- 1Department of Anatomy and Molecular Embryology, Medical Faculty, Ruhr University Bochum, Bochum, Germany
- 2Division of Histology and Embryology, International Joint Laboratory for Embryonic Development and Prenatal Medicine, Medical College, Jinan University, Guangzhou, China
- 3School of Stomatology, Southwest Medical University, Guangzhou, China
- 4School of Stomatology, Jinan University, Guangzhou, China
- 5Clinical Research Center, Clifford Hospital, Guangzhou, China
Background: Millions of people across the globe are affected by conditions like Amyotrophic Lateral Sclerosis (ALS), Parkinson’s Disease (PD), Multiple Sclerosis (MS), Spinal Cord Injury (SCI), and Traumatic Brain Injury (TBI), although most occurrences are common in the elderly population. This systematic review aims to highlight the safety of the procedures, their tolerability, and efficacy of the available therapies conducted over the years using mesenchymal stem cells (MSCs) in treating the neurological conditions mentioned above.
Methods: PubMed was used to search for published data from clinical trials performed using mesenchymal stem cells. Studies that provided the necessary information that mentioned the efficacy and adverse effects of the treatment in patients were considered for this review.
Results: In total, 43 manuscripts were selected after a strategic search, and these studies have been included in this systematic review. Most included studies reported the safety of the procedures used and the treatment’s good tolerability, with mild adverse events such as fever, headache, mild pain at the injection site, or nausea being common. A few studies also reported death of some patients, attributed to the progression of the disease to severe stages before the treatment. Other severe events, such as respiratory or urinary infections reported in some studies, were not related to the treatment. Different parameters were used to evaluate the efficacy of the treatment based on the clinical condition of the patient.
Conclusion: Mesenchymal stem cells transplantation has so far proven to be safe and tolerable in select studies and patient types. This systematic review includes the results from the 43 selected studies in terms of safety and tolerability of the procedures, and several adverse events and therapeutic benefits during the follow-up period after administration of MSCs.
1 Introduction
Cell-based therapies refer to the therapeutic injection of cellular material into individuals. These therapies utilize various cells, including stem cells, which are effective in treating degenerative diseases, blood cancers, and bone marrow disorders (1). Many published and ongoing clinical trials have investigated the use of cell therapy for neurological disorders such as Amyotrophic Lateral Sclerosis (ALS), Parkinson’s Disease (PD), Multiple Sclerosis (MS), Spinal Cord Injury (SCI), and Traumatic Brain Injury (TBI). These trials utilized cells obtained from either the patients themselves (autologous cells) or healthy donors’ bone marrow and peripheral blood (2). The use of autologous cells minimizes the risk of rejection associated with using donor cells. Mesenchymal Stem Cells (MSCs), non-hematopoietic stromal cells that primarily reside in the bone marrow but also occur in fat and other tissues, offer a promising therapeutic option for neurological disorders (3). Upon administration, MSCs migrate to the damaged neural tissue, secrete various cytotropic factors that intervene in the pathomechanisms of those diseases, provoke transdifferentiation of stem cells into neurons, and promote neurogenesis (4). Moreover, MSCs support hematopoiesis and assist in generating mesodermal lineage cells (5).
Alzheimer’s disease, also known as AD, is a neurodegenerative disorder that is the most common cause of dementia. Given AD’s multifaceted pathomechanisms, therapies focusing solely on a single aspect, such as amyloid-beta (Aβ) or tau, often fall short in demonstrating the efficacy of existing disease-modifying treatments (6). Consequently, adopting multi-target approaches could be more beneficial in managing this age-related neurodegenerative disorder than relying on single-target strategies (7).
For ALS, available treatments currently focus more on mitigating symptoms than on achieving a cure for the condition. ALS is a severe motor neuron disease that impairs the brain and spinal cord nerves responsible for movement, resulting in muscle weakness, shrinkage, and difficulties with speaking, swallowing, and breathing. While acute respiratory failure is uncommon, ALS’s severity is underscored by a mortality rate where 50% of patients pass away within 3 years of symptoms appearing; however, about 10% may survive beyond a decade (8). The Food and Drug Administration (FDA) has approved two drugs for ALS, Riluzole and Edaravone, for ALS management. These medications have demonstrated limited success in slowing disease progression and slightly prolonging patients’ lives (9).
Multiple sclerosis is a chronic inflammatory disease that affects the central nervous system (CNS), causing progressive damage to the myelin sheath that surrounds nerve fibers. This inflammation, persisting from months to years, results in a variety of debilitating symptoms such as motor, sensory, and cognitive impairments, which can culminate in disability, severe complications, and a significantly reduced quality of life (10). Current treatments for MS, including steroids, drugs for modifying the disease and drugs for targeting specific symptoms that may help in reduction of the frequency of exacerbations and slow the progression of the disease. However, there remains no effective strategy for repairing damage by regenerating myelin or neurons (11).
Current treatments for PD are primarily limited to managing symptoms without altering the disease’s progression. PD is a progressive neurodegenerative disease which occurs due to the degeneration of dopaminergic nigrostriatal neurons. Symptoms such as tremors, stiffness, slow movement, and imbalance characterize the early stages. As PD advances, other symptoms may also develop, such as motor impairment, dystonia, along with several other non-motor symptoms (12). Consequently, in the early phase, the rate of clinical decline is rapid, with a decrease of approximately eight to ten points on the Unified Parkinson Disease Rating Scale (UPDRS) in the first year (13).
Methylprednisolone is currently the only agent recognized for its effectiveness in treating SCI. Research has shown that it can reduce axonal damage caused by secondary injury processes (14). However, its use is constrained by a narrow treatment window of 8 h, modest effectiveness, and a significant risk of complications associated with high-dose corticosteroid therapy. SCI is a devastating neurological disorder. It may result in paralysis leading to functional deficit and clinical dependency (15). Currently available treatment strategies include drug therapy, surgical procedures, and rehabilitation training. Despite these advances, the improvements they provide are limited. Recent research has deepened our understanding of SCI’s molecular dynamics, yet discovering truly effective treatments continues to be difficult (16).
