- 1Department of Otolaryngology-Head and Neck Surgery, Faculty of Medicine and Graduate School of Medicine, Hokkaido University, Sapporo, Japan
- 2Department of Forensic Medicine, Faculty of Medicine and Graduate School of Medicine Hokkaido University, Sapporo, Japan
- 3Center for Medical Education and International Relations, Hokkaido University, Sapporo, Japan
- 4Department of Surgery–Otorhinolaryngology Head and Neck Surgery, Central Adelaide Local Health Network and the University of Adelaide, Adelaide, SA, Australia
Introduction: Traditionally formal assessment of surgical skills has not been part of a surgeon’s accreditation process. The widely adopted apprentice model of “on-the-job training” does create additional risk for the patients. In the past surgical training has used cadavers, but these are expensive, require dedicated wet-lab facilities and are in increasingly short supply. In many countries religious and cultural practices also preclude cadaveric use. Recent 3D-printed technology allows mass reproduction of high-fidelity 3D models. In this study, we examined the utility of 3D sinus models compared to cadaver dissection for surgical skill assessment for endoscopic sinus surgery (ESS).
Materials and methods: A total of 17 otolaryngologists performed Endoscopic Sinus Surgery (ESS) on 3D printed sinus models and then repeated these procedures on cadavers. Their surgical performance was assessed with the Objective Structured Assessment of Technical Skills (OSATS) score for ESS and time was taken to complete an ESS procedure. Their performance on the 3D models and cadavers was compared.
Results: There were no significant differences in the OSATS score between 3D models and cadavers (50.41 ± 13.31 vs. 48.29 ± 16.01, p = 0.36). There was a strong positive correlation between the score in 3D models and those in cadavers (r = 0.84, p < 0.001). No significant differences were found in time for a mini-ESS (21:29 ± 0:10 vs. 20:33 ± 0:07, p = 0.53). There were positive correlations between 3D models and cadavers in time taken for a mini-ESS (r = 0.55, p = 0.04).
Conclusion: The surgical performance on the 3D models was comparable to that on cadavers. This supports the utility of the 3D models as an inexhaustible alternative for cadavers in ESS surgical skill assessment.
1 Introduction
Surgery is a relatively high-risk activity and the training for surgery is often compared to that needed to fly commercial aircraft or manage a nuclear power station (1, 2). Most sinus surgery is performed for inflammatory or benign pathology to improve quality of life in individuals suffering from chronic rhinosinusitis (CRS). Endoscopic sinus surgery, a standard surgical treatment for medically resistant CRS (3), involves opening ostia and functional drainage units in the sinuses by resecting bony septations within the paranasal sinuses. Incomplete resection increases the risk of disease recurrence, while surgical error can result in severe complications given the close proximity of the sinuses to the orbit and the skull base. The efficacy and safety of ESS largely depends on surgeons’ surgical skills emphasizing the importance of the training of surgeons. Traditionally, such skills have been acquired by trainees through “on-the-job training.” Such a training model inevitably exposes patients to poorer surgical outcomes and a higher risk of complications, particularly during the steep learning curve of ESS surgery (4).
In other high-risk industries, such as aviation and nuclear power, simulation is used for training and also assessment of skills, with a certain level of demonstrated skills required before pilots or scientists are allowed to practice in the “real world” (4, 5). In the airline industry, a certain number of hours on the simulator are required not only during the training process but also as a part of routine certification and recertification throughout a pilot’s career (6, 7).
Similarly, in surgery, having such a credentialing process to assess all surgical trainees’ skills in simulation surgeries before initiating actual surgeries would be beneficial and potentially reduce the risks to the patients. To date, if surgeons wish to practice new techniques or learn/refine their skills, they can attend cadaveric courses. However, surgery on cadavers is not routinely as part of the surgical accreditation and licensing (8). This is in part due to limited availability and access to cadavers and wet lab facilities, the significant expense associated with their use and the fact that cadaveric anatomy is highly variable making standardized training/assessment impossible.
The advent of 3D technologies has been contributing to our daily life, also to many surgical specialties (9). Recent advances in 3D printing technology and polymer materials has paved the way for high fidelity 3D printed models with variations on complex anatomy produced from actual patient CT scans which create a set of challenging surgical situations through which surgical trainees can gain skills but which also allow an opportunity for standardized assessment of surgical skills (10). These models have very similar tactile feedback during surgery and have the elasticity of real mucosa and cartilage. Several studies have demonstrated that 3D anatomical models contribute to medical education for undergraduate students (11), optimization of surgical planning (12) and preoperative device selection (13) and of postoperative treatment (14). We recently reported on the usefulness of newly designed 3D sinus models in ESS skill acquisition and to estimate mental workload during surgeries (15, 16).
In this study, we examined the utility of the 3D sinus models as a medium in ESS skill assessment, by comparing ESS surgical performance for the 3D models and cadavers by surgical trainees.
2 Materials and methods
2.1 Participants
Data for this study was collected concurrently with the previously published studies regarding the validation of 3D-printed sinus models for ESS training (15). The institutional review board approved the present study (no. 018-043). A total of seventeen otolaryngologists in Hokkaido University Hospital voluntarily participated in the present study. The written informed consent was obtained from all the participants.
2.2 ESS for 3D models and for cadavers
Standard ESS instruments (Storz, Tuttlingen, Germany), with a 4-mm rigid nasal endoscope and a monitor (Telepac, Storz, Tuttlingen, Germany), and a powered microdebrider (Medtronic, Jacksonville, FL) were used.
Participants were allocated 45 min to perform full-house ESS (middle meatus antrostomy; MMA, anterior-and posterior-ethmoidectomy, sphenoidotomy, and frontal sinusotomy) on the left side of 3D-printed sinus models (PJW-N2-V2, Fusetec, Adelaide, South Australia), printed from the dicoms of CT scans of actual patients with chronic rhinosinusitis (Figure 1). The validation of the 3D models and the mock surgeries with them for ESS simulation is reported in detail in the previous paper (15). To evaluate the progress within the allocated time under the standardized condition, the order of the surgical steps to be performed, as well as the surgical instrument to be used in each step, was set and performed in the following order, uncinectomy, MMA, anterior-and posterior-ethmoidectomy, sphenoidotomy, and finally, frontal sinusotomy. Within 48 h after their 3D model surgeries, participants performed full-house ESS for cadavers with the same equipment and under the same conditions. All surgeries were video recorded (15).
