculated the sarcopenia index (SI).
Results: For the study, the enrolled population was divided according to the sarcopenia diagnosis: no sarcopenic patients had higher transferrin (p = 0.03), total proteins (p = 0.04), and azotemia pre-dialysis (p = 0.05) values. On the contrary, atherogenic indices were lower in no sarcopenic patients. Moreover, we observed an indirect correlation between the SI and parathyroid hormone (PTH) (p = 0.00138, R2 = 0.54). Finally, we calculated the prevalence of sarcopenia and sarcopenia adjusted for IMAT. We showed a different prevalence between sarcopenia diagnosed with a standard index and an index adjusted for IMAT (p = 0.043). In conclusion, we believe that the most important result obtained is the indirect correlation between SI and PTH. These data corroborate the theories, in which PTH seems to play a central role in the cachexia genesis. Moreover, the SI adjusted for IMAT seems to be a more reliable parameter for the early identification of subjects at risk of developing US, allowing timely implementation of targeted therapeutic strategies.
1 Introduction
The first definition of “sarcopenia” was established in 2010 by the European Working Group on Sarcopenia in Older People (EWGSOP) (1), aiming to identify and manage individuals at risk of developing this pathological condition. In 2018, the EWGSOP updated this definition (EWGSOP2) according to new scientific and clinical evidence (2). The updated definition of sarcopenia emphasizes “low muscle strength” and in addition to this parameter, the assessment of muscle low quantity and quality is necessary for confirming the condition. A third parameter for determining the degree of sarcopenia is physical performance; poor performance indicates severe sarcopenia.
Sarcopenia is a physiological phenomenon that occurs throughout the lifespan and is characterized by adverse muscle changes (muscle failure) due to aging (3). Regarding public health costs, sarcopenia increases the risk of hospitalization and healthcare expenses (4). In fact, sarcopenia impacts significantly on the risk of falls and fractures, and it is related to cardiovascular (CV) and respiratory diseases. Moreover, it affects the ability to perform daily activities, leads to mobility disorders, and can contribute to cognitive impairments, thus reducing the quality of life and increasing the risk of death (5–9). In fact, it has been demonstrated by several studies that the reduction of muscle mass and fat-free mass (FFM) is associated with a more rapid cognitive decline in elderly subjects (10, 11).
A secondary form of sarcopenia is represented by the uremic sarcopenia (US). The “uremic milieu” is a typical condition of those patients affected by end-stage kidney disease (ESKD) (12). This condition leads to reduced muscle strength and an enhancement of morbidity and mortality for all causes. US is due to a “set” of progressive and cumulative effects induced by chronic kidney disease (CKD) on skeletal muscles. The US etiology is multifactorial, and it is linked to several factors, such as hormonal imbalances, vitamin D deficiency, metabolic acidosis, reduced protein intake, physical inactivity, hemodialysis (HD) treatment, accumulation of uremic toxins, secondary anemia, and chronic inflammatory state (13–19).
The overall prevalence of sarcopenia in ESKD patients is very high, reaching 50% in those undergoing HD (20). This muscle alteration, also in CKD patients, increases the risk of fractures and CV events, reduces overall survival, and negatively impacts on personal autonomy and quality of life (21, 22). The poor quality of life causes cognitive impairments, namely a potential alteration of the mood with consequent social isolation and deterioration of nutritional status. Depression, in association with the chronic inflammatory state, contributes to spontaneously reduce protein-energy intake, causing or worsening malnutrition up to, in the most severe cases, cachexia and anasarca status (23, 24). Over the years, different methods have been proposed for assessing body muscle mass. These include (i) anthropometric measurements, (ii) skinfold thickness measurements, (iii) bioimpedance analysis, (v) dual-energy X-ray absorptiometry (DXA) scan, and (vi) computed tomography (CT) scan (25). Anthropometric measurements of muscle mass, particularly mid-arm circumference taken at the midpoint of the upper arm, are among the most commonly used methods (26). These measurements include body weight, height, and circumferences (most notably waist and hip), providing a simple, fast, and cost-effective means of estimating body composition. Starting from the ratio between body weight (expressed in kg) and height squared (expressed in meters), it is possible to calculate the body mass index (BMI) (27). Although the BMI is constantly used for the assessment of body composition, it presents a limitation of not being able to differentiate between lean mass and fat mass (FM) (28). In fact, it should be considered a “crude” measurement of body size as it cannot distinguish between skeletal muscle and adipose tissue (29). Another tool to detect body composition is the thickness of skinfolds. This technique permits the estimation of the subcutaneous adipose tissue, detected in standardized points of the body such as the triceps, iliac crest, biceps, and calf (30–32). Given the relationship between subcutaneous fat and total body fat, it is considered that the result of the measurement of the folds is a good indicator of total body fat (33). The thickness of the adipose panniculus varies according to age, gender, and ethnicity.
In the late 1990s, Baumgartner et al. (34) suggested the use of a new parameter to determine sarcopenia. In fact, these authors stated that through a DXA examination, it is possible to obtain the bone-free mass (BFM) and FFM of the arms and legs, namely the appendicular skeletal muscle mass (ASM). Sarcopenia is defined when reduced values of ASM divided by height squared (ASM/height2) are observed, compared to the reference population matched by age and gender (34). Although the reduction of this index may indicate an increase in the risk of disability up to 3–4 times in elderly women and men (34), it may not always be reliable.
In human beings, different anatomical adipose depots have been described, performing several functions throughout their lifespan (35). The five different fat deposits in the human body are: subcutaneous, visceral, intermuscular, intramuscular, and bone adipose depots (36). Two of these classes of adipocytes, specifically the intermuscular and intramuscular ones, are present inside the skeletal muscle mass. The former are fat deposits present in the spaces between the skeletal muscles, while the latter comprises all the adipocytes that interpose between the various muscle fibers of the skeletal muscle (37). The early differentiation of these two adipose tissues occurs during the third trimester in utero and becomes evident in the postnatal period (38). The differentiation ends during late adolescence, when the intermuscular adipose tissue decreases and, on the contrary, the intramuscular adipose tissue (IMAT) increases (39), and the term “intramuscular fat” refers to the sum of the intermuscular fat and the IMAT (40). Several studies demonstrated that elevated levels of the IMAT are associated with decreased levels of strength and physical performance (41). In fact, the IMAT would seem to replace muscle fibers with a non-contractile component, and, in turn, the strength decreases. Other studies speculated that the IMAT could impair the contraction in the myofibers, caused by altered neuromuscular activation and muscle blood flow. In addition, it could cause the local release of pro-inflammatory adipokines (42–44). For this reason, it is important to consider not only the ASM but also the IMAT for a better assessment of the body composition. This index should be corrected for the IMAT to have a more predictive value.
