- Department of Cybernetics and Biomedical Engineering, VSB–Technical University of Ostrava, Ostrava, Czechia
Electrogastrography (EGG) is a non-invasive method with high diagnostic potential for the prevention of gastroenterological pathologies in clinical practice. In this study, a review of the measurement systems, procedures, and methods of analysis used in electrogastrography is presented. A critical review of historical and current literature is conducted, focusing on electrode placement, measurement apparatus, measurement procedures, and time-frequency domain methods of filtration and analysis of the non-invasively measured electrical activity of the stomach. As a result, 129 relevant articles with primary aim on experimental diet were reviewed in this study. Scopus, PubMed, and Web of Science databases were used to search for articles in English language, according to the specific query and using the PRISMA method. The research topic of electrogastrography has been continuously growing in popularity since the first measurement by professor Alvarez 100 years ago, and there are many researchers and companies interested in EGG nowadays. Measurement apparatus and procedures are still being developed in both commercial and research settings. There are plenty variable electrode layouts, ranging from minimal numbers of electrodes for ambulatory measurements to very high numbers of electrodes for spatial measurements. Most authors used in their research anatomically approximated layout with two++ active electrodes in bipolar connection and commercial electrogastrograph with sampling rate of 2 or 4 Hz. Test subjects were usually healthy adults and diet was controlled. However, evaluation methods are being developed at a slower pace, and usually the signals are classified only based on dominant frequency. The main review contributions include the overview of spectrum of measurement systems and procedures for electrogastrography developed by many authors, but a firm medical standard has not yet been defined. Therefore, it is not possible to use this method in clinical practice for objective diagnosis.
Systematic Review Registration: https://www.prisma-statement.org/.
1 Introduction
Electrogastrography (EGG) is a diagnostic method used to measure the electrical potentials of the stomach muscles. The method is non-invasive and is measured transcutaneously using electrodes placed on the abdomen over the stomach location (1, 2). The first measurement of the electrogastrogram took place in the early 1920s on animal and human subjects by Professor Alvarez and his team (1, 3). Since then, due to the evolution of measuring instruments and its increasing measurement precision, the research popularity of the method has grown, and many researchers are still interested in this topic (4–9). Although this non-invasive method could complement or even replace current, more invasive diagnostic methods such as gastroscopy or X-ray examination in clinical practice, a medical standard for its consistent measurement has not yet been defined. However, several attempts to achieve its definition have been made by many authors (10–16).
The aim of this study is to analyze the research articles from the first measurements to the present and help future researchers to choose the appropriate equipment and methods to obtain a relevant electrogastrogram. The devices used range from universal bioamplifiers to single-purpose electrogastrographs with very low or very high sampling frequency and analog or digital filtering, which can significantly change the resulting measured signal and its analysis. The overall quality and the possible presence of noise in the signal lead to analysis using methods such as Wavelet transform in the time domain or Fourier transform in the frequency domain but also machine learning methods.
2 Review strategy
In this study, a review specifically aimed on existing electrogastrography measurement systems, procedures, and analysis methods in both research and clinical practice is presented. The technique that employed to obtain sources and conduct this review is based on the PRISMA review strategy. Various steps were taken in applying this strategy, including selecting appropriate databases, choosing suitable search terms, applying search criteria, and evaluating the results found. Sources utilized for the creation of this review consisted solely of full papers, original journal papers, conference proceedings, book chapters, other review papers, and graduation theses.
Only papers written in the English language were included, and the Scopus, PubMed, and Web of Science databases were used to search the literature. Query used for search in all mentioned databases was “gastric myoelectrical OR electrogastrographical OR electrogastrographic OR electrogastrography AND system OR device OR apparatus”. The results from the three databases were combined, and duplicates and non-English texts were discarded, resulting in 129 matches in July 2023. The selection process is shown in Figure 1.
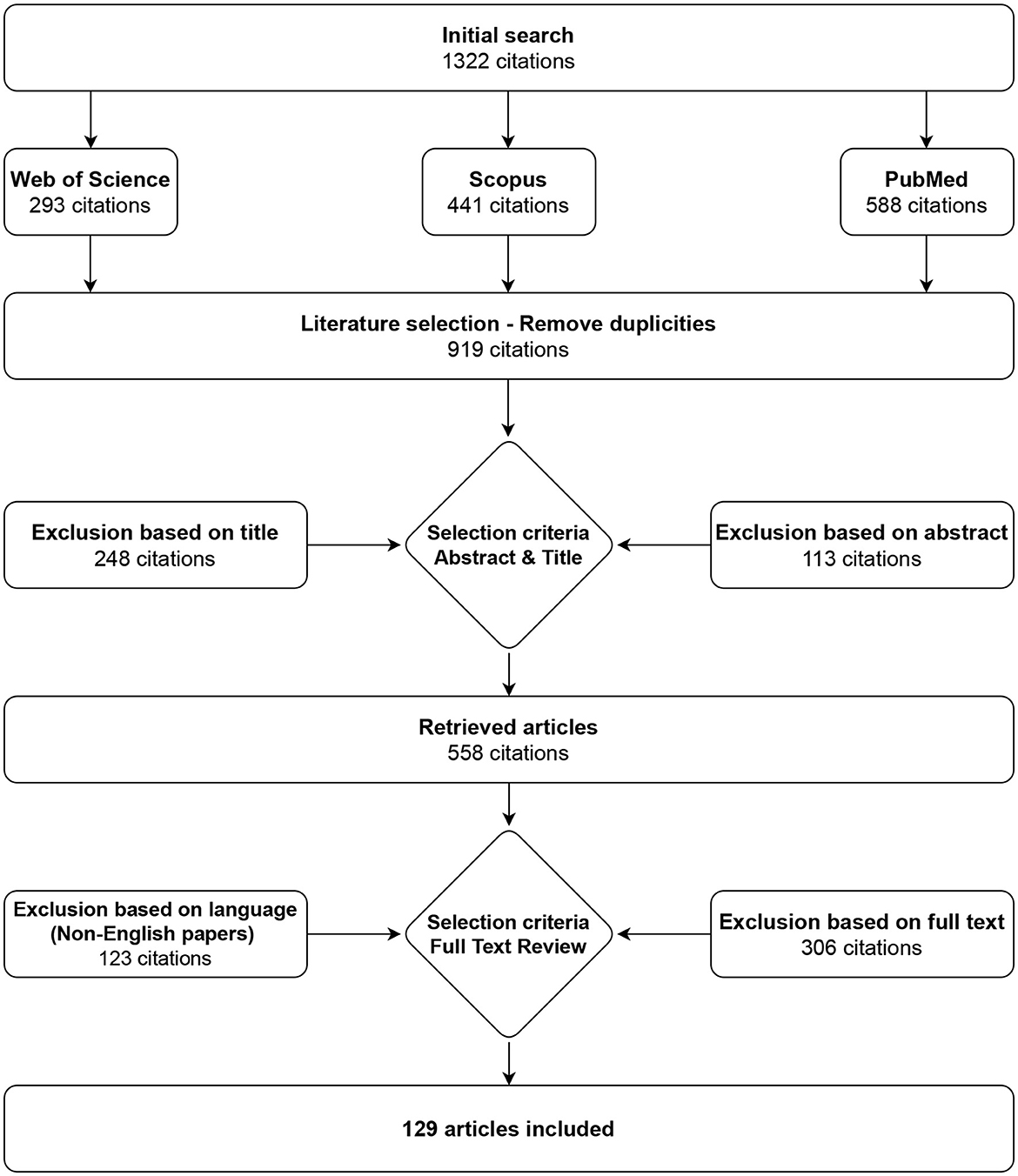
Figure 1. Selection strategy. A total of 129 articles on electrogastrography measurement devices, procedures, filtration, and analysis were identified.
3 Article structure
This study is structured to cover all related areas of assessment of electrical activity of the stomach. First, the principles of electrical activity of the stomach are described in detail, followed by an explanation of measurement and analysis methodologies. Finally, the results from these chapters are discussed and summarized. The overall structure of chapters is shown in Table 1.
4 Electrical activity of the stomach
Electrical potentials that occur on the surface of the stomach are the cause of spontaneous contractions leading to rhythmic activity of the smooth muscles of the stomach (17–19). The frequency of the generated electrical waves is very low, specifically in millihertz; therefore, the common unit describing the signal frequency is cycles per minute (CPM) (2, 20–22). The peak-to-peak voltage of the potentials measured invasively directly on the stomach surface is in the range of 1–2 mV (23–25), and the voltage of signals measured non-invasively on the abdominal wall is in the range of 200–400 μV (24, 26, 27). An example of a normal resting signal of the electrical activity of the stomach is shown in Figure 2. The physiological frequency of gastric electrical waves in the case of an empty stomach is in the range of 2–4 CPM, and in most cases, it is 3 CPM (1, 3, 23–34) as visible in power spectral density spectrum in Figure 3. The electrical activity of an empty stomach is called preprandial, and activity after a meal is called postprandial. The gastric electrical activity originates in places called gastric pacemakers located throughout the body and antrum with different structural characteristics, from which it spreads over the surface of the stomach with different intrinsic frequencies (35). The dominant gastric pacemaker is located in a part of the stomach called the corpus and in its subsection called the greater curvature (31, 36, 37). The electrical activity of the stomach generated by pacemakers originates in the interstitial Cajal cells, as confirmed by the fact that if the cells are removed, the electrical potentials disappear (35, 38–40).
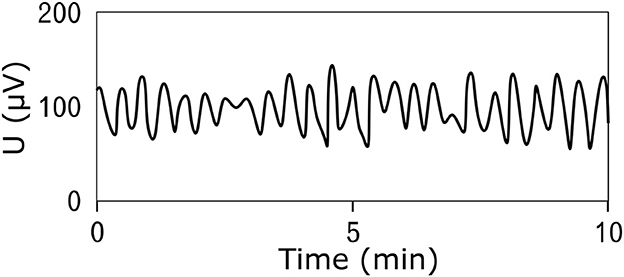
Figure 2. An example of the resting (prepreandial) electrical activity of the stomach (electrogastrogram) of a 22-year-old man obtained by the bipolar method of measurement using an universal bioamplifier. The signal was then low-pass filtered.
According to several studies, the characteristics of stomach electrical activity change drastically in case of pathological events, such as gastric or duodenal ulcers or stomach carcinoma. While some authors describe changes in the amplitude of the signal, mostly substantial changes occur in the frequency of the signal in preprandial and postprandial states (25, 28, 41, 42). The results from a recent study also suggest that signal frequency can be affected by taste, especially salty and sour tastes (43).
5 Measurement methodology
The methodology for measuring electrogastrogram has several steps, such as electrode placement, selecting the measurement instrument, and defining a standardized procedure. To place electrodes, the correct location of the stomach must be identified, the number of electrodes must be chosen, and a specific layout of electrodes must be defined. The measuring apparatus must have standardized parameters to meet the requirements for the acquisition of accurate measurements. The examination procedure must be defined to ensure objectivity and repeatability.
