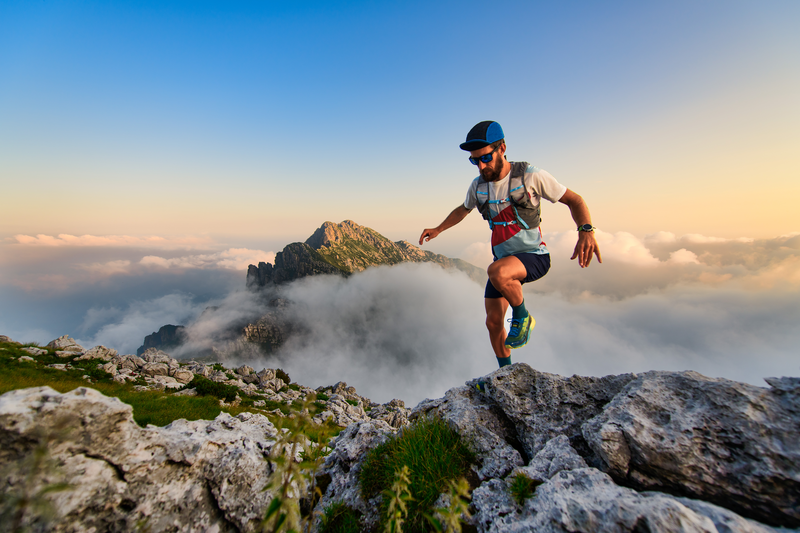
95% of researchers rate our articles as excellent or good
Learn more about the work of our research integrity team to safeguard the quality of each article we publish.
Find out more
REVIEW article
Front. Med. , 27 June 2024
Sec. Precision Medicine
Volume 11 - 2024 | https://doi.org/10.3389/fmed.2024.1360966
This article is part of the Research Topic Molecular Informatics in Precision Medicine View all 10 articles
Background: It is reported that the Ixeris chinensis has high medicinal value, but there are few reports about its potential molecular mechanism. We used a network pharmacology approach to predict the active ingredients, targets of action and possible interventions in diseases of Ixeris chinensis.
Methods: We employed various databases and software to predict the active ingredients, target genes, protein interactions, signaling pathways, network diagrams, and molecular docking of Ixeris chinensis. Simultaneously, we searched multiple Chinese and English databases and conducted meta-analyses of five randomized controlled trials.
Results: The analysis results revealed 12 effective components, including apigenin β-sitosterol, baicalin, baicalein, and luteolin; and selected 40 key targets, including AKT1, TNF, EGFR, ESR1, SRC, among others. GO analysis generated 225 biological processes, 39 cellular components, and 65 molecular functions; KEGG analysis revealed 103 signaling pathways. Molecular docking results indicated that the main active components of Ixeris chinensis can bind well with key targets. Five randomized controlled trials were included. Meta-analysis showed that Ixeris extract can effectively reduce animal blood lipid levels.
Conclusion: This study revealed the main active ingredients and key targets of Ixeris chinensis, analyzed the signaling pathways of potential targets, conducted disease prediction, and performed molecular docking prediction, providing a basis for research on the pathways of Ixeris treatment for related diseases and subsequent new drug development.
Ixeris chinensis (Thunb.) Nakai is the whole herb of Sonchus of the family Asteraceae (1). According to the textual research on the herbal literature, the authentic source of Chinese herbal medicine bitter vegetables should be the main stream of Sonchus oleraceus L, which belongs to Sonchus, Ixeris polycephala Cass of Ixeris Cass, and the dried whole grass of Chinese Ixeris (2). I. chinensis is a traditional medicinal plant, which can be used to treat tumors, inflammation and some infections. I. chinensis contains a variety of flavonoids, which have various pharmacological or health effects such as antioxidant, anti-cancer, antihypertensive, improving cardiovascular diseases, lowering blood lipids, lowering cholesterol, and protecting our liver (3). I. chinensis is one of the traditional Chinese herbs in China, with a wide range of distribution, easy survival and a wide variety of species (4).
In recent years, with the deepening of the research on I. chinensis, the clinical application scope of the herb has been continuously expanded (5). It is often used in combination with other Chinese medicines for treating various inflammatory diseases such as gastritis, enteritis, lung fever and cough, sore throat, etc. (6). The pharmacological effects of I. chinensis have been studied more extensively, but the mechanism of action of the disease is still unknown and needs to be studied thoroughly.
Network pharmacology research uses public databases and literature to screen out the target information of known drug components (7). Then uses network visualization tools to build a multi-dimensional biological network model, through multi-level analysis of specific signal nodes in the network at the cellular, molecular and overall biological levels, to identify key nodes, and from the perspective of the overall biological network balance to discover the target drug’s interference with the “pathogenic network,” and then predicts the pharmacological active ingredients, targets and possible involvement in the regulation of cellular signal transduction pathways, potential pharmacological mechanisms and formulation and combination patterns of known drugs to combat diseases (8). To predict the active ingredients, targets, cellular signal transduction pathways, potential pharmacological mechanisms and prescriptions that may be involved in the regulation of known drugs against diseases (9). Finally, validation at the animal or cellular level, will reveal the modern pharmacological mechanisms of drugs against diseases and explore new indications for drug interventions (10).
This artical adopted the research method of network pharmacology. By screening the active components and key targets of I. chinensis, we systematically analyzed its potential action targets to construct protein interaction networks and the enriched biological processes and pathways, so as to elaborate the pharmacological action mechanism of I. chinensis, which laid a foundation for further in-depth study on the mechanism of action of I. chinensis in the treatment of specific diseases and was of great significance in the field of new drug development.
Traditional Chinese Medicine Systems Pharmacology (TCMSP1), HERB database2, Pubchem database3, Swiss Target Prediction database4, Uniprot database5, STRING database6, DAVID database7, pubmed8, Web of Science9, China National Knowledge Infrastructure (CNKI10), Wangfang database11.
Cytoscape3.9.1, Rstudio, Microlife Letter, PyMOL, AutoDockTools, RevMan 5.4.1.
By searching the Traditional Chinese Medicine Systems Pharmacology Database and Analysis Platform. The results were obtained by searching for “Sonchus oleraceus L,” “Ixeris polycephala Cass,” “I. chinensis (Thunb.) Nakai,” “Lactuca tatarica.” The active ingredients were searched in the HERB database by searching for “I. chinensis.”
We inputted the active components of I. chinensis into the swiss target prediction to predict the target genes, and used the Uniprot database (see foot note 5) to convert the full name of the target gene to the abbreviation, deleted the target without corresponding gene name, and used Cytoscape 3.9.1 to construct the network diagram of I. chinensis – component-target.
The potential targets were entered into the STRING database for protein interaction analysis, free targets were excluded, and the Protein–protein interaction (PPI) network was constructed using Cytoscape 3.9.1 software to screen for key targets, which could be considered as important proteins for bittercress to exert its medicinal effects.
We used the DAVID database to perform Gene Ontology (GO) analysis and Kyoto Encyclopedia of Genes and Genomes (KEGG) pathway enrichment analysis on key targets, including Biological Process (BP), Cell Component (CC) and Molecular Function (MF). With human gene as the background, set p-value<0.01, we screened the top 10 BP, CC, MF entries and the top 20 KEGG pathway entries, and used the microbiota website to make enrichment histogram and bubble chart.