This study aims to compare body composition parameters (both standard and innovative ones) in HD patients, stratified according to the diagnosis of sarcopenia. These parameters were assessed using bioelectrical impedance analysis (BIA), dual-energy X-ray absorptiometry (DXA), handgrip strength (HGS), and anthropometric measurements.
Furthermore, the different indices of sarcopenia were compared to evaluate their predictive capacity, both adjusted or unadjusted, for IMAT.
2 Patients and methods
2.1 Study population
The study was conducted on 48 patients (34 men and 14 women) undergoing chronic maintenance HD for at least 3 months, and it employed a cross-sectional design. The inclusion criteria were ESKD, age over 18 years, and being treated with the same dialysis technique for at least 3 months. Exclusion criteria were the presence of cancer, HIV+, HBsAg+, HCV+, and active autoimmune diseases. The patients followed three-times-weekly scheme with an average duration of 3.5 h both for convective and diffusive techniques.
The patients were enrolled at the Dialysis Unit of Policlinico Tor Vergata (Rome, Italy) and the Dialysis Center of Nuova Clinica Annunziatella (Rome, Italy).
The study protocol complied with the Declaration of Helsinki, and it was approved by the Ethics Committee of Fondazione Policlinico Tor Vergata (PTV) of Rome (experimentation register number 60/16).
Written informed consent for the study participation was obtained for all enrolled patients during the period September 2016- September 2017. At the time of enrolment, all selected patients underwent routine bloodwork and body composition assessment, using BIA, DXA, and anthropometric measurements. The HGS test was assessed to measure muscle strength.
2.2 Laboratory parameters
Blood sampling was performed at the long interdialytic interval. Measurement of laboratory parameters was performed using an automated hematology analyzer XE-2100 (Sysmex, Kobe, Japan) for the determination of hemoglobin (Hb). All routine parameters were determined using the Dimension Vista 1500 (Siemens Healthcare Diagnostics, Milano, Italy).
All laboratory parameters were analyzed according to standard procedures in the Clinical Chemical Laboratories of Fondazione PTV of Rome.
Parathyroid hormone (PTH) was analyzed using the Alinity i Intact PTH Reagent Kit (Alinity i, Abbott GmbH & Co. KG, Germany).
For evaluation of inflammatory status, we examined the C-reactive protein (CRP), the erythrocyte sedimentation rate (ESR), platelet-to-lymphocyte ratio (PLR), neutrophil-to-lymphocyte ratio (NLR), and lymphocyte-to-monocyte ratio (LMR) (45).
2.3 Body composition assessment
For all patients, the body composition assessment was performed. In particular, patients remained supine during the entire dialysis treatment and for an additional 15 min after the end of the HD session. A BIA was performed at the end of this period. The BIA electrodes were placed on the side of the patient where there was no vascular access for HD.
The BIA (QUANTUM V, RJL Systems, Bari Italy) was used to measure resistance (Rz), reactance (Xc), total body water (TBW), and extracellular body water (ECW) (46, 47).
Weight and height were assessed using a scale and stadiometer (Invernizzi, Rome, Italy), and parameters were recorded to the nearest 0.1 kg and 0.1 cm, respectively. The waist and hip circumferences were collected with a flexible and non-stretchable metric tape, and the waist-to-hip ratio was calculated.
The BMI was calculated as body weight (kg)/height (m)2 and classified according to the World Health Organization (WHO) guidelines (48).
The whole and segmental fat mass (FM), lean mass (LM), and bone mass (BM) were measured using a DXA (Primus, X-ray densitometer; software version 1.2.2, Osteosys Co., Ltd., Guro-gu, Seoul, Korea) (49). The intra- and inter-subject coefficient of variation (CV% = 100 standard deviations-SD/mean) was below 5% to validate a measure. The coefficients on this instrument for five participants scanned six times over 9 months were 2.2% for FM and 1.1% for FM and LM.
The DXA is a technique initially used for the determination of bone mineral density and subsequently used in soft tissue analysis for the evaluation of fat and lean mass (50). DXA is one of the most accurate methods for measuring total and district body FM and FFM (51). Currently, it is considered a technique that provides greater accuracy for the evaluation of LM and FM, expressed both in percentage value and in kilogram in the different body districts, allowing to precisely determine the areas of fat accumulation and the possible increase of LM in certain body districts (52).
Percentage FM was computed as whole FM in kilogram divided by the total mass of all tissues, including whole LM and BM, as follows: FM% = [whole FM/(whole FM + LM + BM)] × 100.
The IMAT was obtained according to the formulas provided by Bauer et al. (53, 54):
• Log (IMAT) = −2.21 + (0.12 × fat) + (−0.0013 × fat2) for women
• Log (IMAT) = −2.05 + (0.12 × fat) + (−0.0013 × fat2) for men.
BIA is a technique that analyzes R and Xc using a low-voltage electric current at a high frequency (50 kHz). The flow of the current allows us to estimate the body composition according to the water content in the human body (55, 56). The biological tissues of our body can act both as conductors and as insulators: all fluids and LM are excellent conductors, while the fat is a bad conductor and offers a high resistance to the passage of current (57). From the physical point of view, the impedance is a vector characterized by the R component on the axis of the abscissae and Xc on the axis of the ordinate. The angle created by the vector is defined as the phase angle (PA) and depends on the Xc (58).
2.4 Handgrip strength test
To assess muscle strength, we used the HGS test with a Jamar Plus dynamometer. The cutoffs of HGS are <30 kg for men and < 20 kg for women (59). The patient performed the test in a sitting position with a 90-degree flexed elbow. The researcher set up the instrument and asked the patient to squeeze as hard as possible for a few seconds. Three repetitions were conducted on the arm without the arteriovenous fistula, and the average value was considered for analysis.