5.1 Placement of electrodes
Before the start of measurement, the electrodes must be placed on the abdominal surface to the location supposing overlay of the specific parts of human stomach. However, because the stomach is not visible through the skin, there are several approaches to specify its location reliably or approximately.
5.1.1 Anatomical approximation
Based on an anatomical similarity of each human being, approximative placement of electrodes could be considered. Locations of electrodes are defined in respect to geometrical relations between anatomical features of human body, while proportional differences between specific individuals are taken into account (4, 12, 26, 44–48). The same approach is used also for other standard diagnostic methods, for example, electrocardiography or electroencephalography. The approach could be considered as the fastest and with potential appropriate use in clinical practice as it does not have any other technical requirements and can be done without attendance of physician. An example of anatomical approximation layout is shown in Figure 4.
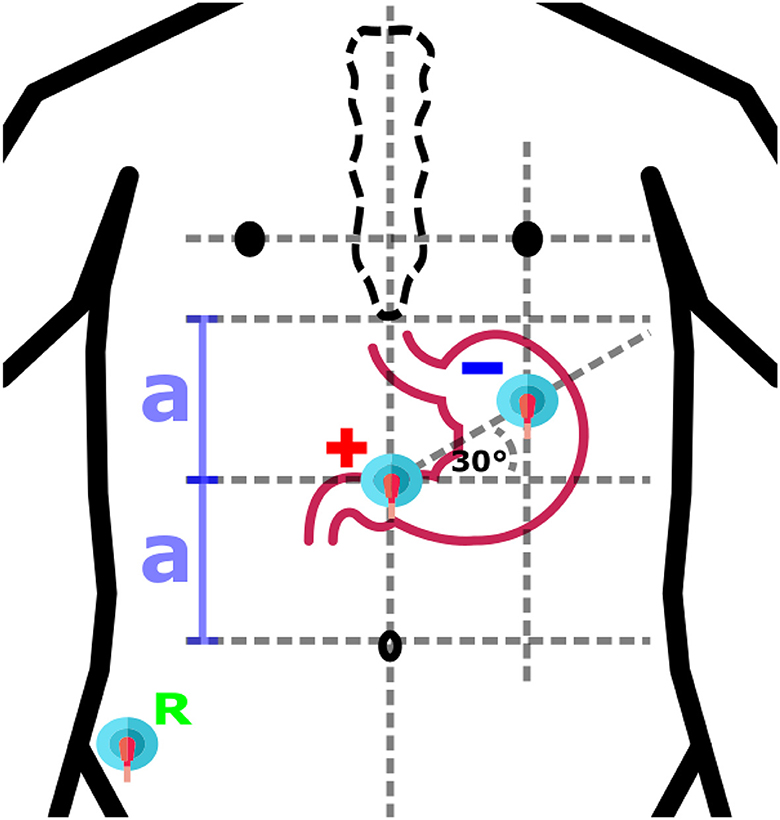
Figure 4. An example of anatomical approximation layout. A bipolar layout consisted of Positive (+), Negative (-), and Reference (R) electrodes is shown. The positive electrode is in the middle of the distance (2a) between the umbilicus and the sternum, while the negative electrode is located at the intersection of the vertical below the left nipple and the 30° line initiated from the location of the positive electrode.
5.1.2 Radiography
The stomach location could be also identified using radiography methods which may consist of ingestion or injection of contrast substance such as iodine or barium meal. An x-ray image showing exact position of the stomach in the abdominal cavity is the result of afterward X-ray examination (49, 50). The method is very precise and provides the location of the stomach of an individual subject, especially if taken shortly before actual measurement. However, the subject must undergo another examination, and moreover, he or she must be in contact with ionizing radiation, therefore it is not very suitable for use in clinical practice. An example of an X-ray image is shown in Figure 5.
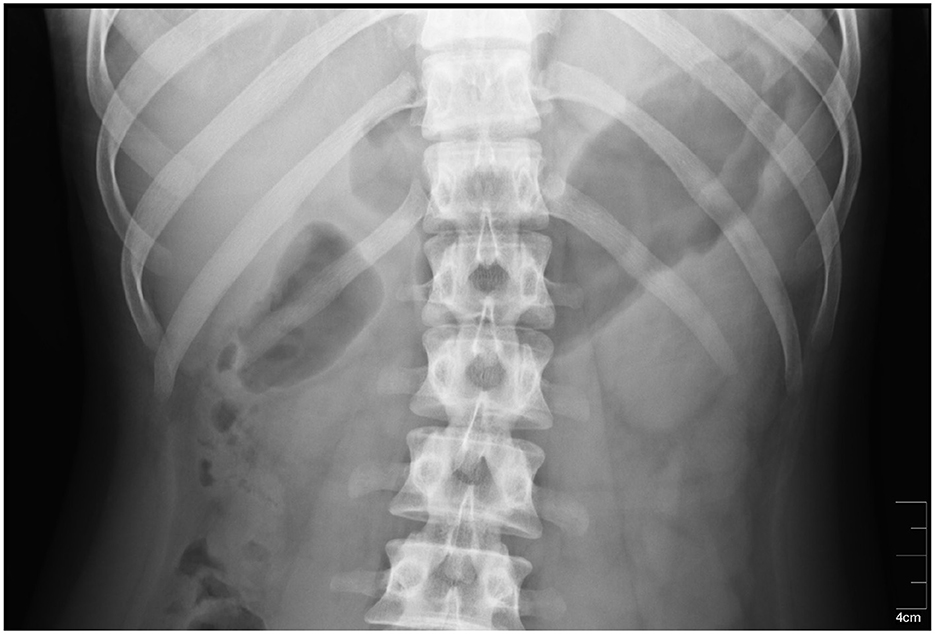
Figure 5. An X-ray image of the stomach. The stomach is located in the dark area on the right side of the radiograph (51).
5.1.3 Ultrasonography
Other possible approach could be usage of ultrasound. Ultrasonography is a fast and non-invasive method which allows specification of precise stomach location right on the spot just before the measurement is done (52–56). In comparison with the radiography, the ultrasonography is much safer and more comfortable for examined subjects. The example of an ultrasound image of the stomach is shown in Figure 6, where the stomach is visible as dark area in the middle of the image which is taken from transversal plane. The only problem is that a doctor or at least a well-trained technician is necessary to perform and evaluate such an exam, what drastically increases costs in human resources.
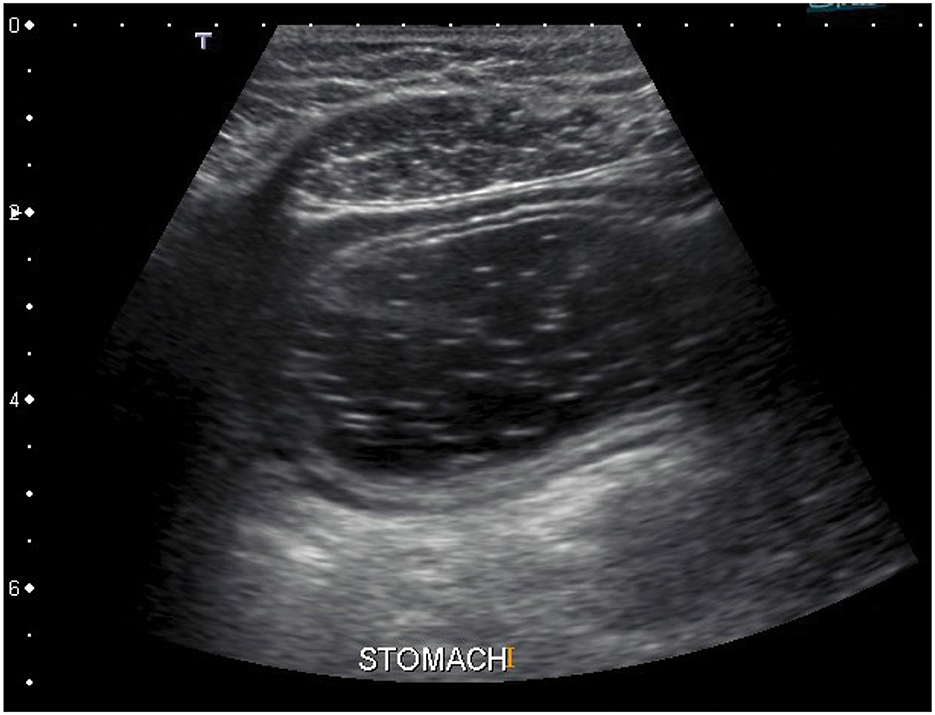
Figure 6. An ultrasound image of the stomach. The stomach is visible as dark area in the middle of the image. The image is taken from transversal plane (57).
5.1.4 Other imaging modalities
There are also alternative methods such as CT or MRI, which can accurately locate the stomach. However, in comparison to the previously described methods, they demand significantly more time, effort, and financial resources, making them economically inefficient (59–61).
5.2 Measurement apparatus
Measurement apparatus consists of electrodes that ensure direct connection better to the skin and measurement device or card performing sampling and leads providing connection between the electrodes and the device.
5.2.1 Electrodes
Historically, zinc electrodes coated with zinc sulfate (Zn/ZnS) were used for measurement of electrical activity of the stomach (1, 3). Technological advancements and inventions of new materials resulted in discovery of silver electrodes coated with silver chloride (Ag/AgCl), and nowadays, this type of electrodes is considered as primary electrodes for the measurement of bioelectric signals. The Ag/AgCl electrodes are also used for the measurement of electrical activity of the stomach (11, 13, 47, 62–64). In most cases, the adhesive floating Ag/AgCl electrodes prefilled with conductive gel are used as they are cheap and easy to use.
Before the electrodes are attached, to increase reliability of electrode contact and reduction of skin-electrode junction impedance, the skin in location of electrode placement should be shaved to remove all hair and gently abraded by sandpaper, and in order to remove grease, it should be cleaned by alcohol at the end (65–68). Measurement should not start immediately after the attachment of electrodes because of the required adaptation of electrode to be fully polarized, which could cause an artifact in other cases (26).
5.2.2 Leads
Electrical connection of leads determines the quality and type of measured signals. Signals could be acquired in unipolar (monopolar) way when electrical potentials are measured between active electrode and reference electrode (47, 64, 69–71). Another way is bipolar acquirement when electrical potentials are measured between two active electrodes with or without use of reference electrode (52, 72–75). Example of one channel bipolar electrode layout with reference electrode is shown in Figure 4. The number of leads used for measurement varies from 1 to an unspecified high number of leads required for multichannel or matrix measurement.