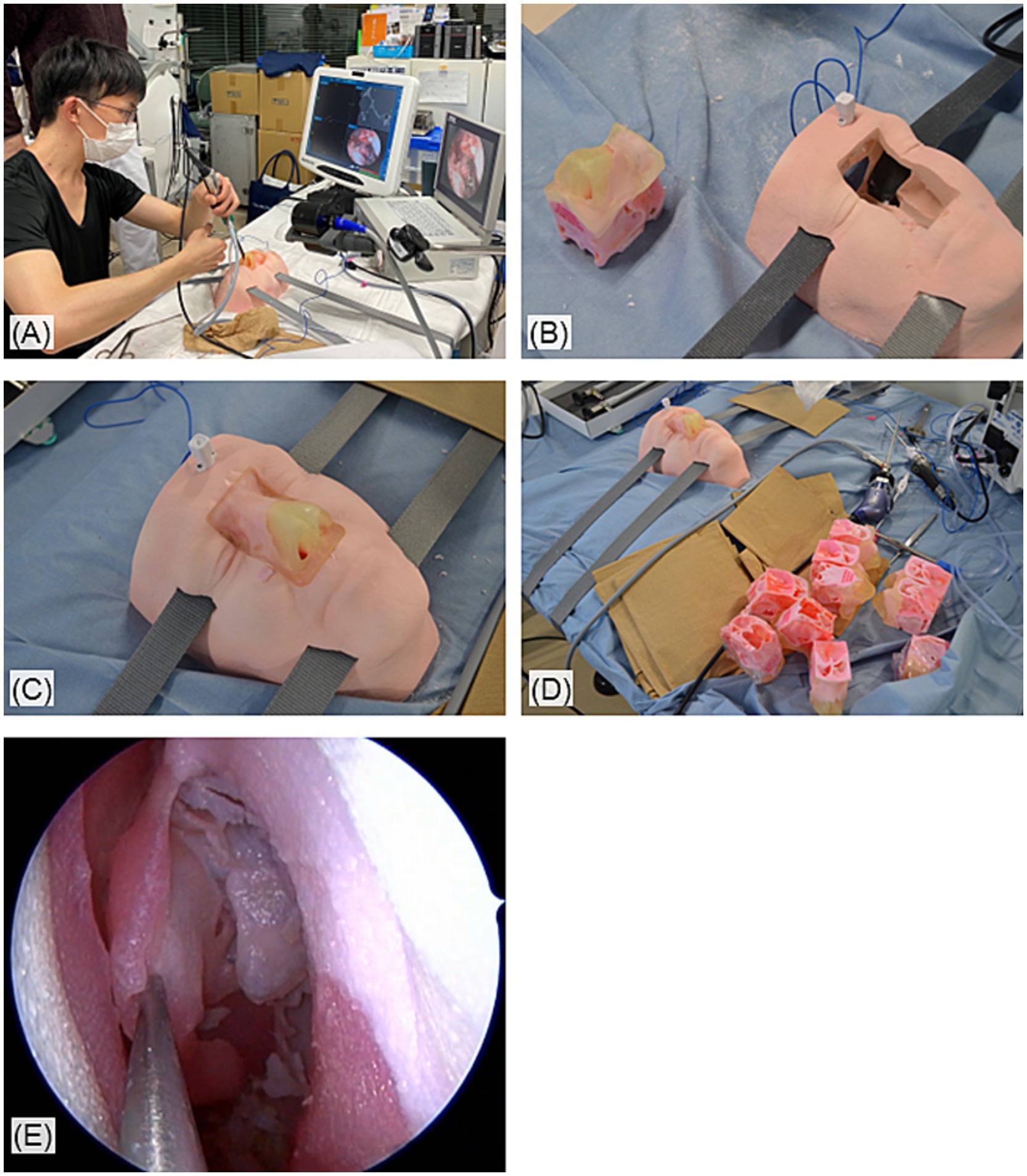
Figure 1. Overview of the simulation surgeries with the 3D-printed sinus models. (A) The setup of the simulation surgeries. (B) The 3D sinus models and its basement. (C) The models were inserted into and hold in the basement which mimics a human face. (D) The 3D models can be mass produced. (E) Endoscopic view of 3D-printed sinuses during the simulation surgeries.
2.3 Assessment of surgical performance
Participants’ surgical performance were assessed with the Objective Structured Assessment of Technical Skills (OSATS) score for ESS (17). Briefly, the scoring system was designed for evaluation of the performance during every step in ESS beginning with handling the endoscope and intranasal preparation going all the way to perform a frontal sinusotomy. The whole process in ESS is subdivided into 21 tasks, and each task is evaluated with a 5-point Likert rating scale with “unable to perform” for 1, “able to perform majority” for 3, and “performs easily with good flow” for 5, respectively (Table 1). In the present study, the scoring for three tasks in the intranasal preparation were excluded because vasoconstrictor and local anesthesia was not required for 3D models and cadavers; the remaining 18 tasks were evaluated with the perfect score of 90. The OSATS score was blindly assessed by other two rhinologists (MS and YN) based on the recorded video. High inter-rater reliability has been confirmed, and the detail is described in previous paper (15).