3 Statistical analysis
Collected data were entered into an Excel spreadsheet (Microsoft, Redmond, WA, United States), and the analysis was performed using the Windows Social Science Statistics Package, version 25.0 (IBM_SPSS, Chicago, IL, United States). The descriptive statistics have been reported, after the confirmation of Kolmogorov–Smirnov test, according to the mean value ± standard deviation (for the parameters with normal distribution). For non-normal variables, we consider the median and the interval (minimum: maximum). In the first case, Student’s t-Testo (parametric test) was applied, while in the second case, the Wilcoxon test (non-parametric test) was applied.
Pearson’s correlation analysis was carried out for the evaluation of the possible linear relationship between the sarcopenia index (SI) through the calculation of the coefficient of Pearson and its statistical significance. To overcome the differences between the ages of the two groups, we preliminary applied a linear regression model (beta regression) to compare the estimators in terms of bias, variance, type-1 error, and power.
A p value of <0.05 was considered statistically significant.
4 Results
A total of 48 HD patients (34 men and 14 women) were recruited for this study and were divided according to the sarcopenia diagnosis (2). Epidemiological findings are shown in Table 1.
The laboratory results (Table 2) showed that patients without sarcopenia diagnosis had a transferrin value higher than those with sarcopenia diagnosis (p = 0.03). Furthermore, higher values were observed for total proteins (p = 0.04) and azotemia pre-dialysis (p = 0.05) in patients without sarcopenia diagnosis compared to those with sarcopenia diagnosis.
We also analyzed the possible differences in the therapy of calcium–phosphorus metabolism (such as vitamin D analogs, phosphate chelating agents, and calcium mimetics) in sarcopenia and no-sarcopenia patients, highlighting no statistical differences between the two groups.
Anthropometric findings in the population of the study, divided according to the sarcopenia diagnosis, are reported in Table 3. Patients without sarcopenia showed higher values, in a statistically significant manner, of BMI (p = 0.01), PA (p = 0.02), FFM (p = 0.001), SI (p = 0.0001), SI adjusted for IMAT (p = 0.006), and femur t-score (p = 0.003) compared to sarcopenia patients.
We also observed an interesting indirect correlation between the SI and PTH, as reported in Figure 1 (p = 0.00138 and R2 = 0.54).
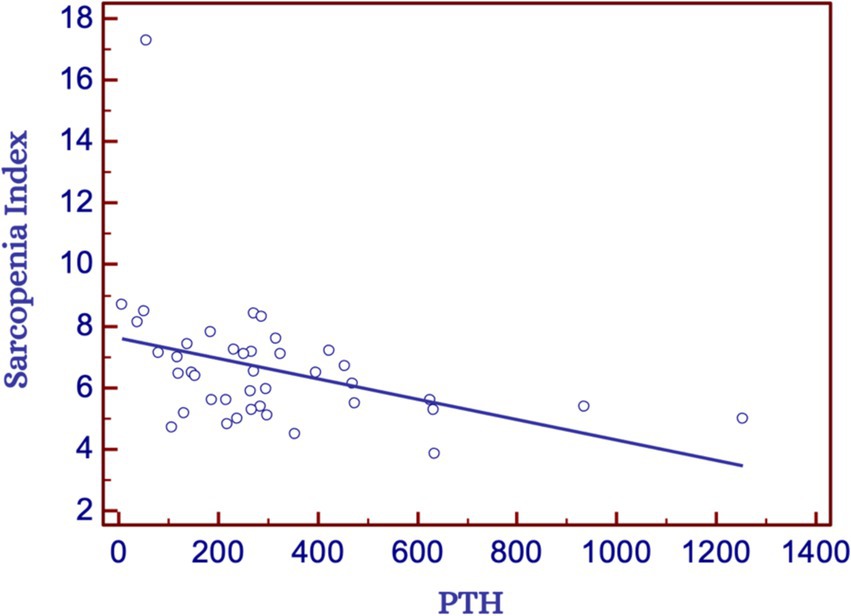
Figure 1. Correlation between sarcopenia index and PTH in the overall study population. PTH, Parathyroid hormone.
In Figure 2, we reported the prevalence of sarcopenia diagnosed according to SI compared to that obtained by SI adjusted for IMAT.
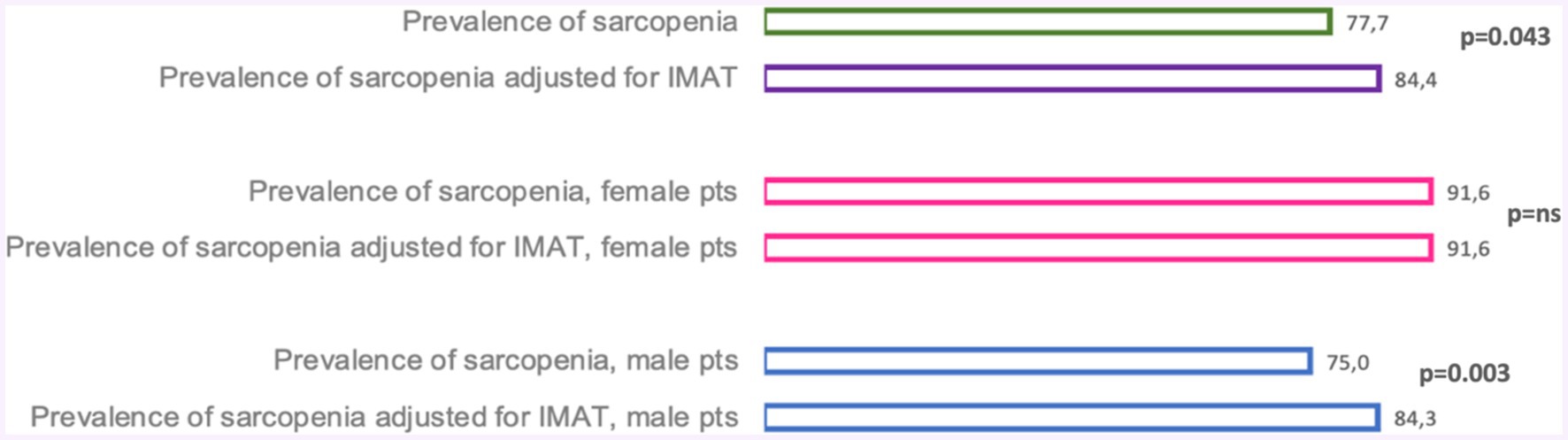
Figure 2. Sarcopenia index adjusted for IMAT in the overall study population and according to the male and female sex. IMAT, Intramuscular adipose tissue; ns, Not significant.