5.2.3 Devices
Because of missing standard for measurement, neither of the standard measuring devices is available. There are several commercial systems (Figure 7) or amplifying modules for the acquirement of electrical activity of the stomach. Such systems are from companies such as Synectics Medical, 3CPM, Biopac (Figure 8), Plux, Alimetry, and others. Many authors used commercial systems to acquire gastric signals for their research. For measurement, devices that especially constructed to acquire electrogastrogram (46, 76–79), multipurpose polygraphs (54, 80), or general bioamplifiers (34, 81, 82) are used. Many other authors were also interested in the design and creation of custom systems for measurement of electrogastrogram (10, 11, 13, 83–89). A very important parameter of measurement device is its sampling rate. Sampling rate value may vary from 1 Hz as very sufficient sampling rate regarding the frequency characteristics of signal up to 500 Hz used for high-resolution measurements of stomach electrical activity. However, a higher sampling rate leads to the acquisition of a larger amount of noise component and therefore requires more complex pre-processing (71, 72, 74, 90).
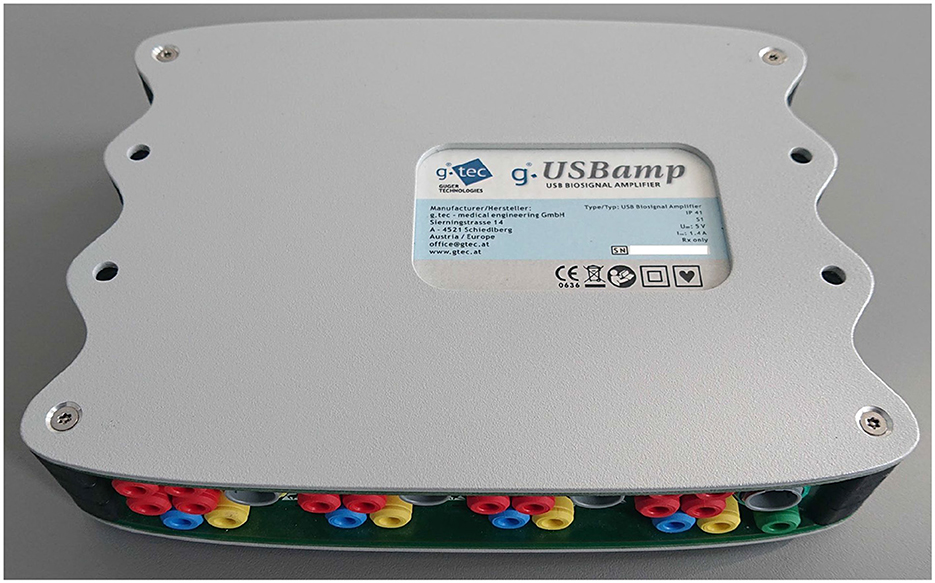
Figure 7. An example of universal bioamplifier with μV precision suitable for electrogastrography measurements (g.USBamp from g.tec) (58).
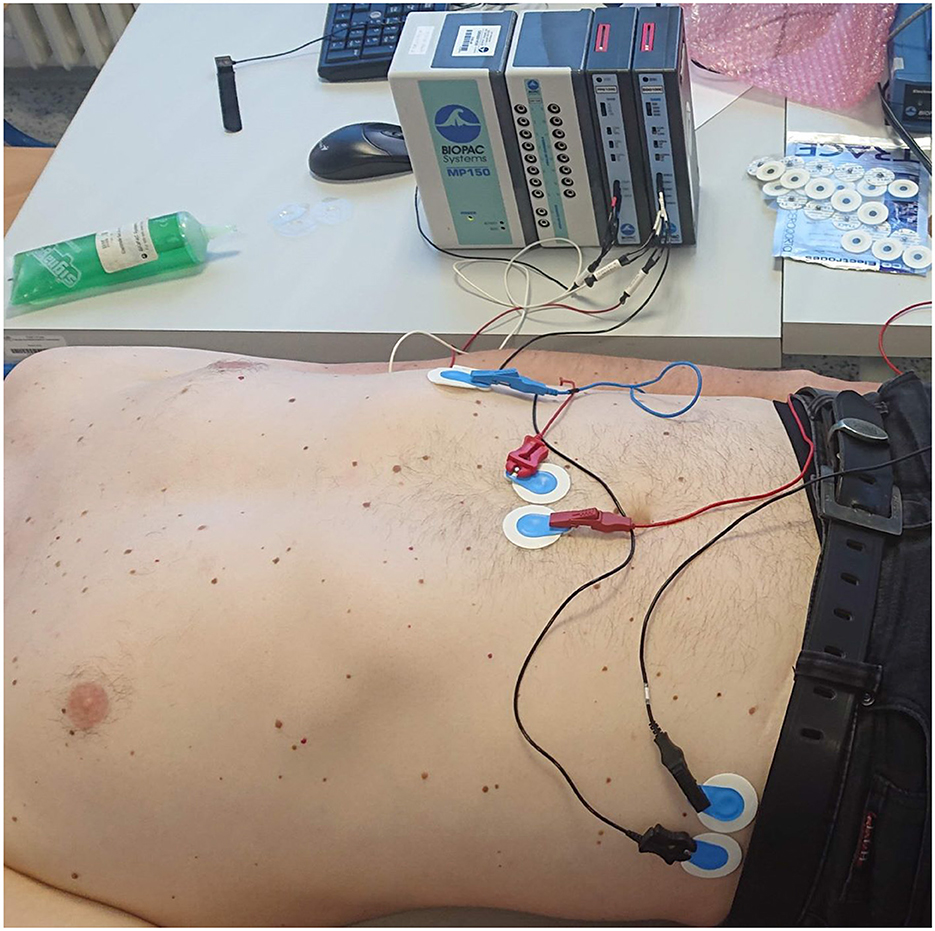
Figure 8. An example of a measuring apparatus consisting of a Biopac electrogastrography module, leads, and disposable electrodes in a comparative experiment with parallel measurement of an electrogastrograph and a universal bioamplifier (the bioamplifier is out of view).
5.2.4 Signal quality
The quality of EGG signals is paramount for accurate interpretation and subsequent clinical or research conclusions. Various studies have employed different methodologies to evaluate and compare the quality of EGG signals. A critical initial step in EGG studies is the acquisition and preprocessing of signals. The quality of EGG signals is heavily dependent on these processes. Authors often compare signal quality based on electrode placement, signal amplification, and filtering techniques. For instance, some studies emphasize the importance of standardized electrode placement on the abdomen to minimize noise and maximize signal integrity. Other researchers focus on the effectiveness of various filtering techniques, such as band-pass filters, to reduce artifacts. The presence of artifacts, such as motion artifacts and respiratory influences, can significantly degrade EGG signal quality. Comparative studies often assess the effectiveness of different noise reduction methods. Studies have mostly evaluated the efficacy of advanced signal processing techniques, such as independent component analysis (ICA) and wavelet transform, in enhancing signal quality (46, 47, 64, 77, 83, 90–93).
Quantitative analysis of EGG signals involves calculating various parameters, such as dominant frequency, power, and the percentage of normal slow waves. Authors compare these parameters to assess the quality and reliability of the EGG recordings. Ultimately, the quality of EGG signals is evaluated based on their clinical relevance and diagnostic utility. Studies often compare the sensitivity and specificity of EGG parameters in diagnosing gastrointestinal disorders. The comparison of EGG signal quality in scientific studies involves a multifaceted approach, considering signal acquisition, preprocessing, noise reduction, quantification, and clinical relevance. Despite the variability in methodologies, a growing consensus on best practices is emerging, which is driven by comparative analyses and reviews. Ensuring high-quality EGG signals is essential for accurate diagnosis and research in gastrointestinal motility disorders. The quality of the acquired signals can be also represented by the signal-to-noise ratio (SNR), which compares the level of the useful signal with its noise. However, this parameter is strongly dependent on the measurement conditions, such as ambient noise and electrode contact. Most authors did not report the actual value of the SNR, although they usually report it as poor. In experimental conditions, it is also used as the main parameter to determine the best location of the measuring electrodes (14, 55, 83, 90–93).
5.3 Measurement procedures
Measurement procedure describes the selection of subjects, the actual procedure of measurement, and the type of experimental meal ingested.
5.3.1 Subject selection
Measured subjects are selected in compliance with specific research; however, to perform statistical comparison, the control group of healthy (26, 94) and pathological group (25, 27, 95) of subjects is needed. Many authors were interested in measurement of electrical activity of the stomach in animals, especially in dogs (96–99), pigs (81, 84, 91, 100–104), and rabbits (105, 106). Measurement with human subjects was usually aimed to pathological cases of adults (107–109) or children (66, 75, 110, 111). Most observed pathology in reviewed articles was gastroparesis and pathologies that required partial or total gastrectomy, e.g., stomach cancer (18, 25, 27, 53, 95).
5.3.2 Procedure
The procedure of measurement consisted of several steps which can include ingestion of specific meal. The electrical activity of stomach is usually measured in the fasting condition (preprandial activity) first and then in the fed condition (postprandial activity). Ingested meal is used as stimuli for stomach activity, and at the same time, it is an experimental intervention required to perform statistical analysis. Many authors do measurements in both conditions with previously defined circumstances and specifically defined experimental meals (65–67, 112, 113). Because of very low frequency of gastric electrical waves, the time of measurement varies from the order of tens of minutes (50, 75, 108, 114) to few hours (94, 114–116). The stomach has a delay after ingestion of experimental meal; therefore, there should be also a pause between measurement of preprandial and postprandial activity. There were also research studies aimed to full-day measurement of electrogastrogram (9, 48, 92, 117). An example measurement procedure consists of a 60-min preprandial measurement, followed by 10 min of food consumption, a 10-min response delay, and finally a 60-min postprandial measurement.
5.3.3 Experimental diet
Experimental diet represents testing substance served to subjects as stimulus for the stomach. It could be yogurt (10, 68, 110), scrambled eggs (66, 79, 94, 118), or a type of pastry (10, 67, 119, 120). Liquids, such as water (10, 121, 122) or milk (115, 118, 123), were also examined in some research studies. However, no study defines what specific parameter of experimental diet is most significant, e.g., consistency or density. Examples of experimental diet are shown in Figure 9.