We used the Rstudio software to analyze DO data, and used the microbiota website for visual analysis. According to the p-value, we screened the top 20 target diseases with the highest credibility, so as to achieve the effect of disease prediction.
According to the PPI analysis results, we selected the top ten key targets AKT1, TNF, EGFR, ESR1, SRC, PTGS2, MAPK1, MMP9, IL2 and AR for docking. Based on the comprehensive analysis of the results of “component-target network” and “component- target-disease network,” the key components in I. chinensis luteolin, apigenin, chinensiolide c, chinensiolide b, chinensiolide a, β-sitosterol, luteolin-7-O-β-D-glucoside played a leading role in the network. We adopted semi flexible docking to find the best binding position and strength of substrate molecules and receptor molecules (11). We searched the 3D structure of key proteins in the PDB protein structure database, and selected the proteins with high resolution and long structure in the human background. PyMOL software was used to remove water and solvent molecules in the protein, and AutoDockTools 1.5.7 software was used to conduct hydrogenation treatment, set as ligand. After downloading the 3D structure of small molecules using Pubchem, swiss target prediction, hydrogenation was performed in AutoDockTools 1.5.7 software to automatically assign charges, set up as ligands and set up torsional bonds. We docked large and small molecules, set docking boxes, docked parameters and arithmetic methods. The screened macromolecules and small molecules from I. chinensis were docked separately, and the binding energy less than 0 indicated that the ligand and the receptor could bind spontaneously, the binding energy ≤ −5. 0 kcal·mol −1 proved that the component was well docked with the target site, and the binding energy ≤ −7. 0 kcal·mol −1 indicated that the component and the binding conformation of the target was strongly active (12). The docking results were thermographed using Rstudio, and those with high binding energy were visualized using PyMOL.
Randomized controlled trial (RCT) of I. chinensis water extract in the treatment of diseases.
Mice fed with high-fat diet or injected with ccl4 and successfully modeled.
Test group treated rats with I. chinensis water extract. The control group used placebo or no special treatment.
Total Cholesterol (TC), Triglycerides (TG), High-Density Lipoprotein Cholesterol (HDL-C), Low-Density Lipoprotein Cholesterol (LDL-C), Malondialdehyde (MDA).
Subject term retrieval (see footnote 8), Web of Science (see footnote 9), CNKI (see footnote 10), Wanfang database (Wanfang, see footnote 11), to be included in the randomized controlled trial with I. chinensis as the treatment method. Take Pubmed as an example. See the table for its specific retrieval strategy (Table 1).
Cochrane risk bias assessment tool and SYRCLE bias risk assessment tool were used to evaluate the quality of the included studies. The evaluation contents included: ① Random sequence generation (selection bias) ② Allocation consideration (selection bias), ③ Blinding of participants and persons (performance bias), ④ Blinding of outcome assessment (detection bias), ⑤ Incomplete outcome data (attribute bias), ⑥ Selective reporting (reporting bias), ⑦ Other biases.
RevMan 5.4.1 software was used for meta analysis. The standardized mean difference (SMD) was used as the effect index for the measurement data. The point estimates and 95% CI were given for each effect. If p ≥ 0.1 and I2 < 50%, it was considered that the heterogeneity between the studies was not significant, and the fixed effect model was selected; If p < 0.1, I2 ≥ 50%, it was considered that there was significant heterogeneity among the studies, and the random effect model was selected (13).
We searched the HERB database for “I. chinensi” and found that there were 12 active ingredients, namely Apigenin, β-sitosterol, calcium carbonate, chinensiolide a, chinensiolide b, chinensiolide c, ixerisosidea, ixerochinolide, ixerochinoside, Lactucin, Luteolin, luteolin-7-O-β-D-glucoside. The above active ingredients were used as research subjects.
Since there was no match between the targets of HERB database and the active ingredients of I. chinensis, so we entered into Pubchem for searching, and the smileIDs of 10 active ingredients were obtained and then entered into Swiss Target Prediction for target gene prediction, among which the remaining two ingredients, “Lactucin” was used for target gene prediction in TCMSP website and “calcium carbonate” was used for target gene prediction in National Library of Medicine website. By screening the target genes with confidence greater than 0.01 or the top 15 target classes, converting the full names of target genes to abbreviations with the help of Uniprot database (see footnote 5) and deleting the targets without corresponding gene names, a total of 238 targets were derived, and using Cytoscape 3.9.1 to construct the I. chinensis–active compound–target network (Figure 1).
Figure 1. Ixeris chinensis active ingredient-target network diagram. The diamond shape represented the active ingredients of this herb, the oval represented the target site.
After entering 238 potential targets into STRING database for protein interaction analysis and eliminating free targets, we used Cytoscape 3.9.1 software to construct PPI network (Figure 2) and calculated parameter thresholds, screened Degree (node centrality, reflecting the number of other targets that participate in different disease pathological processes together with a certain target) greater than 30, BC (mediator centrality value, the target with high proximity centrality was directly connected to more targets, indicating the importance of the target in different disease pathomechanical processes) was greater than 369, CC (closeness centrality, reflecting the proximity between a node and other nodes in the network) was greater than 0.0017, a total of 40 key targets were screened, which could be regarded as important proteins for I. chinensis to exert their medicinal effects (Table 2).
Figure 2. PPI network diagram. The larger the node shape in the figure and the closer to the center, the higher the criticality.
GO-BP analysis showed that 225 biological processes were related to this, and the most significant top 5 were positive regulation of transcription from RNA polymerase II promoter, signal transduction, response to xenobiotic stimulus, positive regulation of gene expression, positive regulation of transcription, DNA-templated (Table 3). GO-CC analysis revealed 39 cellular compositions associated with this, the five most significant being cytoplasm, nucleoplasm, plasma membrane, nucleus, cytosol (Table 4). GO-MF analysis showed that 65 molecular functions were related to this, and the most significant 5 were protein binding, ATP binding, identical protein binding, enzyme binding, protein homodimerization (Table 5). The results suggested that I. chinensis could exert their therapeutic effects by participating in the regulation of various biological processes (Figure 3).
Figure 3. GO enrichment analysis results of key target proteins. Including biological process, cellular components, molecular functions.
KEGG is the main database for systematic analysis of gene function, genomic and proteomic information, where different proteins exercise their biological behaviors in coordination with each other, and it helps researchers to study protein and expression information as a whole network. Included in the database are illustrated cellular biochemical processes such as metabolism, membrane transport, signaling, cell cycle, and also information on homologous conserved subpathways (14).
KEGG analysis showed that the five most significantly enriched pathways were Pathways in cancer, Human papillomavirus infection, Prostate cancer, Breast cancer, Chemical carcinogenesis-receptor activation (Table 6 and Figure 4). Among the pathways associated with disease classes, the main ones are the Pathways in cancer, Human papillomavirus infection, Prostate cancer, Breast cancer, Chemical carcinogenesis – receptor activation, Human cytomegalovirus infection, Cushing syndrome, Hepatitis C, Hepatitis B, Kaposi sarcoma-associated herpesvirus infection, Viral carcinogenesis, Proteoglycans in cancer, Lipid and atherosclerosis, Human T-cell leukemia virus 1 infection, MicroRNAs in cancer, Alzheimer disease, etc. Among the pathways associated with signaling functions were PI3K-Akt signaling pathway, Estrogen signaling pathway, Prolactin signaling pathway, etc. It was suggested that the potential targets were involved in multiple signaling pathways acting in concert.