In the same figure, we showed, in both female and male patients, the prevalence of sarcopenia diagnosed by SI and the one obtained by SI, adjusted for IMAT. The difference in the prevalence of sarcopenia diagnosed using a standard index compared to that diagnosed using the SI adjusted for IMAT was statistically significant (p = 0.043).
For female patients, the prevalence of sarcopenia obtained using standard SI and the one detected using SI adjusted for IMAT was the same (p = ns). While, for male patients, when we adjusted the SI for IMAT, the prevalence was higher (p = 0.003).
5 Discussion
In our study, we enrolled a population of 48 HD patients and divided them into two subgroups based on the diagnosis of sarcopenia. In particular, 36 patients were affected by sarcopenia, namely 75% of our population. The mean age of sarcopenic patients was 69.6 ± 1.6 years vs. 56.3 ± 4.2 years for non-sarcopenic patients. The laboratory parameters highlighted a significant increase in transferrin, total protein, and azotemia pre-dialysis for non-sarcopenic patients. All these parameters are related to a better nutritional state. Several studies demonstrated that also serum transferrin concentration is a biomarker of nutritional state (60, 61).
Moreover, in our study population, we observed how sarcopenic patients had higher PTH values, demonstrating an inverse correlation between the SI and the serum PTH values. This correlation could be explained by the involvement of PTH in the browning of adipocytes through the activation of thermogenic genes (62). In fact, it is known in the literature that high levels of PTH can induce the browning of white adipose tissue, via the pathway involving the protein related to parathyroid hormone (PTHrP). This conversion leads to an increase in resting energy expenditure that, if not supported by an enhancement of caloric intake, induces a reduction of body weight, a loss of muscle mass, and, in the most severe case, cachexia (63, 64).
Furthermore, we highlighted a lower BMI in sarcopenic patients, according to previous literature studies conducted on different populations. In particular, Curtis et al. showed that in older adults, lower BMI values were significantly associated with a probable picture of sarcopenia, while higher BMI values were protective against sarcopenia (65). Another study, conducted on elderly women, demonstrated that higher BMI values were inversely correlated with low muscle mass (66).
In sarcopenic patients, the PA, obtained using the BIA, was lower than that obtained in non-sarcopenic patients. These data are supported by a recent study conducted by Wang et al. (67) in HD patients, which highlighted that PA should be useful in the detection of sarcopenia. The study was conducted on 241 HD patients, of which 28% were sarcopenic (diagnosis-based according to the Asian Sarcopenia Working Group). The sarcopenic patients showed lower PA values than the non-sarcopenic patients. This study concluded that PA should be helpful in predicting the risk of sarcopenia in HD patients. Moreover, as observed in our study population, these authors pointed out a lower HGS in patients affected by sarcopenia, compared to non-sarcopenic patients. This result is easily explained, as the reduction of muscle mass can induce a decrease in the HGS.
In accordance with the literature (68), we observed a lower mean value of IMAT in non-sarcopenic patients than in sarcopenic patients, but the difference between the two groups was not statistically significant. This parameter is also related to the lower value obtained using the HGS test. In fact, the presence of adipose tissue in muscle induces a reduced contraction power of the muscle itself and, thus, a decreased functional capacity (69). In the general population, this phenomenon is mainly related to aging and physical inactivity. In CKD patients, this condition is ascribable to uremic risk factors such as low-grade chronic inflammatory status, metabolic acidosis, hyperparathyroidism, vitamin D deficiency, and mitochondrial dysfunctions (69, 70). The latter is caused by the accumulation of lipids and their derivatives either in and between muscle cells, inducing an impairment of fatty acid beta-oxidation and an enhancement of oxidative stress. These phenomena cause lipotoxicity that induces an increased production of pro-inflammatory cytokines, further worsening the systemic inflammation. This creates a vicious cycle, in which the inflammation induces muscle damage, which in turn exacerbates the inflammatory status (Figure 3).
In several chronic pathological conditions, it has been demonstrated that the loss of muscle strength and FFM are associated with lower bone mineral density (8, 71–73). In our population, we observed that sarcopenic patients had femur t-score values significantly lower than non-sarcopenic patients. In particular, sarcopenic patients present pathological femur t-scores, while non-sarcopenic patients present normal values. This observation is corroborated by a previous study conducted by Cheng et al. (74), in which the authors pointed out a possible link between “muscle-bone-lipid,” namely impairments of lipid metabolism and loss of muscle mass are associated with concomitant bone loss.
Moreover, it is important to underline the possible utility of SI adjusted for IMAT compared to the traditional SI. In fact, in our population, we highlighted a significant difference between the prevalence of sarcopenia diagnosed with the traditional SI compared to the SI adjusted for IMAT (p = 0.043).
Moreover, by dividing our population according to gender, we observed that these data are valid only for male patients. As reported in Figure 2 for female patients, we did not detect any statistical difference between sarcopenia diagnosed using the traditional SI and that diagnosed with the SI corrected for IMAT. We speculate that these data are related to the different adipose tissue expansions in male and female patients (75). In fact, the first ones are characterized by hypertrophic adipose tissue, while female subjects are characterized by hyperplastic adipose tissue. Moreover, we believe that it is necessary during diagnosis to define the sarcopenia category. In fact, sarcopenia can be categorized into: (i) pre-sarcopenia, as defined by the EWGSOP, i.e., by the presence of reduced muscle mass and normal muscle function; (ii) dynapenia, defined as normal muscle mass and reduced muscle function; (iii) and finally, full-blown sarcopenia. The latter has been defined by the diagnostic algorithm, drawn up by the Asian Working Group for Sarcopenia, as the simultaneous presence of low muscle mass associated with reduced muscle function (10, 76). The clinical management of chronic HD patients should therefore include the qualitative and quantitative evaluation of the muscle. In fact, the possible alterations present in the muscle should be studied in a specific and detailed manner, since the diagnosis of this comorbidity is fundamental for the prognosis and quality of life of ESKD patients.