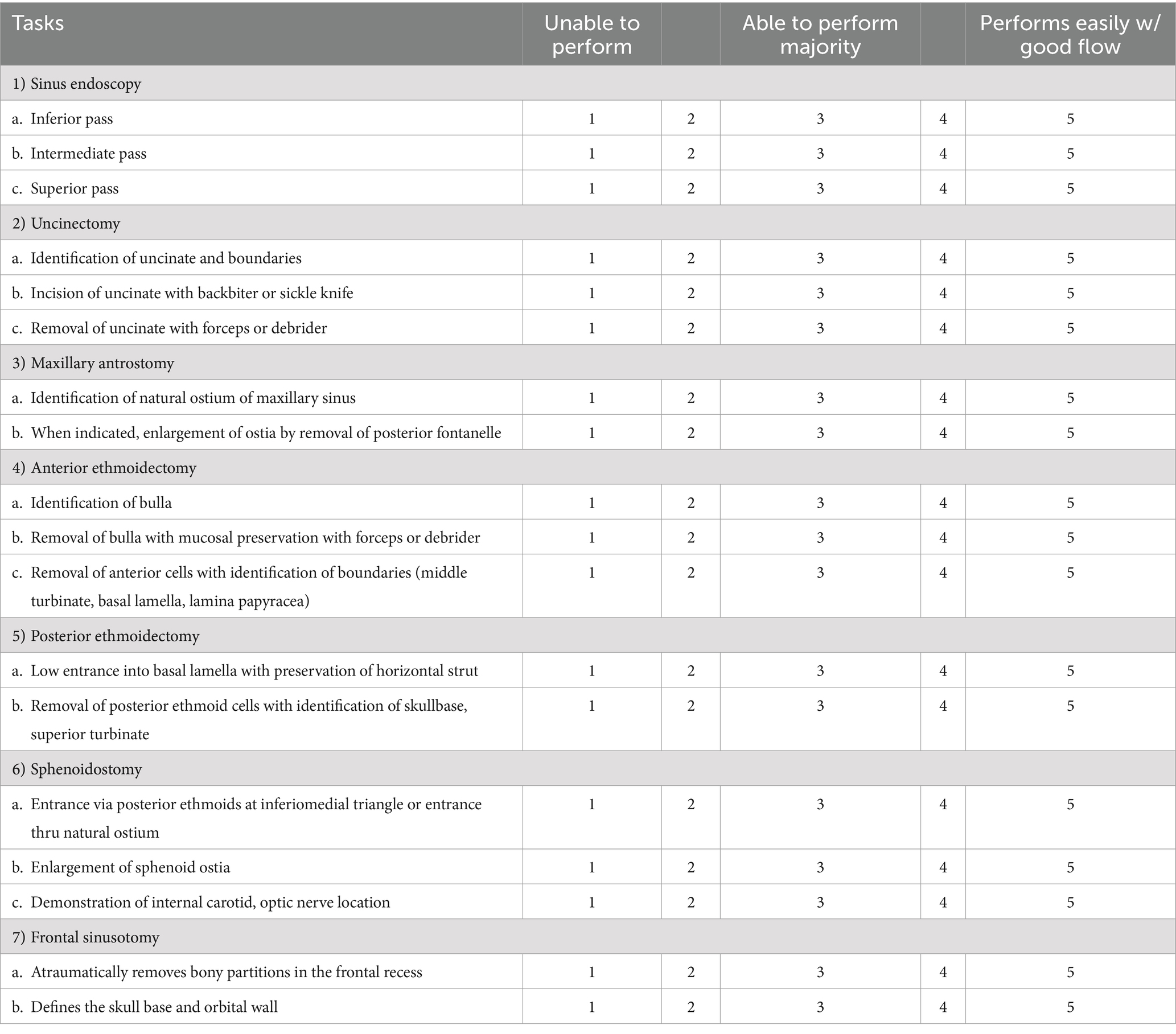
Table 1. The scoring system used in this study that was modified from the Objective Structured Assessment of Technical Skills (OSATS) score for ESS originally reported by Lin et al. (17).
Progress status of surgeries was evaluated with the surgical procedures that the participants had completed by the end of the allocated time. For statistical analysis of procedures, the status was converted to the following number (1: none, 2: uncinectomy, 3: MMA, 4: anterior-ethmoidectomy, 5: posterior-ethmoidectomy, 6: sphenoidotomy, 7: frontal sinusotomy and completion of full-house ESS). For example, if a participant had finished posterior-ethmoidectomy and was performing a sphenoidotomy when time was up, the progress was expressed as “3: posterior-ethmoidectomy.” The time taken to complete a mini-ESS (MMA and anterior ethmoidectomy) was also recorded (15). The reason to select a mini-ESS is that the maxillary sinus and anterior ethmoid anatomy are consistent between cadavers, but frontal sinus anatomy is highly variable, which would make it very difficult to compare a frontal sinus dissection between the model and a cadaver.
2.4 Statistical analysis
Shapiro–Wilk tests were applied to evaluate if the data fitted a normal distribution curve. Parametric data and nonparametric data were expressed as mean (±SD) and median with the interquartile range, respectively. Parametric data were assessed with a paired, two-tailed t-test for comparison between surgical performances in 3D models and in cadavers. Similar, nonparametric data were assessed with Wilcoxon test. Pearson (for parametric data) or spearmen coefficients analysis (for nonparametric data) were used to assess correlation between them. p values of less than 0.05 were considered statistically significant. All the analyses were performed by using JMP 11 (SAS Institute Inc.).
3 Results
3.1 Characteristics of the participants and the assessors
The experienced years of the participants and the assessors as otolaryngologists were 2.7 ± 4.0 and 23.0 ± 4.0, respectively. The previous experienced ESS cases of the participants and the assessors were 41.2 ± 117.6 and 700.0 ± 424.3, respectively. Two out of the participants and both of the assessors were officially certified board members of the Japanese otolaryngology society. All the participants belonged or had belonged to the Department of Otolaryngology, Hokkaido University Hospital, where the assessors worked for. None of the participants hold a conflict of interest to participate in this study. Both assessors hold instructor licenses certified by the Japanese otolaryngology Society.
3.2 Comparison on the surgical quality assessment between 3D models and cadavers
A total of 17 surgeries for 3D models and their paired surgeries for cadavers were assessed (Table 2). The OSATS score in 3D models and cadaver was 50.41 ± 13.31 and 48.29 ± 16.01, respectively (Table 2; Figure 2A). There was no significant difference between them (p = 0.36). There was significant positive correlation between OSATS score in 3D models and cadaver (r = 0.82, p < 0.0001, Figure 2B).