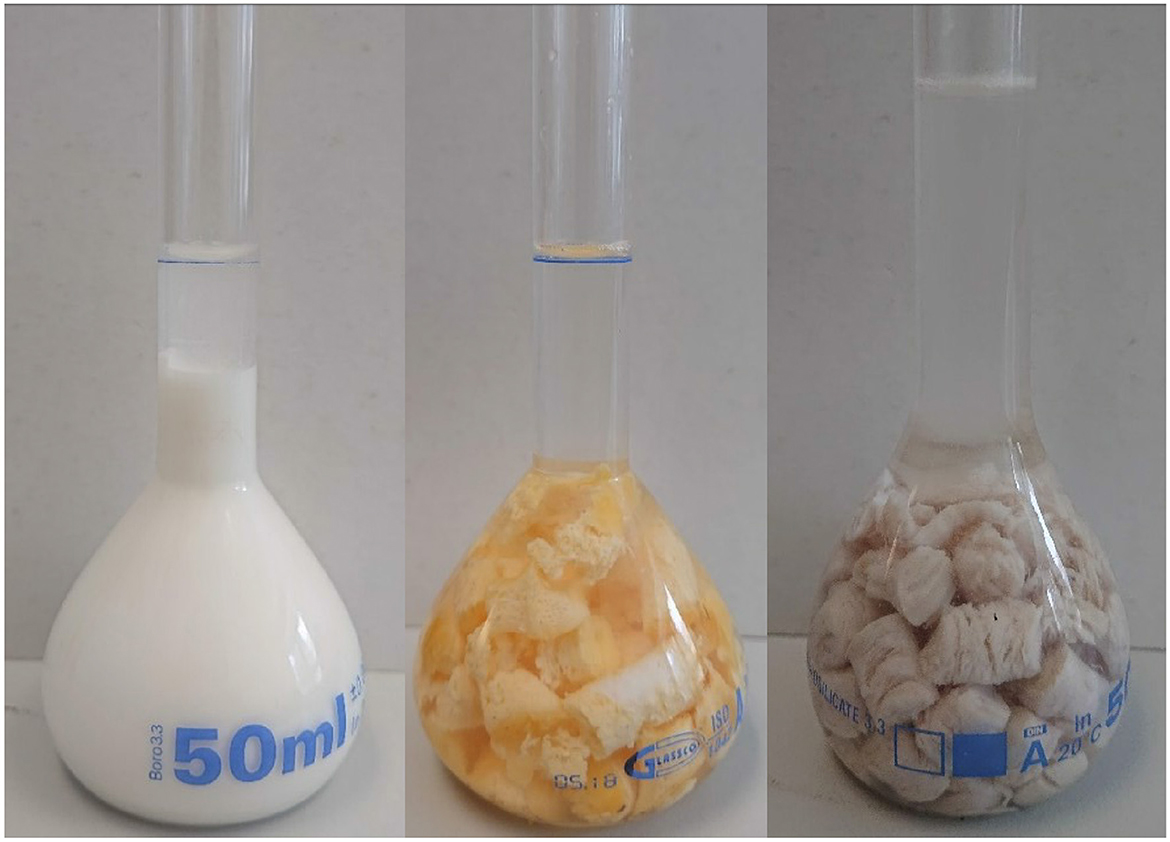
Figure 9. Examples of test meals measured for their density (cut into smaller pieces). From left: yogurt, scrambled eggs, chicken steak.
6 Methods of filtration and analysis
Analysis of measured signal requires filtration because signals contain noise and biological artifacts. One study was carried out to identify form of artifacts, to successfuly remove them (76). In general, the filtration is performed in frequency or time domain. Processed signals are then usually classified into bradygastria, normogastria, and tachygastria based on dominant frequency.
6.1 Frequency domain
Filtration in frequency domain is done using digital frequency filters which preserve only useful part of processed signal which is mainly in range between 0.5 and 9 CPM. Analysis of signal using methods based on Fourier transform usually begins with a division of signal into smaller segments which are processed resulting into chart of power spectral density which is manually evaluated by expert or automated algorithm (4, 10, 52, 68, 72, 94, 124).
6.2 Time domain
If used sampling rate is higher than few hertz, the high-frequency noise and very distinctive ECG interference are present in measured signal. In time domain, the filtration is usually based on convolutional methods. The noise can be removed from acquired signals by smoothing with moving average or median filter (72, 94). The smoothing algorithms are usually just initial phase of filtration process. To remove the ECG noise, the Wavelet transform is a more complex and much more efficient filtration method if wavelet is selected correctly, e.g., Morlet (34, 46) or Daubechies (58, 125, 126) wavelets.
6.3 Other approaches
In few cases, the machine learning algorithms, such as neural networks, were also used to process signals (11, 93, 127). Other methods such as Empirical Mode Decomposition, Hilbert-Huang transform, or Independent Component Analysis are used (119, 121, 128).
7 Comparative analysis of EGG procedures
Electrogastrography is a method known for long time, and many authors contributed to its development. Even if there is no firmly defined medical standard for its measurement, there is a common protocol which most authors follow. In this review, the comparison between electrode placement, leads, devices, measured procedures, and filtration and analysis methods is conducted. The aim of these comparisons is to describe and depict mostly used approaches for defined category and help future researchers to pick a suitable method.
Unlike other review articles, this study provides a comprehensive and technically oriented description of the utilized devices, the placement of electrodes, and the methods employed for signal processing. All of this information is primarily presented in Table 2, followed by subsequent figures featuring charts that illustrate the most commonly used devices, methods, and approaches. The other review articles are mostly focused on the physiological description of the origin of gastric motility, and the technical information provided by the reviewed articles is not very detailed or highly focused on only one method (132–135).
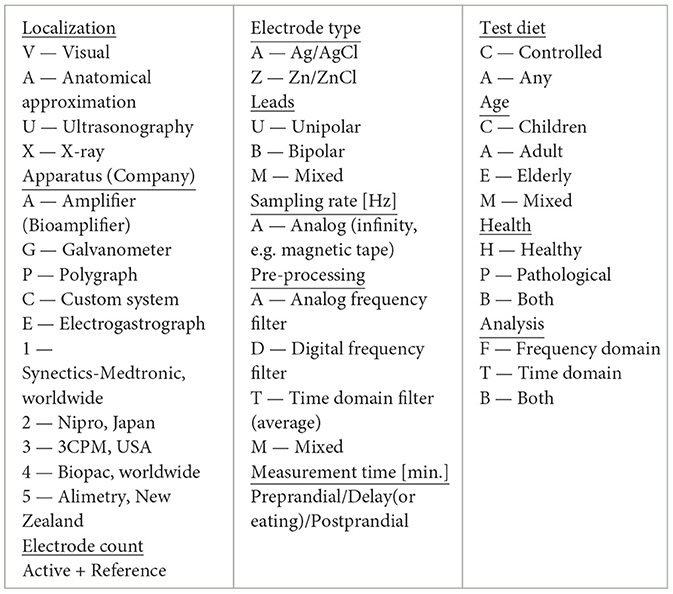
Table 3. Legend of symbols used in Table 2.
7.1 Electrode placement
First, it is necessary to find the placement of the stomach and ensure quality signal the places should be shaved, abraded, and cleaned. Then, the electrodes are placed over in a specific layout. Based on all available descriptions of actual electrode placements from reviewed authors as shown in Table 2, two heat maps were constructed. Figure 10 depicts the most used placement of active electrodes in the abdomen, and Figure 11 shows the most common locations of the reference or ground electrodes.
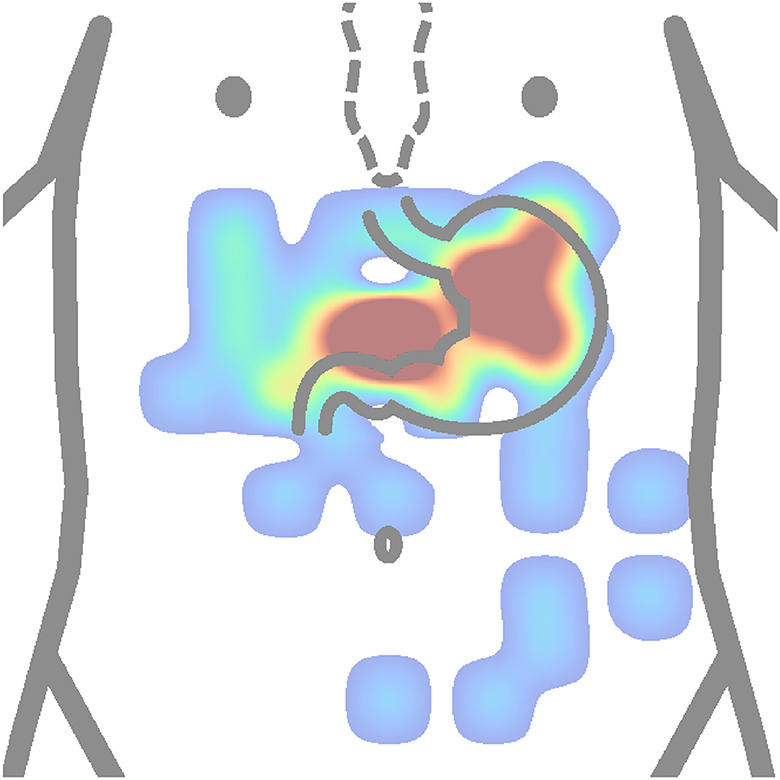
Figure 10. Heatmap (concentration map) of active electrode positions of all authors is presented in Table 2. The matrix layouts were excluded. Positions of active electrodes from layout of each author in the table were added to the same equidistant matrix and summed to produce this heatmap. Red color is high concentration, and blue color is low concentration.
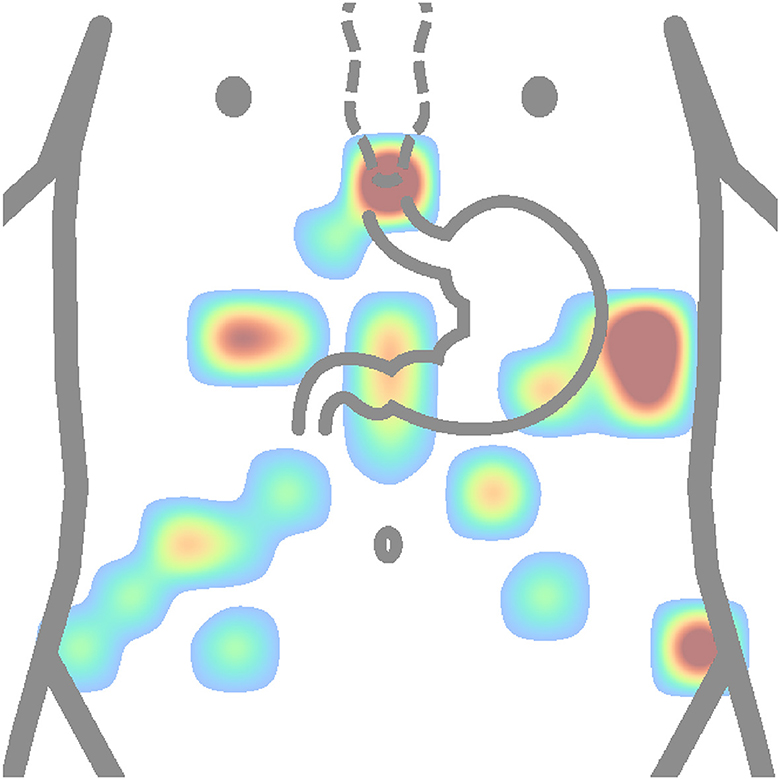
Figure 11. Heatmap (concentration map) of reference electrode positions of all authors is shown in Table 2. The matrix layouts were excluded. Positions of reference electrodes from layout of each author in the table were added to the same equidistant matrix and summed to produce this heatmap. Red color is high concentration, and blue color is low concentration.