Figure 4. Enrichment results of key target protein KEGG. The larger the point was, the more relevant target points were; The redder the color, the higher the credibility.
Cardiovascular and cerebrovascular diseases based on atherosclerosis are the main killers threatening human health. Years of research showed that the common pathological basis of cardiovascular and cerebrovascular diseases comes from atherosclerosis, so the key to prevent cardiovascular and cerebrovascular diseases is to prevent atherosclerosis (15).
There are many factors affecting cardiovascular and cerebrovascular diseases, such as vascular endothelial growth factor A (VEGF-A), a dimer glycoprotein encoded by VEGF-A gene, which plays a crucial role in the process of inducing vascular growth (16). Many studies have also confirmed that Akt and downstream molecular activity changes are related to some cardiovascular diseases. Minamino et al. (17) detected a vascular endothelial cell senescence phenotype in atheromatous plaque tissue. The findings suggested that statins improved endothelial function and reduced myocardial remodeling in the early stages of myocardial infarction through activation of Akt. Yang Hui et al. (18) prepared a hyperlipidemia mouse model, treated the water extract of I. chinensis and injected it intraperitoneally. The study showed that I. chinensis has an important contribution to improving the antioxidant capacity of hyperlipidemia mice, and could reduce the level of lipid peroxidation, but the specific deep mechanism needs further study.
Signaling pathways are important for the study of diseases, based on the key targets in I. chinensis and cardiovascular diseases, KEGG enrichment analysis showed that lipids and atherosclerosis was more significant signaling pathways. Therefore, taking this pathway as an example, it is of great significance to explore the role of the key target in the signal pathway of I. chinensis, and to explore the specific mechanism of disease and treat disease.
The elevated level of low density lipoprotein (LDL) cholesterol is a major risk factor for atherosclerosis. LDL can accumulate in the blood vessel wall and be modified by oxidation. Oxidized LDL (oxLDL) leads to endothelial dysfunction, the expression of adhesion molecules and the recruitment of monocytes in the subendothelial space (19). The extracellular oxLDL binds to the membrane receptor Lox-1. LOX-1 mediates the recognition and internalization of oxLDL, activates the substrate ROCK2, which inhibits PI3K, and then generates PIP3, thereby activating AKT, which promotes Bclxl phosphorylation, thus inducing cell apoptosis (20, 21).
Advanced glycosylation end products (AGEs) are harmful compounds formed by the combination of protein or fat in the blood with sugar. They bind with the RAGE receptor on the cell membrane, and then activate and generate ROS (22). ROS indirectly activates Src, which in turn activates Rac1. Rac1 further activates MKK4/7, promotes JNK phosphorylation, and then promotes AP-1 phosphorylation. The phosphorylated AP-1 will enter the nucleus, regulate DNA transcription, and affect the expression of MCP-1, ICAM-1, and IL-8, thus affecting the attachment and activation of downstream monocytes. ERK activated in cytoplasm can indirectly activate NF-κB, NF-κB affects the expression of MMP1,3,9 and TNF-α by promoting DNA transcription, thus affecting damage, cell apoptosis and inflammation (23).
Minimally modified low density lipoprotein (mmLDL) is LDL in which only the lipid fraction is oxidized and the lysine residue in the Apo B100 structure is not destroyed (24). MmLDL binds with TLR2/4/6 receptor on cell membrane to activate ERK, which in turn indirectly activates rap1, and rap1 activates Rac, which in turn activates NADPH oxidase, promotes the expression of ROS in vivo, and indirectly promotes NF-κB. In addition, mmLDL promotes the separation of intracytoplasmic phosphorylated IκBα from NF-κB through a series of pathways, and the separated NF-κB promotes DNA transcription, thus affecting the expression of MMP1,3,9 and TNF-α, which have effects on monocyte attachment and activation, injury and apoptosis, and inflammatory response.
Tumor necrosis factor (TNF-α) is a cytokine involved in systemic inflammation, and also one of the many cytokines that cause acute response. Extracellular TNF-α binds to the TNF-R1 receptor on the cytomembrane, indirectly activating CASP8, and then activating CASP3, thereby affecting cell apoptosis and plaque instability (25).
Very low density lipoprotein(VLDL)is a kind of lipoprotein which is composed of apolipoprotein synthesized in the liver by using the intermediate metabolites of chyle granules, bile acid, fatty acid, sugar and protein. VLDL binds to VLDLR receptor on the cell membrane, activates substrate PI3K, connects with PI3K-AKT signal, promotes the expression of PIP3, and then activates AKT (26). AKT phosphorylates GSK3β, and the phosphorylated GSK3β phosphorylates NFAT, which promotes DNA transcription and has an effect on cell proliferation (27).
The signal pathways are complex, and the proteins related to the key targets in the I. chinensis were all marked red (Figure 5), such as Src, TNFα, ERK, AKT, CYP, etc. It could be seen from the figure that most of the proteins in this pathway were distributed in the middle reaches, and they had important effects on inflammatory reaction, foam cell formation, cytokines, inflammatory signals, plaque instability, etc. The study of this pathway is helpful for anti atherosclerosis.
Figure 5. Lipid and atherosclerosis signal pathway. The gene marked red is the key target in I. chinensis.
DO data analysis was performed using Rstudio software, setting p-value <0.05 and Q value = 1. Disease enrichment analysis was performed on the key targets of I. chinensis action, removing the same diseases, and then extracting the 20 diseases with the highest number of targets and visualizing them using the Microbiology website (Figure 6 and Table 7). The main diseases included autosomal dominant disease, hereditary breast ovarian cancer, cell type benign neoplasm, female reproductive organ cancer, adenoma, biliary tract cancer, brain disease, diarrhea, renal cell carcinoma, atherosclerosis, arteriosclerotic cardiovascular disease, leiomyoma, osteoporosis, Alzheimer’s disease, tauopathy, bone cancer, connective tissue cancer, lymphoblastic leukemia, obesity, infertility, etc. The key targets proved to be highly relevant to these diseases. In order to explore the association between I. chinensis and diseases, Cytoscape 3.9.1 software was used to construct and visualize the “component-target-disease” network map using collected components, targets and disease data (Figure 7). The network consisted of 12 components, 238 targets and 20 diseases, which visually showed that multiple targets contained in one component could participate in the regulation of multiple diseases at the same time.
Figure 6. DO enrichment results for key target proteins. The longer the bar graph was, the more relevant targets were; The redder the color, the higher the credibility.
Figure 7. Ixeris chinensis component-disease-target map. In the figure, the left circle represented active chemical components, the middle square matrix represented target genes, and the right circle represented diseases.