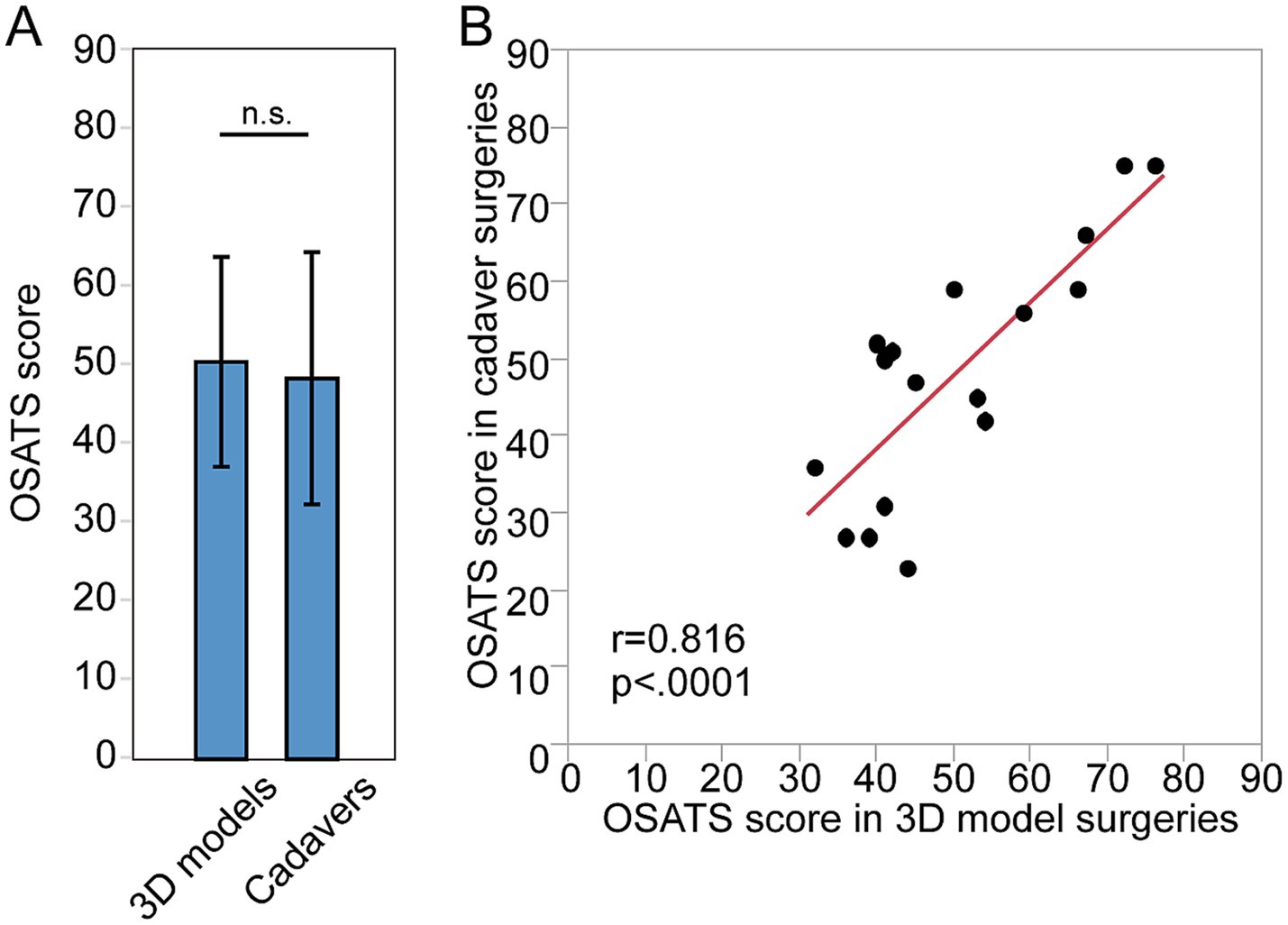
Figure 2. Quality assessment of surgeries done by the same individual surgeons for 3D models and for cadavers. (A) The OSATS score of surgeries done for 3D models and for cadaver. (B) Correlation between the OSATS score in 3D models and in cadaver.
3.3 Assessment on the efficiency of surgeries
As for the progress status of surgeries, there were no significant differences in 3D models and cadavers (p = 0.48, Figure 3A). Significant positive correlation in progress status were found between 3D models and cadavers (r = 0.77, p < 0.01, Figure 3B).
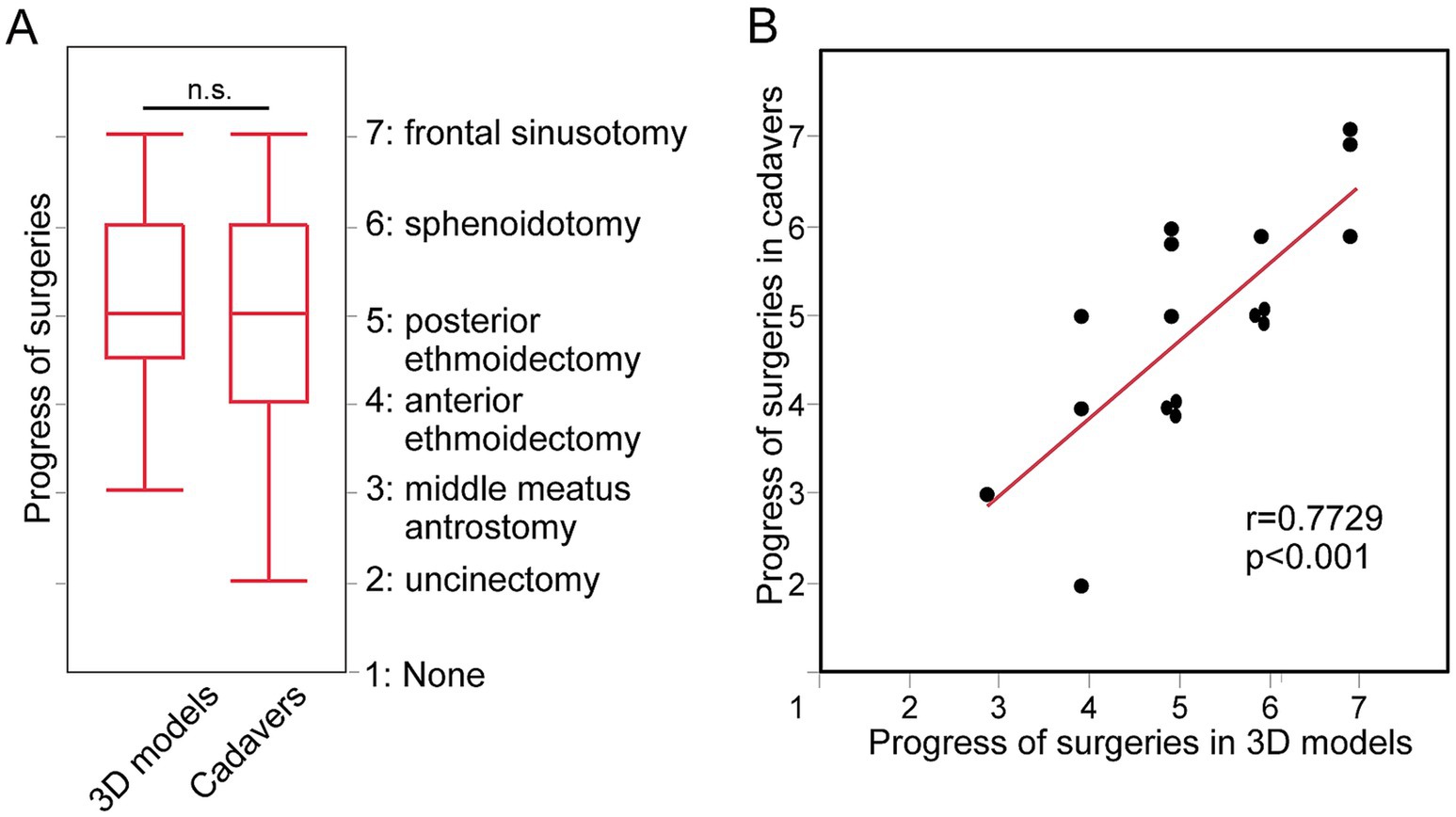