The active electrodes placement heat map (Figure 10) shows that authors used to place electrodes mostly to proximal (cardia) and distal (pylorus) ends of the stomach. Based on anatomical approximation, these two locations can be described as the middle between the process of sternum and the navel, and the intersection of the line constructed from the first electrode location at an approximate angle of 45 ° and perpendicularly lowered from the left nipple. Other electrode placements were more or less experimental or random. It can be observed that several electrodes are placed completely outside the stomach area.
In contrast, based on Figure 11, the most used places for reference or ground electrodes are located at the process of sternum, iliac crest, and intersection of horizontal line constructed from middle point between process of sternum and the navel and left costal margin. Of the reviewed studies by all authors shown in Table 2, the most used method of electrode placement was anatomical approximation, as 79 % of the reviewed authors chose this method for their research.
7.2 Leads
Usually, only a few channels are used, but even one is enough which means that the minimum number of active electrodes is 2 for the bipolar connection method. The most used number of active electrodes is 2, which is 55 % of reviewed authors, followed by the number of 4, which was used by 23 % of reviewed authors. Nevertheless, this is mostly defined by used bioamplifier and its manufacturer. The advantage of using lower number of leads is that there are less signals to process and also measurement device can be simpler. The downside is that information may be missing that we do not currently know. Leads are usually connected in bipolar way, which was used by 60 % of reviewed authors. It is more than twice as much than unipolar way, which was used by 27 % of authors from Table 2. However, this is also very affected by selection of device, aim of study, or final analysis requirements, e.g., slow wave analysis is usually done with bipolar connection while spatial mapping requires unipolar one.
7.3 Devices
In reviewed studies, there were several types of devices used. The majority of authors, 52 %, used commercial electrogastrographs from several companies. Mostly used devices were electrogastrographs from company Synectics, one system with one bipolar channel and second with four unipolar channels. This was 62 % from all electrogastrographs used by reviewed authors, as shown in Table 2. On the other hand, these devices are relatively old and still only experimental. Sampling rate of acquirement is usually 1 Hz or 4 Hz. Both sampling rates took 27 % from all used frequencies. However, not all authors specified the sampling rate they used or the manufacturer does not state this information publicly, and this was for 16 % of reviewed studies shown in Table 2.
7.4 Measurement procedures
When electrode placement and measuring apparatus are prepared, the measurement procedure must be defined. If the 4 wholeday studies are omitted, the 81 % of reviewed studies in Table 2 were consisted of preprandial measurement, serving of test meal, and postprandial measurement. There were 6 studies based only on preprandial measurement and 2 based only on postprandial measurement. Authors whose studies were consisted of preprandial, waiting and postprandial phase spent in average 51 minutes for preprandial measurement, 7 minutes for waiting phase, and 72 minutes for postprandial measurement. The majority of authors had rules that during measurement, the subject should be in supine position and should not move or speak. The majority of the authors have used controlled diet for their measurement, and it was 68 %. On the other hand, 25 % of reviewed authors did not state what diet was used or measured only preprandially.
7.5 Subject selection
The healthy subjects were selected in most studies, as shown in Table 2. In total, 41 % of healthy subjects, 20 % of subjects with pathology, and 34 % subjects were mixed. The rest of the authors did not specify if subjects were healthy or not. The most measured subjects were adults, 63 %, followed by children with 20 %. The 9 % of subjects were mixed and 7 % were not specified.
7.6 Filtration and analysis
Signals are usually pre-processed by frequency or convolutional filters, divided into segments, and evaluated based on value of dominant frequency. Even if the medical standard is not yet defined officially, it is obvious that the procedure of reliable acquirement of electrical activity of the stomach was formed during past years anyway. Below in this chapter, the overview of all authors and their research in relation to electrogastrography are introduced. Some authors or their research studies were not included into table because they have used same system or approach already included. For each author, these parameters are listed: the method of stomach localization, type of measurement apparatus, count of electrodes, electrode type, lead connection type, sampling rate, method of signal pre-processing, time of measurement, test diet, age and health of subject, and method of analysis. In total, the 56 authors interested in non-invasive electrogastrography from 1922 to 2022 are shown in Table 2.
8 Conclusion
Over time, there were many studies aimed to electrogastrography, and in this review, the 129 articles specifically aimed to measurement systems, procedures, and analyses were selected out of total 1,322 papers from initial search. Number of electrodes is usually as low as possible, mostly approximately 3, and measurement systems used in research and clinical practice gradually changed from general amplifier to devices, specifically designed to measure exclusively electrogastrogram. Signal pre-processing is mostly conducted by analog filter which is already at the acquisition level, and after digitalization, the signals are in most cases evaluated using spectral analysis methods. However, this approach may lead to the loss of as-yet-unknown signal components, while from a research perspective, universal bioamplifiers still offer wider possibilities for signal analysis. In clinical practice, however, complete electrogastrographs are of course more suitable. In most cases, experimental studies aimed to compare between pathological and control groups. The majority of studies were interested in examination of subjects from age group of adults or children. The test meal is usually carefully controlled and prepared, but most studies did not wait for the gastric response to the ingested meal and measured immediately, which could affect the quality of postprandial signals. Studies with a high sampling rate required a more complex filtering process than studies with a lower one due to the presence of higher frequency noise. On the other hand, studies with a lower sampling frequency did not need such complex pre-processing, but the information hidden in the higher frequency component could be lost. Currently, measurement devices are readily available, and processing methods are sufficiently efficient to obtain a quality signal that can be analyzed for the purpose of diagnosing specific pathologies in the human stomach. With the current possibilities and trends, there will likely be an expansion of wearable devices capable of measuring EGG over long-time period. With such a significant amount of acquired data, there will be an opportunity to process the data easily using artificial intelligence methods without the necessity of fully understanding the signal.
Data availability statement
The raw data supporting the conclusions of this article will be made available by the authors, without undue reservation.
Author contributions
DO: Formal analysis, Methodology, Software, Writing – original draft. MA: Data curation, Resources, Supervision, Writing – original draft. MP: Formal analysis, Funding acquisition, Resources, Visualization, Writing – review & editing. JK: Conceptualization, Resources, Validation, Writing – original draft.
Funding
The author(s) declare financial support was received for the research, authorship, and/or publication of this article. The study and the contributions were supported by the project SP2024/071 “Biomedical Engineering systems XX”. This article has been produced with the financial support of the European Union under the LERCO CZ.10.03.01/00/22_003/0000003 project via the Operational Programme Just Transition. This publication has been produced with the support of the Integrated Infrastructure Operational Program for the project: Systemic Public Research Infrastructure—Biobank for Cancer and Rare diseases, ITMS: 313011AFG5, co-financed by the European Regional Development Fund.
Conflict of interest
The authors declare that the research was conducted in the absence of any commercial or financial relationships that could be construed as a potential conflict of interest.
Publisher's note
All claims expressed in this article are solely those of the authors and do not necessarily represent those of their affiliated organizations, or those of the publisher, the editors and the reviewers. Any product that may be evaluated in this article, or claim that may be made by its manufacturer, is not guaranteed or endorsed by the publisher.
References
1. Alvarez WC. The electrogastrogram and what it shows. JAMA. (1922) 78:1116–9. doi: 10.1001/jama.1922.02640680020008
2. Smout AJ, van der Schee EJ, Grashuis JL. What Is measured in electrogastrography? Dig Dis Sci. (1980) 25:179–87. doi: 10.1007/BF01308136
3. Alvarez WC. Action currents in stomach and intestine. Am J Physiol.-Legacy Content. (1922) 58:476–93. doi: 10.1152/ajplegacy.1922.58.3.476
4. Popović NB, Miljković N, Stojmenova K, Jakus G, Prodanov M, Sodnik J. Lessons learned: gastric motility assessment during driving simulation. Sensors. (2019) 19:3175. doi: 10.3390/s19143175
5. Waluga M, Jonderko K, Domoslawska E, Matwiejszyn A, Dzielicki M, Krusiec-Swidergol B, et al. Effects of taste stimulation on gastric myoelectrical activity and autonomic balance. Saudi J Gastroenterol. (2018) 24:100–8. doi: 10.4103/sjg.SJG_419_17
6. Ma R, Zhang H. EGG signals recognition based on LMD and relevance vector machine. Revista Ibérica de Sistemas e Tecnologias de Informaçao. (2016) E9:141–9.
7. Seligman WH, Low DA, Asahina M, Mathias CJ. Abnormal gastric myoelectrical activity in postural tachycardia syndrome. Clini Auton Res. (2013) 23:73–80. doi: 10.1007/s10286-012-0185-3
8. Konkka I, Punkkinen J, Pikkarainen P, Helenius KP, Turjanmaa V. Alterations in electric activity in stomach in diabetic patients suffering from nephropathy. In: The 1st International Conference on Bioelectromagnetism. Tampere: Medical & Biological Engineering & Computing (1996). p. 1.
9. Gharibans AA, Smarr BL, Kunkel DC, Kriegsfeld LJ, Mousa HM, Coleman TP. Artifact rejection methodology enables continuous, noninvasive measurement of gastric myoelectric activity in ambulatory subjects. Sci Rep. (2018) 8:5019. doi: 10.1038/s41598-018-23302-9
10. Komorowski D, EGG. DWPack: system for multi-channel electrogastrographic signals recording and analysis. J Med Syst. (2018) 42:201. doi: 10.1007/s10916-018-1035-1
11. Haddab S, Laghrouche M. Microcontroller -based system for electrogastrography monitoring through wireless transmission. Measurem Sci Rev. (2009) 9:122–6. doi: 10.2478/v10048-009-0022-6
12. Pietraszek S, Komorowski D. The simultaneous recording and analysis both EGG and HRV signals. In: 2009 Annual International Conference of the IEEE Engineering in Medicine and Biology Society, Vols 1-20. New York: IEEE (2009). p. 396.
13. Gopu G, Neelaveni R, Porkumaran K. Acquisition and Analysis of Electrogastrogram for Digestive System Disorders Using a Novel Approach. New York: IEEE. (2008).