Molecular docking technology is of great significance for further research in plant chemistry and biology (28). The current study docked each of the 7 active ingredients in I. chinensis with 10 key targets, yielding 70 docking results (Figure 8). The results revealed that luteolin, apigenin, chinensiolide c, chinensiolide b, chinensiolide a, β-sitosterol, and luteolin-7-O-β-D-glucoside had better docking ability with EGFR, ESR1, PTGS2, MAPK1, MMP9, and AR. The lower the binding energy, the better the molecule’s ability to bind to the protein (Table 8). Among them, MMP9 showed strong docking ability with each active ingredient, and β-sitosterol also showed strong binding ability with each protein. The docking results of the top 8 components with strong binding activity (binding energy ≤ −7 kcal·mol-1) to the targets were visualized and presented using PyMOL software (Figure 9). β-sitosterol forms one hydrogen bond with MMP9 via amino acid residue GLU-111, one hydrogen bond with PTGS2 via amino acid residue ARG-44, two hydrogen bonds with AR via amino acid residues GLN-802 and GLU-687, and one hydrogen bond with EGFR via amino acid residue GLU-758 forming one hydrogen bond; luteolin forming four hydrogen bonds with MMP9 via amino acid residues ALA-189, ALA-417, GLU-402, and LEU-188; apigenin forming three hydrogen bonds with MMP9 via amino acid residues ALA-189, ALA-417, and LEU-188, and three hydrogen bonds with MMP9 via amino acid residues ARG-752, ASN-705, GLN-711, and LEU-873 to form four hydrogen bonds with AR, and chinensiolide b to form three hydrogen bonds with PTGS2 via amino acid residues HIS-388, TRP-387, and TYR-385. The high binding energy between the main active components and key targets in I. chinensis indicated that I. chinensis might play its therapeutic role by regulating the above related targets.
Figure 8. Docking and binding energy information of active ingredients and key target molecules in Ixeris chinensis. The redder the color, the better the binding ability.
Figure 9. Docking analysis of the active components and key targets of I. chinensis. In the figure, the blue macromolecule is the target protein, and the green smaller molecule is the active component. (A) β-sitosterol and MMP9, (B) β-sitosterol and PTGS2, (C) β-sitosterol and AR, (D) β-sitosterol and EGFR, (E) luteolin and MMP9, (F) apigenin and MMP9, (G) apigenin and AR, (H) chinensiolide b and PTGS2.
The document screening process and basic characteristics of included documents are shown in the figure below (Figure 10 and Table 8). A total of five randomized controlled trials (RCTs) were conducted, including studies employing Sprague–Dawley (SD) rats, ICR mice, C57BL/6J mice, Wistar rats, and KM mice. For modeling, one study was fed with high-fat and high-sugar diets, two studies used carbon tetrachloride (CCl4), and two studies were fed with high-fat diets. The interventions were mainly treated with I. chinensis water extract. The diseases included in the studies mainly consisted of two cases of lipid-based diseases and three cases of hepatitis-based diseases. The included outcome indicators contained TC, TG, HDL-C, LDL-C, and MDA.
Figure 10. (A) Flow chart of document screening. (B,C) Risk assessment of literature bias. Green is low risk bias, yellow is unknown risk bias, and red is high risk bias. The length of the bar indicates the proportion of this bias in the total number of articles.
The five research baselines included were comparable. In the Random sequence generation, four studies described the random allocation method in detail and were rated as low risk bias, while one study only mentioned the word “random” without specifying the specific method and was rated as unknown risk bias. In the Allocation consideration, only one described the allocation concealment scheme, which was rated as low risk bias, and the rest as unknown risk bias. Among Blinding, only one described the allocation concealment scheme, which was rated as low risk bias, and the rest as unknown risk bias. In the Incomplete outcome data, all studies reported complete results and were rated as low risk bias; In selective reporting, none of the five studies reported selectively, which was rated as low risk bias. In Other bias, five studies were unable to judge whether there were other sources of bias, and all were rated as unknown risk bias (Figure 10).
Included in the study, three articles reported four changes in blood lipids (29–31) (Figure 11A). The above three articles were divided into four subgroups according to blood lipid indicators. Heterogeneity between studies (I2 > 50%) was analyzed by random effect model. Meta analysis results showed that I. chinensis could significantly reduce the blood TC level, [SMD = –1.85, 95% CI (−2.87, −0.38), I2 = 53%, p = 0.0004], indicating significant heterogeneity and statistically significant difference. I. chinensis could also significantly reduce the blood TG level, [SMD = –1.95, 95% CI (−3.02, −0.87), I2 = 51%, p = 0.0004], indicating significant heterogeneity and statistically significant difference. I. chinensis showed no statistically significant difference in improving HDL-C [SMD = 1.34, 95% CI (0.26, 2.42), I2 = 65%, p = 0.02]. I. chinensis significantly reduced blood LDL-C levels, [SMD = –2.77, 95% CI (−3.87, −1.66), I2 = 43%, p < 0.00001], suggesting that the difference was statistically significant. The sensitivity analysis of the included literatures was carried out one by one with the method of exclusion. None of the literatures had a great impact on the results of this Meta-analysis, which meant that this study has a good stability.
Figure 11. The study was grouped according to outcome indicators. The random effect model was used for analysis. Heterogeneity was calculated using I2. The summary estimates of the standard mean difference and 95% confidence zone were calculated using Review Manager 5.4.1. (A) Take the four items of blood lipid as the outcome index. (B) Take MDA as the outcome indicator.
Among the included studies, two articles reported changes in MDA with heterogeneity between studies (I2 > 50%), analyzed using a random-effects model (32, 33) (Figure 11B). The difference in I. chinensis in improving HDL-C was not statistically significant [SMD = −9.76, 95% CI (−26.39, 6.86), I2 = 96%, p = 0.25].
We used the four indicators of blood lipids as an example to create a funnel plot. Funnel diagram showed that the included articles were asymmetric with the symmetry axis as the boundary, indicating that there was publication bias, which might be related to the small sample size of the included articles (Figure 12).
As a traditional Chinese herbal medicine, I. chinensis has complex types and various functions. It has been used as a medicinal material for lowering blood glucose, cholesterol, diuresis, bloodpressure, anticoagulation, liver protection, antiinflammatory, antibacterial, anti-tumor and so on (6). The emergence of traditional.
Chinese medicine network pharmacology provides an opportunity to systematically explore the molecular complexity of traditional Chinese medicine prescriptions and the molecular relationship between traditional Chinese medicine ingredients and complex diseases (34). In recent years, the pharmacological effects of I. chinensis have been widely studied, but the specific mechanism of disease action is still unclear and needs to be further studied. Therefore, we should start from the analysis of active components to explore the key role targets of I. chinensis, and carry out GO and KEGG analysis, conduct molecular docking which is of great significance to predict the biological process, cell composition, molecular function and signal pathway of I. chinensis, and has a profound influence on the research of specific disease mechanism and the development of new drugs.
As a traditional Chinese herbal medicine, there are many kinds of I. chinensis. Now we pay more attention to the pharmacodynamic material basis of I. chinensis, that is, the effective components in the curative effect, which requires us to screen and analyze the effective components in it. By searching “I. chinensis “in HERB database, the current study found 12 active ingredients of it, including apigenin, β-sitosterol, luteolin, luteolin-7-O-β-D-glucoside and so on. These active ingredients were used as the basis of the whole analysis of I. chinensis.