Figure 3. The progress status of surgeries done by the same individual surgeons for 3D models and for cadavers. (A) The progress status of surgeries done for 3D models and for cadavers. (B) Correlation between the status in 3D models and in cadavers.
There were no significant differences in spending time for a mini-ESS (3D models 21:29 ± 0:10 and cadavers 20:33 ± 0:07, p = 0.53, Figure 4A). The time taken to complete a mini-ESS in 3D models were significantly positively correlated to the time taken in cadavers (a mini-ESS r = 0.553, p = 0.0402, Figure 4B).
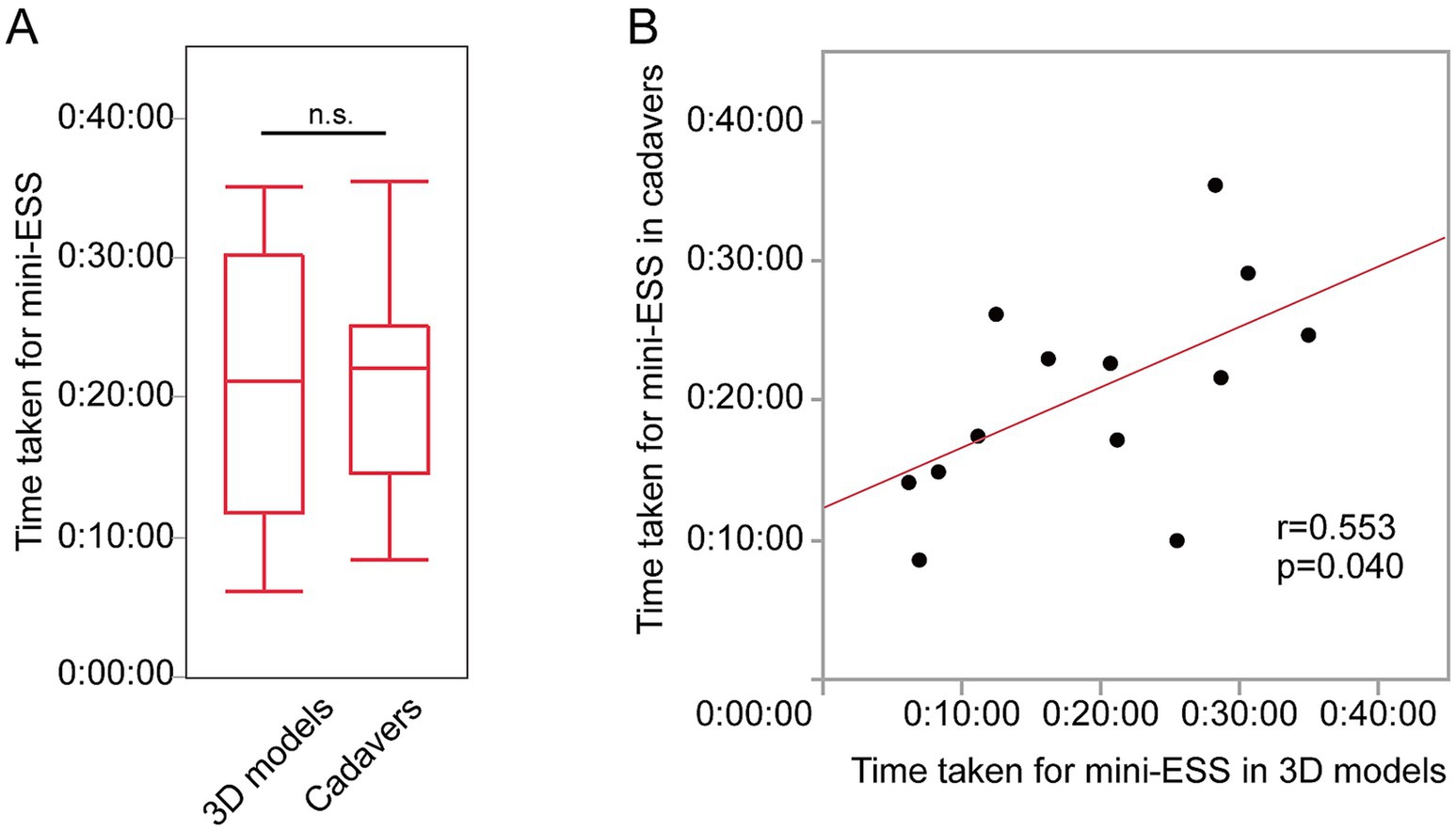
Figure 4. The time taken for mini-ESS by the same individual surgeons for 3D models and cadavers. (A) The time taken for mini-ESS done on 3D models and for cadavers. (B) Correlation between the time for a mini-ESS in 3D models and in cadavers.