14. Chang FY, Lee CT, Lee SD, Jang HC, Tsai DS, Fu SE. An assembled electrogastrographic device to examine the meal effect on gastric slow wave. J Gastroenterol Hepatol. (1996) 11:506–10. doi: 10.1111/j.1440-1746.1996.tb00299.x
15. Bruijs P, Vanderschee E, Smout A, Akkermans L, Vanstrien H. Bedside system for cutaneous recording and analysis of gastric myoelectrical and impedance signals. Med Biol Eng Comp. (1991) 29:609–15. doi: 10.1007/BF02446106
16. Contreras TJ, Mayen MA, Servin A, Garay LI. System for acquisition and analysis of multichannel electrogastrogram. In: 2010 7th International Conference on Electrical Engineering Computing Science and Automatic Control. Tuxtla Gutierrez, Mexico: IEEE (2010). p. 220–224.
17. Kim CH, Malagelada JR. Electrical activity of the stomach: clinical implications. Mayo Clini Proc. (1986) 61:205–10. doi: 10.1016/S0025-6196(12)61851-5
18. Krusiec-ŚwidergołB, Jonderko K. Multichannel electrogastrography under a magnifying glass - an in-depth study on reproducibility of fed state electrogastrograms. Neurogastroenterol Motil. (2008) 20:625–34. doi: 10.1111/j.1365-2982.2008.01087.x
19. el-Sharkawy TY, Morgan KG, Szurszewski JH. Intracellular electrical activity of canine and human gastric smooth muscle. J Physiol. (1978) 279:291–307. doi: 10.1113/jphysiol.1978.sp012345
20. Rhee PL, Lee JY, Son HJ, Kim JJ, Rhee JC, Kim S, et al. Analysis of pacemaker activity in the human stomach. J Physiol. (2011) 589:6105–18. doi: 10.1113/jphysiol.2011.217497
21. Lee HT, Hennig GW, Fleming NW, Keef KD, Spencer NJ, Ward SM, et al. Septal interstitial cells of Cajal conduct pacemaker activity to excite muscle bundles in human jejunum. Gastroenterology. (2007) 133:907–17. doi: 10.1053/j.gastro.2007.06.024
22. Suzuki H, Kito Y, Hashitani H, Nakamura E. Factors modifying the frequency of spontaneous activity in gastric muscle. J Physiol. (2006) 576:667–74. doi: 10.1113/jphysiol.2006.117093
23. Hamilton JW, Bellahsene BE, Reichelderfer M, Webster JG, Bass P. Human electrogastrograms: comparison of surface and mucosal recordings. Dig Dis Sci. (1986) 31:33–9. doi: 10.1007/BF01347907
24. Chen JD, Schirmer BD, McCallum RW. Serosal and cutaneous recordings of gastric myoelectrical activity in patients with gastroparesis. Am J Physiol-Gastrointest Liver Physiol. (1994) 266:G90–8. doi: 10.1152/ajpgi.1994.266.1.G90
25. Kwong NK, Brown BH, Whittaker GE, Duthie HL. Electrical activity of the gastric antrum in man. Br J Surg. (1970) 57:913–6. doi: 10.1002/bjs.1800571211
26. Brown BH, Smallwood RH, Duthie HL, Stoddard CJ. Intestinal smooth muscle electrical potentials recorded from surface electrodes. Med Biolog Eng. (1975) 13:97–103. doi: 10.1007/BF02478194
27. Geldof H, van der Schee EJ, van Blankenstein M, Grashuis JL. Electrogastrographic study of gastric myoelectrical activity in patients with unexplained nausea and vomiting. Gut. (1986) 27:799–808. doi: 10.1136/gut.27.7.799
29. Monges H, Salducci J. A method of recording the gastric electrical activity in man. Am J Dig Dis. (1970) 15:271–6. doi: 10.1007/BF02233459
30. You CH, Lee KY, Chey WY, Menguy R. Electrogastrographic study of patients with unexplained nausea, bloating, and vomiting. Gastroenterology. (1980) 79:311–4. doi: 10.1016/0016-5085(80)90147-X
31. Hinder RA, Kelly KA. Human Gastric Pacesetter Potential. Site of origin, spread, and response to gastric transection and proximal gastric vagotomy. Am J Surg. (1977) 133:29–33. doi: 10.1016/0002-9610(77)90187-8
32. Bellahsene BE, Hamilton JW, Webster JG, Bass P, Reichelderfer M. An improved method for recording and analyzing the electrical activity of the human stomach. IEEE Trans Biomed Eng. (1985) 32:911–5. doi: 10.1109/TBME.1985.325623
33. Gopu G, Neelaveni R, Pokumaran K, Shekar M. An enhanced technique for recording and analysis of electrogastrogram using active electrodes. Sri Lanka J Bio-Med Informat. (2010) 1:21. doi: 10.4038/sljbmi.v1i1.1482
34. Komorowski D, Pietraszek S. The use of continuous wavelet transform based on the fast fourier transform in the analysis of multi-channel electrogastrography recordings. J Med Syst. (2016) 40:10. doi: 10.1007/s10916-015-0358-4
35. Kito Y, Suzuki H. Electrophysiological properties of gastric pacemaker potentials. J Smooth Muscle Res. (2003) 39:163–73. doi: 10.1540/jsmr.39.163
36. Hirst G, Beckett E, Sanders K, Ward S. Regional variation in contribution of myenteric and intramuscular interstitial cells of Cajal to generation of slow waves in mouse gastric antrum. J Physiol. (2002) 540:1003–12. doi: 10.1113/jphysiol.2001.013672
37. Camborova P, Hubka P, Sulkova I, Hulin I. The pacemaker activity of interstitial cells of Cajal and gastric electrical activity. Physiol Res. (2003) 52:275–84. doi: 10.33549/physiolres.930269
38. Zhu MH, Kim TW, Ro S, Yan W, Ward SM, Koh SD, et al. A Ca(2+)-activated Cl(-) conductance in interstitial cells of cajal linked to slow wave currents and pacemaker activity. J Physiol. (2009) 587:4905–18. doi: 10.1113/jphysiol.2009.176206
39. Berridge MJ. Smooth muscle cell calcium activation mechanisms. J Physiol. (2008) 586:5047–61. doi: 10.1113/jphysiol.2008.160440
40. Hirst GDS, Ward SM. Interstitial cells: involvement in rhythmicity and neural control of gut smooth muscle. J Physiol. (2003) 550:337–46. doi: 10.1113/jphysiol.2003.043299
41. Tack J. Gastric motor and sensory function. Curr Opin Gastroenterol. (2009) 25:557–65. doi: 10.1097/MOG.0b013e328331b5ad
42. Rosen JM, Saps M. Gastric motor disorders. In:Faure C, Thapar N, Di Lorenzo C, , editors. Pediatric Neurogastroenterology. Cham: Springer International Publishing (2017). p. 261–71.
43. Waluga M, Kasicka-Jonderko A, Dzielicki M. Kamińska M, Bo zek M, Laskowska J, et al. Resistance of postprandial gastric functions and autonomic balance to taste stimulation. J Smooth Musc Res. (2021) 57:68–78. doi: 10.1540/jsmr.57.68
44. Chen J, McCallum RW. Electrogastrography: measuremnt, analysis and prospective applications. Med Biol Eng Comp. (1991) 29:339–50. doi: 10.1007/BF02441653
45. Kohno N, Nomura M, Okamoto H, Kaji M, Ito S. The use of electrogastrography and external ultrasonography to evaluate gastric motility in crohn's disease. J Med Investigat. (2006) 53:277–84. doi: 10.2152/jmi.53.277
46. Dirgenali F, Kara S, Okkesim S. Estimation of wavelet and short-time fourier transform sonograms of normal and diabetic subjects' electrogastrogram. Comput Biol Med. (2006) 36:1289–302. doi: 10.1016/j.compbiomed.2005.07.005
47. Frasko R, Maruna P, Gurlich R, Trca S. Transcutaneous electrogastrography in patients with ileus. Eur Surg Res. (2008) 41:197–202. doi: 10.1159/000134918
48. Kaiho T, Shimoyama I, Nakajima Y, Ochiai T. Gastric and non-gastric signals in electrogastrography. J Auton Nerv Syst. (2000) 79:60–6. doi: 10.1016/S0165-1838(99)00098-3
49. Nguyen HN, Silny J. Wüller S, Marschall HU, Rau G, Matern S. Abnormal postprandial duodenal chyme transport in patients with long standing insulin dependent diabetes mellitus. Gut. (1997) 41:624–31. doi: 10.1136/gut.41.5.624
50. Shah N, Rodriguez M, Louis DS, Lindley K, Milla PJ. Feeding difficulties and foregut dysmotility in noonan's syndrome. Arch Dis Child. (1999) 81:28–31. doi: 10.1136/adc.81.1.28
52. Patterson M, Rintala R, Lloyd D, Abernethy L, Houghton D, Williams J. Validation of electrode placement in neonatal electrogastrography. Dig Dis Sci. (2001) 46:2245–9. doi: 10.1023/A:1011931502985
53. Franzese A, Borrelli O, Corrado G, Rea P, Di Nardo G, Grandinetti AL, et al. Domperidone is more effective than cisapride in children with diabetic gastroparesis. Aliment Pharmac Therap. (2002) 16:951–7. doi: 10.1046/j.1365-2036.2002.01240.x
54. Cucchiara S, Riezzo G, Minella R, Pezzolla F, Giorgio I, Auricchio S. Electrogastrography in nonulcer dyspepsia. Arch Dis Child. (1992) 67:613–7. doi: 10.1136/adc.67.5.613
55. Ohtaki M, Yagi M, Kubota M, Homma S. A Disturbance of the gastric myoelectric activity in post-operative patients with biliary atresia. Pediatr Surg Int. (2004) 20:77–82. doi: 10.1007/s00383-003-1091-5
56. Homma S, Shimakage N, Yagi M, Hasegawa J, Sato K, Matsuo H, et al. Electrogastrography prior to and following total gastrectomy, subtotal gastrectomy, and gastric tube formation. Dig Dis Sci. (1995) 40:893–900. doi: 10.1007/BF02064997
57. Deslandes A. Sonographic demonstration of stomach pathology: reviewing the cases. Aust J Ultrasound Med. (2013) 16:202–9. doi: 10.1002/j.2205-0140.2013.tb00249.x
58. Kubicek J, Penhaker M, Oczka D, Buzga M, Augustynek M, Cerny M, et al. A proposal of optimal wavelet filter design for EGG signal decomposition based on modified ABC evolutionary optimization. In: 2019 IEEE 17th World Symposium on Applied Machine Intelligence and Informatics (SAMI). Herlany, Slovakia: IEEE (2019). p. 83–88.