Recently, there has been a substantial improvement in the prehospital and intensive care facilities of patients with TBI, and there has also been development in the evidence-based guidelines for management of these facilities (17). TBI represents a significant global public health issue, notably leading to coma and disability among children and young adults (18). Severe TBI often results in disturbed consciousness and motor disorders, with prognosis remaining generally poor. Most patients show recovery within 6 months post-injury. However, a gradual improvement is possible over the following 12–18 months. The mortality rate for acute severe TBI is as high as 36%. Even under the best circumstances, 15% of patients will suffer severe disability, 20% will have moderate disability, and only 25% will make a complete recovery (19).
Adult-derived MSCs have been extensively studied in neurological diseases. These cells have several advantages over other stem cells, including ease of collection, high availability, and ease of culture; lower immunogenicity which allows the possibility of allotransplant, immunomodulation, no oncogenic transformation, and limited ethical concerns (20). The most dominant sources of MSCs are autologous or allogeneic bone marrow and adipose tissue, but they can also be isolated from other tissues, such as placenta, umbilical cord, and peripheral blood (21). Adipose tissues can be obtained by visceral or subcutaneous aspiration or excision of the fat tissue from the abdomen, femoral, brachium or gluteal areas of the patients (22). These methods include enzymatic digestion of the samples, red blood cell (RBC) removal with specific RBC lysis followed by cell filtration (23). Systemic injection of MSCs has been shown to have several limitations in clinical trials, including low cell survival and poor distribution in the CNS, but there are several encouraging trials as well (24). Alternative administration routes are being investigated to overcome these obstacles.
Due to the growing interest in stem cell applications for neurological disorders, this systematic review was performed to investigate safety, tolerability, efficacy, and related adverse effects of MSC therapies based on the data published in the literature.
2 Methods
This systematic review was conducted following the Preferred Reporting Items for Systematic Reviews and Meta-Analyses (PRISMA) guidelines (25).
2.1 Search strategy
The electronic database PubMed was used for this systematic review as it contains most of the published clinical trials related to the title of this systematic review. Clinicaltrials.gov was not used because most of the clinical trials were either ongoing, or still recruiting or were unpublished. The following keywords and combinations were used, and final selection was done on 30th of June 2023:
(Neurodegenerative disorders OR Dementia OR Alzheimer’s Disease OR Vascular Dementia OR Lewy body Dementia OR Frontotemporal Dementia OR Huntington’s Disease OR Traumatic Brain Injury OR Creutzfeldt-Jakob Disease OR Parkinson’s Disease OR Spinal cord injury OR Multiple Sclerosis OR Amyotrophic Lateral Sclerosis) AND (Mesenchymal Stem Cells) (The full search strategy is provided in Supplementary material 5).
2.2 Eligibility, inclusion and exclusion criteria
Experimental studies or clinical trials with information on the effects of mesenchymal stem cell therapies in patients with conditions like AD, ALS, PD, MS, SCI, and TBI met the eligibility criteria. The following records were eliminated from the selection process: books and documents, reviews, meta-analysis, systematic reviews, animal studies and other topics such as studies that did not use MSC therapies in the treatment of the chosen neurological conditions. After reading whole text of the selected articles, only focus on MSC therapies were included.
Reviewers (Xuesong Yang, Gaurav Patel, and Lichao Liu) independently assessed the records screened. After agreement, full text articles of every potentially eligible and relevant studies were downloaded. After reading whole text of the selected articles, only focus on mesenchymal stem cells therapies were included. Gaurav Patel and Lichao Liu extracted data from the selected studies and presented the extracted content in tables. This method allowed us to simultaneously screen eligible manuscripts and incorporate manuscripts that meet the criteria into this systematic review.
2.3 Data extraction
Information about the names of the authors, year of publication, country, type of study, follow-up period, characteristics of the participants, source of MSCs, administration route, improved condition, adverse events and clinical discussion were collected independently by the reviewers (Gaurav Patel and Lichao Liu) from the original reports. Our primary focus is on the improved condition of relevant diseases following MSC therapies, with the improved condition typically measured through corresponding assessment scales. For example, in the treatment of ALS, the improvement in patients after treatment is measured by the Revised ALS Functional Rating Scale. The evaluation of the condition of the patients, whether improvement or deterioration, was made using clinical indicators that were relevant to the conditions described in the selected studies; These parameters were chosen to measure the efficacy outcomes of these conditions. The patients in these clinical studies ranged in age from 18 to 80. For those studies that did not report relevant information, we assumed that they followed standard clinical practices in the industry.
2.4 Risk of bias assessment
We used the standard Cochrane Collaboration tool for evaluation of the risks of bias within the included studies (26). Based on this tool, the methodological quality of the included trials was evaluated and reviewed by two authors (Gaurav Patel and Lichao Liu).
3 Results
3.1 Design and samples
In this systematic review, the initial search of databases yielded 3,597 results. After the first screening, 3,519 results were excluded (books and documents, meta-analysis, reviews, and systematic reviews). After further evaluation of the titles and abstracts of the remaining 78 articles, we excluded 25 articles that were not published in English, did not involve MSC therapies, or were animal studies. The remaining 53 eligible articles were further analyzed by reviewers using standardized review forms. The research in these 43 articles involved the safety and/or efficacy of MSCs for the treatment of neurological diseases. Figure 1 shows the number of studies for each neurological conditions, included in this systematic review and the geographical distribution of the selected published studies. Few papers consisted of trials on multiple neurological conditions. Flow chart of the review process in presented in Figure 1.