The limitation of this study is the small sample size. In the future, it would be interesting to expand the population of enrolled patients to confirm the results obtained on a larger study population. The major strength is the originality of the study. In fact, for the first time in literature, the possible predictive value of IMAT on sarcopenia in an HD population has been examined, obtaining promising results.
6 Conclusion
In our study, we observed several interesting results, but the most important seems to be the indirect correlation between PTH and SI. In fact, these data corroborate new theories, already highlighted by other authors in the literature, in which PTH seems to play a central role in the cachexia genesis. Moreover, the SI adjusted for IMAT appears to be a reliable parameter, particularly for male patients, and is useful for the early identification of those at risk of developing US. This adjustment could facilitate the timely implementation of targeted therapeutic strategies.
Data availability statement
The raw data supporting the conclusions of this article will be made available by the authors, without undue reservation.
Ethics statement
The studies involving humans were approved by Comitato Etico Indipendente, Policlinico Tor Vergata, Rome. The studies were conducted in accordance with the local legislation and institutional requirements. The participants provided their written informed consent to participate in this study.
Author contributions
AN: Writing – review & editing, Supervision, Methodology, Conceptualization. MJC: Writing – original draft, Formal analysis. PG: Writing – original draft, Data curation. GM: Writing – original draft, Visualization, Investigation, Formal analysis, Data curation. LR: Writing – original draft, Methodology, Formal analysis, Data curation. AS: Writing – original draft, Investigation. MDL: Writing – original draft, Visualization, Investigation. ADL: Writing – review & editing, Supervision, Methodology, Conceptualization.
Funding
The author(s) declare that no financial support was received for the research, authorship, and/or publication of this article.
Acknowledgments
We would like to thank Gabriella Venafro for the English language revision.
Conflict of interest
The authors declare that the research was conducted in the absence of any commercial or financial relationships that could be construed as a potential conflict of interest.
AN, PG, GM and ADL, declared that they were an editorial board member of Frontiers, at the time of submission. This had no impact on the peer review process and the final decision.
Publisher’s note
All claims expressed in this article are solely those of the authors and do not necessarily represent those of their affiliated organizations, or those of the publisher, the editors and the reviewers. Any product that may be evaluated in this article, or claim that may be made by its manufacturer, is not guaranteed or endorsed by the publisher.
References
1. Wing, JS, Brender, JD, Sanderson, LM, Perrotta, DM, and Beauchamp, RA. Acute health effects in a community after a release of hydrofluoric acid. Arch Environ Health. (1991) 46:155–60. doi: 10.1080/00039896.1991.9937443
2. Cruz-Jentoft, AJ, Bahat, G, Bauer, J, Boirie, Y, Bruyere, O, Cederholm, T, et al. Sarcopenia: revised European consensus on definition and diagnosis. Age Ageing. (2019) 48:16–31. doi: 10.1093/ageing/afy169
3. Wilkinson, DJ, Piasecki, M, and Atherton, PJ. The age-related loss of skeletal muscle mass and function: measurement and physiology of muscle fibre atrophy and muscle fibre loss in humans. Ageing Res Rev. (2018) 47:123–32. doi: 10.1016/j.arr.2018.07.005
4. Bruyere, O, Beaudart, C, Ethgen, O, Reginster, JY, and Locquet, M. The health economics burden of sarcopenia: a systematic review. Maturitas. (2019) 119:61–9. doi: 10.1016/j.maturitas.2018.11.003
5. Bahat, G, and Ilhan, B. Sarcopenia and the cardiometabolic syndrome: a narrative review. Eur Geriatr Med. (2016) 7:220–3. doi: 10.1016/j.eurger.2015.12.012
6. Beaudart, C, Biver, E, Reginster, JY, Rizzoli, R, Rolland, Y, Bautmans, I, et al. Validation of the SarQoL(R), a specific health-related quality of life questionnaire for sarcopenia. J Cachexia Sarcopenia Muscle. (2017) 8:238–44. doi: 10.1002/jcsm.12149
7. Morley, JE, Abbatecola, AM, Argiles, JM, Baracos, V, Bauer, J, Bhasin, S, et al. Sarcopenia with limited mobility: an international consensus. J Am Med Dir Assoc. (2011) 12:403–9. doi: 10.1016/j.jamda.2011.04.014
8. Noce, A, Marrone, G, Ottaviani, E, Guerriero, C, Di Daniele, F, Pietroboni Zaitseva, A, et al. Uremic sarcopenia and its possible nutritional approach. Nutrients. (2021a) 13:147. doi: 10.3390/nu13010147
9. Schaap, LA, van Schoor, NM, Lips, P, and Visser, M. Associations of sarcopenia definitions, and their components, with the incidence of recurrent falling and fractures: the longitudinal aging study Amsterdam. J Gerontol A Biol Sci Med Sci. (2018) 73:1199–204. doi: 10.1093/gerona/glx245