The active compounds of I. chinensis have many targets. The active compounds of I. chinensis were input into swiss target prediction for target gene prediction. The targets without corresponding gene names were deleted by Uniprot database, and the related targets were screened out. To explore the relationship between the active compounds of I. chinensis and the target sites, Cytoscape3.9.1 was used to construct the I. chinensis composition-target network diagram. There were 238 targets of I. chinensis. Two hundred and thirty-eight potential targets were input into STRING database for protein interaction analysis, and free targets were eliminated. Cytoscape 3.9.1 software was used to construct PPI network, and 40 key targets were screened out. They were mainly AKT1, TNF, EGFR, ESR1, SRC, PTGS2, MAPK1, which were regarded as important proteins of I. chinensis. It was suggested that it might play a therapeutic role in diseases through the interaction of active compounds with key targets. Most of the key targets were closely related to monocyte attachment and activation, injury and apoptosis, inflammation, matrix digestion, cell migration, differentiation, proliferation and other biological functions. They had similar biological effects and synergistic effects of multiple targets. Akt1 is an important gene regulating cell survival and proliferation, which can regulate tumor proliferation, metastasis and invasion. Transplanting mesenchymal stem cells overexpressing Akt1 into rat myocardium can reduce infarct size and delay cardiac remodeling (35). TNF is a substance that can damage tumor cells and make them necrotic. TNF-α plays a role in inducing cell apoptosis, regulating the immune response of the body, regulating the vascular system of tumor tissue, and inducing programmed cell necrosis. It plays different regulatory roles in many malignant tumors, such as gastric cancer, liver cancer, breast cancer, etc. (36). EGFR belongs to the epidermal growth factor receptor family, which is activated after binding with ligands. It transmits information through Ras/Raf/MEK/ERK/MAPK pathway, PI3K/AKT (PKB) pathway and JAK/STAT pathway, thus affecting tumor cell proliferation, angiogenesis, invasion and metastasis (37). Estrogen Receptor 1 (ESR1) expression is lost or diminished in human Hepatocellular carcinoma (HCC) cells and liver tumors, suggesting a potential protective role of estrogen signaling in HCC and that low ESR1 gene expression plays.
an important role in the development of hepatocellular carcinoma (38). The sparse representation based classifier gene is the earliest proto oncogene discovered by human beings. Its protein product, Src, is the most widely distributed protein kinase in vivo. It participates in a series of physiological activities such as regulating cell proliferation and differentiation by phosphorylating various signal molecules in cells (39). PTGS2 gene is an inducible immediate response gene, and its expression is rapidly upregulated by certain cytokines, growth factors, inflammatory mediators, pro-oncogenic factors and other stimulating factors during the occurrence of pathological responses such as inflammation or tumor, and its main product catalyzed by PG is an important inflammatory mediator in liver injury, which is an important link in inflammation (40). Mitogen-activated protein Kinase (MAPK) signal pathway is an important inflammation related signal pathway. Some studies have shown that MAPK1 has neuroprotective effect after stroke, and some studies have shown that MAPK1 has harmful effects on stroke due to its activation promoting inflammation and oxidative stress and inhibiting and reducing ischemic injury (41).
Comprehensive analysis of the results of “component-target” network and “component-target-disease network,” The key components in I. chinensis were apigenin, chinensiolide c, chinensiolide a, chinensiolide b, luteolin, luteolin-7-O-β-D-glucoside and β-sitosterol, which were dominant in the network. According to relevant literature reports, apigenin, luteolin, luteolin-7-O-β-D-glucoside belong to flavonoids (6, 42–44), chinensiolide c, chinensiolide a and chinensiolide b belong to sesquiterpenes (45),β-sitosterol belongs to triterpenes and steroids (6). Flavonoids have the advantages of scavenging free radicals, protecting liver, protecting heart and brain, regulating blood lipids and so on (46). Sesquiterpenes have a wide range of biological activities, such as anti-tumor, antibacterial, anti-inflammatory, anti-neurotoxic, antiviral, immunosuppressive, hepatoprotective and heart-strengthening activities (47). Triterpenes and steroids have anti-inflammatory, antibacterial and anti-tumor effects (48). Studies have shown that apigenin can inhibit the proliferation of colorectal cancer CL187 cells and promote apoptosis by inhibiting PI3K/Akt signaling pathway and regulating the expression of MAPK signaling pathway related proteins (49). Apigenin can inhibit the EMT of liver tissue cells by inhibiting PDK1/AKT signal pathway through liver bypass, and play an anti-fibrosis role (50). Luteolin can eliminate free radicals, enhance the activity of antioxidant enzymes, regulate proinflammatory mediators, and inhibit IκB kinase β Phosphorylation of subunits, down regulating TNF-α and the mRNA level of IL-6 (51). Luteolin-7-O-β-D-glucoside has protective effect on myocardial cells cultured under ischemia and hypoxia. Its mechanism may be related to scavenging oxygen free radicals, stabilizing cell membrane and inhibiting apoptosis (52). The water extract of I. chinensis A can effectively inhibit the growth of lung adenocarcinoma A549 cells, liver cancer Blx10-7402 cells and LoVo cells in vitro, and has strong anti-tumor activity (53). The sesquiterpene lactones, chinensiolide A-C, show strong anti-inflammatory and anti-tumor activities (54, 55). The pharmacological studies of the above active ingredients were in accordance with the results of I. chinensis network analysis, which was speculated to be an important component of I. chinensis to exert drug effects, and to a certain extent, it provided the value of medical research for the treatment of diseases.
Molecular docking technology can analyze the optimal binding sites between active components and targets, providing valuable insights into the mechanisms of drug action in treating diseases. Lin Shenghua and others (56) conducted molecular docking on the top 6 targets and the top 3 active components of Xuefu Zhuyu oral liquid for anti-thrombotic activity. The binding energies were between −5 and − 9.5KJ/mol, indicating that the active components of Xuefu Zhuyu oral liquid effectively bind with disease-related targets. Through molecular docking technology, the docking results between the main active components of 7 kinds of I. chinensis found in this study and key targets were pretty good, with binding energies concentrated between −7 and -9KJ/mol, indicating that the main active components of I. chinensis and key targets have good affinity, providing a basis for future drug design.
GO and KEGG analysis revealed that the biological processes of I. chinensis were mainly enriched in positive regulation of transcription from RNA polymerase II promoter, signal transduction, response to xenobiotic stimulus, positive regulation of gene expression, positive regulation of transcription, DNA-templated, response to drug, negative regulation of transcription from RNA polymerase II promoter, protein phosphorylation, negative regulation of apoptotic process, peptidyl-serine phosphorylation, etc. The potential action pathways of I. chinensis included 103 pathways of Pathways in cancer, Chemical carcinogenesis-receptor activation, PI3K-Akt signaling pathway, Hepatitis B, Proteoglycans in cancer, Lipid and atherosclerosis, etc. AKT1, TNF, EGFR and other proteins that regulated tumor cell proliferation, migration, apoptosis, inflammatory expression, immune response, MAPK1, MMP9, ESR1 and other proteins participated in nerve cell regulation, brain tissue repair, and regulation of vascular function were also at the center of the PPI network, indicating that I. chinensis may play a role in promoting tumor cell death, regulating blood lipids, and protecting the heart and brain by regulating these targets. In addition, the active compounds of I. chinensis could regulate inflammatory pathways, and the pathways with a high degree of significance include TNF signaling pathway, PI3K/AKT signaling pathway, and Estrogen signaling pathway, and the inflammatory response was related to the occurrence and development of diseases. Tumor necrosis factor-α (TNF-α) is a cytokine involved in systemic inflammation and is also among the many cytokines that cause acute phase responses. Extracellular TNF- α bound to the TNF-R1 receptor on the cytosolic membrane and indirectly activates CASP8, which in turn activated CASP3, and thus had an impact on apoptosis and plaque instability (57, 58). In addition, lignans and lignans 7-O-β-D-glucoside scavenged free radicals and enhanced antioxidant enzyme activity. It was evident that the active compounds of I. chinensis cought have myocardial protective and antiatherosclerotic effects by modulating the above-mentioned inflammatory pathways (59).