3.2 Studies characteristics
43 studies were included in this systematic review, and their principal characteristics are presented in Table 1. All of the selected studies were clinical trials. The phases of these trials varied, with 30 being phase I, seven being phase II, and six being phase I/II. Although all the studies focused on the use of MSCs to treat neurological conditions, the sources of MSCs and the routes of administration varied. The sources of MSCs used in the 43 studies were as follows: 30 studies used autologous bone marrow-derived MSCs, five studies used autologous adipose-derived MSCs, six studies used autologous or allogeneic umbilical cord-derived MSCs, and one study used MSCs derived from placenta and peripheral blood. The routes of administration of MSCs varied. Six studies used intravenous (IV) infusion, 11 used intrathecal administration, three used both, and 13 studies injected MSCs into the spinal cord using lumbar puncture or direct injection at the injury site. Some studies also administered the cells via face and nasal cavity, intracerebroventricular route or cerebral arteries, and intraparenchymal, endovascular or intracisternal transplantation. The period of follow-up also varied, most of the studies followed-up for at least 6–12 months, with the shortest follow-up period being just 14 days and two studies had follow-up period of 7 and 9 years.
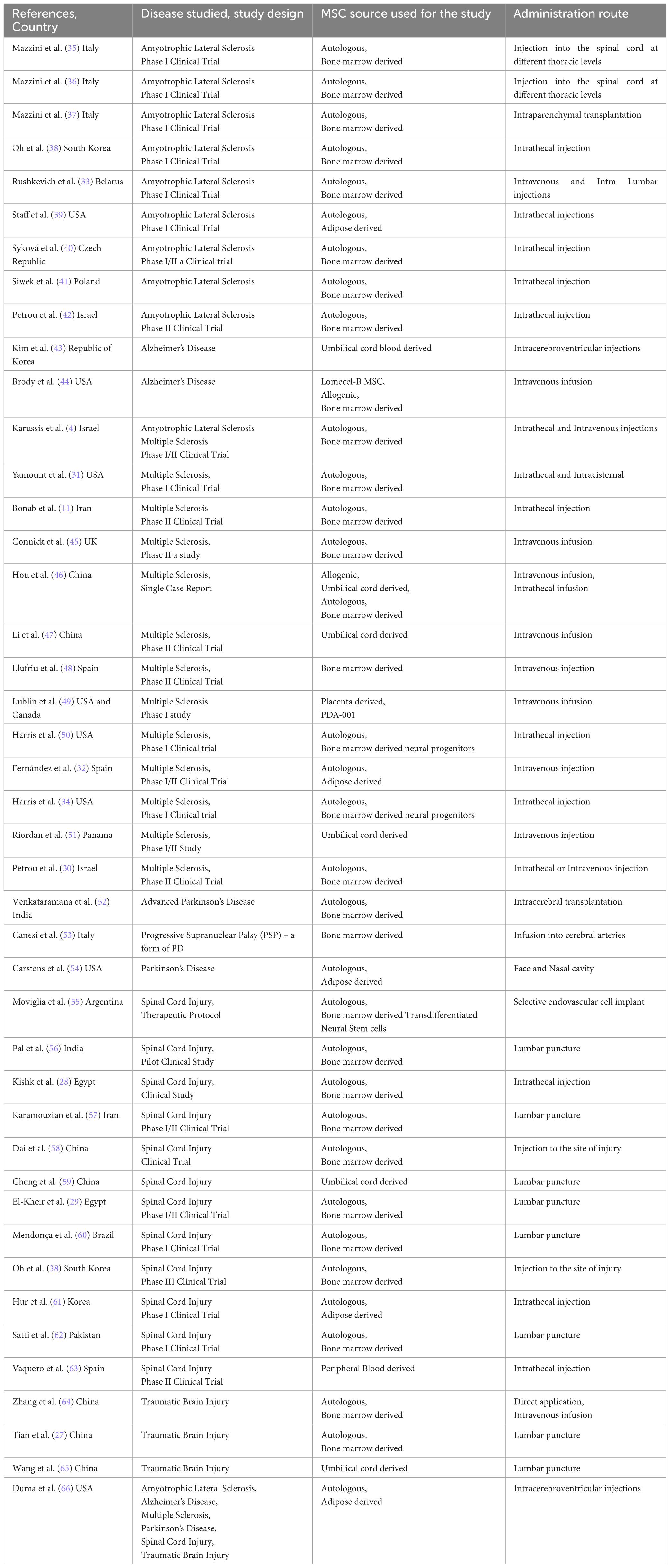
Table 1. Author, year and country of the published study, the type of disease studied and its design, source of MSC used for the study and its route of administration into the patients who participated in the study, number of patients and their characteristics like gender and age.
3.3 Main outcomes
The relevant information statistics of the research papers included in this systematic review are shown in Figure 2, which categorizes them by disease type (A), country of origin (B), and publication year (C). The studies involved different types of disease, sources of mesenchymal stem cells, and patient numbers and ages. This review hence focused on two primary outcomes: adverse reactions after treatment and changes in clinical parameters for evaluating treatment efficacy for specific diseases.