4 Discussion
This study examines the use of 3D models compared to cadavers as an assessment tool for surgeons performing ESS. Overall, we observed that the surgical performance of dissectors on 3D models was similar to that seen on cadavers. There was a high level of correlation between the 3D printed model and the cadaver for both quality (OSATS score) of the dissection as well as surgical progression through the dissection steps. The significant benefit of the 3D models is the high level of similarity in tactile feedback and similarity of the surgical experience when dissecting mucosa and bony septations. Unlike a cadaver, the 3D models allow for repetitive training on a model until the acquisition of a surgical skill has been attained and also facilitates for a graduated learning experience by allowing models of different degrees of anatomical complexity to be printed. 3D models are also able to be mass produced allowing for both surgical skill level development and standardized assessment of trainee’s surgical skill.
To date there have been various 3D-printed models developed in the field of otolaryngology (18–31) with most involving the temporal bone. To date there is only one study where 3D printed models have been compared to cadavers (32). In this study as a part of the validation of their 3D sinus models, they found a positive correlation in their ESS score between their 3D models and cadavers. This study focused on the validation of the model rather than on their utility as an assessment medium, and they did not investigate surgical efficiency either (32).
Obtaining a sufficient supply of cadavers has been problematic in many countries around the world (33, 34). If training on cadavers were introduced as a prerequisite to trainees performing surgery on patients, it is estimated that at least 100–150 cadavers per year would be necessary for assessment for ESS skills in Japan. This is an unrealistic number even though Japan is one of the most successful countries to establish highly successful body donation programs for surgical education (33). Furthermore donated cadavers are often unsuitable for ESS training due to prior surgery or disease presence (29.2% of the total cadavers assessed in this study were unsuitable). During this study some trainees, who were assigned cadavers found that the cadavers had already undergone surgery, had distorted anatomy/fractures due to the handling and storage process or were contaminated. These factors were perceived by surgeons to have negatively affected their surgical skill acquisition.
3D models have many advantages over cadavers (15, 35). These include a lack of regulatory and ethical issues with regards to their acquisition, use and disposal. Furthermore, they can be produced en mass with reliable, and predictable anatomy with variable levels of difficulty which make them ideal for the graduated training of surgeons of different levels. It should be noted that disadvantages do exist. These include tissue feel and handling and the lack of neighboring anatomy such as the neurovascular structures, the orbit, brain and infra-temporal fossa. With this said, as polymer technology continues to evolve and improve, many of these limitations are being addressed with the gap closing between cadavers and 3D models.
The utility of 3D models in surgical training and assessment as an alternative to cadavers, as demonstrated in this study, could be developed for other surgical disciplines. Several anatomical models have already been developed for the fields of orthopedic, neurology, and gynecology. Also, same methodologies could be applied to Endonasal Endoscopic Odontoidectomies for cranio-cervical junction pathologies (36), as the surgeries hold similarities with ESS.
There are several limitations to this study. Due to the rarity of cadavers, the number of analyzed subjects was limited. The numbers of surgical trainees undertaking this study was limited, complications occurring in the dissections were not recorded and it is unknown if the skill acquired directly translated to better surgical performance in patients. Ideally, a randomized controlled study will be necessary where surgical performance in actual surgery should be compared to those in 3D models. Despite these limitations, this pilot study illustrates the utility of 3D models as a promising alternative tool to both train surgeons and to assess skill acquisition for ESS accreditation.
5 Conclusion
The surgical performance of trainees in 3D sinus models was similar to that seen in cadavers, which supports the utility of the 3D models in ESS surgical skill training and assessment. With the lack of ethical concerns, the ability to conduct the assessment in any facility, predictability, reproducibility of anatomy for the simulation, and a lack of infectivity, 3D models can be an inexhaustible alternative for cadavers in ESS surgical skill assessment.
Data availability statement
The raw data supporting the conclusions of this article will be made available by the authors, without undue reservation.
Ethics statement
The studies involving humans were approved by Medical Ethics Committee, Faculty of Medicine and Graduate School of Medicine, Hokkaido University. The studies were conducted in accordance with the local legislation and institutional requirements. The participants provided their written informed consent to participate in this study.
Author contributions
MS: Conceptualization, Data curation, Funding acquisition, Investigation, Methodology, Writing – original draft, Writing – review & editing. RW: Investigation, Writing – review & editing. AN: Data curation, Investigation, Writing – review & editing. YN: Data curation, Formal analysis, Investigation, Validation, Writing – review & editing. TS: Data curation, Investigation, Writing – review & editing. SK: Data curation, Formal analysis, Visualization, Writing – review & editing. KM: Data curation, Writing – review & editing, Formal analysis. MM: Writing – review & editing, Conceptualization. DH: Validation, Writing – original draft. AP: Conceptualization, Supervision, Validation, Writing – original draft. AH: Conceptualization, Writing – review & editing, Supervision. PW: Supervision, Writing – original draft.
Funding
The author(s) declare financial support was received for the research, authorship, and/or publication of this article. This work was partially supported by JSPS Grant-in-Aid for Scientific Research JP18H04102, 22K16923, and 22K10599.
Acknowledgments
We thank Prof Atsushi Konno, Dr. Takashige Abe, Dr. Aya Honma, Ms. Yuu Manabe, Ms. Shizuka Sugawara, Ms. Yuka Masuta, and Ms. Yumiko Kimura for their support in the surgical training course and in the writing of the manuscript. We would also like to thank KARL STORZ, Medtronic, and Stryker for providing the endoscopes, surgical equipment, instruments and the Building Block software.