10. Jung, H, Tanaka, S, Kataoka, S, and Tanaka, R. Association of sarcopenia, pre-sarcopenia, and dynapenia with the onset and progression of locomotive syndrome in Japanese older adults: a cross-sectional study. J Physiol Anthropol. (2023) 42:16. doi: 10.1186/s40101-023-00334-3
11. Uchida, K, Sugimoto, T, Tange, C, Nishita, Y, Shimokata, H, Saji, N, et al. Association between reduction of muscle mass and faster declines in global cognition among older people: a 4-year prospective cohort study. J Nutr Health Aging. (2023) 27:932–9. doi: 10.1007/s12603-023-2007-9
12. Noce, A, Fabrini, R, Dessi, M, Bocedi, A, Santini, S, Rovella, V, et al. Erythrocyte glutathione transferase activity: a possible early biomarker for blood toxicity in uremic diabetic patients. Acta Diabetol. (2014) 51:219–24. doi: 10.1007/s00592-013-0497-3
13. Caldiroli, L, Armelloni, S, Eskander, A, Messa, P, Rizzo, V, Margiotta, E, et al. Association between the uremic toxins indoxyl-sulfate and p-cresyl-sulfate with sarcopenia and malnutrition in elderly patients with advanced chronic kidney disease. Exp Gerontol. (2021) 147:111266. doi: 10.1016/j.exger.2021.111266
14. Fahal, IH. Uraemic sarcopenia: aetiology and implications. Nephrol Dial Transplant. (2014) 29:1655–65. doi: 10.1093/ndt/gft070
15. Miyazaki, S, Iino, N, Koda, R, Narita, I, and Kaneko, Y. Brain-derived neurotrophic factor is associated with sarcopenia and frailty in Japanese hemodialysis patients. Geriatr Gerontol Int. (2021) 21:27–33. doi: 10.1111/ggi.14089
16. Nishi, H, Takemura, K, Higashihara, T, and Inagi, R. Uremic sarcopenia: clinical evidence and basic experimental approach. Nutrients. (2020) 12:1814. doi: 10.3390/nu12061814
17. Noce, A, Marrone, G, Wilson Jones, G, Di Lauro, M, Pietroboni Zaitseva, A, Ramadori, L, et al. Nutritional approaches for the management of metabolic acidosis in chronic kidney disease. Nutrients. (2021c) 13:2534. doi: 10.3390/nu13082534
18. Uchitomi, R, Oyabu, M, and Kamei, Y. Vitamin D and sarcopenia: potential of vitamin D supplementation in sarcopenia prevention and treatment. Nutrients. (2020) 12:3189. doi: 10.3390/nu12103189
19. Watanabe, H, Enoki, Y, and Maruyama, T. Sarcopenia in chronic kidney disease: factors, mechanisms, and therapeutic interventions. Biol Pharm Bull. (2019) 42:1437–45. doi: 10.1248/bpb.b19-00513
20. Hren, M, Bevc, S, Hojs, R, Knehtl, M, Dvoršak, B, Galuf, TS, et al. Uraemic sarcopenia. Clin Nutr ESPEN. (2016) 14:48–9. doi: 10.1016/j.clnesp.2016.04.014
21. Doherty, TJ. Invited review: aging and sarcopenia. J Appl Physiol. (2003) 95:1717–27. doi: 10.1152/japplphysiol.00347.2003
22. Hanatani, S, Izumiya, Y, Onoue, Y, Tanaka, T, Yamamoto, M, Ishida, T, et al. Non-invasive testing for sarcopenia predicts future cardiovascular events in patients with chronic kidney disease. Int J Cardiol. (2018) 268:216–21. doi: 10.1016/j.ijcard.2018.03.064
23. Gunalay, S, Ozturk, YK, Akar, H, and Mergen, H. The relationship between malnutrition and quality of life in haemodialysis and peritoneal dialysis patients. Rev Assoc Med Bras. (2018) 64:845–52. doi: 10.1590/1806-9282.64.09.845
24. Lodebo, BT, Shah, A, and Kopple, JD. Is it important to prevent and treat protein-energy wasting in chronic kidney disease and chronic Dialysis patients? J Ren Nutr. (2018) 28:369–79. doi: 10.1053/j.jrn.2018.04.002
25. Kuriyan, R. Body composition techniques. Indian J Med Res. (2018) 148:648–58. doi: 10.4103/ijmr.IJMR_1777_18
26. Hu, F-J, Liu, H, Liu, X-L, Jia, S-L, Hou, L-S, Xia, X, et al. Mid-upper arm circumference as an alternative screening instrument to appendicular skeletal muscle mass index for diagnosing sarcopenia. Clin Interv Aging. (2021) 16:1095–104. doi: 10.2147/CIA.S311081
27. Santos, DA, Dawson, JA, Matias, CN, Rocha, PM, Minderico, CS, Allison, DB, et al. Reference values for body composition and anthropometric measurements in athletes. PLoS One. (2014) 9:e97846. doi: 10.1371/journal.pone.0097846
28. Flegal, KM, Shepherd, JA, Looker, AC, Graubard, BI, Borrud, LG, Ogden, CL, et al. Comparisons of percentage body fat, body mass index, waist circumference, and waist-stature ratio in adults. Am J Clin Nutr. (2009) 89:500–8. doi: 10.3945/ajcn.2008.26847
29. Paris, MT. Body composition analysis of computed tomography scans in clinical populations: the role of deep learning. Lifestyle Genom. (2020) 13:28–31. doi: 10.1159/000503996
30. Garn, SM. Anthropometry in clinical appraisal of nutritional status. Am J Clin Nutr. (1962) 11:418–32. doi: 10.1093/ajcn/11.5.418
31. Legaz, A, and Eston, R. Changes in performance, skinfold thicknesses, and fat patterning after three years of intense athletic conditioning in high level runners. Br J Sports Med. (2005) 39:851–6. doi: 10.1136/bjsm.2005.018960
32. Uccioli, L, Fleury, M, De Gregorio, M, Spilabotte, S, Pennica, M, Maiello, MR, et al. Can the body mass index and the waist:hips ratio (WHR) affect the correlation between impedance measurement and anthropometry in the evaluation of body composition? Minerva Endocrinol. (1990) 15:251–5.
33. Buscemi, S, Maneri, R, Di Noto, A, and Verga, S. Assessment of body composition in groups of subjects with different body size. Comparison of skinfold thickness and impedance methods. Ann Ital Med Int. (1994) 9:223–7.