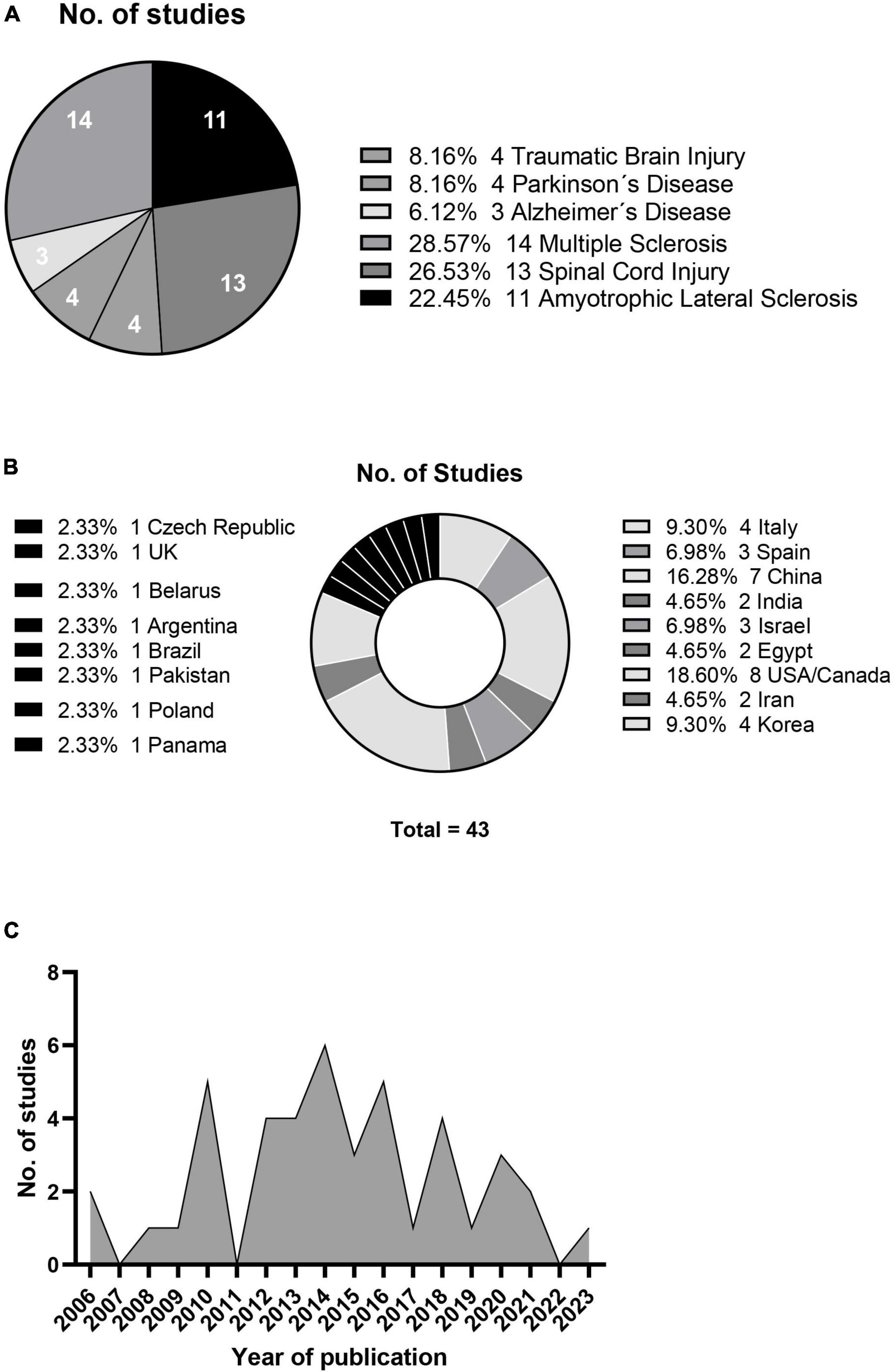
Figure 2. Neurological condition studies (A), geographical distribution (B) and annual publication count (C).
In the studies that reported adverse events, eight studies reported frequent and low-grade fevers after treatment, regardless of the route of administration. Seven studies reported urinary or respiratory infections which were mild and tolerated by patients. Other common adverse effects reported were back pain or pain at the site of injection, which subsided in few hours or days. These common adverse effects were reported in 15 of the included studies while seven studies reported no adverse effects.
Three studies showed 100% improvement rate, with all their patients showing clinical improvements, but the sample size for those studies were relatively low, 10 or below. This is the reason why these studies requested for larger sample size for better evaluation results. Two of these studies had only two participants for PD and SCI. Thirteen other studies had improvement rate higher than or equal to 50%, but the limitation remained the same, of low sample size. One study on MS had only one participant whose improvement based on any clinical parameter, or score was not mentioned in the manuscript.
None of the studies had higher sample size, The highest sample size was 97 for a study related to TBI (27), 64 and 70 for SCI (28, 29) and 48 for MS (30), with improvement rates being 39%, 41% and 46% and 48% respectively.
Eight studies out of 43 included in this review showed no improvement, and 13 studies had no mention of any improvement scores due to their focus only on the safe administration of the cells and tolerance by the patients. The above results are summarized in Table 2.

Table 2. Author, year and country of the published study, the type of disease studied and its design, period of follow-up of the participants in the study, the percentage of improved patients, the adverse events that occurred in patients after treatment during the study as observed and reported during the follow-up period and the clinical discussions of the respective study.
3.4 Risk of bias assessment
A summary of the risk of bias assessment for each study is shown in Supplementary material. When more than five of the seven assessment criteria are rated as low risk, the study is considered to have an overall low risk of bias. Therefore, studies classified with a low risk of bias include: Five on ALS (S1), one on AD (S2), six on MS (S2), two on PD (S3), five on SCI (S3 and S4), and two on TBI (S4).
4 Discussion
This systematic review explored the increasing interest and significance of MSC therapies on some of the serious neurological conditions like AD, ALS, MS, PD, SCI and TBI that affect millions of people worldwide (1). MSCs are a promising stem cell type with the advantages of pluripotency, immunomodulatory properties, and low immunogenicity (3). This systematic review primarily investigated the safety of the procedures used in the studies, adverse effects after treatment, and efficacy of MSC therapy for the above-mentioned conditions.