The DO analysis found that, I. chinensis could be applied to tumors such as hereditary breast, ovarian cancer, cell type benign neoplasm, female reproductive organ cancer, adenoma, biliary tract cancer, renal cell carcinoma, bone cancer, connective tissue cancer. It could also be applied to diseases such as autosomal dominant disease, brain disease, diarrhea, atherosclerosis, arteriosclerotic cardiovascular disease, leiomyoma, osteoporosis, Alzheimer’s, tauopathy, lymphoblastic leukemia, obesity, infertility, etc. It could be seen that the I. chinensis could be mainly used to treat cancer related diseases, visceral system diseases, reproductive system diseases, nervous system diseases, musculoskeletal system diseases, lymphatic system diseases, etc.
In conclusion, network pharmacology research can shorten the research process of molecular targets (60). Researchers can use network pharmacology studies to help discover effective and specific compounds for treating diseases (61). Based on meta analysis, network pharmacology and molecular docking methods, this study preliminarily explored the active components, key targets, docking binding energy, signal pathways and disease prediction of I. chinensis. It further verified the feasibility of I. chinensis as a drug to treat many diseases, and provided a basis for exploring the specific mechanism in treating diseases and developing new drugs. At the same time, current study found that in the five articles included, the extract of I. chinensis had a good effect on the improvement of blood lipid indicators. However, due to the small sample size, general literature quality, and fewer types of diseases studied, the reliability of outcomes had been affected to some extent, so more in vitro and in vivo experiments are needed to verify in the future.
ZN: Resources, Writing – original draft. ZM: Writing – original draft, Conceptualization. XQ: Data curation, Writing – original draft. YG: Formal analysis, Software, Writing – review & editing. CR: Methodology, Project administration, Writing – original draft, Writing – review & editing. YW: Resources, Writing – original draft. YY: Investigation, Writing – original draft.
The author(s) declare that financial support was received for the research, authorship, and/or publication of this article. This work was supported by the National Natural Science Foundation of China (82201627), the Military Medicine Upgrade Program of Air Force Military Medical University (2020SWAQ04), Shaanxi Provincial Innovation Capacity Support Programme (2023-CX-PT-33), Shaanxi Provincial Natural Science Basic Research Programme (2024JC-ZDXM-60, 2022JQ-820), Shaanxi Provincial Natural Science Basic Research Programme Key Projects (2024JC-ZDXM-51), and Xijing Hospital Clinical New Technology (2023XJSY27).
The authors declare that the research was conducted in the absence of any commercial or financial relationships that could be construed as a potential conflict of interest.
All claims expressed in this article are solely those of the authors and do not necessarily represent those of their affiliated organizations, or those of the publisher, the editors and the reviewers. Any product that may be evaluated in this article, or claim that may be made by its manufacturer, is not guaranteed or endorsed by the publisher.
1. ^ https://old.tcmsp-e.com/tcmsp.php
2. ^ http://herb.ac.cn/Search/
3. ^ https://pubchem.ncbi.nlm.nih.gov/
4. ^ http://swisstargetprediction.ch/
6. ^ https://cn.string-db.org/
7. ^ https://david.ncifcrf.gov/
1. Tai, WJ, Chen, SK, and Gan, XH. Flavonoid extraction from Ixeris chinensis (Thunb) Nakai and its antioxidant activity with DPPH. J Guizhou Norm Univ. (2016) 32:59–63. doi: 10.13391/j.cnki.issn.1674-7798.2016.12.014
2. Cui, GZ, Li, F, and Liu, Y. Literature research of the sow thistle. Chin J Experiment Formulas. (2012) 18:360–2. doi: 10.13422/j.cnki.syfjx.2012.23.021
3. Hou, YG, Wang, F, Li, HY, Tian, C, and Yang, L. Optimization on the extraction conditions of flavonoids from Lactuca Tatarica using response surface methodology. J Liaoning Univ. (2019) 39:21–6. doi: 10.3969/j.issn.1672⁃6952.2019.02.004
4. Song, YX, Chen, YQ, and Min, YT. Purification of flavonoids from variegated Picris hieracioides using macroporous resin and their antioxidant activity study. Food Sci Technol. (2023) 48:154–60. doi: 10.13684/j.cnki.spkj.2023.09.016
5. Xu, Z, Jin, YT, Zhao, ZY, Wang, Y, Gong, BY, Zhou, HF, et al. Study on the compatibility rules and action mechanism of Chinese herbal compound in the treatment of ulcerative colitis based on national patent database and network pharmacology. Tianjin J Traditional Chin Med. (2023) 40:219–27. doi: 10.11656/j.issn.1672-1519.2023.02.16
6. Wang, ZM. Anti-inflammatory effects and mechanism of water extract of Sonchus oleraceus. Shijiazhuang: Hebei Medical University (2015).
7. Li, MY, Chao, B, Zhou, T, and Zhang, JP. Effects of Sonchus oleraceus on inflammatory factors and HMGB1_TLR4_NF-κB signaling pathway in mice with pneumonia. J Chin Med Materials. (2023) 46:911–7. doi: 10.16431/j.cnki.1671-7236.2023.12.044
8. Cao, Y, Wang, P, Liu, S, and Yang, H. Exploration of the mechanism of action of modified Zhixiao Pingchuan decoction in the treatment of bronchial asthma based on network pharmacology. China Med Pharmacy. (2024) 14:129–32. doi: 10.20116/j.issn2095-0616.2024.05.29
9. Zhou, L, and Liu, MH. Exploration of the mechanism of action of honeysuckle in the treatment of 2019-nCoV infection with myocarditis based on network pharmacology. Hunan J Trad Chin Med. (2024) 40:160–7. doi: 10.16808/j.cnki.issn1003-7705.2024.03.034
10. Xie, J, Gao, S, Li, L, Xu, YL, Gao, SM, and Yu, CQ. Research progress and application strategy on network pharmacology in Chinese materia medica. Chin Tradit Herb Drug. (2019) 50:2257–65. doi: 10.7501/j.issn.0253-2670.2019.10.001
11. Fu, K. Prediction and screening of active components in Chinese medicine for SLE treatment using semi-flexible molecular docking. Nanchang, Jiangxi: Jiangxi University of Traditional Chinese Medicine (2019).