34. Baumgartner, RN, Koehler, KM, Gallagher, D, Romero, L, Heymsfield, SB, Ross, RR, et al. Epidemiology of sarcopenia among the elderly in New Mexico. Am J Epidemiol. (1998) 147:755–63. doi: 10.1093/oxfordjournals.aje.a009520
35. Billon, N, and Dani, C. Developmental origins of the adipocyte lineage: new insights from genetics and genomics studies. Stem Cell Rev Rep. (2012) 8:55–66. doi: 10.1007/s12015-011-9242-x
36. Hausman, GJ, Basu, U, Du, M, Fernyhough-Culver, M, and Dodson, MV. Intermuscular and intramuscular adipose tissues: bad vs. good adipose tissues. Adipocytes. (2014) 3:242–55. doi: 10.4161/adip.28546
37. Wueest, S, Schoenle, EJ, and Konrad, D. Depot-specific differences in adipocyte insulin sensitivity in mice are diet- and function-dependent. Adipocytes. (2012) 1:153–6. doi: 10.4161/adip.19910
38. Tran, TT, Yamamoto, Y, Gesta, S, and Kahn, CR. Beneficial effects of subcutaneous fat transplantation on metabolism. Cell Metab. (2008) 7:410–20. doi: 10.1016/j.cmet.2008.04.004
39. Kishida, K, Funahashi, T, Matsuzawa, Y, and Shimomura, I. Visceral adiposity as a target for the management of the metabolic syndrome. Ann Med. (2012) 44:233–41. doi: 10.3109/07853890.2011.564202
40. Ogawa, M, Lester, R, Akima, H, and Gorgey, AS. Quantification of intermuscular and intramuscular adipose tissue using magnetic resonance imaging after neurodegenerative disorders. Neural Regen Res. (2017) 12:2100–5. doi: 10.4103/1673-5374.221170
41. Khoja, SS, Moore, CG, Goodpaster, BH, Delitto, A, and Piva, SR. Skeletal muscle fat and its association with physical function in rheumatoid arthritis. Arthritis Care Res. (2018) 70:333–42. doi: 10.1002/acr.23278
42. Kelley, DE, and Goodpaster, BH. Stewing in not-so-good juices: interactions of skeletal muscle with adipose secretions. Diabetes. (2015) 64:3055–7. doi: 10.2337/db15-0403
43. Yim, JE, Heshka, S, Albu, J, Heymsfield, S, Kuznia, P, Harris, T, et al. Intermuscular adipose tissue rivals visceral adipose tissue in independent associations with cardiovascular risk. Int J Obes. (2007) 31:1400–5. doi: 10.1038/sj.ijo.0803621
44. Yoshida, Y, Marcus, RL, and Lastayo, PC. Intramuscular adipose tissue and central activation in older adults. Muscle Nerve. (2012) 46:813–6. doi: 10.1002/mus.23506
45. Ahbap, E, Sakaci, T, Kara, E, Sahutoglu, T, Koc, Y, Basturk, T, et al. Neutrophil-to-lymphocyte ratio and platelet-tolymphocyte ratio in evaluation of inflammation in end-stage renal disease. Clin Nephrol. (2016) 85:199–208. doi: 10.5414/CN108584
46. De Lorenzo, A, Di Renzo, L, Morini, P, de Miranda, RC, Romano, L, and Colica, C. New equations to estimate resting energy expenditure in obese adults from body composition. Acta Diabetol. (2018) 55:59–66. doi: 10.1007/s00592-017-1061-3
47. De Lorenzo, A, Siclari, M, Gratteri, S, Romano, L, Gualtieri, P, Marchetti, M, et al. Developing and cross-validation of new equations to estimate fat mass in Italian population. Eur Rev Med Pharmacol Sci. (2019) 23:2513–24. doi: 10.26355/eurrev_201903_17399
48. WHO (2000). Obesity: preventing and managing the global epidemic. Report of a WHO consultation. World Health Organ Tech Rep Ser 894, i-xii, 1–253.
49. De Lorenzo, A, Romano, L, Di Renzo, L, Di Lorenzo, N, Cenname, G, and Gualtieri, P. Obesity: a preventable, treatable, but relapsing disease. Nutrition. (2020) 71:110615. doi: 10.1016/j.nut.2019.110615
50. Shepherd, JA, Ng, BK, Sommer, MJ, and Heymsfield, SB. Body composition by DXA. Bone. (2017) 104:101–5. doi: 10.1016/j.bone.2017.06.010
51. Haarbo, J, Gotfredsen, A, Hassager, C, and Christiansen, C. Validation of body composition by dual energy X-ray absorptiometry (DEXA). Clin Physiol. (1991) 11:331–41. doi: 10.1111/j.1475-097x.1991.tb00662.x
52. Bazzocchi, A, Ponti, F, Albisinni, U, Battista, G, and Guglielmi, G. DXA: technical aspects and application. Eur J Radiol. (2016) 85:1481–92. doi: 10.1016/j.ejrad.2016.04.004
53. Bauer, J, Thornton, J, Heymsfield, S, Kelly, K, Ramirez, A, Gidwani, S, et al. Dual-energy X-ray absorptiometry prediction of adipose tissue depots in children and adolescents. Pediatr Res. (2012) 72:420–5. doi: 10.1038/pr.2012.100
54. Colica, C, Merra, G, Gasbarrini, A, De Lorenzo, A, Cioccoloni, G, Gualtieri, P, et al. Efficacy and safety of very-low-calorie ketogenic diet: a double blind randomized crossover study. Eur Rev Med Pharmacol Sci. (2017) 21:2274–89.