Many of the included studies had limitations, such as small sample sizes and short follow-up periods. In certain cases, with only one or two participants, assessing the treatment’s efficacy proved challenging. There were some serious adverse effects and even deaths, most were not attributed to the treatment itself, as described by the authors. Minor adverse effects in most of the cases were either pain in the site of injection or headache or nausea, which were mild and subsided soon. These minor adverse events reported can be deemed negligible if the treatment resulted in better efficacy rate. Nonetheless, a significant concern remains regarding the potential for long-term adverse events, given the short follow-up periods in many studies.
Autologous stem cells, unlike allogeneic stem cells from donors, do not pose the risk of immunogenic reactions or transmission of other diseases. Notably, none of the studies mentioned in this systematic review reported any rejection of cells by the patients after treatment. The studies that used allogenic MSCs did not report any improvement in individual patients, but an overall improvement was reported. It was not clearly confirmed if the improvement shown was due to allogenic cells or natural recovery. Hence, further studies need to be performed to validate the efficacy of use of allogenic MSCs.
This systematic review primarily investigates the efficacy and safety of MSC therapies, but the optimal administration route for MSCs in patients is also worth discussing. Some studies have suggested that IV infusion may be as effective as other administration routes (30–32). Other studies have suggested that IV infusion may reduce the number of cells that can migrate to the CNS. Therefore, intrathecal administration may be a more efficacious route of delivery (33, 34). However, due to the limited number of studies using the intrathecal route and the small number of participants in those studies, encouraging this route will be difficult until a sufficient number of successful studies adequate sample size are recorded. Autologous bone marrow-derived MSCs (BM-MSCs) were the most prevalent type of cells in the selected studies. These cells can be harvested from adult bone marrow with ease and safety. There is considerable variation in patient ages and stages of disease progression among the studies, with specific details available in Tables 1, 2, making it impossible to generalize these outcomes to other patients. The heterogeneity of research methods used in different studies makes it challenging to draw definitive conclusions. Although the search strategy included two databases, PubMed and Clinicaltrials.gov, results from only PubMed were selected for further eligibility, inclusion and exclusion process because most of the clinical trials resulted from Clinicaltrials.gov search were either ongoing, or still recruiting or were unpublished. Inclusion of studies only from one database in this case could be a possible limitation of this systematic review.
5 Conclusion
This systematic review focused on the cell therapies based on transplantation of MSCs to patients suffering from deadly neurological disorders likes AD, ALS, MS, PD, SCI and TBI. MSCs transplantation is generally considered to be a safe and well-tolerated therapy, according to the findings of most studies included in this systematic review. However, stronger evidence in the results are still needed to know the exact efficacy and potency of this therapy. In order for these treatments to be considered for clinical practice and benefit millions of patients, future research should focus on larger-scale clinical trials and longer follow-up periods.
Data availability statement
The original contributions presented in this study are included in the article/Supplementary material, further inquiries can be directed to the corresponding authors.
Author contributions
GP: Conceptualization, Investigation, Writing – original draft. LL: Formal analysis, Visualization, Writing – original draft. C-CS: Methodology, Writing – original draft. AL: Data curation, Methodology, Writing – original draft. Y-HY: Data curation, Methodology, Writing – original draft. BB-S: Conceptualization, Supervision, Writing – review and editing. XY: Conceptualization, Methodology, Supervision, Writing – review and editing.
Funding
The author(s) declare that no financial support was received for the research, authorship, and/or publication of this article.
Conflict of interest
The authors declare that the research was conducted in the absence of any commercial or financial relationships that could be construed as a potential conflict of interest.
Publisher’s note
All claims expressed in this article are solely those of the authors and do not necessarily represent those of their affiliated organizations, or those of the publisher, the editors and the reviewers. Any product that may be evaluated in this article, or claim that may be made by its manufacturer, is not guaranteed or endorsed by the publisher.
Supplementary material
The Supplementary Material for this article can be found online at: https://www.frontiersin.org/articles/10.3389/fmed.2024.1361723/full#supplementary-material
References
1. Zakrzewski W, Dobrzyński M, Szymonowicz M, Rybak Z. Stem cells: Past, present, and future. Stem Cell Res Ther. (2019) 10:68.
2. Alessandrini M, Preynat-Seauve O, De Bruin K, Pepper M. Stem cell therapy for neurological disorders. S Afr Med J. (2019) 109:70–7.
3. Naji A, Eitoku M, Favier B, Deschaseaux F, Rouas-Freiss N, Suganuma N. Biological functions of mesenchymal stem cells and clinical implications. Cell Mol Life Sci. (2019) 76:3323–48.
4. Karussis D, Karageorgiou C, Vaknin-Dembinsky A, Gowda-Kurkalli B, Gomori J, Kassis I, et al. Safety and immunological effects of mesenchymal stem cell transplantation in patients with multiple sclerosis and amyotrophic lateral sclerosis. Arch Neurol. (2010) 67:1187–94. doi: 10.1001/archneurol.2010.248
5. Lennon D, Caplan A. Isolation of human marrow-derived mesenchymal stem cells. Exp Hematol. (2006) 34:1604–5.
6. Kent S, Spires-Jones T, Durrant C. The physiological roles of tau and Aβ: Implications for Alzheimer’s disease pathology and therapeutics. Acta Neuropathol. (2020) 140:417–47.
7. Duncan T, Valenzuela M. Alzheimer’s disease, dementia, and stem cell therapy. Stem Cell Res Ther. (2017) 8:111.
8. Niedermeyer S, Murn M, Choi P. Respiratory failure in amyotrophic lateral sclerosis. Chest. (2019) 155:401–8.
9. Dash R, Babu R, Srinivas N. Two decades-long journey from riluzole to edaravone: Revisiting the clinical pharmacokinetics of the only two amyotrophic lateral sclerosis therapeutics. Clin Pharmacokinet. (2018) 57:1385–98. doi: 10.1007/s40262-018-0655-4
10. Ghasemi N, Razavi S, Nikzad E. Multiple sclerosis: Pathogenesis, symptoms, diagnoses and cell-based therapy. Cell J. (2017) 19:1–10.