12. Ding, J, Zheng, P, Sun, YY, Wang, XR, and Feng, YC. Analysis of Wumei pills in treating chronic digestive system diseases with concept of "treating different diseases with same method" based on network pharmacology and molecular docking. China J Chinese Materia Medica. (2022) 47:4164–76. doi: 10.19540/j.cnki.cjcmm.20220412.401
13. Han, KY, Dang, H, and Zhang, H. Efficacy of repetitive transcranial magnetic stimulation on cognitive dysfunction after traumatic brain injury: a meta-analysis. Chin J Rehabil Theory Pract. (2021) 27:1282–90. doi: 10.3969/j.issn.1006⁃9771.2021.11.007
14. Li, QH, Ju, LW, Wang, N, and Liu, ZZ. Analysis of the effect of ginsenoside Rh1 on gene expression in breast cancer SKBR3 cells by KEGG biological pathway enrichment analysis. Chinese J Med. (2019) 25:36–41. doi: 10.13862/j.cnki.cn43-1446/r.2019.20.010
15. Guo, P, and Guan, LK. The significance of JAK1 signal pathway in cardiovascular and cerebrovascular diseases. Jilin Med J. (2017) 38:175–8. doi: 10.3969/j.issn.1004-0412.2017.01.071
16. Zheng, J, Li, XS, Peng, F, Luo, J, and Tang, XH. Chitosan modulates neointimal hyperplasia in arteriovenous fistula models in rabbits by downregulating serum MCP-1 and VEGF-A expression. Chin J Gerontol. (2023) 43:336–41. doi: 10.3969/j.issn.1005-9202.2023.03.033
17. Minamino, T, Yoshida, T, Tateno, K, Miyauchi, H, Zou, Y, Toko, H, et al. Ras induces vascular smooth muscle cell senescence and inflammation in human atherosclerosis. Circulation. (2003) 108:2264–9. doi: 10.1161/01.CIR.0000093274.82929.22
18. Yang, H, and Zeng, CP. Effects of water extract from Scorzonera sinensis Lipsch, Pteridium aquilinum and Sonchus oleraceus L. on plasma-lipids metabolism in mice fed high fats diet. Food Res Develop. (2015) 36:11–3. doi: 10.3969/j.issn.1005-6521.2015.06.003
19. Lou, CX, Wang, Y, Dai, LL, Long, SX, and Huang, XQ. Effects of three traditional Chinese medicine ingredients on oxidized low-density lipoprotein-induced injury in human aortic endothelial cells. China Pharmac. (2024) 33:39–42. doi: 10.3969/j.issn.1006-4931.2024.04.010
20. Ni, Q, and Sun, P. Research Progress on the correlation between LOX-1 and Tumors. Advances Clin Med. (2023) 13:8319–24. doi: 10.12677/acm.2023.1351164
21. Wang, MQ, Liu, KK, Liu, XY, Zhang, XL, and Li, HX. Research progress on inhibitors of the PI3K/Akt signaling pathway in the treatment of gynecological malignant tumors. World J Cancer Res. (2024) 14:35–40. doi: 10.12677/WJCR.2024.141006
22. Liu, QB, and Li, HW. Advanced glycation end products and atherosclerosis. Chin J Cardiovasc Diseases. (2018) 23:87–91. doi: 10.3969/j.issn.1007-5410.2018.01.020
23. Cao, NB, and Yu, WX. Role of Connexin 43 in oxidative damage of periodontal tissues through regulation of the JNK/NF-κB signaling pathway. Changchun: School of Stomatology, Jilin University (2023).
24. Chen, G. The mechanism of mmLDL impaired endothelium-dependent relaxation in mice mesenteric arteries. Hengyang: University of South China (2014).
25. Chu, MY, Lu, YT, Liu, T, and Liu, J. Expression and clinical application prospects of tumor necrosis factor alpha in autoimmune diseases. J Hebei Med Univ. (2020) 41:853–63. doi: 10.3969/j.issn.1007-3205.2020.03.027
26. Zhu, BJ, and Zhou, XD. Study of the PI3K/AKT pathway in lung cancer metastasis and drug resistance. Chin J Lung Cancer. (2011) 14:689–94. doi: 10.3779/j.issn.1009-3419.2011.08.10
27. Krishnankutty, A, Kimura, T, Saito, T, Aoyagi, K, Asada, A, Takahashi, S, et al. In vivo regulation of glycogen synthase kinase 3β activity in neurons and brains. Sci Rep. (2017) 7:8602. doi: 10.1038/s41598-017-09239-5
28. Mohammed, MH, Hamed, AN, Sayed, AM, Afifi, AH, Rateb, ME, Thissera, B, et al. Antiproliferative potential of Dypsis decaryi seeds supported by metabolic profiling and molecular docking (J/OL). J Herbal Med. (2024) 44:100846. doi: 10.1016/j.hermed.2024.100846
29. Cheng, LH. The study of the preparation of Ixeris Chinensis (thumb.) Nakai total flavonoids extract and the effect and mechanism to T2DM. Haerbin: Heilongjiang University Of Chinese Medicine (2016).
30. Jin, W, Cho, S, Laxi, N, Bao, T, Dai, L, Yu, H, et al. Hepatoprotective effects of Ixeris chinensis on nonalcoholic fatty liver disease induced by high-fat diet in mice: an integrated gut microbiota and metabolomic analysis. Molecules. (2022) 27:3148. doi: 10.3390/molecules27103148
31. Zhao, J. Formulation of plant milk-tea products and comparing of its lipid-lowering activity. Changchun: Jilin Agricultural University (2015).
32. Zheng, QS, Sun, XL, Xu, B, Song, M, and Wang, CH. Protective effects of luteolin-7-glucoside against liver injury caused by carbon tetrachloride in rats. Pharmazie. vol. 5 (2004).
33. Liu, HX, Pei, XP, Pei, MR, Li, HF, and Xue, YM. Research on anti-inflammatory and hepatoprotective activities between ixeris chinensis and s.brachyotus. Taiyuan: Journal of Shanxi University of Traditional Chinese Medicine (2016) 17:19–20+56.
34. Meng, FC, and Tang, LD. Challenges and prospect in research of Chinese materia medica network pharmacology. Chin Tradit Herb Drug. (2020) 51:2232–7. doi: 10.7501/j.issn.0253-2670.2020.08.034
35. Huang, XF, Yu, GZ, and Tong, JJ. Analysis of the pharmacological mechanism of action of Chen pi based on network pharmacology. Chin Trad Patent Med. (2019) 41:3038–45. doi: 10.3969/i.iSsn.1001-1528.2019.12.043
36. Gao, SY, and Li, D. Advances in tumor necrosis factor and cancer-related research. Chin Pharm Bull. (2020) 36:1209–13. doi: 10.3969/j.issn.1001-1978.2020.09.006
37. Huang, JX, and Wang, H. Targeted therapy and mechanism of drug resistance in non-small cell lung Cancer with epidermal growth factor receptor gene mutation. Chin J Lung Cancer. (2022) 25:183–92. doi: 10.3779/j.issn.1009-3419.2022.101.05
38. Han, XW. Expression and significance of ESR1 and ACOX2 in hepatocellular carcinoma. Hefei: Anhui Medical University (2021).
39. Bin, W. Expression of Src gene in glioma and its effect on the malignant biological behavior of glioma cell. Shanghai: Naval Medical University (2016).
40. Liu, CL. Association between polymorphisms of PTGS2 gene and Clinicopathologic characteristics and prognosis to hepatocellular carcinoma. Nanning: Guangxi Medical University (2013).
41. Wu, YL. Association of Mitogen-activated protein kinase 1 (MAPK1) and mitogen-activated protein kinase 4(MAP2K4)gene polymorphism with risk of ischemic stroke and IS-related traditional Chinese medicine syndrome. Nanning: Guangxi University of Chinese Medicine (2017).
42. Liu, C, Yan, MM, Shao, S, Wu, CY, Fu, ML, Tian, S, et al. Chemical constituents and pharmacological effects of Ixeris chinensis. Chin J Exp Tradit Med Formulae. (2015) 21:231–4. doi: 10.13422/j.cnki.syfjx.2015200231
43. Chen, L, Lin, X, Xiao, J, Tian, Y, Zheng, B, and Teng, H. Sonchus oleraceus Linn protects against LPS-induced sepsis and inhibits inflammatory responses in RAW264.7 cells. J Ethnopharmacol. (2019) 236:63–9. doi: 10.1016/j.jep.2019.02.039
44. Chen, L, Lin, X, Fan, X, Qian, Y, Lv, Q, and Teng, H. Sonchus oleraceus Linn extract enhanced glucose homeostasis through the AMPK/Akt/GSK-3β signaling pathway in diabetic liver and HepG2 cell culture. Food Chem Toxicol. (2020) 136:111072. doi: 10.1016/j.fct.2019.111072
45. Wang, JL, Lv, D, Liang, XY, Zhao, M, and Zhang, SJ. Study on Chemical Constituents of the Ixeris chinensis. J Chin Med Mater. Qiqihar University 34: 1706–1708. (2011).