55. Bohm, A, and Heitmann, BL. The use of bioelectrical impedance analysis for body composition in epidemiological studies. Eur J Clin Nutr. (2013) 67:S79–85. doi: 10.1038/ejcn.2012.168
56. Park, JH, Jo, YI, and Lee, JH. Clinical usefulness of bioimpedance analysis for assessing volume status in patients receiving maintenance dialysis. Kor J Intern Med. (2018) 33:660–9. doi: 10.3904/kjim.2018.197
57. Saladino, CF. The efficacy of bioelectrical impedance analysis (BIA) in monitoring body composition changes during treatment of restrictive eating disorder patients. J Eat Disord. (2014) 2:34. doi: 10.1186/s40337-014-0034-y
58. Lukaski, HC. Requirements for clinical use of bioelectrical impedance analysis (BIA). Ann N Y Acad Sci. (1999) 873:72–6. doi: 10.1111/j.1749-6632.1999.tb09451.x
59. Van Ancum, JM, Alcazar, J, Meskers, CGM, Nielsen, BR, Suetta, C, and Maier, AB. Impact of using the updated EWGSOP2 definition in diagnosing sarcopenia: a clinical perspective. Arch Gerontol Geriatr. (2020) 90:104125. doi: 10.1016/j.archger.2020.104125
60. Boland, M, and Gaskill, TD. Managing AIDS in children. MCN Am J Matern Child Nurs. (1984) 9:384–9. doi: 10.1097/00005721-198411000-00006
61. Sa Martins, V, Aguiar, L, Dias, C, Lourenco, P, Pinheiro, T, Velez, B, et al. Predictors of nutritional and inflammation risk in hemodialysis patients. Clin Nutr. (2020) 39:1878–84. doi: 10.1016/j.clnu.2019.07.029
62. Srisuwarn, P, and Disthabanchong, S. Role of parathyroid hormone and parathyroid hormone-related protein in protein-energy malnutrition. Front Biosci. (2023) 28:167. doi: 10.31083/j.fbl2808167
63. He, Y, Liu, RX, Zhu, MT, Shen, WB, Xie, J, Zhang, ZY, et al. The browning of white adipose tissue and body weight loss in primary hyperparathyroidism. EBioMedicine. (2019) 40:56–66. doi: 10.1016/j.ebiom.2018.11.057
64. Hedesan, OC, Fenzl, A, Digruber, A, Spirk, K, Baumgartner-Parzer, S, Bilban, M, et al. Parathyroid hormone induces a browning program in human white adipocytes. Int J Obes. (2019) 43:1319–24. doi: 10.1038/s41366-018-0266-z
65. Curtis, M, Swan, L, Fox, R, Warters, A, and O'Sullivan, M. Associations between body mass index and probable sarcopenia in community-dwelling older adults. Nutrients. (2023) 15:1505. doi: 10.3390/nu15061505
66. Yoo, MC, Won, CW, and Soh, Y. Association of high body mass index, waist circumference, and body fat percentage with sarcopenia in older women. BMC Geriatr. (2022) 22:937. doi: 10.1186/s12877-022-03643-x
67. Wang, Y, Hu, Y, Zhang, M, Jin, H, Wen, Y, Tang, R, et al. Bioelectrical impedance analysis-derived phase angle predicts sarcopenia in patients on maintenance hemodialysis. Nutr Clin Pract. (2023) 38:881–8. doi: 10.1002/ncp.10967
68. Perkisas, S, De Cock, AM, Verhoeven, V, and Vandewoude, M. Intramuscular adipose tissue and the functional components of sarcopenia in hospitalized geriatric patients. Geriatrics (Basel). (2017) 2:11. doi: 10.3390/geriatrics2010011
69. Avesani, CM, de Abreu, AM, Ribeiro, HS, Brismar, TB, Stenvinkel, P, Sabatino, A, et al. Muscle fat infiltration in chronic kidney disease: a marker related to muscle quality, muscle strength and sarcopenia. J Nephrol. (2023) 36:895–910. doi: 10.1007/s40620-022-01553-0
70. Gamboa, JL, Roshanravan, B, Towse, T, Keller, CA, Falck, AM, Yu, C, et al. Skeletal muscle mitochondrial dysfunction is present in patients with CKD before initiation of maintenance hemodialysis. Clin J Am Soc Nephrol. (2020) 15:926–36. doi: 10.2215/CJN.10320819
71. Bering, T, Diniz, KGD, Coelho, MPP, Vieira, DA, Soares, MMS, Kakehasi, AM, et al. Association between pre-sarcopenia, sarcopenia, and bone mineral density in patients with chronic hepatitis C. J Cachexia Sarcopenia Muscle. (2018) 9:255–68. doi: 10.1002/jcsm.12269
72. Cerulli, C, Moretti, E, Parisi, A, Tranchita, E, Di Lauro, M, Minganti, C, et al. Correlation between physical activity, nutritional intake, and osteoporosis in postmenopausal women: a preliminary evaluation. Eur Rev Med Pharmacol Sci. (2023) 27:5822–30. doi: 10.26355/eurrev_202306_32821
73. Noce, A, Marrone, G, Rovella, V, Cusumano, A, Di Daniele, N, and Casasco, M. Beneficial effects of physical activity on uremic sarcopenia. Med Sport. (2018) 71:370–92. doi: 10.23736/S0025-7826.18.03389-6
74. Cheng, L, and Wang, S. Correlation between bone mineral density and sarcopenia in US adults: a population-based study. J Orthop Surg Res. (2023) 18:588. doi: 10.1186/s13018-023-04034-7
75. Merra, G, Gualtieri, P, Cioccoloni, G, Falco, S, Bigioni, G, Tarsitano, MG, et al. FTO rs9939609 influence on adipose tissue localization in the Italian population. Eur Rev Med Pharmacol Sci. (2020) 24:3223–35. doi: 10.26355/eurrev_202003_20689
Keywords: uremic sarcopenia, dialysis patients, intramuscular adipose tissue, parathyroid hormone, sarcopenia index, body composition analysis
Citation: Noce A, Ceravolo MJ, Gualtieri P, Marrone G, Romano L, Shoshi A, Di Lauro M and De Lorenzo A (2024) Uremic sarcopenia: the role of intramuscular adipose tissue as a potential early identifier. Front. Med. 11:1372668. doi: 10.3389/fmed.2024.1372668
Edited by:
Philip M. Gallagher, University of Kansas, United StatesReviewed by:
Simone Vettoretti, IRCCS Ca ‘Granda Foundation Maggiore Policlinico Hospital, ItalyHugo Luca Corrêa, Catholic University of Brasilia (UCB), Brazil
Copyright © 2024 Noce, Ceravolo, Gualtieri, Marrone, Romano, Shoshi, Di Lauro and De Lorenzo. This is an open-access article distributed under the terms of the Creative Commons Attribution License (CC BY). The use, distribution or reproduction in other forums is permitted, provided the original author(s) and the copyright owner(s) are credited and that the original publication in this journal is cited, in accordance with accepted academic practice. No use, distribution or reproduction is permitted which does not comply with these terms.
*Correspondence: Annalisa Noce, YW5uYWxpc2Eubm9jZUB1bmlyb21hMi5pdA==; Paola Gualtieri, cGFvbGEuZ3VhbHRpZXJpQHVuaXJvbWEyLml0