11. Bonab M, Sahraian M, Aghsaie A, Karvigh S, Hosseinian S, Nikbin B, et al. Autologous mesenchymal stem cell therapy in progressive multiple sclerosis: An open label study. Curr Stem Cell Res Ther. (2012) 7:407–14.
12. Lang A, Obeso J. Time to move beyond nigrostriatal dopamine deficiency in Parkinson’s disease. Ann Neurol. (2004) 55:761–5. doi: 10.1002/ana.20102
13. Fahn S, Oakes D, Shoulson I, Kieburtz K, Rudolph A, Lang A, et al. Levodopa and the progression of Parkinson’s disease. N Engl J Med. (2004) 351:2498–508.
14. Sandhu M, Gray E, Kocherginsky M, Jayaraman A, Mitchell G, Rymer W. Prednisolone pretreatment enhances intermittent hypoxia-induced plasticity in persons with chronic incomplete spinal cord injury. Neurorehabil Neural Repair. (2019) 33:911–21. doi: 10.1177/1545968319872992
15. Rolls A, Shechter R, Schwartz M. The bright side of the glial scar in CNS repair. Nat Rev Neurosci. (2009) 10:235–41. doi: 10.1038/nrn2591
16. Dasari V, Veeravalli K, Dinh D. Mesenchymal stem cells in the treatment of spinal cord injuries: A review. World J Stem Cells. (2014) 6:120–33.
17. Curry P, Viernes D, Sharma D. Perioperative management of traumatic brain injury. Int J Crit Illness Inj Sci. (2011) 1:27–35.
18. Zink B. Traumatic brain injury outcome: Concepts for emergency care. Ann Emerg Med. (2001) 37:318–32.
19. Andreassen J, Thorsen K, Søreide K, Werner D, Weber C. Is there a weekend effect on mortality rate and outcome for moderate and severe traumatic brain injury? A population-based, observational cohort study. Brain Spine. (2022) 2:101699. doi: 10.1016/j.bas.2022.101699
20. Lukomska B, Stanaszek L, Zuba-Surma E, Legosz P, Sarzynska S, Drela K. Challenges and controversies in human mesenchymal stem cell therapy. Stem Cells Int. (2019) 2019:9628536.
21. Zheng Y, Huang C, Liu F, Lin H, Yang X, Zhang Z. Comparison of the neuronal differentiation abilities of bone marrow-derived and adipose tissue-derived mesenchymal stem cells. Mol Med Rep. (2017) 16:3877–86.
22. Varghese J, Griffin M, Mosahebi A, Butler P. Systematic review of patient factors affecting adipose stem cell viability and function: Implications for regenerative therapy. Stem Cell Res Ther. (2017) 8:45. doi: 10.1186/s13287-017-0483-8
23. Beyer Nardi N, da Silva Meirelles L. Mesenchymal stem cells: Isolation, in vitro expansion and characterization. Handb Exp Pharmacol. (2006) 174:249–82.
24. Isaković J, Šerer K, Barišić B, Mitrečić D. Mesenchymal stem cell therapy for neurological disorders: The light or the dark side of the force? Front Bioeng Biotechnol. (2023) 11:1139359. doi: 10.3389/fbioe.2023.1139359
25. Page M, McKenzie J, Bossuyt P, Boutron I, Hoffmann T, Mulrow C, et al. The PRISMA 2020 statement: An updated guideline for reporting systematic reviews. BMJ. (2021) 372:n71.
26. Higgins J, Altman D, Gøtzsche P, Jüni P, Moher D, Oxman A, et al. The Cochrane collaboration’s tool for assessing risk of bias in randomised trials. BMJ. (2011) 343:d5928.
27. Tian C, Wang X, Wang X, Wang L, Wang X, Wu S, et al. Autologous bone marrow mesenchymal stem cell therapy in the subacute stage of traumatic brain injury by lumbar puncture. Exp Clin Transplant. (2013) 11:176–81. doi: 10.6002/ect.2012.0053
28. Kishk N, Gabr H, Hamdy S, Afifi L, Abokresha N, Mahmoud H, et al. Case control series of intrathecal autologous bone marrow mesenchymal stem cell therapy for chronic spinal cord injury. Neurorehabil Neural Repair. (2010) 24:702–8. doi: 10.1177/1545968310369801
29. El-Kheir W, Gabr H, Awad M, Ghannam O, Barakat Y, Farghali H, et al. Autologous bone marrow-derived cell therapy combined with physical therapy induces functional improvement in chronic spinal cord injury patients. Cell Transplant. (2014) 23:729–45. doi: 10.3727/096368913X664540
30. Petrou P, Kassis I, Levin N, Paul F, Backner Y, Benoliel T, et al. Beneficial effects of autologous mesenchymal stem cell transplantation in active progressive multiple sclerosis. Brain J Neurol. (2020) 143:3574–88. doi: 10.1093/brain/awaa333
31. Yamout B, Hourani R, Salti H, Barada W, El-Hajj T, Al-Kutoubi A, et al. Bone marrow mesenchymal stem cell transplantation in patients with multiple sclerosis: A pilot study. J Neuroimmunol. (2010) 227:185–9.
32. Fernández O, Izquierdo G, Fernández V, Leyva L, Reyes V, Guerrero M, et al. Adipose-derived mesenchymal stem cells (AdMSC) for the treatment of secondary-progressive multiple sclerosis: A triple blinded, placebo controlled, randomized phase I/II safety and feasibility study. PLoS One. (2018) 13:e0195891. doi: 10.1371/journal.pone.0195891
33. Rushkevich Y, Kosmacheva S, Zabrodets G, Ignatenko S, Goncharova N, Severin I, et al. The use of autologous mesenchymal stem cells for cell therapy of patients with amyotrophic lateral sclerosis in Belarus. Bull Exp Biol Med. (2015) 159:576–81.