Conflict of interest
PW: consultant for Fusetec and receiving royalties from Fusetec. AP: consultant for Fusetec, Medtronic, ENT technologies, Tissium, and Aerin Medical, shareholder of Chitogel, and speaker’s bureau for Sequiris.
The remaining authors declare that the research was conducted in the absence of any commercial or financial relationships that could be construed as a potential conflict of interest.
Publisher’s note
All claims expressed in this article are solely those of the authors and do not necessarily represent those of their affiliated organizations, or those of the publisher, the editors and the reviewers. Any product that may be evaluated in this article, or claim that may be made by its manufacturer, is not guaranteed or endorsed by the publisher.
References
1. O'Connor, T, Papanikolaou, V, and Keogh, I. Safe surgery, the human factors approach. Surgeon. (2010) 8:93–5. doi: 10.1016/j.surge.2009.10.004
2. Pearse, RM, Moreno, RP, Bauer, P, Pelosi, P, Metnitz, P, Spies, C, et al. Mortality after surgery in Europe: a 7 day cohort study. Lancet. (2012) 380:1059–65. doi: 10.1016/s0140-6736(12)61148-9
3. Fokkens, WJ, Lund, VJ, Hopkins, C, Hellings, PW, Kern, R, Reitsma, S, et al. European position paper on rhinosinusitis and nasal polyps 2020. Rhinology. (2020) 58:1–464. doi: 10.4193/Rhin20.600
4. Ziv, A, Wolpe, PR, Small, SD, and Glick, S. Simulation-based medical education: an ethical imperative. Acad Med. (2003) 78:783–8. doi: 10.1097/00001888-200308000-00006
5. Bilotta, FF, Werner, SM, Bergese, SD, and Rosa, G. Impact and implementation of simulation-based training for safety. ScientificWorldJournal. (2013) 2013:652956. doi: 10.1155/2013/652956
6. Gogalniceanu, P, Calder, F, Callaghan, C, Sevdalis, N, and Mamode, N. Surgeons are not pilots: is the aviation safety paradigm relevant to modern surgical practice? J Surg Educ. (2021) 78:1393–9. doi: 10.1016/j.jsurg.2021.01.016
7. Collins, JW, and Wisz, P. Training in robotic surgery, replicating the airline industry. How far have we come? World J Urol. (2020) 38:1645–51. doi: 10.1007/s00345-019-02976-4
8. Anastakis, DJ, Regehr, G, Reznick, RK, Cusimano, M, Murnaghan, J, Brown, M, et al. Assessment of technical skills transfer from the bench training model to the human model. Am J Surg. (1999) 177:167–70. doi: 10.1016/s0002-9610(98)00327-4
9. Balak, N, Tsianaka, E, Zoia, C, Sekhar, A, and Ganau, M. Editorial: from simulation to the operating theatre: new insights in translational surgery. Frontiers in medical. Technology. (2023) 5:1282248. doi: 10.3389/fmedt.2023.1282248
10. Langridge, B, Momin, S, Coumbe, B, Woin, E, Griffin, M, and Butler, P. Systematic review of the use of 3-dimensional printing in surgical teaching and assessment. J Surg Educ. (2018) 75:209–21. doi: 10.1016/j.jsurg.2017.06.033
11. Takoutsing, BD, Wunde, UN, Zolo, Y, Endalle, G, Djaowé, DAM, Tatsadjieu, LSN, et al. Assessing the impact of neurosurgery and neuroanatomy simulation using 3d non-cadaveric models amongst selected African medical students. Front Med Technol. (2023) 5:1190096. doi: 10.3389/fmedt.2023.1190096
12. Zahid, F, Memon, A, Siddiqui, M, Deewani, MH, Asif, O, Javer, A, et al. Successful use of a patient specific 3d-printed biomodel as surgical guide for excision of juvenile nasopharyngeal Angiofibroma extending to Skull Base: a case report. Surg Neurol Int. (2024) 15:44. doi: 10.25259/sni_743_2023
13. Shkorbotun, YV, and Liakh, KV. Modern approaches to choosing shaver blade for endoscopic surgery of the nasopharynx and paranasal sinuses using 3d modeling. Wiad Lek. (2022) 75:2646–51. doi: 10.36740/WLek202211117
14. Zhao, K, Kim, K, Craig, JR, and Palmer, JN. Using 3d printed Sinonasal models to visualize and optimize personalized Sinonasal sinus irrigation strategies. Rhinology. (2020) 58:266–72. doi: 10.4193/Rhin19.314
15. Suzuki, M, Miyaji, K, Watanabe, R, Suzuki, T, Matoba, K, Nakazono, A, et al. Repetitive simulation training with novel 3d-printed sinus models for functional endoscopic sinus surgeries. Laryngoscope Investig Otolaryngol. (2022) 7:943–54. doi: 10.1002/lio2.873
16. Suzuki, M, Miyaji, K, Matoba, K, Abe, T, Nakamaru, Y, Watanabe, R, et al. Mental workload during endoscopic sinus surgery is associated with surgeons’ skill levels. Front Med. (2023) 10:743. doi: 10.3389/fmed.2023.1090743
17. Lin, SY, Laeeq, K, Ishii, M, Kim, J, Lane, AP, Reh, D, et al. Development and pilot-testing of a feasible, reliable, and valid operative competency assessment tool for endoscopic sinus surgery. Am J Rhinol Allergy. (2009) 23:354–9. doi: 10.2500/ajra.2009.23.3275
18. Chien, WW, da Cruz, MJ, and Francis, HW. Validation of a 3d-printed human temporal bone model for otology surgical skill training. World J Otorhinolaryngol Head Neck Surg. (2021) 7:88–93. doi: 10.1016/j.wjorl.2020.12.004
19. Da Cruz, MJ, and Francis, HW. Face and content validation of a novel three-dimensional printed temporal bone for surgical skills development. J Laryngol Otol. (2015) 129:S23–9. doi: 10.1017/s0022215115001346