59. Teramoto H, Shimizu T, Yogo H, Nishimiya Y, Hori S, Kosugi T, et al. Gastric emptying and duodenal motility upon intake of a liquid meal with monosodium glutamate in healthy subjects. Physiol Rep. (2014) 2:e00187. doi: 10.1002/phy2.187
60. Calder S, O'Grady G, Cheng LK, Du P, A. Simulated anatomically accurate investigation into the effects of biodiversity on electrogastrography. IEEE Trans Biomed Eng. (2020) 67:868–75. doi: 10.1109/TBME.2019.2922449
61. Allegra AB, Gharibans AA, Schamberg GE, Kunkel DC, Coleman TP. Bayesian inverse methods for spatiotemporal characterization of gastric electrical activity from cutaneous multi-electrode recordings. PLoS ONE. (2019) 14:e0220315. doi: 10.1371/journal.pone.0220315
62. Chien-Lin C, Hsien-Hong L, Lu-Chin H, Shih-Che H, Tso-Tsai L. Electrogastrography differentiates reflux disease with or without dyspeptic symptoms. Dig Dis Sci. (2004) 49:715–9. doi: 10.1023/B:DDAS.0000030079.20501.62
63. Siegl A, Mayr J, Huber A, Uray E. Postprandial tachygastria is frequent in infants with gastroesophageal reflux. Pediatr Surg Int. (1998) 13:569–71. doi: 10.1007/s003830050405
64. Zena-Giménez V, Garcia-Casado J, Ye-Lin Y, Garcia-Breijo E, Prats-Boluda G. A flexible multiring concentric electrode for non-invasive identification of intestinal slow waves. Sensors. (2018) 18:396. doi: 10.3390/s18020396
65. Jones MP, Shah D, Ebert CC. Effects of rabeprazole sodium on gastric emptying, electrogastrography, and fullness. Dig Dis Sci. (2003) 48:69–73. doi: 10.1023/A:1021782330906
66. Friesen CA, Lin Z, Jennifer Verril Schurman, Andre L, McCallum RW. An evaluation of adult electrogastrography criteria in healthy children. Dig Dis Sci. (2006) 51:1824–8. doi: 10.1007/s10620-006-9323-x
67. Gonlachanvit S, Chey WD, Goodman KJ, Parkman HP. Effect of meal size and test duration on gastric emptying and gastric myoelectrical activity as determined with simultaneous [C-13]octanoate breath test and electrogastrography in normal subjects using a muffin meal. Dig Dis Sci. (2001) 46:2643–50. doi: 10.1023/A:1012758925461
68. Filho JR, De Rezende JM, Da Cunha Melo JR. Electrogastrography in patients with chagas' disease. Dig Dis Sci. (2005) 50:1882–8. doi: 10.1007/s10620-005-2956-3
69. Nohara S, Iwase M, Imoto H, Sasaki N, Nakamura U, Uchizono Y, et al. Gastric emptying in patients with type 2 diabetes mellitus and diabetes associated with mitochondrial DNA 3243 mutation using 13C-octanoic acid breath test. J Diabetes Complications. (2006) 20:295–301. doi: 10.1016/j.jdiacomp.2005.07.007
70. Ohmure H, Takada H, Nagayama K, Sakiyama T, Tsubouchi H, Miyawaki S. Mastication suppresses initial gastric emptying by modulating gastric activity. J Dent Res. (2012) 91:293–8. doi: 10.1177/0022034511433847
71. Erickson JC. O'grady G, Du P, Egbuji JU, Pullan AJ, Cheng LK. Automated gastric slow wave cycle partitioning and visualization for high-resolution activation time maps. Ann Biomed Engi. (2011) 39:469–83. doi: 10.1007/s10439-010-0170-8
72. Tokumaru O, Mizumoto C, Takada Y, Tatsuno J, Ashida H. Vector analysis of electrogastrography during motion sickness. Dig Dis Sci. (2003) 48:498–507. doi: 10.1023/A:1022580431102
73. Mintchev MP, Stickel A, Bowes KL. Comparative assessment of power dynamics of gastric electrical activity. Dig Dis Sci. (1997) 42:1154–7. doi: 10.1023/A:1018829402171
74. Riezzo G, Clemente C, Leo S, Russo F. The role of electrogastrography and gastrointestinal hormones in chemotherapy-related dyspeptic symptoms. J Gastroenterol. (2005) 40:1107–15. doi: 10.1007/s00535-005-1708-7
75. C Di Lorenzo, Reddy SN, Flores AF, Hyman PE. Is electrogastrography a substitute for manometric studies in children with functional gastrointestinal disorders? Dig Dis Sci. (1997) 42:2310–6. doi: 10.1023/A:1018879020479
76. Calder S, Schamberg G, Varghese C, Waite S, Sebaratnam G, Woodhead JST, et al. An automated artifact detection and rejection system for body surface gastric mapping. J Neurogastroenterol Motil. (2022) 34:e14421. doi: 10.1111/nmo.14421
77. Nakao M, Nishikitani M, Nomura K, Karita K, Yano E. Gastric electrical activity and cardiovascular risk factors in relation to autonomic nervous function, hormonal responses, and health-related lifestyles in young men. J Gastroenterol. (2006) 41:855–61. doi: 10.1007/s00535-006-1882-2
78. Pfaffenbach B, Adamek RJ, Bartholomaus C, Wegener M. Gastric dysrhythmias and delayed gastric emptying in patients with functional dyspepsia. Dig Dis Sci. (1997) 42:2094–9. doi: 10.1023/A:1018826719628
79. Lin X, Mellow MH, Southmayd L III, Pan J, Chen JDZ. Impaired gastric myoelectrical activity in patients with chronic renal failure. Dig Dis Sci. (1997) 42:898–906. doi: 10.1023/A:1018856112765
80. Usami A, Mizukami Y, Onji M. Abnormal gastric motility in liver cirrhosis: roles of secretin. Dig Dis Sci. (1998) 43:2392–7. doi: 10.1023/A:1026613711375
81. Kvetina J, Tacheci I, Pavlik M, Kopacova M, Rejchrt S, Douda T, et al. Use of electrogastrography in preclinical studies of cholinergic and anticholinergic agents in experimental pigs. Physiol Res. (2015) 64:S647–52. doi: 10.33549/physiolres.933227
82. Alagumariappan P, Krishnamurthy K, Kandiah S, Ponnuswamy MJ. Effect of electrode contact area on the information content of the recorded electrogastrograms: an analysis based on Renyi entropy and Teager-Kaiser energy. Polish J Med Phys Eng. (2017) 23:37–42. doi: 10.1515/pjmpe-2017-0007
83. Guo X, Wang Z, He F, Qi H, Chen L, Li C, et al. A high-precision, low-cost, wireless, multi-channel electrogastrography system. Annu Int Conf IEEE Eng Med Biol Soc. (2021) 2021:6779–82. doi: 10.1109/EMBC46164.2021.9629576
84. Perley A, Roustaei M, Aguilar-Rivera M, Kunkel DC, Hsiai TK, Coleman TP, et al. Miniaturized wireless gastric pacing via inductive power transfer with non-invasive monitoring using cutaneous electrogastrography. Bioelectron Med. (2021) 7:12. doi: 10.1186/s42234-021-00074-8
85. Zaynidinov H, Makhmudjanov S, Rajabov F, Singh D. IoT-enabled mobile device for electrogastrography signal processing. In:Singh M, Kang D, Lee J, Tiwary U, Singh D, Chung W, , editors. Intelligent Human Computer Interaction, IHCI 2020 (2021). p. 346–356.
86. Morello R, Fabbiano L, Oresta P, de Capua C. Design of a non-invasive sensing system for diagnosing gastric disorders. Acta IMEKO. (2021) 10:73–9. doi: 10.21014/acta_imeko.v10i4.1175
87. Komorowski D, Pietraszek S, Grzechca D. The Wireless System for EGG Signal Acquisition. New York: IEEE. (2012). doi: 10.1109/ICECS.2012.6463724
88. McNearney T, Lin X, Shrestha J, Lisse J, Chen JDZ. Characterization of gastric myoelectrical rhythms in patients with systemic sclerosis using multichannel surface electrogastrography. Dig Dis Sci. (2002) 47:690–8. doi: 10.1023/A:1014759109982
89. Full-Young C, Ching-Liang L, Chih-Yen C, Shou-Dong L, et al. Electrogastrographic characteristics in patients of stomach cancer. Dig Dis Sci. (2001) 46:1458–65.
90. Du P, O'Grady G, Egbuji JU, Lammers WJ, Budgett D, Nielsen P, et al. High-resolution mapping of in vivo gastrointestinal slow wave activity using flexible printed circuit board electrodes: methodology and validation. Ann Biomed Eng. (2009) 37:839–46. doi: 10.1007/s10439-009-9654-9
91. Sukasem A, Calder S, Angeli-Gordon TR, Andrews CN, O'Grady G, Gharibans A, et al. In vivo experimental validation of detection of gastric slow waves using a flexible multichannel electrogastrography sensor linear array. Biomed Eng Online. (2022) 21:43. doi: 10.1186/s12938-022-01010-w
92. Lindberg G, Iwarzon M, Hammarlund B. 24-hour ambulatory electrogastrography in healthy volunteers. Scand J Gastroenterol. (1996) 31:658–64. doi: 10.3109/00365529609009146
93. Agrusa AS, Gharibans AA, Allegra AA, Kunkel DC, Coleman TP. A deep convolutional neural network approach to classify normal and abnormal gastric slow wave initiation from the high resolution electrogastrogram. IEEE Trans Biomed Eng. (2020) 67:854–67. doi: 10.1109/TBME.2019.2922235
94. Simonian HP, Panganamamula K, Parkman HP, Xu XH, Chen JDZ, Lindberg G, et al. Multichannel electrogastrography (EGG) in normal subjects: a multicenter study. Dig Dis Sci. (2004) 49:594–601. doi: 10.1023/B:DDAS.0000026304.83214.50
95. Simonian HP, Panganamamula K, Chen JZ, Fisher RS, Parkman HP. Multichannel electrogastrography (EGG) in symptomatic patients: a single center study. Am J Gastroenterol. (2004) 99:478–85. doi: 10.1111/j.1572-0241.2004.04103.x
96. Atanassova E, Daskalov I, Dotsinsky I, Christov I, Atanassova A. Non-invasive electrogastrography. Part 2 human electrogastrogram. Arch Physiol Biochem. (1995) 103:436–41. doi: 10.3109/13813459509047135
97. Koenig JB, Martin CEW, Dobson H, Mintchev MP. Use of multichannel electrogastrography for noninvasive assessment of gastric myoelectrical activity in dogs. Am J Vet Res. (2009) 70:11–5. doi: 10.2460/ajvr.70.1.11
98. Xu XH, Wang ZS, Hayes J, Chen JDZ. Is there a one-to-one correlation between gastric emptying of liquids and gastric myoelectrical or motor activity in dogs? Dig Dis Sci. (2002) 47:365–72. doi: 10.1023/a:1013726223531
99. Mintchev MP, Girard A, Bowes KL. Nonlinear adaptive noise compensation in electrogastrograms recorded from healthy dogs. IEEE Trans Biomed Eng. (2000) 47:239–48. doi: 10.1109/10.821769
100. Salimi-Jazi F, Thomas AL, Rafeeqi T, Diyaolu M, Wood LSY, Axelrod S, et al. Gastrointestinal myoelectric measurements via simultaneous external and internal electrodes in pigs. J Surg Res. (2022) 279:119–26. doi: 10.1016/j.jss.2022.05.012
101. Calder S, Cheng LK, Andrews CN, Paskaranandavadivel N, Waite S, Alighaleh S, et al. Validation of noninvasive body-surface gastric mapping for detecting gastric slow-wave spatiotemporal features by simultaneous serosal mapping in porcine. Am J Physiol Gastrointest Liver Physiol. (2022) 323:G295–305. doi: 10.1152/ajpgi.00049.2022
102. Chan CHA, Aghababaie Z, Paskaranandavadivel N, Avci R, Cheng LK, Angeli-Gordon TR. Localized gastric distension disrupts slow-wave entrainment leading to temporary ectopic propagation: a high-resolution electrical mapping study. Am J Physiol Gastrointest Liver Physiol. (2021) 321:G656–67. doi: 10.1152/ajpgi.00219.2021
103. Tacheci I, Kvetina J, Kunes M, Pavlik M, Kopacova M, Cerny V, et al. The effect of general anaesthesia on gastric myoelectric activity in experimental pigs. BMC Gastroenterol. (2013) 13:48. doi: 10.1186/1471-230X-13-48
104. Kvetina J, Varayil J, Ali S, Kunes M, Bures J. Tachecí I, et al. Preclinical electrogastrography in experimental pigs. Interdiscip Toxicol. (2010) 3:53. doi: 10.2478/v10102-010-0011-5
105. Fiantoro T, Eveline L. Single Channel Local White Rabbit (Oryctolagus Cuniculus) Cutaneous Electrogastrography and Its Electrogastrogram Classification Algorithm. (2014).