34. Harris V, Stark J, Vyshkina T, Blackshear L, Joo G, Stefanova V, et al. Phase I trial of intrathecal mesenchymal stem cell-derived neural progenitors in progressive multiple sclerosis. EBioMedicine. (2018) 29:23–30. doi: 10.1016/j.ebiom.2018.02.002
35. Mazzini L, Mareschi K, Ferrero I, Vassallo E, Oliveri G, Boccaletti R, et al. Autologous mesenchymal stem cells: Clinical applications in amyotrophic lateral sclerosis. Neurol Res. (2006) 28:523–6.
36. Mazzini L, Ferrero I, Luparello V, Rustichelli D, Gunetti M, Mareschi K, et al. Mesenchymal stem cell transplantation in amyotrophic lateral sclerosis: A phase I clinical trial. Exp Neurol. (2010) 223:229–37.
37. Mazzini L, Mareschi K, Ferrero I, Miglioretti M, Stecco A, Servo S, et al. Mesenchymal stromal cell transplantation in amyotrophic lateral sclerosis: A long-term safety study. Cytotherapy. (2012) 14:56–60.
38. Oh K, Moon C, Kim H, Oh S, Park J, Lee J, et al. Phase I trial of repeated intrathecal autologous bone marrow-derived mesenchymal stromal cells in amyotrophic lateral sclerosis. Stem Cells Transl Med. (2015) 4:590–7. doi: 10.5966/sctm.2014-0212
39. Staff N, Madigan N, Morris J, Jentoft M, Sorenson E, Butler G, et al. Safety of intrathecal autologous adipose-derived mesenchymal stromal cells in patients with ALS. Neurology. (2016) 87:2230–4.
40. Syková E, Rychmach P, Drahorádová I, Konrádová Š, Rùžičková K, Voříšek I, et al. Transplantation of mesenchymal stromal cells in patients with amyotrophic lateral sclerosis: Results of phase I/IIa clinical trial. Cell Transplant. (2017) 26:647–58.
41. Siwek T, Jezierska-Woźniak K, Maksymowicz S, Barczewska M, Sowa M, Badowska W, et al. Repeat administration of bone marrow-derived mesenchymal stem cells for treatment of amyotrophic lateral sclerosis. Med Sci Monit. (2020) 26:e927484. doi: 10.12659/MSM.927484
42. Petrou P, Kassis I, Yaghmour N, Ginzberg A, Karussis D. A phase II clinical trial with repeated intrathecal injections of autologous mesenchymal stem cells in patients with amyotrophic lateral sclerosis. Front Biosci. (2021) 26:693–706. doi: 10.52586/4980
43. Kim H, Cho K, Jang H, Lee N, Jung Y, Kim J, et al. Intracerebroventricular injection of human umbilical cord blood mesenchymal stem cells in patients with Alzheimer’s disease dementia: A phase I clinical trial. Alzheimers Res Ther. (2021) 13:154. doi: 10.1186/s13195-021-00897-2
44. Brody M, Agronin M, Herskowitz B, Bookheimer S, Small G, Hitchinson B, et al. Results and insights from a phase I clinical trial of Lomecel-B for Alzheimer’s disease. Alzheimers Dement. (2023) 19:261–73. doi: 10.1002/alz.12651
45. Connick P, Kolappan M, Crawley C, Webber D, Patani R, Michell A, et al. Autologous mesenchymal stem cells for the treatment of secondary progressive multiple sclerosis: An open-label phase 2a proof-of-concept study. Lancet Neurol. (2012) 11:150–6. doi: 10.1016/S1474-4422(11)70305-2
46. Hou Z, Liu Y, Mao X, Wei C, Meng M, Liu Y, et al. Transplantation of umbilical cord and bone marrow-derived mesenchymal stem cells in a patient with relapsing-remitting multiple sclerosis. Cell Adh Migr. (2013) 7:404–7. doi: 10.4161/cam.26941
47. Li J, Zhang D, Geng T, Chen L, Huang H, Yin H, et al. The potential of human umbilical cord-derived mesenchymal stem cells as a novel cellular therapy for multiple sclerosis. Cell Transplant. (2014) 23(Suppl. 1):S113–22.
48. Llufriu S, Sepúlveda M, Blanco Y, Marín P, Moreno B, Berenguer J, et al. Randomized placebo-controlled phase II trial of autologous mesenchymal stem cells in multiple sclerosis. PLoS One. (2014) 9:e113936. doi: 10.1371/journal.pone.0113936
49. Lublin F, Bowen J, Huddlestone J, Kremenchutzky M, Carpenter A, Corboy J, et al. Human placenta-derived cells (PDA-001) for the treatment of adults with multiple sclerosis: A randomized, placebo-controlled, multiple-dose study. Mult Scler Relat Disord. (2014) 3:696–704. doi: 10.1016/j.msard.2014.08.002
50. Harris V, Vyshkina T, Sadiq S. Clinical safety of intrathecal administration of mesenchymal stromal cell-derived neural progenitors in multiple sclerosis. Cytotherapy. (2016) 18:1476–82. doi: 10.1016/j.jcyt.2016.08.007
51. Riordan N, Morales I, Fernández G, Allen N, Fearnot N, Leckrone M, et al. Clinical feasibility of umbilical cord tissue-derived mesenchymal stem cells in the treatment of multiple sclerosis. J Transl Med. (2018) 16:57.