46. Wang, HY, Ben, LF, Jiao, LH, Ma, Y, and Su, XH. Research status of Total flavonoids from Sonchus Oleraceus L. Shandong Chem Industry. (2018) 47:64–5. doi: 10.19319/j.cnki.issn.1008-021x.2018.21.024
47. Guo, LM, Lv, JL, and Zhang, LB. Research progress on anti-inflammatory mechanism of natural sesquiterpenoids. China J Chin Materia Medic. (2018) 43:3989–99. doi: 10.19540/j.cnki.cjcmm.20180726.013
48. Lan, GC, Wu, ZL, Wang, SM, Wu, ZL, Wang, SM, Li, HL, et al. A new triterpenoid and a new steroid from roots of Vladimiria souliei. Chin Tradit Herb Drug. (2019) 50:793–7. doi: 10.7501/j.issn.0253-2670.2019.04.001
49. Lin, S, Qin, HZ, Deng, LY, Li, ZY, Xie, FF, Zhang, M, et al. Apigenin induces apoptosis of human colorectal Cancer CL187 cells via PI3K/Akt and MAPK Signaling pathways. Chin J Exp Tradit Med Formulae. (2022) 28:97–104. doi: 10.13422/j.cnki.syfjx.20221122
50. Chen, XD, Zhong, WL, Yan, PY, Kuang, JN, Qiao, KL, Sun, T, et al. Apigenin inhibits mouse liver fibrosis via PDK1/AKT signaling pathway. Chinese Pharmacol Bull. (2022) 38:1010–6. doi: 10.12360/CPB202106091
51. Han, NX, Sun, YL, Sheng, S, and Zhang, W. Mechanisms of lignocaine regulation of oxidative stress and inflammation. Chin J Anim Nutr. (2022) 34:2856–61. doi: 10.3969/j.issn.1006-267x.2022.05.015
52. Zhou, L, Xie, YY, Li, J, Wang, XJ, and Mou, YL. Protective effects of Luteolin-7-O-β-D-glucopyranoside on hypoxic-ischemic injury of myocardial cells in neonatal rats. Trad Chin Drug Res Clin Pharmacol. (2008) 4:259–61. doi: 10.19378/j.issn.1003-9783.2008.04.006
53. Zhou, L, Zhao, Y, Wang, Y, Li, LB, Zhang, SJ, and Li, T. In vitro anti-tumor activity of extract Chinensiolide a from the Ixeris Chinensis. Chin Med Innov. (2015) 12:109–11. doi: 10.3969/j.issn.1674-4985.2015.34.034
54. Zhang, S, Zhao, M, Bai, L, Hasegawa, T, Wang, J, Wang, L, et al. Bioactive Guaianolides from Siyekucai (Ixerischinensis). J Nat Prod. (2006) 69:1425–8. doi: 10.1021/np068015j
55. Zhang, S, Wang, J, Xue, H, Deng, Q, Xing, F, and Ando, M. Three new Guaianolides from Siyekucai (Ixeris chinenseis). J Nat Prod. (2002) 65:1927–9. doi: 10.1021/np0202632
56. Lin, SH, Xue, C, Ma, HL, Fan, W, Shen, CM, Chen, JY, et al. Revealing the active components and mechanisms of action of Xuefu Zhuyu oral liquid against thrombosis based on network pharmacology and molecular docking technology. Shandong Sci. (2023):1–8. doi: 10.3976/j.issn.1002-4026.20230130
57. Qing, P, Zhao, JF, Zhao, CH, Hu, J, Lin, YL, and He, KJ. Effect of acupuncture on cancer-related fatigue and its impact on serum levels of CRP, IL-6, TNF-α, and sTNF-R1. Chin Acupuncture Moxibust. (2020) 40:505–7. doi: 10.13703/j.0255-2930.20190423-k0002
58. Wang, L, Du, F, and Wang, X. TNF-alpha induces two distinct caspase-8 activation pathways (J/OL). Cell. (2008) 133:693–703. doi: 10.1016/j.cell.2008.03.036
59. Jang, WY, Kim, M-Y, and Cho, JY. Antioxidant, anti-inflammatory, anti-menopausal, and anti-Cancer effects of Lignans and their metabolites. Int J Mol Sci. (2022) 23:15482. doi: 10.3390/ijms232415482
60. Mohammed, MHH, Hamed, ANE, Elhabal, SF, Mokhtar, FA, Abdelmohsen, UR, Fouad, MA, et al. Metabolomic profiling and anti-proliferative activities of Dypsis leptocheilos ethanol extract and its green synthesized silver nanoparticles supported by network pharmacology analysis. S Afr J Bot. (2023) 161:648–65. doi: 10.1016/j.sajb.2023.08.026
61. Mohammed, MHH, Hamed, ANE, Elhabal, SF, Abdelmohsen, UR, Fouad, MA, and Kamel, MS. Chemical composition and anti-proliferative activities of Hyophorbe lagenicaulis aerial parts and their biogenic nanoparticles supported by network pharmacology study. S Afr J Bot. (2023) 156:398-410. doi: 10.1016/j.sajb.2023.03.018
Keywords: Ixeris chinensis, network pharmacology, molecular docking, meta analysis, drug development
Citation: Ni Z, Ma Z, Qiao X, Guo Y, Ruan C, Wang Y and Yang Y (2024) Prediction and analysis of components and functions of Ixeris chinensis based on network pharmacology and molecular docking. Front. Med. 11:1360966. doi: 10.3389/fmed.2024.1360966
Received: 24 December 2023; Accepted: 29 May 2024;
Published: 27 June 2024.
Edited by:
Hari S. Sharma, Erasmus Medical Center, NetherlandsReviewed by:
Himansu Kumar, University of Texas Health Science Center at Houston, United StatesCopyright © 2024 Ni, Ma, Qiao, Guo, Ruan, Wang and Yang. This is an open-access article distributed under the terms of the Creative Commons Attribution License (CC BY). The use, distribution or reproduction in other forums is permitted, provided the original author(s) and the copyright owner(s) are credited and that the original publication in this journal is cited, in accordance with accepted academic practice. No use, distribution or reproduction is permitted which does not comply with these terms.
*Correspondence: Cailian Ruan, eWFkeGpwMjAyMTAzMjVAMTYzLmNvbQ==; Yayun Wang, d2FuZ3lheXVuZm1tdUBxcS5jb20=; Ying Yang, eXlpbmcxOTMwQDE2My5jb20=
Disclaimer: All claims expressed in this article are solely those of the authors and do not necessarily represent those of their affiliated organizations, or those of the publisher, the editors and the reviewers. Any product that may be evaluated in this article or claim that may be made by its manufacturer is not guaranteed or endorsed by the publisher.
Research integrity at Frontiers
Learn more about the work of our research integrity team to safeguard the quality of each article we publish.