106. Fiantoro T, Susanto A, Winduratna B. Gastric Slow Wave Modelling Based on Stomach Morphology and Neuronal Firings. (2014).
107. Chen DD, Xu X, Zhao Q, Yin J, Sallam H, Chen JDZ. Gastroenterology - effects of audio stimulation on gastric myoelectrical activity and sympathovagal balance in healthy adolescents and adults. J Gastroenterol Hepatol. (2008) 23:141–9. doi: 10.1111/j.1440-1746.2007.05123.x
108. Orr WC, Zhang M, Mcclanahan J, Sloan S, Chen JDZ. Gastric myoelectric activity in older adults treated with cisapride for gastro-oesophageal reflux disease. Alimen Pharmacol Therapeut. (2000) 14:337–43. doi: 10.1046/j.1365-2036.2000.00716.x
109. Atanassova E, Daskalov I, Dotsinsky I, Christov I, Atanassova A. Non-invasive electrogastrography. Part 1: correlation between the gastric electrical activity in dogs with implanted and cutaneous electrodes. Arch Physiol. (1995) 103:431–5. doi: 10.3109/13813459509047134
110. Chen JDZ, Lin X, Zhang M, Torres-Pinedo RB, Orr WC. Gastric myoelectrical activity in healthy children and children with functional dyspepsia. Dig Dis Sci. (1998) 43:2384–91.
111. Borrelli O, Salvia G, Mancini V, Santoro L, Tagliente F, Romeo EF, et al. Evolution of gastric electrical features and gastric emptying in children with duchenne and becker muscular dystrophy. Am J Gastroenterol. (2005) 100:695–702. doi: 10.1111/j.1572-0241.2005.41303.x
112. Parkman HP, Trate DM, Knight LC, Brown KL, Maurer AH, Fisher RS. Cholinergic effects on human gastric motility. Gut. (1999) 45:346. doi: 10.1136/gut.45.3.346
113. Mathur R, Pimentel M, Sam CL, De Z. Chen J, et al. Postprandial improvement of gastric dysrhythmias in patients with type ii diabetes: identification of responders and nonresponders. Dig Dis Sci. (2001) 46:705–12. doi: 10.1023/A:1010736528276
114. Parkman HP, Urbain JL, Knight LC, Brown KL, Trate DM, Miller MA, et al. Effect of gastric acid suppressants on human gastric motility. Gut. (1998) 42:243–50. doi: 10.1136/gut.42.2.243
115. Riezzo G, Castellana RM, De Bellis T, Laforgia F, Indrio F, Chiloiro M. Gastric electrical activity in normal neonates during the first year of life: effect of feeding with breast milk and formula. J Gastroenterol. (2003) 38:836–43. doi: 10.1007/s00535-003-1158-z
116. Dibaise JK, Park FL, Lyden E, Brand RE, Brand RM. Effects of low doses of erythromycin on the 13c spirulina platensis gastric emptying breath test and electrogastrogram: a controlled study in healthy volunteers. Am J Gastroenterol. (2001) 96:2041–50. doi: 10.1111/j.1572-0241.2001.03937.x
117. Kirjavainen J, Lehtonen L, Kirjavainen T, Kero P. 24-hour ambulatory sleep polygraphy study. Sleep of excessively crying infants: a 24-hour ambulatory sleep polygraphy study. Pediatrics. (2004) 114:592–600. doi: 10.1542/peds.2003-0651-L
118. Vargas-Luna FM, Huerta-Franco MR, Schurman JV, Deacy AD, Bagherian A, Harvey L, et al. Electrogastrographic and autonomic nervous system responses to solid and liquid meals in youth with functional dyspepsia. Neurogastroenterol Motil. (2020) 32:13785. doi: 10.1111/nmo.13785
119. Mika BT, Tkacz EJ. Assessment of slow wave propagation in different phases of food stimulation in the multichannel electrogastrographic signal (EGG). In:Pietka E, Badura P, Kawa J, Wieclawek W, , editors. Information Technologies in Medicine, ITIB. (2016). Berlin: Springer-Verlag Berlin (2016). p. 325–39.
120. Porter JA, MacKenzie KE, Darlow BA, Butler R, Day AS. Gastric emptying in children with type 1 diabetes mellitus: a pilot study. J Paediatr Child Health. (2019) 55:416–20. doi: 10.1111/jpc.14215
121. Tkacz EJ, Mika BT. Both independent component and running spectrum analyses of electrogastrographic signal with the water load. test. Aust Phys Eng Sci Med. (2008) 31:490–1.
122. Friesen CA, Lin Z, Schurman JV, Andre L, McCallum RW. Autonomic nervous system response to a solid meal and water loading in healthy children: its relation to gastric myoelectrical activity. Neurogastroenterol Motil. (2007) 19:376–82. doi: 10.1111/j.1365-2982.2007.00906.x
123. Chen J, McCallum RW. Effect of milk on myoelectrical activity of the stomach-an electrogastrographic study. Med Biol Eng Comp. (1992) 30:564–7. doi: 10.1007/BF02457839
124. Bonapace ES, Parkman HP, Fisher RS. Edrophonium provocative testing during electrogastrography (EGG): effects on dyspeptic symptoms and the EGG. Dig Dis Sci. (1998) 43:1494–500. doi: 10.1023/A:1018806729894
125. Sikkandar M, Muniyandi M, Chakravarthy S. Effect of gastric myoelectric activity on photoplethysmographic signals. In: SHORT PAPER International Journal of Recent Trends in Engineering. (2009). p. 2.
126. Al Kafee A, Akan A. Analysis of gastric myoelectrical activity from the electrogastrogram signals based on wavelet transform and line length feature. In: Proc Institut Mech Eng Part H: J Eng Med. (2018) 232:403–11. doi: 10.1177/0954411918757812
127. Gandhi C, Ahmad S, Mehbodniya A, Webber J, Hemalatha S, Elwahsh H, et al. Biosensor-assisted method for abdominal syndrome classification using machine learning algorithm. Comp Intellig Neurosci. (2022). 2022:4454226 doi: 10.1155/2022/4454226
128. Komorowski D, Mika B. Gastric slow wave rhythm identification using new approach based on noise-assisted multivariate empirical mode decomposition and hilbert-huang transform. Neurogastroenterol Motil. (2021) 33:e13997. doi: 10.1111/nmo.13997
129. Levanon D, Zhang M, Orr WC, Chen JD. Effects of meal volume and composition on gastric myoelectrical activity. Am J Physiol. (1998) 274:G430–434. doi: 10.1152/ajpgi.1998.274.2.G430
130. Katoh K, Nomura M, Iga A, Hiasa A, Uehara K, Harada K, et al. Comparison of gastric peristalsis inhibition by scopolamine butylbromide and glucagon: evaluation by electrogastrography and analysis of heart rate variability. J Gastroenterol. (2003) 38:629–35. doi: 10.1007/s00535-003-1114-y
131. Wang PT, Wellington J, Koch KL. Clinical features and gastric myoelectrical activity in patients with idiopathic and post-surgical rapid gastric emptying who present with unexplained chronic nausea. Neurogastroenterol Motil. (2021) 33:e13988. doi: 10.1111/nmo.13988
132. Wang Y, Chen JDZ, Nojkov B. Diagnostic methods for evaluation of gastric motility—mini review. Diagnostics. (2023) 13:803. doi: 10.3390/diagnostics13040803
133. Riezzo G, Russo F, Indrio F. Electrogastrography in adults and children: the strength, pitfalls, and clinical significance of the cutaneous recording of the gastric electrical activity. BioMed Res Int. (2013) 2013:282757. doi: 10.1155/2013/282757
134. Abell TL, Malagelada JR. Electrogastrography: current assessment and future perspectives. Dig Dis Sci. (1988) 33:982–92. doi: 10.1007/BF01535995
Keywords: electrogastrography, non-invasive method, measurement systems, electrode placement, measurement apparatus, signal processing
Citation: Oczka D, Augustynek M, Penhaker M and Kubicek J (2024) Electrogastrography measurement systems and analysis methods used in clinical practice and research: comprehensive review. Front. Med. 11:1369753. doi: 10.3389/fmed.2024.1369753
Received: 19 January 2024; Accepted: 03 June 2024;
Published: 01 July 2024.
Edited by:
Huan Tong, Sichuan University, ChinaReviewed by:
Recep Avci, The University of Auckland, New ZealandFrancisco Miguel Vargas-Luna, University of Guanajuato, Mexico
Maria-Raquel Huerta-Franco, University of Guanajuato, Mexico
Copyright © 2024 Oczka, Augustynek, Penhaker and Kubicek. This is an open-access article distributed under the terms of the Creative Commons Attribution License (CC BY). The use, distribution or reproduction in other forums is permitted, provided the original author(s) and the copyright owner(s) are credited and that the original publication in this journal is cited, in accordance with accepted academic practice. No use, distribution or reproduction is permitted which does not comply with these terms.
*Correspondence: Jan Kubicek, amFuLmt1YmljZWtAdnNiLmN6