52. Venkataramana N, Kumar S, Balaraju S, Radhakrishnan R, Bansal A, Dixit A, et al. Open-labeled study of unilateral autologous bone-marrow-derived mesenchymal stem cell transplantation in Parkinson’s disease. Transl Res. (2010) 155:62–70. doi: 10.1016/j.trsl.2009.07.006
53. Canesi M, Giordano R, Lazzari L, Isalberti M, Isaias I, Benti R, et al. Finding a new therapeutic approach for no-option Parkinsonisms: Mesenchymal stromal cells for progressive supranuclear palsy. J Transl Med. (2016) 14:127. doi: 10.1186/s12967-016-0880-2
54. Carstens M, Haq I, Martinez-Cerrato J, Dos-Anjos S, Bertram K, Correa D. Sustained clinical improvement of Parkinson’s disease in two patients with facially-transplanted adipose-derived stromal vascular fraction cells. J Clin Neurosci. (2020) 81:47–51. doi: 10.1016/j.jocn.2020.09.001
55. Moviglia G, Fernandez Viña R, Brizuela J, Saslavsky J, Vrsalovic F, Varela G, et al. Combined protocol of cell therapy for chronic spinal cord injury. Report on the electrical and functional recovery of two patients. Cytotherapy. (2006) 8:202–9. doi: 10.1080/14653240600736048
56. Pal R, Venkataramana N, Bansal A, Balaraju S, Jan M, Chandra R, et al. Ex vivo-expanded autologous bone marrow-derived mesenchymal stromal cells in human spinal cord injury/paraplegia: A pilot clinical study. Cytotherapy. (2009) 11:897–911. doi: 10.3109/14653240903253857
57. Karamouzian S, Nematollahi-Mahani S, Nakhaee N, Eskandary H. Clinical safety and primary efficacy of bone marrow mesenchymal cell transplantation in subacute spinal cord injured patients. Clin Neurol Neurosurg. (2012) 114:935–9. doi: 10.1016/j.clineuro.2012.02.003
58. Dai G, Liu X, Zhang Z, Yang Z, Dai Y, Xu R. Transplantation of autologous bone marrow mesenchymal stem cells in the treatment of complete and chronic cervical spinal cord injury. Brain Res. (2013) 1533:73–9. doi: 10.1016/j.brainres.2013.08.016
59. Cheng H, Liu X, Hua R, Dai G, Wang X, Gao J, et al. Clinical observation of umbilical cord mesenchymal stem cell transplantation in treatment for sequelae of thoracolumbar spinal cord injury. J Transl Med. (2014) 12:253. doi: 10.1186/s12967-014-0253-7
60. Mendonça M, Larocca T, de Freitas Souza B, Villarreal C, Silva L, Matos A, et al. Safety and neurological assessments after autologous transplantation of bone marrow mesenchymal stem cells in subjects with chronic spinal cord injury. Stem Cell Res Ther. (2014) 5:126.
61. Hur J, Cho T, Park D, Lee J, Park J, Chung Y. Intrathecal transplantation of autologous adipose-derived mesenchymal stem cells for treating spinal cord injury: A human trial. J Spinal Cord Med. (2016) 39:655–64.
62. Satti H, Waheed A, Ahmed P, Ahmed K, Akram Z, Aziz T, et al. Autologous mesenchymal stromal cell transplantation for spinal cord injury: A phase I pilot study. Cytotherapy. (2016) 18:518–22. doi: 10.1016/j.jcyt.2016.01.004
63. Vaquero J, Zurita M, Rico M, Aguayo C, Bonilla C, Marin E, et al. Intrathecal administration of autologous mesenchymal stromal cells for spinal cord injury: Safety and efficacy of the 100/3 guideline. Cytotherapy. (2018) 20:806–19. doi: 10.1016/j.jcyt.2018.03.032
64. Zhang Z, Guan L, Zhang K, Zhang Q, Dai L. A combined procedure to deliver autologous mesenchymal stromal cells to patients with traumatic brain injury. Cytotherapy. (2008) 10:134–9. doi: 10.1080/14653240701883061
65. Wang S, Cheng H, Dai G, Wang X, Hua R, Liu X, et al. Umbilical cord mesenchymal stem cell transplantation significantly improves neurological function in patients with sequelae of traumatic brain injury. Brain Res. (2013) 1532:76–84. doi: 10.1016/j.brainres.2013.08.001
66. Duma C, Kopyov O, Kopyov A, Berman M, Lander E, Elam M, et al. Human intracerebroventricular (ICV) injection of autologous, non-engineered, adipose-derived stromal vascular fraction (ADSVF) for neurodegenerative disorders: Results of a 3-year phase 1 study of 113 injections in 31 patients. Mol Biol Rep. (2019) 46:5257–72. doi: 10.1007/s11033-019-04983-5
Keywords: MSCs, neurological disorders, cell therapy, ALS, PD, MS, SCI, TBI
Citation: Patel GD, Liu L, Li A, Yang Y-H, Shen C-C, Brand-Saberi B and Yang X (2024) Mesenchymal stem cell-based therapies for treating well-studied neurological disorders: a systematic review. Front. Med. 11:1361723. doi: 10.3389/fmed.2024.1361723
Received: 26 December 2023; Accepted: 13 March 2024;
Published: 27 March 2024.
Edited by:
Brenda Laky, Austrian Society for Regenerative Medicine (ASRM), AustriaReviewed by:
Paola Palumbo, University of L’Aquila, ItalyPhilipp Heuberer, HealthPi Medical Center, Austria
Copyright © 2024 Patel, Liu, Li, Yang, Shen, Brand-Saberi and Yang. This is an open-access article distributed under the terms of the Creative Commons Attribution License (CC BY). The use, distribution or reproduction in other forums is permitted, provided the original author(s) and the copyright owner(s) are credited and that the original publication in this journal is cited, in accordance with accepted academic practice. No use, distribution or reproduction is permitted which does not comply with these terms.
*Correspondence: Xuesong Yang, yang_xuesong@126.com; Beate Brand-Saberi, Gabriela.Morosan-Puopolo@ruhr-uni-bochum.de
†These authors have contributed equally to this work and share first authorship