20. Mooney, MA, Cavallo, C, Zhou, JJ, Bohl, MA, Belykh, E, Gandhi, S, et al. Three-dimensional printed models for lateral Skull Base surgical training: anatomy and simulation of the Transtemporal approaches. Oper Neurosurg (Hagerstown). (2020) 18:193–201. doi: 10.1093/ons/opz120
21. Chan, HH, Siewerdsen, JH, Vescan, A, Daly, MJ, Prisman, E, and Irish, JC. 3d rapid prototyping for otolaryngology-head and neck surgery: applications in image-guidance, surgical simulation and patient-specific modeling. PLoS One. (2015) 10:e0136370. doi: 10.1371/journal.pone.0136370
22. Chang, DR, Lin, RP, Bowe, S, Bunegin, L, Weitzel, EK, McMains, KC, et al. Fabrication and validation of a low-cost, medium-Fidelity silicone injection molded endoscopic sinus surgery simulation model. Laryngoscope. (2017) 127:781–6. doi: 10.1002/lary.26370
23. Alrasheed, AS, Nguyen, LHP, Mongeau, L, Funnell, WRJ, and Tewfik, MA. Development and validation of a 3d-printed model of the Ostiomeatal complex and frontal sinus for endoscopic sinus surgery training. Int Forum Allergy Rhinol. (2017) 7:837–41. doi: 10.1002/alr.21960
24. Barber, SR, Jain, S, Son, YJ, and Chang, EH. Virtual functional endoscopic sinus surgery simulation with 3d-printed models for mixed-reality nasal endoscopy. Otolaryngol Head Neck Surg. (2018) 159:933–7. doi: 10.1177/0194599818797586
25. Tai, BL, Wang, AC, Joseph, JR, Wang, PI, Sullivan, SE, McKean, EL, et al. A physical simulator for endoscopic Endonasal drilling techniques: technical note. J Neurosurg. (2016) 124:811–6. doi: 10.3171/2015.3.jns1552
26. Hsieh, TY, Cervenka, B, Dedhia, R, Strong, EB, and Steele, T. Assessment of a patient-specific, 3-dimensionally printed endoscopic sinus and Skull Base surgical model. JAMA Otolaryngol Head Neck Surg. (2018) 144:574–9. doi: 10.1001/jamaoto.2018.0473
27. Zheng, JP, Li, CZ, Chen, GQ, Song, GD, and Zhang, YZ. Three-dimensional printed Skull Base simulation for Transnasal endoscopic surgical training. World Neurosurg. (2018) 111:e773–82. doi: 10.1016/j.wneu.2017.12.169
28. Ding, CY, Yi, XH, Jiang, CZ, Xu, H, Yan, XR, Zhang, YL, et al. Development and validation of a multi-color model using 3-dimensional printing Technology for Endoscopic Endonasal Surgical Training. Am J Transl Res. (2019) 11:1040–8.
29. Mowry, SE, Jabbour, N, Rose, AS, Wiet, GJ, Svrakic, M, Zopf, DA, et al. Multi-institutional comparison of temporal bone models: a collaboration of the Aao-Hnsf 3d-printed temporal bone working group. Otolaryngol Head Neck Surg. (2021) 164:1077–84. doi: 10.1177/0194599820960474
30. Gadaleta, DJ, Huang, D, Rankin, N, Hsue, V, Sakkal, M, Bovenzi, C, et al. 3d printed temporal bone as a tool for Otologic surgery simulation. Am J Otolaryngol. (2020) 41:102273. doi: 10.1016/j.amjoto.2019.08.004
31. Rose, AS, Kimbell, JS, Webster, CE, Harrysson, OL, Formeister, EJ, and Buchman, CA. Multi-material 3d models for temporal bone surgical simulation. Ann Otol Rhinol Laryngol. (2015) 124:528–36. doi: 10.1177/0003489415570937
32. Alwani, MM, Svenstrup, TJ, Bandali, EH, Sharma, D, Higgins, TS, Wu, AW, et al. Validity testing of a three-dimensionally printed endoscopic Sinonasal surgery simulator. Laryngoscope. (2020) 130:2748–53. doi: 10.1002/lary.28356
33. Zdilla, MJ, and Balta, JY. Human body donation and surgical training: a narrative review with global perspectives. Anat Sci Int. (2022) 98:1–11. doi: 10.1007/s12565-022-00689-0
34. Rai, R, Shereen, R, Protas, M, Greaney, C, Brooks, KN, Iwanaga, J, et al. Social media and cadaveric dissection: a survey study. Clin Anat. (2019) 32:1033–41. doi: 10.1002/ca.23421
35. Suzuki, M, Vyscocil, E, Ogi, K, Matoba, K, Nakamaru, Y, Homma, A, et al. Remote training of functional endoscopic sinus surgery with advanced manufactured 3d sinus models and a telemedicine system. Front Surg. (2021) 8:746837. doi: 10.3389/fsurg.2021.746837
Keywords: surgical education, 3D printer, cadaver surgery, surgical training, endoscopic surgery
Citation: Suzuki M, Watanabe R, Nakazono A, Nakamaru Y, Suzuki T, Kimura S, Matoba K, Murakami M, Hinder D, Psaltis AJ, Homma A and Wormald PJ (2024) Can high-fidelity 3D models be a good alternative for cadaveric materials in skill assessment for endoscopic sinus surgery? A comparison study in assessment for surgical performance in 3D models and cadavers. Front. Med. 11:1301511. doi: 10.3389/fmed.2024.1301511
Edited by:
Jacqueline G. Bloomfield, The University of Sydney, AustraliaReviewed by:
Gionata Fragomeni, Magna Græcia University, ItalyMario Ganau, Oxford University Hospitals NHS Trust, United Kingdom
Copyright © 2024 Suzuki, Watanabe, Nakazono, Nakamaru, Suzuki, Kimura, Matoba, Murakami, Hinder, Psaltis, Homma and Wormald. This is an open-access article distributed under the terms of the Creative Commons Attribution License (CC BY). The use, distribution or reproduction in other forums is permitted, provided the original author(s) and the copyright owner(s) are credited and that the original publication in this journal is cited, in accordance with accepted academic practice. No use, distribution or reproduction is permitted which does not comply with these terms.
*Correspondence: Masanobu Suzuki, bWFzYW5vYnV3b3JrQHlhaG9vLmNvLmpw